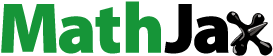
Abstract
Background
Thermal dose in clinical hyperthermia reported as cumulative equivalent minutes (CEM) at 43 °C (CEM43) and its variants are based on direct thermal cytotoxicity assuming Arrhenius ‘break’ at 43 °C. An alternative method centered on the actual time–temperature plot during each hyperthermia session and its prognostic feasibility is explored.
Methods and materials
Patients with bladder cancer treated with weekly deep hyperthermia followed by radiotherapy were evaluated. From intravesical temperature (T) recordings obtained every 10 secs, the area under the curve (AUC) was computed for each session for T > 37 °C (AUC > 37 °C) and T ≥ 39 °C (AUC ≥ 39 °C). These along with CEM43, CEM43(>37 °C), CEM43(≥39 °C), Tmean, Tmin and Tmax were evaluated for bladder tumor control.
Results
Seventy-four hyperthermia sessions were delivered in 18 patients (median: 4 sessions/patient). Two patients failed in the bladder. For both individual and summated hyperthermia sessions, the Tmean, CEM43, CEM43(>37 °C), CEM43(≥39 °C), AUC > 37 °C and AUC ≥ 39 °C were significantly lower in patients who had a local relapse. Individual AUC ≥ 39 °C for patients with/without local bladder failure were 105.9 ± 58.3 °C-min and 177.9 ± 58.0 °C-min, respectively (p = 0.01). Corresponding summated AUC ≥ 39 °C were 423.7 ± 27.8 °C-min vs. 734.1 ± 194.6 °C-min (p < 0.001), respectively. The median AUC ≥ 39 °C for each hyperthermia session in patients with bladder tumor control was 190 °C-min.
Conclusion
AUC ≥ 39 °C for each hyperthermia session represents the cumulative time–temperature distribution at clinically defined moderate hyperthermia in the range of 39 °C to 45 °C. It is a simple, mathematically computable parameter without any prior assumptions and appears to predict treatment outcome as evident from this study. However, its predictive ability as a thermal dose parameter merits further evaluation in a larger patient cohort.
Introduction
Reporting hyperthermia (HT) treatments in clinical practice using a common denominator is a matter of active deliberation. Thermal dose, first proposed in 1984 by Sapareto and Dewey, is based on the concept of the cumulative equivalent of minutes at 43 °C (CEM43) [Citation1]. Even today, the thermal dose is expressed as CEM43 or its variants were in the temperatures (T), T90, T50, T10 or a suitable index temperature have been integrated with CEM43 [Citation2–5]. These have been incorporated in the CEM43 expression to account for the substantial heterogeneity in the temperatures attained in in vivo conditions (both experimental and clinical) in contrast to uniformly controlled temperature achievable in in vitro cell culture studies. T90, T50, T10 are the index temperatures chosen at which 90%, 50% and 10% of the measured temperature points would exceed and denoted as CEM43T90, CEM43T50, CEM43T10, respectively [Citation2,Citation6]. Clinical outcomes are thus reported variably using maximum (Tmax), minimum (Tmin) and mean (Tmean) temperatures, HT duration with or without CEM43 [Citation3,Citation4,Citation7–18].
CEM43 converts all thermal exposures to ‘equivalent minutes’ at 43 °C. Based on the biphasic Arrhenius plots, the value of the constant ‘R’ is assumed to be 0.50 for T > 43 °C and 0.25 for T ≤ 43 °C [Citation1,Citation2,Citation5]. As stated by Sapareto and Dewey, the choice of reference temperature of 43 °C was ‘arbitrary’. This was intended to reflect the heat-induced cell lethality caused by protein denaturation and aggregation represented in the Arrhenius ‘break’ at 43 °C [Citation1,Citation2]. Thus, CEM43 represents the direct cytotoxicity effect of heat and is a normalizing method to convert the various time–temperature events into a consolidated expression of equivalent exposure time [Citation5,Citation19].
Conceptually, CEM43 does not take into account the composite effects of HT relevant in the clinical context. In clinics, HT is seldom used alone. It is usually applied with radiotherapy (RT) and/or chemotherapy (CT) [Citation20]. Furthermore, temperatures attained during clinical mild to moderate HT rarely exceed 43 °C. HT is known to be one of the most potent radiosensitizers through its favorable effects on perfusion, enhanced oxygenation, reduced repair of RT induced DNA damages and immunomodulation [Citation21–23]. All these are evident even at T < 43 °C, usually achieved in clinical practice [Citation5,Citation6,Citation24]. Thus, the Kadota Fund International Forum defines moderate HT as a temperature in the range of 39 °C to 45 °C to consider the cumulative thermodynamic and immunomodulatory effects of moderate HT in this temperature range [Citation25]. A clinical thermal dose parameter ideally should therefore take into consideration the impact of thermal-induced changes between 39 °C and 45 °C.
The present study, examines the actual time–temperature plots by computing the area under the curve (AUC) during each HT session for T > 37 °C and T ≥ 39 °C. The original definition of CEM43 has no lower cutoff for temperature [Citation1]. On many occasions, as was evident in this study, the initial intravesical temperatures were even <37 °C. Furthermore, temperatures between 37 °C and 38.9 °C, which are below the lower limit of moderate hyperthermia would also contribute to the computation of CEM 43 values. Thus, it would be interesting to examine the AUC for each session for T > 37 °C and T ≥ 39 °C and compare them with CEM43 and the corresponding CEM43 > 37 °C and CEM43 ≥ 39 °C to assess the prognostic values of each of these parameters in muscle-invasive bladder cancers (MIBC) being treated with HT and RT (HTRT).
Material and methods
Patient population
Between December 2012 and December 2019, 21 consecutive patients with MIBC with associated comorbidities that rendered them unfit for radical surgery or intensive chemoradiotherapy (CTRT) or those who refused these interventions were considered for bladder preservation treatment with HTRT following transurethral resection of bladder tumor (TURBT). All patients underwent a detailed workup before initiating treatment and also during the follow-up as has been stated in our earlier publication [Citation17]. All these patients were initially considered for an ongoing study protocol of CTRT and HT in MIBC, approved by the Kantonale Ethics Commission (EK-Number: 2011/076). All patients were treated in accordance with the Declaration of Helsinki.
Of the 21 patients, two had received neoadjuvant CT before HTRT while one declined treatment midway. These three patients were excluded from the present analysis. Thus, 18 patients who completed the assigned HTRT treatment were available for this study.
Radiation therapy
The details of target volume delineation and RT dose schedules have been summarized in an earlier report [Citation17]. Briefly, patients with unifocal tumors (n = 7) received RT to the empty bladder to a dose of 36 Gy in 12 fractions (3 fractions/week). Meanwhile, a 12 Gy boost in 4 fractions (once a week) was applied to the tumor bed with a partially filled bladder to a total dose of 48 Gy in 16 fractions (4 fractions/week). Those with multifocal tumors (n = 11) received 50 Gy in 20 fractions to the entire empty bladder (5 fractions/week) (). Assuming a tumor α/β of 10 Gy, the estimated biological equivalent dose was 62.4 Gy10 and 62.5 Gy10 for unifocal and multifocal tumors, respectively. All patients received HT prior to RT as discussed below. The RT dose schedules for unifocal and multifocal tumors were chosen to keep the overall treatment time in these elderly patients below 4 weeks and deliver a biological equivalent dose of more than 60 Gy10. The treatment schedules were modified and adapted from the previously reported studies of Piet et al. [Citation26] and Turgeon et al. [Citation27] for unifocal and multifocal MIBCs, respectively.
Figure 1. Schematic representation of the radiotherapy and hyperthermia schedules in unifocal and multifocal muscle invasive bladder cancers (Reproduced with permission from Datta et al. [Citation17]).
![Figure 1. Schematic representation of the radiotherapy and hyperthermia schedules in unifocal and multifocal muscle invasive bladder cancers (Reproduced with permission from Datta et al. [Citation17]).](/cms/asset/dc9e4413-3c6b-4b18-a85e-7b463cea143a/ihyt_a_1875060_f0001_c.jpg)
Hyperthermia
HT was delivered once weekly and RT was performed within 15–20 min of completion of HT. Deep HT was delivered using a BSD-2000 with Sigma-60 or Sigma-Eye phased array applicator (Pyrexar Medical, USA, formerly BSD Medical Corporation, USA) in accordance with the European Society of Hyperthermic Oncology (ESHO) quality guidelines [Citation28]. HT planning for these tumors has been detailed in a previous publication [Citation17]. Before each HT session, a flexible thermometry probe was guided through an indwelling Foley catheter and placed within the bladder. Time and temperature were recorded every 10 s during the entire HT session consisting of 30 min of pre-heating followed by 60 min of active heating. The power settings, antenna phase, and frequency were set to not exceed a temperature of 43 °C in the bladder, rectum or vagina. These were also adjusted from time-to-time to conform to the patient’s tolerance of HT during the procedure. Temperature readings were also taken during the entire procedure in the rectum, groins, gluteal fold, vagina (in females) and skin. Systemic body temperature, pulse rate, oxygen saturation and blood pressure were monitored as and when required during HT.
Response evaluation and toxicity scoring
On completion of HTRT, patients were followed up every 3 months for the first 2 years following treatment and every 6 months thereafter. The local tumor status in the bladder was evaluated at each follow-up using urine cytology and cystoscopy with or without biopsies. Outcomes were recorded as per the Response Evaluation Criteria for Solid Tumors (RECIST) v1.1 [Citation29]. Acute and late morbidities were monitored and scored according to the Common Terminology Criteria for Adverse Events (CTCAE), v4.03 [Citation30].
Estimation of the time–temperature area under the curve (AUC)
The output of the time–temperature recordings at every 10 s were extracted from the HT treatment unit and were used to compute the AUC using the linear trapezoidal method [Citation31]. This consists of a linear interpolation between data points of time, t (X-axis) and temperature, T (Y-axis). For a given time interval, t1 − t2, and corresponding temperature difference, T1 − T2, the AUC for that specific trapezoidal segment would be as follows:
Thus, for each HT session, the summated AUC for the entire treatment session including pre-heating and active heating was computed as follows:
where, t is the time (in minutes) and T is temperature (in °C), Tn is the temperature at time instant tn, Tn−1 is the temperature at time instant tn−1, n = 1, 2, 3, …, N indicates discrete-time indices at which temperatures are recorded and N indicates the final time index of the heating session.
For mild to moderate HT, the temperature range ≥39 °C, the summated AUC was computed for T > 37 °C (AUC >37 °C) to represent any rise above the normal body (AUC ≥ 39 °C) to represent moderate HT would be of interest (). For AUC > 37 °C, n = 1, when T > 37 °C and for AUC ≥ 39 °C, n = 1, when T > 38.9 °C, assuming the resolution of temperature recording at 0.1 °C. Thus, AUC > 37 °C and AUC ≥ 39 °C were computed as follows:
and,
Figure 2. A representative time–temperature plot from a patient of urinary bladder cancer undergoing hyperthermia. Temperature represents the intraluminal temperature in bladder at every 10 s during the entire 90 min of deep hyperthermia. The area under the curve (AUC) for >37 °C (AUC > 37 °C) and ≥39 °C (AUC ≥ 39 °C) represents the corresponding areas enclosed within these temperatures.
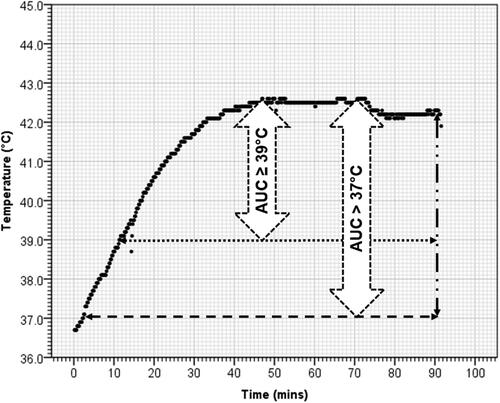
Estimation of the CEM43 and corresponding values for T > 37 °C and T ≥ 39 °C
The CEM43 values were estimated as proposed by Sapareto and Dewey [Citation1] as follows:
where T is the temperature (in °C), t is the time (in minutes) and R = 0.25 for T ≤ 43 °C while R = 0.50 for T > 43 °C. As with AUCs, the corresponding CEM43 for T > 37 °C [CEM43(>37 °C)] and T ≥ 39 °C [CEM43(≥39 °C)] were computed as follows:
and,
Statistical analysis
Statistical analysis was performed using IBM SPSS Statistic v24. To test the significance of the difference between patients who were free of any local disease in the bladder, a χ2 test was used for categorical variables, while a t-test was performed for continuous variables.
Results
Patient demographics and outcome
The demographic features of the 18 patients are summarized in . Their age ranged between 52 and 88 years and 66.6% of these patients had a Karnosfsky Performance Status (KPS) below 80 due to age and associated comorbid conditions. Seven patients had solitary tumors. 16 of the 18 patients achieved an R0 status following TURBT. Most of the patients had T2 tumors (n = 14) while 16 patients had N0 nodal status. Of these parameters, only age (p = 0.026) and KPS (p = 0.049) were significantly different between patients with or without local bladder failure.
Table 1. Patient demography: all evaluable patients, patients with or without local failure in bladder at follow-up.
All patients completed their prescribed treatment with a mean overall treatment time of 27.2 days (SD: ±3.9). The RT dose ranged from 48–50 Gy (mean ± SD: 49.2 ± 1.0). 15 of the 18 patients received four HT sessions while three received five sessions. Thus, 75 HT sessions were delivered. There was no significant difference in the number of HT sessions received by those with/without local bladder failure. As the record of one HT session was missing for one patient, a total of 74 HT sessions were available for analysis as detailed in Supplementary Table 1. The summated values of the various thermal dose parameters of each patient are given in .
Table 2. Details of the thermal dose parameters for summated hyperthermia sessions – AUC >37 °C, AUC≥ 39 °C, CEM 43, CEM43 (>37 °C), CEM 43(≥ 39 °C), Tmean, Tmax, Tmin, duration of hyperthermia session, radiotherapy dose and the local disease status for each patient.
During the 74 HT sessions, the mean duration of HT was 92.5 min (SD: ±10.4) and the mean ± SD of Tmean, Tmin and Tmax were 40.5 ± 0.7 °C, 36.7 ± 0.5 °C and 42.1 ± 0.8 °C, respectively. Considering the summated HT sessions in individual patients, the corresponding Tmean, Tmin and Tmax in 18 patients were 40.5 ± 0.5 °C, 36.7 ± 0.3 °C and 42.0 ± 0.6 °C, respectively (). The Tmean of 74 individual hyperthermia applications ranged from 38.9 to 42.1 °C while the maximum temperatures attained ranged from 39.7 to 43.7 °C (median: 42.1 °C) Although every endeavor was made to reach and maintain a temperature of 41 °C to 42.5 °C, the power settings had to be adapted to the heat tolerance of these elderly patients, which at times did not allow us to reach the desired temperature. The mean total duration of HT in these patients was 380.1 min (SD: ±43.54).
Table 3. Hyperthermia treatment characteristics both for individual and combined hyperthermia sessions of all patients and those with or without local failure in bladder at follow-up.
Follow-up of the patients ranged between 4 and 91 months (median: 19 months). 16 patients were free of disease in the bladder as confirmed through periodic cystoscopy with/without biopsies until their last follow-up or death. Of the 13 patients without any evidence of disease, eight have died due to other unrelated causes while five are still alive at 9 to 38 months. One patient who failed in the pelvic nodes has died. Of the two patients with isolated distant metastasis, one is dead and the other is alive at 65 months.
Two patients who failed exclusively in the bladder had solitary tumors at presentation. Both had achieved R0 status on TURBT resection prior to HTRT. Their recurrences were confirmed on cystoscopic biopsies. One of them, who recurred at 19 months was successfully treated with BCG instillation and is still alive at 39 months. The other patient developed local recurrence at 10 months following HTRT. He underwent a salvage TURBT and died at 19 months due to an unrelated cause.
AUC > 37 °C and AUC ≥ 39 °C: patients with or without local failure
The time–temperature plots were different in patients with local control compared to the two patients who had a recurrence in the bladder (). A paired t-test revealed that the computed AUC > 37 °C and AUC ≥ 39 °C values were significantly different – both for 74 individual sessions and summated sessions in 18 patients (both p < 0.001). One sample t-test revealed a significant difference in the AUC > 37 °C and also AUC ≥ 39 °C for individual HT sessions and also the summated HT sessions in 18 patients (all p < 0.001). The AUC >37 °C for the 74 sessions ranged between 134.8 and 465.2 °C-min (338.3 ± 69.2) (p < 0.001) and was significantly higher in patients without failure in the bladder (p = 0.018) (). AUC ≥ 39 °C for all 74 sessions varied between 28.4 and 286.7 °C-min and was also significantly lower in patients who failed in the bladder (p = 0.01). On assessing the combined AUCs of all the HT sessions in 18 patients, both the AUC > 37 °C and AUC ≥ 39 °C were significantly lower in patients who failed in the bladder (both p < 0.001) (, ).
Figure 3. Time–temperature plots for each of the four hyperthermia sessions in a patient who had (a–d) local bladder tumor control and (e–h) who had local bladder tumor failure. Corresponding CEM43 (≥39 °C) and AUC ≥ 39 °C for each of these sessions are stated. The graphs correspond to patient number 15 (a–d) who had no local failure and patient nos. 14 (e–h) who had local failure. The details of the CEM and AUC values for these patients are given in Supplementary Table 1.
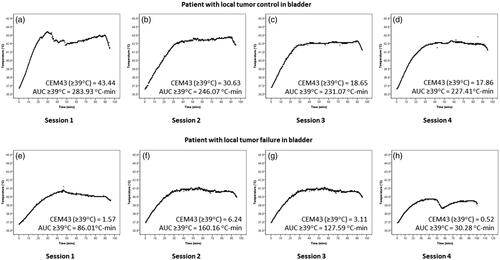
Figure 4. Box plots and superimposed scatter plots of AUC > 37 °C, AUC ≥ 39 °C, CEM43(>37 °C) and CEM43(≥39 °C) in patients with or without local failure in bladder (a) Individual hyperthermia sessions (n = 74) [AUC > 37 °C, p = 0.018; AUC ≥ 39 °C, p = 0.01; CEM43(>37 °C), p < 0.001 and CEM43(≥39 °C), p < 0.001)] and (b) Summated hyperthermia sessions (n = 18) [AUC > 37 °C, p < 0.001; AUC ≥ 39 °C, p < 0.001; CEM43(>37 °C), p < 0.001 and CEM43(≥39 °C), p < 0.001].
![Figure 4. Box plots and superimposed scatter plots of AUC > 37 °C, AUC ≥ 39 °C, CEM43(>37 °C) and CEM43(≥39 °C) in patients with or without local failure in bladder (a) Individual hyperthermia sessions (n = 74) [AUC > 37 °C, p = 0.018; AUC ≥ 39 °C, p = 0.01; CEM43(>37 °C), p < 0.001 and CEM43(≥39 °C), p < 0.001)] and (b) Summated hyperthermia sessions (n = 18) [AUC > 37 °C, p < 0.001; AUC ≥ 39 °C, p < 0.001; CEM43(>37 °C), p < 0.001 and CEM43(≥39 °C), p < 0.001].](/cms/asset/8fe47b99-ec4b-45c5-b15f-5e507363dcd7/ihyt_a_1875060_f0004_c.jpg)
CEM43, CEM43 (>37 °C) and CEM43 (≥39 °C): patients with or without failure
The differences in CEM43, CEM43 (>37 °C) and CEM43 (≥39 °C) for both the 74 individual HT sessions and combined HT sessions in 18 patients were tested using paired sample t-test. For both individual and summated HT sessions, no significant differences between CEM43 vs. CEM43(>37 °C) was observed (mean difference ± SD: for individual sessions = 0.0004 ± 0.0019, p: ns; for summated HT session = 0.001 ± 0.003, p: ns). For CEM43 vs. CEM43 (≥39 °C) and CEM43 (>37 °C) vs. CEM43 (≥39 °C), although the differences were significant for individual and summated HT sessions, quantitatively, the mean differences were minimal [For CEM43 vs. CEM43 (≥39 °C): individual sessions = 0.018 ± 0.011, p < 0.001; summated HT session = 0.075 ± 0.022, p < 0.001; For CEM43 (>37 °C) vs. CEM43 (≥39 °C): individual sessions = 0.018 ± 0.011, p < 0.001; summated HT session = 0.074 ± 0.023, p < 0.001)]. This was in contrast to the mean differences observed with both AUC >37 °C vs AUC ≥ 39 °C values (individual session mean difference: 168.1 ± 15.8, p < 0.001, summated session mean difference: 691.3 ± 71.0, p < 0.001).
The mean CEM43, CEM43(>37 °C) vs. CEM43(≥39 °C) values in 74 individual HT sessions in patients with local failure were identical at 3.5 (SD: ±3.0) while in those without failure it was 15.9 (SD: ±13.9). However, this difference between the two groups of patients (with/without local failure) was significant for each of these parameters (all p < 0.001) (, ). For combined sessions, the differences in these values were significant but similar (all p < 0.001). Other parameters including Tmean, Tmax, Tmin and HT treatment time were also compared between the two groups of patients. Of these, Tmean was the only consistent parameter, being significantly greater in patients with no local bladder failure for both individual and summated HT sessions ().
Discussion
Determination of an appropriate parameter to define the thermal dose has been one of the major bottlenecks for uniform reporting of clinical HT at moderate temperatures of 39 °C to 45 °C. This has led to a guarded skepticism amongst clinicians to accept HT as a therapeutic modality along with RT and/or CT. HT has multifaceted therapeutic benefits including potent radiosensitization, chemosensitization and immunomodulation without significant additional acute or late morbidities [Citation21,Citation23]. A number of phase III randomized trials, pairwise meta-analyses and network meta-analyses have shown the efficacy of HT in a wide range of malignancies [Citation7,Citation8,Citation20,Citation32–36]. Thermoradiobiologically, HT with photons is analogous to that of neutrons but devoid of any added acute or late morbidity while with protons it mimics 12C beam therapy [Citation37–40]. In phase III randomized study, HT with CT has improved survival outcomes in soft tissue sarcoma [Citation41]. Further, the immunomodulating effects of HT with RT is akin to ‘in situ tumor vaccination’ and is an area of active research [Citation22,Citation42–45]. Moderate HT thus exerts its effect at physiological, cellular, and molecular levels within the range of 39 °C to 45 °C, not just limited to a specific temperature [Citation5,Citation24,Citation25].
Limitations of CEM43
The initial attempts to define and determine thermal dose lead to the proposal of CEM43 by Sapareto and Dewey in 1984 using an arbitrary temperature of 43 °C to normalize and convert time–temperature exposures to an equivalent time [Citation1]. This was based on cell culture experiments conducted with heat alone, mainly on non-human cell lines with virtually no supportive data directly related to the thermal sensitivity of human tissues [Citation46]. Moreover, CEM43 was not supposed to predict, nor was it required that different tissues would exhibit similar heat sensitivity [Citation1]. Nevertheless, CEM43 and its variants, like CEM43T90 have been used to report outcomes in both animal sarcomas and patients with mixed results [Citation9–11,Citation47,Citation48].
Efforts were made in the past to attain 43 °C in patients during an HT session, as an analogy to cell culture experiments. However, the reference temperature of 43 °C in tumors is difficult to achieve in clinical settings. Dewhirst et al. [Citation24] proposed a pragmatic approach of ‘Re-setting the biologic rationale for thermal therapy’ Jones et al. [Citation3] observed, ‘One disservice that the CEM 43 °C concept did for the hyperthermia community, however, was to foster the view that 43 °C was a treatment goal and that inability to achieve this temperature meant that treatment had failed’. Furthermore, the value of the constant ‘R’ in the CEM43 was chosen without consideration of the radiosensitizing capability of HT, as HT in clinics is seldom advocated as a sole therapeutic modality, but is always used in conjunction with RT and/or CT. Thus, the utility of CEM43 and its variants continues to be debated and its acceptance as a standard thermal dose parameter is yet to garner general acceptance within the HT community [Citation5,Citation19].
Juang et al. [Citation12] discussed thermal dosimetry characteristics in noninvasive bladder cancer in 14 patients, treated with external deep HT and mitomycin-C. A mean thermal dose of 21.3 CEM43 (range: 0.6–80 CEM43) was achieved with an average of 61.9 min of heating. In their subsequent report [Citation49], 67% of the patients recurred with a median time to recurrence of 15.4 months. Correlation of the recurrences with the corresponding thermal dose could not be ascertained from their publication.
Alternative thermal dose parameters
Sherar et al. [Citation50] studied the impact of thermal dose on the outcome with HTRT in recurrent breast cancers. They tested five thermal parameters associated with low regions of the measured temperature distributions and reported that parameters representative of the low end of measured temperature distributions, namely sum of the minimum tumor dose summed over a series of treatments in a particular patient, SumTD(min) were significantly associated with initial complete response (77% for Sum(TDmin) >10 min vs 43% for Sum(TDmin) ≤ 10 min, p = 0.022), local disease free survival, time to local failure and overall survival.
One of the other alternatives is to consider temperature as Tmean, Tmax, Tmin or a suitable percentile. T90, T50 and T10 have been proposed in the European Society of Hyperthermic Oncology (ESHO) guidelines for superficial tumors [Citation10,Citation13,Citation16,Citation51]. Franckena et al. [Citation14] proposed a thermal dose parameter, TRISE, which they defined as a product of T50 rise >37 °C during HT with the duration of treatment for all sessions and normalized to 450 min, the scheduled total treatment time for all patients of cancer cervix. In their initial report, the mean intraluminal temperature (ALT50) for patients with or without failure was similar at 40.5 °C and 40.6 °C, respectively [Citation14]. However, in their recent publication [Citation15], they observed that both CEM43T90 and TRISE had a significant effect on local control. TRISE is certainly a step beyond normalization to a specific temperature as CEM43. The confirmation of the correlation in an independent separate patient cohort by Kroesen et al. [Citation15] is unique. In this respect, the close resemblance of TRISE with AUC can be considered positive support for the correlation reported in this manuscript for AUC and local control.
Leopold et al. [Citation4] reported that the number of cumulative minutes with T90 greater than an index temperature of >40 °C was predictive of tumor response in superficial tumors treated with HT and RT. Other variables like mean Tmin, median Tmean, median T50 and number of HT treatments did not significantly influence the tumor response.
AUC and its implications
The AUC concept applied in this study is based on the premise that it captures comprehensively the thermoradiobiological events related to HT and its radiosensitization in the entire temperature range of moderate HT. Thus, a select group of patients who had successfully completed their planned treatment was chosen to evaluate the implications of AUC. The intravesical temperature has been shown to correlate well with intratumoral temperatures in bladder tumors [Citation18]. Thus, all temperature measurements in this study were based using intravesical thermometry. Moreover, as all 18 patients had TURBT resection prior to commencement of HTRT, with 16 of them achieving an R0 status, the intravesical temperature recorded could be expected to correspond to tumor bed temperatures. This is in contrast to the solid tumors, where for a realistic and composite assessment of temperature distribution, one would either need noninvasive thermometry using MRI or multiple invasive probes, a major limitation in patients [Citation52].
Both AUC > 37 °C and AUC ≥ 39 °C evaluated the time–temperature effects beyond normothermia. However, AUC ≥ 39 °C was primarily considered as it would better reflect the temperature range of moderate HT. Moreover, AUC ≥ 39 °C also reflects the true time–temperature effect and does not incorporate any unknown variable/constant requiring assumptions derived from in vitro studies.
The analysis also reveals that although CEM43 values were significantly different between those with/without local bladder failures, the magnitude of difference was minimal between CEM43, CEM43(>37 °C) and CEM43(≥39 °C) compared to AUC > 37 °C and AUC ≥ 39 °C values (). A closer perusal of the relation between the temperature vs CEM43 shows that the slope of the CEM43 curve at temperatures 39 °C to 42 °C is minimal (). It only starts to rise after 42 °C and a clear change is evident at the ‘breakup’ temperature of 43 °C. In contrast, the AUC values show a steady linear rise over the entire temperature range ().
Figure 5. (a) CEM43 (≥39 °C) values for the temperature range 39–45 °C (r2 = 0.999). The slope for changes in CEM43(≥39 °C) values for temperature ranging between 39 °C and 41.5 °C is minimal. The slope changes abruptly at 43 °C due to change of the value of constant ‘R’ from 0.25 for T ≤ 43 °C and 0.50 for T > 43 °C (indicated by black filled arrow). (b) AUC ≥ 39 °C values for the temperature range 39–45 °C (r2 = 1.000). For both CEM43(≥39 °C) and AUC ≥ 39 °C, duration of hyperthermia beyond 39 °C is considered for 60 min to mimic the hyperthermia treatment in clinical situation beyond the initial heating phase.
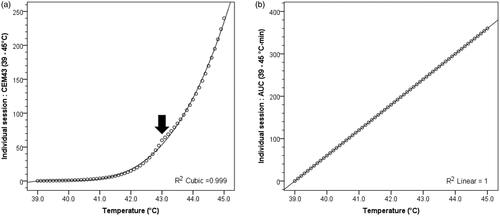
On comparing CEM43(>37 °C) and CEM43(≥39 °C) with the corresponding AUC > 37 °C and AUC ≥ 39 °C for patients with or without failures for individual HT treatments, it is evident that those who failed attained lower end of AUC and CEM values (). The dispersion of individual AUC values is greater relative to the CEM values. This could indicate relatively higher sensitivity of AUC compared to CEM43. The summated values of AUC and CEM for all sessions show that the patients who failed also occupied the lowest end of the scatter plots representing the least values of AUC and CEMs (). In addition, there were 3 patients who even with lower values experienced no local failures. Of these, for one patient the AUC and CEM values from 3 of the 4 HT sessions were computed as the details of the 4th session were missing. Thus, the actual AUC and CEM values in this patient would be higher than those represented in the plots. The 2nd patient, although free of local disease in the bladder, died due to distant failure and also had suspected pelvic nodal failure. The 3rd patient continues to remain free of disease even with lower AUC and CEM values. Thus, although the plots indicate that lower AUC and CEM values, both for individual and summated sessions, could have a prognostic value in terms of local disease control, these observations require further confirmation using a larger sample size.
Figure 6. AUC and CEM43 values for patients with or without local bladder failure. (a) CEM43(>37 °C) vs AUC > 37 °C for each hyperthermia session; (b) CEM43(≥39 °C) vs AUC ≥ 39 °C for each hyperthermia session; (c) Summated CEM43(>37 °C) vs AUC > 37 °C for all hyperthermia sessions; (d) summated CEM43(≥39 °C) vs AUC ≥ 39 °C for all hyperthermia sessions.
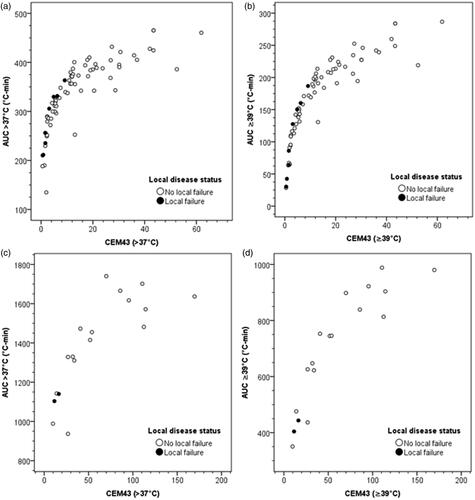
The median AUC ≥ 39 °C of each HT session for patients with no local disease was 190 °C-min compared to 106 °C-min for those with failures (p = 0.018). This includes 30 min of pre-heating and 60 min of active heating. The time to attain a temperature of 39 °C during each HT session varied between 8.5 and 27.2 min (median: 14.8 min). At the end of 30 min pre-heating, a median intravesical temperature of 40.6 °C (range: 38.4 °C to 43.4 °C) was achieved. There was no significant difference in the time taken to reach 39 °C in patients with/without local failure. However, the mean difference of temperature reached at end of 30 min of pre-heating was significantly lower in patients who failed in the bladder (with or without failure: 40.1 ± 0.7 °C vs. 40.7 ± 0.9 °C, p = 0.04). In addition, the temperature attained at the end of 60 min of active heating (i.e., at the end of 90 min of HT) was also significantly lower in patients who had failed locally (with or without failure: 40.5 ± 0.7 °C vs. 41.7 ± 0.8 °C, p = 0.002).
A closer perusal of the HT treatment records of the two patients who failed revealed that both patients had experienced extreme discomfort during their heating sessions. This required reduction in the power output of the HT unit. In one, the bladder had to be also partially emptied during the active phase of heating, resulting in lowering the intravesical temperature. However, the HT duration in both cases for each of the four sessions was around 90 min. These practical issues highlight that although one would always try to aim for the desired temperatures during an HT delivery, an individual patient’s tolerance needs to be respected, and this at times could lead to inadequate heating.
It would be too ambitious to propose an optimal cutoff value for AUC ≥ 39 °C in bladder HT. However, as evident from this study, AUC ≥ 39 °C of 190 °C-min should be targeted in bladder cancers treated with weekly HT and RT. Thus, to achieve a target AUC ≥ 39 °C of 190 °C-min, it is imperative to estimate the temperature to be attained and maintained during the active heating phase after reaching 39 °C in the pre-heating phase. Depending on the time taken to reach 39 °C during each pre-heating session, the temperature required to be achieved and maintained during active heating phase can be expressed as, where ΔT is the additional temperature required beyond 39 °C and x, is the time (in minutes) to attain 39 °C in the pre-heating phase (). The temperatures to be achieved and maintained during the active heating phase could be computed from the above relation and are listed in . As evident from , the lesser the time taken to reach 39 °C, the lesser would be the additional temperature required to be maintained during the active heating phase. One of the possible ways to achieve this could be a pretreatment hyperthermia planning that could guide the operator with determined adequate phase settings, heating ability analysis, hot spot suppression, applicator selection, evaluation of target coverage, heating depth, and predicting possible thermal toxicity. This could help in optimizing the thermal delivery. However real-time thermometry and auto modulation during active treatment could ensure better patient compliance and tolerance during the actual heating session. Together these could help reach as close as possible to the desired temperature, respecting the patient’s heat tolerance.
Figure 7. Computation of the additional temperature that is needed to be acquired during the active heating phase of 60 min following attaining 39 °C during the preheating phase. The target AUC ≥ 39 °C to be achieved during each hyperthermia session is 190 °C-min.
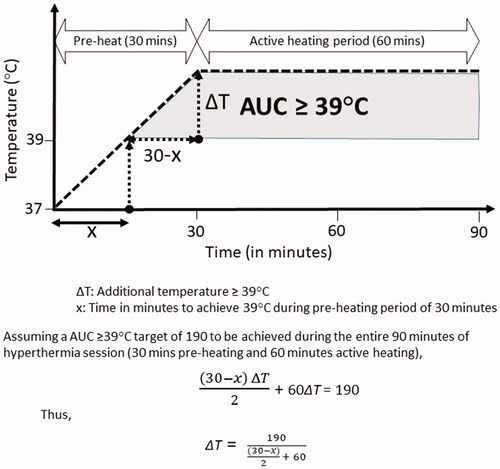
Table 4. Temperature required to be achieved during the active heating phase of 60 min following 30 min of preheating to achieve a target of AUC ≥ 39 °C.
The data of 18 patients were collected over a period of 7 years with a median follow-up of 19 months. Only those who had been treated with HT and RT alone and had completed the planned treatment were selected to minimize the impact of any other extraneous factors that might be influencing the local failure outcomes. Thus, the outcomes observed in these patients could be solely ascribed to insufficient HT delivery resulting in local failure as was reflected in the AUC ≥ 39 °C and CEM43 values. The study provides an insight into the thermal dose quantification and its impact on local tumor response. Although the findings provide a realistic solution toward quantification of thermal dose, this concept needs further evaluation in a larger patient cohort.
Limitations of the study and future endeavors
One of the limitations of this study is the number of patients (n = 18) of which only 2 of these failed locally. In view of the limited number of patients in this study, it would not have been appropriate to carry out a logistic regression to identify the predictive thermal parameter that could be considered with certainty for reporting thermal dose in clinical hyperthermia. Although, in one way, this study demonstrates the efficacy of HTRT in MIBCs, on the other hand the limited patient number needs a careful interpretation of the significance of AUC. Our observations could therefore be considered nearly as anecdotal and it is therefore highly desirable that the implication of AUC should be explored in prospective studies using a larger sample size to further evaluate its utility as a valid thermometric parameter. This may also include CEM43 and its variants irrespective of the tumor type for a comparative assessment with AUC ≥ 39 °C. Larger sample size is therefore highly desirable to ascertain the most appropriate variable amongst those evaluated in this study. Some of the questions that need to be addressed in future studies are,
Could the treatment times be reduced once the desired AUC ≥ 39 °C is achieved?
Could an unsatisfactory HT session be compensated for by an extra session?
What would be the optimal AUC ≥39 °C for individual and combined HT sessions?
Would the optimal AUC ≥ 39 °C vary for different tumors?
Would the optimal AUC ≥ 39 °C vary for different thermometry techniques – interstitial, intraluminal, surface or noninvasive?
Would the sequencing of HT and RT alter the optimal AUC ≥ 39 °C values? and,
What would be the optimal AUC ≥ 39 °C for HT when combined with CTRT?
These highly relevant issues need to be explored in future studies.
The recent progress in the development of patient-specific hyperthermia treatment planning systems could help optimize tumor heating and provide guidance for HT treatments during their execution [Citation53]. Incorporating AUC ≥ 39 °C could enhance the capabilities of these planning systems and facilitate the appropriate setting of the various HT unit parameters during treatment. With the availability of MRI-based non-invasive thermometry, volumetric temperature displays over the entire tumor volume and critical normal tissues/organs are now feasible [Citation6,Citation52]. These should facilitate quantifications of various AUC levels on a volumetric basis for both tumors and normal tissues. Thus, AUC ≥ 39 °C could assist HT treatment planning and delivery with concurrent online temperature monitoring during the entire HT session and objectively modulate the HT machine power inputs to establish realistic temperatures within the patients’ tolerance. This would allow HT to be performed in a definitive and predictive manner and open up novel opportunities to use HT in combination with RT and/or CT in the clinics.
Conclusions
AUC ≥ 39 °C is a simple, mathematically computable parameter devoid of any assumptions. It could realistically capture the multifactorial effects of HT across the entire temperature range of 39 °C to 45 °C and help to fill the void in thermal dose prescription in clinical HT. It would be a uniform, quality controlled, the easily quantifiable parameter for reporting and comparison of treatment outcomes with HT with RT and/or CT during the clinical practice. The predictive ability of AUC ≥ 39 °C as a thermal dose parameter as evident from this study could be considered as a proof of concept and merits further evaluation in prospective studies in a larger patient cohort.
Acknowledgment
The authors acknowledge Dr. Indranil Pan of Imperial College, London and The Alan Turing Institute, London for his support and guidance for computation of some of the parameters.
Disclosure statement
No potential conflict of interest was reported by the author(s).
Data availability statement
All data generated and analyzed during this study are included in this published article.
References
- Sapareto SA, Dewey WC. Thermal dose determination in cancer therapy. Int J Radiat Oncol Biol Phys. 1984;10(6):787–800.
- Dewey WC. Arrhenius relationships from the molecule and cell to the clinic. Int J Hyperthermia. 2009;25(1):3–20.
- Jones E, Thrall D, Dewhirst MW, et al. Prospective thermal dosimetry: the key to hyperthermia’s future. Int J Hyperthermia. 2006;22(3):247–253.
- Leopold KA, Dewhirst MW, Samulski TV, et al. Cumulative minutes with T90 greater than Tempindex is predictive of response of superficial malignancies to hyperthermia and radiation. Int J Radiat Oncol Biol Phys. 1993;25(5):841–847.
- van Rhoon GC. Is CEM43 still a relevant thermal dose parameter for hyperthermia treatment monitoring? Int J Hyperthermia. 2016;32(1):50–62.
- Schooneveldt G, Bakker A, Balidemaj E, et al. Thermal dosimetry for bladder hyperthermia treatment. An overview. Int J Hyperthermia. 2016;32(4):417–433.
- van der Zee J, Gonzalez Gonzalez D, van Rhoon GC, et al. Comparison of radiotherapy alone with radiotherapy plus hyperthermia in locally advanced pelvic tumours: a prospective, randomised, multicentre trial. Dutch Deep Hyperthermia Group. Lancet. 2000;355(9210):1119–1125.
- Jones EL, Oleson JR, Prosnitz LR, et al. Randomized trial of hyperthermia and radiation for superficial tumors. J Clin Oncol. 2005;23(13):3079–3085.
- Thrall DE, Rosner GL, Azuma C, et al. Using units of CEM 43 degrees C T90, local hyperthermia thermal dose can be delivered as prescribed. Int J Hyperthermia. 2000;16(5):415–428.
- Oleson JR, Samulski TV, Leopold KA, et al. Sensitivity of hyperthermia trial outcomes to temperature and time: implications for thermal goals of treatment. Int J Radiat Oncol Biol Phys. 1993;25(2):289–297.
- Maguire PD, Samulski TV, Prosnitz LR, et al. A phase II trial testing the thermal dose parameter CEM43 degrees T90 as a predictor of response in soft tissue sarcomas treated with pre-operative thermoradiotherapy. Int J Hyperthermia. 2001;17(4):283–290.
- Juang T, Stauffer PR, Craciunescu OA, et al. Thermal dosimetry characteristics of deep regional heating of non-muscle invasive bladder cancer. Int J Hyperthermia. 2014;30(3):176–183.
- de Bruijne M, van der Holt B, van Rhoon GC, et al. Evaluation of CEM43 degrees CT90 thermal dose in superficial hyperthermia: a retrospective analysis. Strahlenther Onkol. 2010;186(8):436–443.
- Franckena M, Fatehi D, de Bruijne M, et al. Hyperthermia dose-effect relationship in 420 patients with cervical cancer treated with combined radiotherapy and hyperthermia. Eur J Cancer. 2009;45(11):1969–1978.
- Kroesen M, Mulder HT, van Holthe JML, et al. Confirmation of thermal dose as a predictor of local control in cervical carcinoma patients treated with state-of-the-art radiation therapy and hyperthermia. Radiother Oncol. 2019;140:150–158.
- Dewhirst MW, Sim DA, Sapareto S, et al. Importance of minimum tumor temperature in determining early and long-term responses of spontaneous canine and feline tumors to heat and radiation. Cancer Res. 1984;44(1):43–50.
- Datta NR, Stutz E, Puric E, et al. A pilot study of radiotherapy and local hyperthermia in elderly patients with muscle-invasive bladder cancers unfit for definitive surgery or chemoradiotherapy. Front Oncol. 2019;9:889.
- Fatehi D, van der Zee J, Notenboom A, et al. Comparison of intratumor and intraluminal temperatures during locoregional deep hyperthermia of pelvic tumors. Strahlenther Onkol. 2007;183(9):479–486.
- Pearce JA. Comparative analysis of mathematical models of cell death and thermal damage processes. Int J Hyperthermia. 2013;29(4):262–280.
- Datta NR, Ordonez SG, Gaipl US, et al. Local hyperthermia combined with radiotherapy and-/or chemotherapy: recent advances and promises for the future. Cancer Treat Rev. 2015;41(9):742–753.
- Overgaard J. The heat is (still) on-the past and future of hyperthermic radiation oncology. Radiother Oncol. 2013;109(2):185–187.
- Frey B, Weiss EM, Rubner Y, et al. Old and new facts about hyperthermia-induced modulations of the immune system. Int J Hyperthermia. 2012;28(6):528–542.
- Datta NR, Kok HP, Crezee H, et al. Integrating loco-regional hyperthermia into the current oncology practice: SWOT and TOWS analyses. Front Oncol. 2020;10:819.
- Dewhirst MW, Vujaskovic Z, Jones E, et al. Re-setting the biologic rationale for thermal therapy. Int J Hyperthermia. 2005;21(8):779–790.
- van der Zee J, Vujaskovic Z, Kondo M, et al. The Kadota Fund International Forum 2004-clinical group consensus. Int J Hyperthermia. 2008;24(2):111–122.
- Piet AH, Hulshof MC, Pieters BR, et al. Clinical results of a concomitant boost radiotherapy technique for muscle-invasive bladder cancer. Strahlenther Onkol. 2008;184(6):313–318.
- Turgeon GA, Souhami L, Cury FL, et al. Hypofractionated intensity modulated radiation therapy in combined modality treatment for bladder preservation in elderly patients with invasive bladder cancer. Int J Radiat Oncol Biol Phys. 2014;88(2):326–331.
- Bruggmoser G, Bauchowitz S, Canters R, et al. Quality assurance for clinical studies in regional deep hyperthermia. Strahlenther Onkol. 2011;187(10):605–610.
- Eisenhauer EA, Therasse P, Bogaerts J, et al. New response evaluation criteria in solid tumours: revised RECIST guideline (version 1.1). Eur J Cancer. 2009;45(2):228–247.
- US Department of Health and Human Services NCI. Common Terminology Criteria for Adverse Events (CTCAE) version 4.0 [Internet]. Bethesda (MD): National Institute of Health; 2010 [cited 2015 November 17]. Available from: http://evs.nci.nih.gov/ftp1/CTCAE/CTCAE_4.03_2010-06-14_QuickReference_5x7.pdf
- Certara. Calculating AUC (linear and log-linear) [Internet]. Princeton (NJ): Certara; 2011. [cited 2020 Sept 3] Available from: https://www.certara.com/knowledge-base/calculating-auc-linear-and-log-linear/
- Datta NR, Rogers S, Klingbiel D, et al. Hyperthermia and radiotherapy with or without chemotherapy in locally advanced cervical cancer: a systematic review with conventional and network meta-analyses. Int J Hyperthermia. 2016;32(7):809–821.
- Datta NR, Rogers S, Ordonez SG, et al. Hyperthermia and radiotherapy in the management of head and neck cancers: a systematic review and meta-analysis. Int J Hyperthermia. 2016;32(1):31–40.
- Datta NR, Stutz E, Gomez S, et al. Efficacy and safety evaluation of the various therapeutic options in locally advanced cervix cancer: a systematic review and network meta-analysis of randomized clinical trials. Int J Radiat Oncol Biol Phys. 2019;103(2):411–437.
- Overgaard J, Gonzalez Gonzalez D, Hulshof MC, et al. Randomised trial of hyperthermia as adjuvant to radiotherapy for recurrent or metastatic malignant melanoma. European Society for Hyperthermic Oncology. Lancet. 1995;345(8949):540–543.
- Datta NR, Puric E, Klingbiel D, et al. Hyperthermia and radiation therapy in locoregional recurrent breast cancers: a systematic review and meta-analysis. Int J Radiat Oncol Biol Phys. 2016;94(5):1073–1087.
- Datta NR, Bodis S. Hyperthermia with photon radiotherapy is thermoradiobiologically analogous to neutrons for tumors without enhanced normal tissue toxicity. Int J Hyperthermia. 2019;36(1):1073–1078.
- Datta NR, Puric E, Schneider R, et al. Could hyperthermia with proton therapy mimic carbon ion therapy? Exploring a thermo-radiobiological rationale. Int J Hyperthermia. 2014;30(7):524–530.
- Tran S, Puric E, Walser M, et al. Early results and volumetric analysis after spot-scanning proton therapy with concomitant hyperthermia in large inoperable sacral chordomas. Br J Radiol. 2019;92:20180883.
- Datta NR, Schneider R, Puric E, et al. Proton irradiation with hyperthermia in unresectable soft tissue sarcoma. Int J Part Ther. 2016;3(2):327–336.
- Issels RD, Lindner LH, Verweij J, et al. Effect of neoadjuvant chemotherapy plus regional hyperthermia on long-term outcomes among patients with localized high-risk soft tissue sarcoma: the EORTC 62961-ESHO 95 randomized clinical trial. JAMA Oncol. 2018;4(4):483–492.
- Repasky EA, Evans SS, Dewhirst MW. Temperature matters! And why it should matter to tumor immunologists. Cancer Immunol Res. 2013;1(4):210–216.
- Toraya-Brown S, Fiering S. Local tumour hyperthermia as immunotherapy for metastatic cancer. Int J Hyperthermia. 2014;30(8):531–539.
- Minnaar CA, Kotzen JA, Ayeni OA, et al. The effect of modulated electro-hyperthermia on local disease control in HIV-positive and -negative cervical cancer women in South Africa: early results from a phase III randomised controlled trial. PLoS One. 2019;14(6):e0217894.
- Datta NR, Grobholz R, Puric E, et al. Enhanced tumour regression in a patient of liposarcoma treated with radiotherapy and hyperthermia: hint for dynamic immunomodulation by hyperthermia. Int J Hyperthermia. 2015;31(5):574–577.
- Dewhirst MW, Viglianti BL, Lora-Michiels M, et al. Basic principles of thermal dosimetry and thermal thresholds for tissue damage from hyperthermia. Int J Hyperthermia. 2003;19(3):267–294.
- Thrall DE, LaRue SM, Yu D, et al. Thermal dose is related to duration of local control in canine sarcomas treated with thermoradiotherapy. Clin Cancer Res. 2005;11(14):5206–5214.
- Stahl R, Wang T, Lindner LH, et al. Comparison of radiological and pathohistological response to neoadjuvant chemotherapy combined with regional hyperthermia (RHT) and study of response dependence on the applied thermal parameters in patients with soft tissue sarcomas (STS). Int J Hyperthermia. 2009;25(4):289–298.
- Inman BA, Stauffer PR, Craciunescu OA, et al. A pilot clinical trial of intravesical mitomycin-C and external deep pelvic hyperthermia for non-muscle-invasive bladder cancer. Int J Hyperthermia. 2014;30(3):171–175.
- Sherar M, Liu FF, Pintilie M, et al. Relationship between thermal dose and outcome in thermoradiotherapy treatments for superficial recurrences of breast cancer: data from a phase III trial. Int J Radiat Oncol Biol Phys. 1997;39(2):371–380.
- Trefná HD, Crezee H, Schmidt M, et al. Quality assurance guidelines for superficial hyperthermia clinical trials: I. Clinical requirements. Int J Hyperthermia. 2017;33(4):471–482.
- Curto S, Aklan B, Mulder T, et al. Quantitative, multi-institutional evaluation of MR thermometry accuracy for deep-pelvic MR-hyperthermia systems operating in multi-vendor MR-systems using a new anthropomorphic phantom. Cancers (Basel. 2019;11(11):1709.
- Gavazzi S, van Lier A, Zachiu C, et al. Advanced patient-specific hyperthermia treatment planning. Int J Hyperthermia. 2020;37(1):992–1007.