Abstract
Purpose
To evaluate the effect of Hashimoto's thyroiditis (HT) on the extent of ablation zone in ultrasound (US)-guided radiofrequency ablation (RFA) for early stages of papillary thyroid microcarcinoma (PTMC).
Method
We selected 772 patients with 797 PTMCs who underwent with RFA from August 2017 to August 2020. They were subdivided into two groups as follows: (i) 216 patients (224 PTMCs) with HT in the ‘HT + PTMC’ group and (ii) 556 patients (573 PTMCs) with healthy thyroid in the ‘PTMC’ group. We assessed the extent (maximum diameter and volume) of the ablation zone by contrast-enhanced ultrasound (CEUS) immediately, one day, and 1 week following RFA.
Results
The ablation zone of the ‘HT + PTMC’ group was smaller than that of the ‘PTMC’ group at 1 week of RFA (maximum diameter: 14.6 ± 3.1 mm vs. 15.2 ± 3.2 mm and volume: 0.932 ± 0.498 mL vs. 1.028 ± 0.540 mL, respectively, p < .05). However, there were no differences before, immediately, and one day post-RFA (p > .05). Life-threatening complications did not develop in any of the patients.
Conclusion
RFA-treated PTMCs were smaller in size in patients with HT than in those with a healthy thyroid at 1 week of RFA. However, the exact mechanism underlying this phenomenon and its clinical significance warrant further investigation.
Introduction
The widespread use of high-frequency ultrasound (US) has rapidly increased the incidence of thyroid cancer (TC). In the United States, the incidence increased by 211% between 1975 and 2013 [Citation1], whereas it revealed a trend of rapid growth during 2005–2015 in China [Citation2]. The primary reason for this phenomenon is the increasing incidence of papillary thyroid microcarcinoma (PTMC) [Citation3]. Papillary thyroid carcinoma (PTC) is the most common and inert histological subtype of TC [Citation4]. PTMC is defined as PTC with a maximum diameter ≤10 mm [Citation5]. Thyroidectomy is the standard treatment for PTMC; however, active surveillance (AS) may be used for low-risk PTMC [Citation6,Citation7]. Moreover, the 2015 American Thyroid Association (ATA) recommended AS for low-risk PTMC [Citation8]. AS prevents the risks of surgery in some patients; however, anxiety over possible metastasis by clinicians and patients hinders its implementation [Citation9]. The aforementioned strategies also cause a dilemma for clinicians and patients during treatment selection. However, US-guided thermal ablation (TA), including radiofrequency ablation (RFA), laser ablation (LA), and microwave ablation (MWA), has demonstrated satisfactory efficacy and safety in PTMC treatment [Citation10].
Hashimoto's thyroiditis (HT) is closely related to PTC [Citation11,Citation12], and approximately 1/3 cases of PTC are concurrent with HT [Citation13,Citation14]. HT is known as chronic autoimmune thyroiditis and chronic lymphocytic thyroiditis (CLT), caused by the destruction of thyroid cells by antibodies or immune cell-mediated cytotoxicity. This results in morphological and microscopic changes in the thyroid gland, including pathophysiologic changes, such as enlarged thyroid morphology, inflammatory cell infiltration, fibrous tissue hyperplasia, calcification, and vascular hyperplasia [Citation15].
Researchers have extensively investigated the use of RFA in solid tumors of the liver [Citation16]. Low thermal conductivity of the background tissue is conducive to achieving a higher temperature within a defined ablation target [Citation17,Citation18], termed ‘the oven effect.’ For example, low thermal conductivity of cirrhosis would benefit the efficacy of RFA for liver tumors [Citation19]. Similarly, the efficiency of RFA may increase in patients with extrarenal renal cell carcinoma surrounding the perirenal fat and bone tumor around the cortical bone [Citation20]. There are reports on differences in the ablation size of RFA between liver cirrhosis and normal liver background [Citation21]. Considering the differences between HT and normal thyroid parenchyma, we assume that variations in the range of ablation zone of RFA for PTMC exist between patients with HT and those with a healthy thyroid.
We aimed to evaluate the effect of HT on the extent of the ablation zone in early stage in US-guided RFA for PTMC.
Materials and methods
Patients
This prospective study was approved by medical ethics committee of Ruijin Hospital, Shanghai Jiaotong University School of Medicine, Shanghai, China (protocol number: KY2017-125). All patients provided written informed consent for the TA of PTMC before RFA. The inclusion criteria were as follows: (1) nodules confirmed as PTC on cytology by US-guided fine-needle aspiration; (2) the maximum diameter of the nodule ≤1 cm; (3) less than two PTC tumors; (4) no capsular infiltration and extrathyroidal invasion; (5) no sign of lymph node metastasis on clinical examination; and (6) inability or the refusal of surgery. The exclusion criteria were as follows: (1) severe coagulation disorders; (2) respiratory failure, myocardial infarction, or other serious systemic diseases; (3) impaired neck extension; (4) contralateral vocal cord paralysis; (5) the fusion of two ablation regions following ablation; and (6) re-ablation owing to suspicious residuals following the initial ablation.
depicts the selection procedure for this study. A total of 788 patients with PTMC were treated with US-guided RFA from August 2017 to August 2020. Of the 16 patients excluded from the study, six were lost to follow-up (did not participate in the follow-up within 1 week after RFA), three had two lesions and the fusion of the two ablation foci occurred after RFA, and seven were re-ablated because of suspicious residuals following the first ablation. Eventually, we analyzed 772 patients with 797 PTMCs treated with RFA. There were 621 women and 151 men, with an average age of 40.8 ± 11.0 years (range 19–74 years). Patients were divided into two groups as follows: (i) 216 patients (224 PTMCs) with HT in the ‘HT + PTMC’ group and (ii) 556 patients (573 PTMCs) with healthy thyroid in the ‘PTMC’ group.
HT was diagnosed by combining high-frequency US with serological examinations. High-frequency US of the thyroid revealed diffuse hypoechoic thyroid parenchyma. Serum examination displayed positive thyroid peroxidase antibody (TPOAb) or thyroglobulin antibody (TgAb) [Citation15].
Pre-ablation assessment
All patients completed preoperative examinations, including thyroid US, laboratory examinations, electrocardiography (ECG), and laryngoscopy. All US scans were obtained with commercial US scanners (Mindray Resona 7 and 8, Shenzhen, China) equipped with 9–14 MHz linear array transducer probes. During US examinations, two radiologists (J.Q.Z. and Y.J.D.) with >15 years of experience in thyroid US carefully evaluated the position, size, and volume of the nodules.
Laboratory examinations consisted of serum thyroid stimulating hormone, triiodothyronine (T3), thyroxine (T4), free T3, free T4, TPOAb (negative <5.61 IU/mL), TgAb (negative <4.11 IU/mL), and blood coagulation test platelet count (including prothrombin time and activated partial thromboplastin time).
RF ablation procedures
One operator (J.Q.Z.) performed all RFA procedures in the outpatient department. The patients were placed in a supine position with their neck extended. A multi-parameter device was connected to continuously monitor their ECG, noninvasive blood pressure, oxygen saturation, and pulse. An intravenous catheter was inserted into the superficial vein of the upper limb. We used a radiofrequency generator (VIVA RF generator, STARmed, Goyang, South Korea, and VRS01; RF Medical, Seoul, South Korea) and an 18-gauge thyroid-dedicated internally cooled electrode with a 10-mm exposure tip (Star RF Electrode_Fixed, STARmed; VIVA RF System, Goyang, South Korea).
Following local anesthesia with 2% lidocaine, 0.9% sodium chloride solution was injected around the thyroid gland to preserve important structures surrounding the ablation, such as the trachea, esophagus, recurrent laryngeal nerves, and carotid artery. Under real-time US guidance, an 18-gauge ablation needle was inserted into the target tumor and the ablation power was set to 65 W according to the literature [Citation22].
In this study, single- or multi-point fixed ablation technology was used. Multi-point ablation was sometimes used in order to completely cover the tumor in three-dimensional space with the hyperechoic zone produced by ablation. The ablation process can be stopped only after the lesion was completely covered with a hyperechoic zone caused by vaporization in three-dimensional space on gray-scale US [Citation23]. Simultaneously, the needle track was ablated to avoid the metastasis of tumor cells along the needle channel. The ablation energy of each nodule was subsequently recorded.
Conventional ultrasound and contrast-enhanced ultrasound (CEUS)
We used B-mode US, Color Doppler US, and CEUS to evaluate the ablation zone immediately, one day, and 1 week following ablation. B-mode US and Color Doppler US principally focused on morphological changes and complications, such as hematoma, in the ablation area. We used CEUS to assess the extent of the ablation area. All CEUS were performed using sulfur hexafluoride-filled microbubbles (SonoVue, Bracco, Milan, Italy). All dynamic and static US images were digitally stored on the hard disk of the US scanner and uploaded to the picture archiving and communication systems for subsequent analysis.
All nodules were measured with three orthogonal diameters (length, width, and thickness) in CEUS graphs. Their volumes were evaluated using the following formula: V=πABC/6 (where V is the volume, A is the length, and B and C are the remaining two vertical diameters). The post-ablation follow-up was performed by our team, each of whom had undergone standardized training to minimize inter-operator variation.
Statistical analysis
We used SPSS version 22.0 for Windows (SPSS Inc., Chicago, IL) to analyze the results. All data are expressed as the mean ± standard deviation. We analyzed categorical variables, such as sex and continuous variables, such as the age, ablation energy, maximum diameter, and volume, using the chi-square test and two independent samples nonparametric tests (Mann–Whitney’s U test), respectively. Differences were considered statistically significant at p values <.05.
Results
Patients and ablation data
summarizes the demographic and ablation data of the selected patients and their lesions. There were significant differences in sex and age (p < .05) between the ‘PTMC’ group and ‘HT + PTMC’ group. Patients in the ‘HT + PTMC’ group were younger than those in the ‘PTMC’ group (38.8 ± 10.5 years vs. 41.5 ± 11.1 years, p= .001). The proportion of women was higher in the ‘HT + PTMC’ group than that in the ‘PTMC’ group (91.2%, 197/216 > 76.26%, 424/556, p= .000). The ablation energies and ablation time of the ‘PTMC’ and ‘HT + PTMC’ groups were 1.880 ± 1.485 kJ and 1.966 ± 1.792 kJ and 61.1 ± 42.7 s and 66.2 ± 55.5 s, respectively (both p > .05). The initial maximum tumor diameters and tumor volumes in the ‘PTMC’ and ‘HT + PTMC’ groups were 5.5 ± 1.8 mm and 5.6 ± 1.8 mm and 0.078 ± 0.079 mL and 0.078 ± 0.073 mL, respectively, without significant differences (p > .05).
Table 1. The demographic and ablation data of the selected patients and lesions.
Maximum diameter and lesion volume
In all cases, the tumor nodules were completely covered by the ablation areas. The maximum diameters and volumes of the ablation zone 1 week following RFA were significantly larger than those before ablation. The maximum diameters and volumes of the ablation zone reached the peak one day post-RFA (–). There were no significant differences between the ‘PTMC’ and ‘HT + PTMC’ groups in the maximum diameter immediately and one day post-RFA (14.0 ± 2.6 vs. 14.0 ± 2.5 mm, 16.1 ± 3.1 vs. 15.8 ± 3.2 mm, respectively, p > .05 for both). However, the maximum diameter 1 week post-RFA in the ‘HT + PTMC’ group was significantly smaller than that in the ‘PTMC’ group (14.6 ± 3.1 vs. 15.2 ± 3.2 mm, respectively, p= .005) (, ).
Figure 2. Changes in maximum diameters in PTMC cases with/without HT. Error bar: 95% confidence interval; PTMC: papillary thyroid microcarcinoma; HT: Hashimoto's thyroiditis.
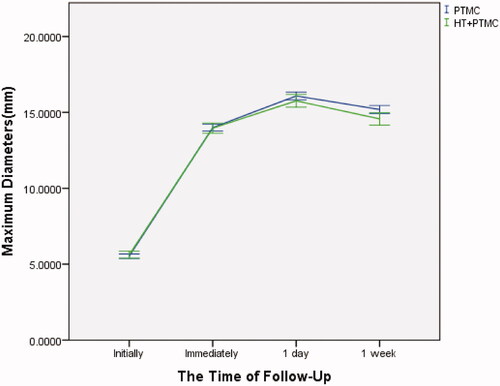
Table 2. The maximum diameters of the lesion before and after RFA (mm).
Similarly, there were no significant differences in the ablation volume between the ‘PTMC’ and ‘HT + PTMC’ groups immediately and one day post-RFA (0.838 ± 0.426 vs. 0.841 ± 0.434 mL, 1.259 ± 0.624 vs. 1.214 ± 0.629 mL, respectively, p > .05 for both). However, the volume at 1 week post-RFA in the ‘HT + PTMC’ group was significantly smaller than that in the ‘PTMC’ group (1.028 ± 0.540 vs. 0.932 ± 0.498 mL, respectively, p= .007) (, ).
Figure 3. Changes in volumes in PTMC cases with/without HT. Error bar: 95% confidence interval; PTMC: papillary thyroid microcarcinoma; HT: Hashimoto's thyroiditis.
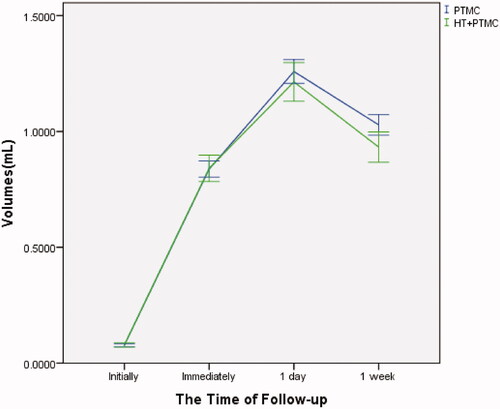
Table 3. The volumes of the lesion before and after RFA (mL).
Complications
Life-threatening complications did not occur in any patient. Seventeen patients experienced hoarseness following RFA. Of these patients, 16 recovered after 1 week to 3 months without any special treatment, whereas one did not recover after 6 months. Laryngoscopy examinations revealed fixation of one vocal cord. In addition, two patients had postoperative hematomas, fixed hemostasis was used, and absorption was recovered naturally. Rashes and pain occurred in one patient each. There was no evidence for the recurrence of ablated areas within 1 week of the follow-up.
Figure 4. Representative case of RFA-treated PTMC with HT within 1 week in a 29-year-old woman. High-frequency US of the thyroid revealed diffuse hypoechoic thyroid parenchyma and a nodule in the middle of the right thyroid. The nodule was confirmed as PTC on cytology by US-guided fine-needle aspiration. (A) The sonograms of the nodule before RFA, including transverse section and longitudinal section (size: 5.0 × 9.5 × 6.7 mm, the volume about 0.17 mL); (B) the sonograms immediately after RFA (including the gray-scale US and CEUS of transverse and longitudinal sections), showed that no contrast was filling in the ablation area (size: 10.1 × 15.3 × 11.8 mm, the volume about 0.69 mL); (C) the sonograms at one day of RFA (including the gray-scale US and CEUS of transverse and longitudinal sections), showed that there was no contrast filling in the ablation area (size: 13.0 × 16.0 × 14.1 mm, the volume about 1.54 mL); (D) the sonograms at 1 week of RFA (including the gray-scale US and CEUS of transverse and longitudinal sections), showed that there was no contrast filling in the ablation area (size: 10.9 × 14.5 × 13.4 mm, the volume about 1.11 mL).
Discussion
TA uses extremely high or low temperatures to cause protein denaturation, irreversible cell damage, and coagulative necrosis of the tumor cells [Citation20,Citation24]. TA has become a promising treatment for several types of tumors [Citation25], such as liver [Citation26], kidney [Citation27,Citation28], bone [Citation29], and lung tumors [Citation30]. RFA, MWA, and LA are the most common techniques used in clinical practice.
Rossi et al. and McGahan et al. [Citation31,Citation32] first reported on a study on RFA for liver tumors in animal models in 1990. In 1994, Seki et al. [Citation33] reported on a preliminary study on US-guided percutaneous microwaves for human liver cancer (≤2 cm maximum diameter). In 1985, Ohyama et al. [Citation34] developed the contact Nd–YAG laser system with ceramic rods and applied it to head and neck tumors. In 1995, Anzai et al. [Citation35] published the first report on RFA in humans for the treatment of cerebral tumors. Pacella et al. [Citation36] first reported on the use of percutaneous LA for human untreatable recurrent TC in 2000, whereas Dupuy et al. [Citation37] reported on the use of RFA for locally recurrent lesions of well-differentiated TC in 2001. In 2006, Kim et al. [Citation38] used RFA to treat 35 cold benign thyroid nodules, which relieved mass-related symptoms without damaging thyroid function and severe complications.
RFA is a safe, cost-effective, and minimally invasive procedure for treating benign and/or malignant thyroid nodules [Citation39]. TA significantly reduces the volume of benign thyroid tumor or eliminates it by avoiding thyroidectomy and hypothyroidism [Citation40–42]. Numerous studies on TC treatment have verified the safety and feasibility of US-guided TA for low-risk PTMC [Citation43–46]. In a recent multicenter study, Cao et al. [Citation47] reported on the efficacy and safety of TA in patients with isolated T1bN0M0 PTC. Moreover, a recent meta-analysis reported that TA for PTC was as effective, secure, and economical as conventional surgery [Citation48].
CEUS is considered a valuable tool for assessing ablation zones. It facilitates identifying tumor sites that are not adequately covered by ablation. Therefore, early ablation can be supplemented in time to reduce the risk of recurrence [Citation49–51]. CEUS is useful to assess the efficacy of ablation by displaying the parenchyma microvasculature and assessing angiogenesis and tissue perfusion using contrast agents [Citation52]. Therefore, we used CEUS to accurately measure the extent of ablation lesions.
PTC is associated with HT [Citation11,Citation12], which is the most common inflammatory thyroid disease. It is principally characterized by lymphocyte infiltration, fibrosis, and atrophy of the thyroid parenchyma [Citation15]. The blood supply characteristics and histology of the thyroid parenchyma in HT differ from those of the normal thyroid, which may affect the efficacy of RFA for PTC. However, few studies have been conducted to understand this further. Zhang et al. [Citation23] compared the effects of RFA for PTMC with/without HT using 60 cases of PTMC, and concluded that the recovery of patients with PTMC in combination with HT following ablation was similar to those with PTMC alone. However, their sample size was considerably small (n = 60) and data were compared during 1–18 months follow-up. Moreover, there is limited research on differences in the early stage. However, we used a higher sample size to study the effects of HT on the extent of RFA of PTMC in the early stage (within 1 week).
Previous studies have found that the ablation zone could be differentiated macro- and micro-morphologically into central zone with coagulation necrosis and peripheral zone with dilated sinusoid and congestion of erythrocytes, perhaps with inflammation or edema, adjacent to the unaffected tissue [Citation53,Citation54]. Low thermal conductivity of the thyroid fibrous tissue in HT may exert an effect similar to ‘the oven effect,’ [Citation16] which accumulated heat in the lesions, thus limiting the degree of inflammation or edema in the peripheral zone. Moreover, the increase of thyroid blood supply in HT may accelerate the absorption of inflammation and edema, as well as the regression of dilated sinusoid and congestion of erythrocytes in peripheral zone. This may explain that the maximum diameters and volumes of the ablation zone in the ‘HT + PTMC’ group were smaller than those in the ‘PTMC’ group at 1 week post-RFA, while there were no differences immediately, and one day following the RFA.
The present study had some limitations. First, this was a single-center, prospective study. Second, the maximum diameter and volume of the ablation zone were smaller in the ‘HT + PTMC’ group than that in the ‘PTMC’ group; however, our follow-up time was insufficient to determine if the smaller ablation zone increased the recurrence rate of HT + PTMC. Third, we did not collect information on the volume of the treatment lobe, which may be affected by diffuse diseases, such as HT. Patients with HT often have smaller thyroid glands; therefore, nodules near the thyroid capsule are more likely to be present. In these nodules, the ablation zone may be smaller for purely geometric reasons. Our results should be confirmed by more multicenter and prospective studies with larger sample size. In addition, a relatively high ablation power, i.e., 65 W, was used in this study. Considering that different ablation powers may lead to differences in the degree of carbonation, which can lead to differences in ablation volume, future studies on the effectiveness of high-power ablation and low-power ablation in the treatment of PTMC can be conducted.
Conclusion
RFA-treated PTMCs were smaller in size in patients with HT than in those with a healthy thyroid at 1 week of RFA. Therefore, HT exerts an influence on the ablation extent of PTMCs treated with RFA. However, the exact mechanism underlying this phenomenon and its clinical significance warrant further investigation.
Acknowledgements
We thank the site investigators, study coordinators, and patients who participated in the trial.
Disclosure statement
The authors report there are no competing interests to declare.
References
- Lim H, Devesa SS, Sosa JA, et al. Trends in thyroid cancer incidence and mortality in the United States, 1974–2013. JAMA. 2017;317(13):1338–1348.
- Wang J, Yu F, Shang Y, et al. Thyroid cancer: incidence and mortality trends in China, 2005–2015. Endocrine. 2020;68(1):163–173.
- Grodski S, Brown T, Sidhu S, et al. Increasing incidence of thyroid cancer is due to increased pathologic detection. Surgery. 2008;144(6):1038–1043; discussion 43.
- Cabanillas ME, McFadden DG, Durante C. Thyroid cancer. Lancet. 2016;388(10061):2783–2795.
- Hedinger C, Williams ED, Sobin LH. The WHO Histological Classification of Thyroid Tumors: a commentary on the second edition. Cancer. 1989;63(5):908–911.
- Ito Y, Miyauchi A, Oda H. Low-risk papillary microcarcinoma of the thyroid: a review of active surveillance trials. Eur J Surg Oncol. 2018;44(3):307–315.
- Leboulleux S, Tuttle RM, Pacini F, et al. Papillary thyroid microcarcinoma: time to shift from surgery to active surveillance? Lancet Diabetes Endocrinol. 2016;4(11):933–942.
- Luster M, Aktolun C, Amendoeira I, et al. European perspective on 2015 American Thyroid Association Management Guidelines for Adult Patients with Thyroid Nodules and Differentiated Thyroid Cancer: proceedings of an interactive international symposium. Thyroid. 2019;29(1):7–26.
- Haser GC, Tuttle RM, Su HK, et al. Active surveillance for papillary thyroid microcarcinoma: new challenges and opportunities for the health care system. Endocr Pract. 2016;22(5):602–611.
- Tong M, Li S, Li Y, et al. Efficacy and safety of radiofrequency, microwave and laser ablation for treating papillary thyroid microcarcinoma: a systematic review and meta-analysis. Int J Hyperthermia. 2019;36(1):1278–1286.
- Singh B, Shaha AR, Trivedi H, et al. Coexistent Hashimoto's thyroiditis with papillary thyroid carcinoma: impact on presentation, management, and outcome. Surgery. 1999;126(6):1070–1076; discussion 6–7.
- Vita R, Ieni A, Tuccari G, et al. The increasing prevalence of chronic lymphocytic thyroiditis in papillary microcarcinoma. Rev Endocr Metab Disord. 2018;19(4):301–309.
- Ieni A, Vita R, Magliolo E, et al. One-third of an archivial series of papillary thyroid cancer (years 2007–2015) has coexistent chronic lymphocytic thyroiditis, which is associated with a more favorable tumor-node-metastasis staging. Front Endocrinol. 2017;8(337):337.
- Resende de Paiva C, Gronhoj C, Feldt-Rasmussen U, et al. Association between Hashimoto's thyroiditis and thyroid cancer in 64,628 patients. Front Oncol. 2017;7:53.
- Caturegli P, De Remigis A, Rose NR. Hashimoto thyroiditis: clinical and diagnostic criteria. Autoimmun Rev. 2014;13(4–5):391–397.
- Ni Y, Mulier S, Miao Y, et al. A review of the general aspects of radiofrequency ablation. Abdom Imaging. 2005;30(4):381–400.
- Liu Z, Ahmed M, Weinstein Y, et al. Characterization of the RF ablation-induced 'oven effect': the importance of background tissue thermal conductivity on tissue heating. Int J Hyperthermia. 2006;22(4):327–342.
- Ahmed M, Liu Z, Humphries S, et al. Computer modeling of the combined effects of perfusion, electrical conductivity, and thermal conductivity on tissue heating patterns in radiofrequency tumor ablation. Int J Hyperthermia. 2008;24(7):577–588.
- Goldberg SN, Gazelle GS, Mueller PR. Thermal ablation therapy for focal malignancy: a unified approach to underlying principles, techniques, and diagnostic imaging guidance. AJR Am J Roentgenol. 2000;174(2):323–331.
- Ahmed M, Brace CL, Lee FT Jr., et al. Principles of and advances in percutaneous ablation. Radiology. 2011;258(2):351–369.
- Wang H, Lee JC, Cao K, et al. What is the difference in ablation zone of multi-bipolar radiofrequency ablation between liver cirrhosis and normal liver background? A prospective clinical study. Int J Hyperthermia. 2020;37(1):1248–1259.
- Aysan E, Idiz UO, Akbulut H, et al. Single-session radiofrequency ablation on benign thyroid nodules: a prospective single center study: radiofrequency ablation on thyroid. Langenbecks Arch Surg. 2016;401(3):357–363.
- Zhang Y, Zhang MB, Luo YK, et al. Effect of chronic lymphocytic thyroiditis on the efficacy and safety of ultrasound-guided radiofrequency ablation for papillary thyroid microcarcinoma. Cancer Med. 2019;8(12):5450–5458.
- Nikfarjam M, Muralidharan V, Christophi C. Mechanisms of focal heat destruction of liver tumors. J Surg Res. 2005;127(2):208–223.
- Gillams A. Tumour ablation: current role in the liver, kidney, lung and bone. Cancer Imaging. 2008;8(Spec. No. A):S1–S5.
- Izzo F, Granata V, Grassi R, et al. Radiofrequency ablation and microwave ablation in liver tumors: an update. Oncologist. 2019;24(10):e990–e1005.
- Mahnken AH, Gunther RW, Tacke J. Radiofrequency ablation of renal tumors. Eur Radiol. 2004;14(8):1449–1455.
- Joniau S, Tsivian M, Gontero P. Radiofrequency ablation for the treatment of small renal masses: safety and oncologic efficacy. Minerva Urol Nefrol. 2011;63(3):227–236.
- Cantwell CP, Obyrne J, Eustace S. Current trends in treatment of osteoid osteoma with an emphasis on radiofrequency ablation. Eur Radiol. 2004;14(4):607–617.
- Li G, Xue M, Chen W, et al. Efficacy and safety of radiofrequency ablation for lung cancers: a systematic review and meta-analysis. Eur J Radiol. 2018;100:92–98.
- Rossi S, Fornari F, Pathies C, et al. Thermal lesions induced by 480 kHz localized current field in guinea pig and pig liver. Tumori. 1990;76(1):54–57.
- McGahan JP, Browning PD, Brock JM, et al. Hepatic ablation using radiofrequency electrocautery. Invest Radiol. 1990;25(3):267–270.
- Seki T, Wakabayashi M, Nakagawa T, et al. Ultrasonically guided percutaneous microwave coagulation therapy for small hepatocellular carcinoma. Cancer. 1994;74(3):817–825.
- Ohyama M, Katsuda K, Nobori T, et al. Treatment of head and neck tumors by contact Nd–YAG laser surgery. Auris Nasus Larynx. 1985;12(Suppl. 2):S138–S142.
- Anzai Y, Lufkin R, DeSalles A, et al. Preliminary experience with MR-guided thermal ablation of brain tumors. AJNR Am J Neuroradiol. 1995;16(1):39–48; discussion 9–52.
- Pacella CM, Bizzarri G, Guglielmi R, et al. Thyroid tissue: US-guided percutaneous interstitial laser ablation—a feasibility study. Radiology. 2000;217(3):673–677.
- Dupuy DE, Monchik JM, Decrea C, et al. Radiofrequency ablation of regional recurrence from well-differentiated thyroid malignancy. Surgery. 2001;130(6):971–977.
- Kim YS, Rhim H, Tae K, et al. Radiofrequency ablation of benign cold thyroid nodules: initial clinical experience. Thyroid. 2006;16(4):361–367.
- Kim JH, Baek JH, Lim HK, et al. 2017 thyroid radiofrequency ablation guideline: Korean Society of Thyroid Radiology. Korean J Radiol. 2018;19(4):632–655.
- Rangel L, Volpi LM, Stabenow E, et al. Radiofrequency for benign and malign thyroid lesions. World J Otorhinolaryngol Head Neck Surg. 2020;6(3):188–193.
- Cesareo R, Palermo A, Pasqualini V, et al. Radiofrequency ablation for the management of thyroid nodules: a critical appraisal of the literature. Clin Endocrinol. 2017;87(6):639–648.
- Radzina M, Cantisani V, Rauda M, et al. Update on the role of ultrasound guided radiofrequency ablation for thyroid nodule treatment. Int J Surg. 2017;41(Suppl. 1):S82–S93.
- Yan L, Lan Y, Xiao J, et al. Long-term outcomes of radiofrequency ablation for unifocal low-risk papillary thyroid microcarcinoma: a large cohort study of 414 patients. Eur Radiol. 2021;31(2):685–694.
- Chen J, Cao J, Qiu F, et al. The efficacy and the safety of ultrasound-guided ablation therapy for treating papillary thyroid microcarcinoma. J Cancer. 2019;10(21):5272–5282.
- Zhang M, Luo Y, Zhang Y, et al. Efficacy and safety of ultrasound-guided radiofrequency ablation for treating low-risk papillary thyroid microcarcinoma: a prospective study. Thyroid. 2016;26(11):1581–1587.
- Wang L, Xu D, Yang Y, et al. Safety and efficacy of ultrasound-guided percutaneous thermal ablation in treating low-risk papillary thyroid microcarcinoma: a pilot and feasibility study. J Cancer Res Ther. 2019;15(7):1522–1529.
- Cao XJ, Liu J, Zhu YL, et al. Efficacy and safety of thermal ablation for solitary T1bN0M0 papillary thyroid carcinoma: a multicenter study. J Clin Endocrinol Metab. 2020;106(2):e573–e581.
- Shen K, Xue S, Xie Y, et al. Comparison of thermal ablation and routine surgery for the treatment of papillary thyroid microcarcinoma: a systematic review and meta-analysis. Int J Hyperthermia. 2020;37(1):913–924.
- Rennert J, Wiesinger I, Beyer LP, et al. Color coded perfusion analysis and microcirculation imaging with contrast enhanced ultrasound (CEUS) for post-interventional success control following thermal ablative techniques of primary and secondary liver malignancies. Clin Hemorheol Microcirc. 2019;73(1):73–83.
- Zhang L, Zhou W, Zhan W, et al. Percutaneous laser ablation of unifocal papillary thyroid microcarcinoma: utility of conventional ultrasound and contrast-enhanced ultrasound in assessing local therapeutic response. World J Surg. 2018;42(8):2476–2484.
- da Silva NPB, Beyer LP, Hottenrott MC, et al. Efficiency of contrast enhanced ultrasound for immediate assessment of ablation status after intraoperative radiofrequency ablation of hepatic malignancies. Clin Hemorheol Microcirc. 2017;66(4):357–368.
- Yan L, Luo Y, Xiao J, et al. Non-enhanced ultrasound is not a satisfactory modality for measuring necrotic ablated volume after radiofrequency ablation of benign thyroid nodules: a comparison with contrast-enhanced ultrasound. Eur Radiol. 2021;31(5):3226–3236.
- Song KD, Lee MW, Rhim H, et al. Chronological changes of radiofrequency ablation zone in rabbit liver: an in vivo correlation between gross pathology and histopathology. Br J Radiol. 2017;90(1071):20160361.
- Gemeinhardt O, Poch FG, Hiebl B, et al. Comparison of bipolar radiofrequency ablation zones in an in vivo porcine model: correlation of histology and gross pathological findings. Clin Hemorheol Microcirc. 2017;64(3):491–499.