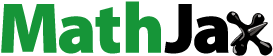
Abstract
Purpose
In nonmuscle invasive bladder cancer (NMIBC) patients who fail standard intravesical treatment and are unfit or unwilling to undergo a radical cystectomy, radiofrequency (RF)-induced hyperthermia combined with intravesical chemotherapy (RF-CHT) has shown promising results. We studied whether higher thermal dose improves clinical NMIBC outcome.
Methods and materials
The cohort comprised 108 patients who started with RF-CHT between November 2013 and December 2019. Patients received intravesical mitomycin-C or epirubicin. Bladder hyperthermia was accomplished with an intravesical 915 MHz RF device guided by intravesical thermometry. We assessed the association between thermal dose parameters (including median temperature and Cumulative Equivalent Minutes of T50 at 43 °C [CEM43T50]) and complete response (CR) at six months for patients with (concomitant) carcinoma in situ (CIS), and recurrence-free survival (RFS) for patients with papillary disease.
Results
Median temperature and CEM43T50 per treatment were 40.9 (IQR 40.8–41.1) °C and 3.1 (IQR 0.9–2.4) minutes, respectively. Analyses showed no association between any thermal dose parameter and CR or RFS (p > 0.05). Less bladder spasms during treatment sessions was associated with increased median temperature and CEM43T50 (adjusted OR 0.01 and 0.34, both p < 0.001).
Conclusions
No significant association between thermal dose and NMIBC outcome was found. Possibly thermal dose effect in patients of the current cohort exceeds a certain threshold value. On the other hand, occurrence of bladder spasms had a thermal dose limiting effect. We advise to treat patients with temperatures >40.5 °C for at least 45 min while respecting individual tolerability, including occurrence of bladder spasms.
Introduction
Bladder cancer carries a significant societal burden, ranking fifth among the most frequently diagnosed cancers and first among costliest cancers per capita in Europe and Northern America [Citation1,Citation2]. About 75% of bladder cancer patients present with nonmuscle invasive bladder cancer (NMIBC), including carcinoma in situ (CIS), papillary tumors (Ta), and tumors that invade the lamina propria (T1) [Citation3]. The recommended treatment for NMIBC is a transurethral resection of the bladder tumor (TURBT) with or without adjuvant intravesical instillations with Bacillus Calmette-Guérin (BCG) or with chemotherapeutic agents including mitomycin-C (MMC) or epirubicin, depending on the risk group.
The main issue with intermediate and high-risk NMIBC management is the high recurrence rate, and when intravesical treatment fails, patients may not be candidates for more aggressive treatment. In five years, high-risk patients have a probability of recurrence and progression to muscle invasive disease (MIBC) up to 52% and 20%, respectively, despite intravesical therapy [Citation4]. Moreover, a worldwide scarcity of BCG which has evolved over the last decade, and a significant part of patients who do receive BCG discontinue treatment due to side effects [Citation5]. Urology guidelines recommend a radical cystectomy with urinary diversion for NMIBC patients in whom standard intravesical treatment fails [Citation3]. However, this complex operation is associated with perioperative complication and mortality rates of up to 60% and 10%, respectively [Citation6–8]. Because bladder cancer patients on average have an advanced age and multiple comorbidities, patients are frequently medically unfit or unwilling to undergo this major surgery. Consequentially, radical cystectomy is left markedly underutilized [Citation9]. For these reasons, development of effective alternative noninvasive treatments for NMIBC is imperative.
Mild hyperthermia (40–43 °C) is a device-assisted approach designed to potentiate the effect of chemotherapy for various types of cancer, including NMIBC. Randomized controlled trials have demonstrated that the addition of hyperthermia to standard chemotherapy (thermochemotherapy) causes significantly better outcome for soft tissue sarcoma, ovarian cancer with peritoneal involvement and NMIBC [Citation10–12]. Mechanisms of action of thermochemotherapy include enhanced cytotoxic effect of chemotherapeutic agents [Citation13], increased drug delivery to tumor tissue by increased perfusion and drug extravasation [Citation14,Citation15], and induction of an immune response [Citation10,Citation16]. As NMIBC treatment, bladder hyperthermia is combined with intravesical MMC or epirubicin instillation [Citation17]. Most trials reporting on bladder hyperthermia use microwave induced heating with an intravesical radiofrequency (RF)-emitting antenna in combination with intravesical chemotherapy (RF-CHT) [Citation12,Citation18–21]. The results of a randomized controlled trial (RCT) of Colombo et al. in high-risk NMIBC patients showed that adding hyperthermia to intravesical MMC increases two-year recurrence-free survival (RFS) from 17% to 57% [Citation19]. Despite the clear benefit from RF-CHT, a recently published study with long-term follow-up of high-risk NMIBC patients (the majority of whom were unresponsive to BCG) reported six-month complete response (CR) for CIS patients of only 56.0% and five-year RFS for patients with papillary disease of only 37.2% [Citation22]. These numbers indicate that there is room for improvement of the RF-CHT strategy.
An important clinical question not addressed so far is what thermal dose is needed to ensure therapeutically optimal sensitization of chemotherapy in cancer patients. Thermal dose is a measure of cumulative exposure of tissue to hyperthermia, reflecting temperature level and/or heat duration, and may be a prognostic factor in patients receiving thermochemotherapy. Over the past decades, several clinical studies using the combination of radiotherapy plus hyperthermia have demonstrated a thermal dose–effect relationship with cancer outcome in different tumor sites other than the bladder [Citation23–25]. The cumulative equivalent minutes at 43 °C (CEM43) a common method to report thermal dose applied by hyperthermia, which is a normalizing tool to integrate both temperature and time [Citation26]. CEM43 has shown to be a predictive thermal dose parameter in studies on radiotherapy in combination with hyperthermia [Citation23,Citation25,Citation27]. While clinical studies investigating a thermal dose response in thermochemotherapy are scarce [Citation15], there are a few reports that showed an association between thermal dose and improved cancer outcome. Patients with soft tissue sarcoma who responded to neoadjuvant chemotherapy combined with regional hyperthermia had a significantly higher median temperature, compared to non-responders (40.9 vs. 40.3 °C, p = 0.04) [Citation28]. Moreover, overall survival (OS) and progression-free survival (PFS) of patients with peritoneal carcinomatosis treated with hyperthermic intraperitoneal chemotherapy (HIPEC) was significantly enhanced if a temperature of >40 °C was reached [Citation29]. A thermal dose–effect relationship may depend on both the tumor site and the chemotherapeutic agent used.
It is unknown whether thermal dose is a prognostic factor for NMIBC patients treated with RF-CHT. Moreover, there is no uniform protocol for RF-CHT in NMIBC stating the minimal temperature and duration of hyperthermia per session. Here, we study whether NMIBC outcome is improved 1. by higher thermal dose (the higher the better), 2. by overcoming a certain thermal dose cutoff point (high- vs. low thermal dose group), or 3. by a higher fraction of treatment sessions that achieved a certain thermal dose ‘goal’.
Materials and methods
Ethical approval
The study follows the principles of the Declaration of Helsinki and the ethical committee of the RadboudUMC approved the study protocol under the number 2020-6097. Written informed consent was obtained from patients who were in follow-up at the time of conduction of the study and patient data were anonymized. Medical Research Involving Human Subjects Act (WMO) is not applicable due to the retrospective nature of this study.
Cohort and data collection
Temperature variables of RF-CHT sessions have been recorded in health records. We reviewed all medical records of NMIBC patients who started RF-CHT from the moment on our institution implemented an electronic health record system in November 2013 through January 2020. Patients were excluded who did not complete at least six treatment sessions (induction schedule) as well as patients who were not evaluable due to unavailable follow-up data within six months after start RF-CHT.
Follow-up
Cystoscopy and urinary cytology were performed every three months for two years and every two treatments from there on. Patients with CIS underwent biopsies at three or six months, in accordance with the EAU guidelines at the time.
RF-CHT sessions and treatment schedule
A treatment session consisted of two 30-min cycle with instillation of intravesical MMC or epirubicin, which was subsequently heated aiming at 40–43 °C to the extent that is tolerated by patients with regard to side effects (e.g., bladder spasms). In between the two cycles, intravesical chemotherapy was replaced to minimize dilution of the instillation with urine. In principle, patients received MMC and switched to epirubicin in case of an MMC allergy. Papillary tumors were commonly resected previous to induction treatment. Patients with CIS or unresected papillary tumor at baseline received an ablative dose (40 mg MMC or 50 mg epirubicin per cycle) and patients with resected papillary tumor an adjuvant dose (20 mg MMC or 30 mg epirubicin per cycle).
The induction schedule comprised six weekly RF-CHT sessions. In case of good response, patients continued to receive maintenance treatment sessions with six-week interval in the first year. After that, the interval between treatment sessions was extended on the basis on the urologist’s expertise. In general, the interval was eight weeks in the second year and 12 weeks thereafter. Since optimal treatment duration is unknown, treatment was continued two to three years or until patients showed recurrence or progression.
Hyperthermia device and thermometry
Bladder hyperthermia was accomplished using a Synergo SB-TS 101 System (Medical Enterprises Europe B.V., Amstelveen, the Netherlands) with an intravesical 915 MHz RF applicator, which is situated at the distal part of a three-way urinary catheter. Three copper-constantan (T-type) thermocouples with an accuracy of ± 0.1 °C are also incorporated in the catheter and are tangentially distended once the catheter balloon is inflated (). The temperature is measured every second during treatment, while RF stops one to two seconds every two minutes so that possible RF interference can be detected.
Thermal dose parameters
The following temperature and power parameters were recorded by the Synergo SB-TS 101 system for each RF-CHT session and were recorded in patients’ electronical health records: the mean temperature, the duration of a temperature of >40.5 °C, the duration of a temperature of >41.5 °C, the mean power of RF energy that the transmitter produced, and the total duration from instillation of chemotherapy to removal of chemotherapy.
CEM43T50 was estimated for each session based on the CEM43 in a point as described by Sapareto and Dewey [Citation26]:
where t is the time in minutes, T is the temperature in °C and the constant R = 0.25 for a temperature below 43 °C. CEM43T50 is defined as the CEM43 achieved in at least 50% of the measurement points. We used the mean temperature as approximation to the median temperature distribution (T50). Therefore, the estimated median CEM43T50 was based on mean temperature and duration:
where D is the treatment duration in minutes, Tmean the mean temperature in °C and the constant R = 0.25 for temperatures below 43 °C.
The median thermal dose of multiple treatments per patient were used as variables for analyses, including median CEM43T50, Tmedian, median duration of a temperature of >40.5 °C, median duration of a temperature >41.5 °C and median power of RF energy that the transmitter produced. To prevent that intra-patient (or treatment-treatment) variability would be eliminated, we used the median interquartile range (IQR; thus variability between all treatments per patient) of Tmedian and CEM43T50 as separate variables. Moreover, we defined the following thermal dose ‘goals’: a mean temperature of >41.0 °C, a CEM43T50 of >2 min, a temperature >40.5 °C of >40 min and a temperature >41.5 °C of >10 min. Additional variables were the fraction (or percentage) of treatments that achieved these thermal dose ‘goals’. The sum of CEM43T50 was not used as a variable, since this variable is directly linked with prognosis as the treatment discontinued when patients showed recurrence or progression.
Objectives and endpoints
The objective is to study whether increased thermal dose improves NMIBC outcome. We hypothesized that either 1. increasing thermal dose or 2. overcoming a thermal cutoff point may improve outcome. Moreover, we studied whether 3. the percentage (or fraction) of treatments in which a thermal dose ‘goal’ (see previous section) was achieved was associated with NMIBC outcome.
The primary endpoints were CR at six months and durable response for patients with CIS or for patients with unresected papillary tumor at baseline, and RFS for patients with previous papillary tumors who received a complete TURBT. CR was defined as negative biopsies or negative cystoscopy and urine cytology. Durable response was estimated for responders from the moment of first evidence of CR. A recurrence was defined as histology of urothelial cancer or high-grade cytology for durable response and RFS. If a low-grade recurrence occurred once, this was not recorded as such and the papillary tumor was resected while the RF-CHT schedule continued, in line with the management of a low-grade recurrence during BCG treatment [Citation30]. Patients who did not have recurrence were censored at the date of last follow-up or death.
Secondary endpoints were PFS, OS and safety. Muscle-invasive and/or metastasized disease defined progression. The Statistics Netherlands (CBS) registry was utilized to determine whether patients had died [Citation31]. Regarding safety, the maximum severity of adverse events (AEs) was recorded according to the Common Terminology Criteria for Adverse Events (CTCAE) version 5.0, 2017. Tolerability was assessed using the number of patients that discontinued treatment due to Aes.
Statistical analysis
Descriptions of continuous variables were presented as medians and IQR. Thermal dose subgroups (high vs. low) were defined using the median value as cutoff point, in order to achieve optimal statistical power. Rates of durable response, RFS, PFS and OS were estimated with the Kaplan–Meier method. Subgroups were compared using a stratified log-rank test. The associations between thermal dose parameters and RFS, PFS and OS were determined with (uni- and multivariable) cox regression and are presented as unadjusted and adjusted hazard ratios (HR). Logistic regression (uni- and multivariable) was used to assess the associations between thermal dose parameters and CR, which are presented as unadjusted and adjusted odds ratios (OR). The association between thermal dose and the occurrence of side effects was also assessed with logistic regression. The means of the three bladder spasm groups were compared using one-way ANOVA with a post-hoc Tukey HSD test.
The effect of thermal dose parameters on NMIBC outcome (CR, RFS, PFS and OS) was first determined using univariable analyses and subsequently using multivariable analyses adjusting for baseline confounders. Since univariable analysis showed no relationship between any of these parameters and NMIBC outcome (see results section), we chose to include the following thermal dose parameters to include in multivariable analyses: Tmedian together with intra-patient variability of temperature and median CEM43T50 together with intra-patient variability of CEM43T50. CEM43 has shown to be a predictive thermal dose parameter in studies on radiotherapy in combination with hyperthermia [Citation23,Citation25,Citation27]. The following clinical baseline patient characteristics were also included in multivariable analysis, if applicable: CIS status, stage (T1 vs. Ta/CIS), previous recurrence rate (≥1 vs. <1/year), BCG failure and chemotherapy dose (ablative or adjuvant). No multivariable analysis was performed for six-month complete response of papillary patients, since this subgroup consisted of only 11 patients.
The level of statistical significance was considered <0.05. IBM SPSS Statistics version 25.0 (Armonk, NY, USA) was used for statistical analysis. Microsoft PowerPoint for Microsoft 365 MSO (16.0.14326.21092) was used for figures.
Results
Patients
Between November 2013 and January 2020, 128 NMIBC patients started RF-CHT. Twenty patients were excluded because patients received less than six RF-CHT sessions (n = 12) or had no follow-up data available at three or six months (n = 8). The study cohort thus comprised 108 patients and for all patients thermal dose data were available.
Baseline patient and tumor characteristics are presented in and shows a flow-chart of the study. Any papillary tumors were resected prior to RF-CHT in all patients, except for 11 patients. All patients with unresected papillary tumor at baseline received adjuvant dose. All patients with low grade papillary tumors had a high recurrence rate of >1 recurrence per year.
Table 1. Baseline characteristics of the study population.
Thermal dose descriptions
A total of 1875 RF-CHT sessions have been carried out in 108 patients. Patients received a median of 15.0 (IQR 9.0–23.0) sessions. The median session duration, from administration of chemotherapeutic agent (prior to induction of hyperthermia) to removal of chemotherapy (after completing hyperthermia) was 49.0 (IQR 48.0–50.0) minutes. All patients received a Tmedian above 40.0 °C, and 97.2% (105/108) a Tmedian above 40.5 °C. All patients were treated with a median duration of at least 45 min.
For each thermal dose parameter per session, the median and IQR is shown in . Tmedian and median CEM43T50 per session were 40.9 (IQR 40.8–41.1) °C and 3.1 (IQR 2.4–3.6) minutes, respectively. Median intra-patient variability (IQR) of temperature and of CEM43T50 was 0.4 (IQR 0.3–0.5) °C and 1.4 (IQR 0.9–2.4) minutes, respectively. In Supplementary Figure 1, the mean temperature (A) and the CEM43T50 (B) of each session for each patient is depicted in a scatterplot. The fraction of sessions in which the thermal dose ‘goals’ (as defined in the method section) have been achieved, are summarized in . The median percentage of sessions in which the goals ‘mean temperature >41.0 °C’ and ‘CEM43T50 > 2 min’ were achieved are 47.7 (IQR 25.0–68.4) and 83.3 (IQR 68.1–93.2) %. The median sum of CEM43T50 of total sessions per patient was 47.16 (IQR 26.0–79.0) minutes.
Table 2. Descriptions of thermal dose parameters.
Table 3. Descriptions of fraction of thermal dose ‘goals’ achieved of multiple sessions per patient.
NMIBC outcome
Median follow-up was 47 months. The six-month CR rate of (concomitant) CIS patients and patients with unresected papillary tumor was 60.3% (38/63) and 36.4% (4/11), respectively. Durable response rates of CIS patients at 1 and 2 years were 93.7 (95% CI 85.3–102.1) and 81.3% (95% CI 66.2–96.4), respectively. RFS rates of papillary patients at these timepoints were 91.6 (95% CI 82.6–100.6) and 85.2% (95% CI 73.2–97.2), respectively. In total, 10 patients (9.3%) showed progression to muscle invasive disease and/or metastasized. One-year PFS was 91.9 (95% CI 86.4–97.4) and two-year PFS was 93.4% (95% CI 88.1–98.7). OS at two and five years was 91.9 (95% CI 86.4–97.4) and 80.1% (95% CI 70.5–89.7), respectively.
After RF-CHT, 30 patients (27.8%) received a cystectomy with or without neoadjuvant chemotherapy and three patients (2.8%) chemoradiation. Thus, the bladder preservation rate was 72.2%.
Effect of thermal dose on NMIBC outcome
No significant association between any of the thermal dose parameters as continuous variable and 6-mo CR, RFS, PFS or OS was observed in univariable analysis (p > 0.05, ). Multivariable analysis showed no association between median CEM43T50, intra-patient variability of CEM43T50, Tmedian or intra-patient variability of temperature and 6-mo CR, RFS, PFS or OS in (Supplementary Table 1). Moreover, no difference in these endpoints between high- and low thermal dose groups was observed, using the median thermal dose as cutoff point (Supplementary Table 2. Additionally, the fraction of sessions in which a thermal dose ‘goal’ was achieved (described in the method section) was not associated with 6-mo CR, RFS, PFS or OS (Supplementary Table 3).
Table 4. Unadjusted odds ratios (OR) and hazard ratios (HR) with corresponding p-values for complete response at six months (6-mo CR; for CIS patients [CIS] and for papillary patients [papillary]), recurrence-free survival (RFS), progression-free survival (PFS) and overall survival (OS).
Safety and tolerability
Adverse events were observed in 100 (92.6%) patients, with the maximal reported grade being moderate (n = 63, 58.3%) or mild (n = 35, 32.4%). Detailed frequencies of adverse events by severity are shown in Supplementary Table 4. The most frequently observed adverse events were bladder spasms during RF-CHT (n = 74, 68.5%), dysuria (n = 43, 39.8%) and hematuria (n = 36, 33.3%). Severe adverse events were reported for two patients (1.9%), which both involved urosepsis requiring hospitalization. No grade 4 or 5 adverse events related to RF-CHT were reported. Anticholinergics were used around RF-CHT sessions by 26 patients (24.1%) to limit side effects. Eleven patients (10.2%) discontinued treatment due to side effects and were deemed intolerant.
Association between thermal dose and side effects
Patients who did experience bladder spasms during RF-CHT were more likely to receive a lower thermal dose: Tmedian, CEM43T50, duration >40.5 °C and duration >41.5 °C were all associated with lower occurrence of bladder spasms during RF-CHT. Even after correcting for baseline patient characteristics in multivariable analysis, these associations remained significant. The ORs and corresponding p-values are presented in . Tmedian, CEM43T50, duration >40.5 °C and duration >41.5 °C of patients who experienced no bladder spasms was significantly different than patients who had mild (CTCAE grade 1, no intervention was indicated; p < 0.001) or moderate (CTCAE grade 2, anticholinergics were prescribed; p < 0.001); however, thermal dose of patients with mild bladder spasms was not significantly different patients with moderate bladder spasms (p > 0.05). Distribution of patients in whom bladder spasms occurred by Tmedian groups and median CEM43T50 groups are depicted in . There was no association found between thermal dose and occurrence of other adverse events (data not shown).
Figure 3. Distribution of patients in whom bladder spasms occurred by A. Tmedian and B. median CEM43T50 groups.
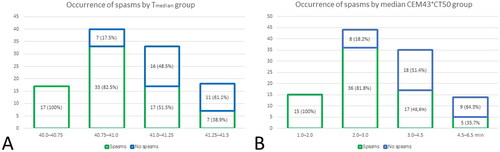
Table 5. Association between thermal dose parameters and the occurrence of bladder spasms, presented as odds ratio (OR) with corresponding p-value.
Discussion
Our aim was to study the effect of thermal dose of RF-CHT on NMIBC outcome, in order to improve treatment strategy. To our knowledge, we are the first to study the association between thermal dose and NMIBC outcome in a clinical cohort. Patients received a Tmedian of 40.9 (IQR 40.8–41.1) °C and a median CEM43T50 of 3.1 (IQR 2.4–3.6) minutes per session. No significant association between any thermal dose parameter as a continuous variable and outcome (6-mo CR, RFS, PFS or OS) in the clinically observed thermal dose range. Also no significant difference was seen between patients in the high- or low-thermal dose group using the median as cutoff point. Additionally, the fraction of sessions in which a thermal dose ‘goal’ was achieved (defined in the method section) was not associated with improved outcome. In contrast, occurrence of bladder spasms during RF-CHT had a significant dose limiting effect. Adjusted ORs of Tmedian and median CEM43T50 were 0.01 (95% CI 0.00–0.01, p < 0.001) and 0.34 (95% CI 0.20–0.56, p < 0.001), respectively.
A number of factors may explain why no significant correlation between thermal dose and NMBIC outcome was found in the current study. Firstly, because the number of treatment sessions differed we used the median CEM43T50 of multiple treatment sessions per patient instead of the sum of CEM43T50, while the latter has shown to be associated with improved outcome of cervical carcinoma [Citation23,Citation32]. Treatment continued two to three years (optimal treatment duration is unknown) or until NMIBC would recur or progress. Hence, the sum of CEM43T50 is closely linked to the number of treatments and thus to the time to recurrence or progression. Using the median thermal dose of multiple treatments per patient might lead to less precise calculations. However, by using the intra-patient variability as separate variable this risk was minimized. Also, we only had access to time-averaged temperature and power data per session, not to the detailed temperature over time and therefore had to estimate the CEM43T50 per session. Moreover, thermometry might have been suboptimal since the thermocouples that are incorporated in the urinary catheter may cover only the part of the bladder wall close to the distal tip of the catheter, while the bladder wall is known to be heated inhomogeneously [Citation33].
Furthermore, there is an ongoing discussion which thermal dose parameter is an appropriate clinical parameter. For example, the most commonly used CEM43 is a normalizing tool expressing thermal exposure in ‘equivalent minutes’ at 43 °C and is mainly based on in vitro experiments with mostly non-human cell lines while in vivo studies with human cancers are extremely rare [Citation34]. Moreover, temperatures in studies evaluating CEM43 range from 41.5 °C (murine) or 44 °C (human) and up, leaving uncertainty about the slope of the Arrhenius plot (constant R) below 43 °C [Citation35,Citation36]. Clinical temperatures are usually below 43 °C because of the need to limit side effects [Citation15]. Moreover, this normalizing tool does not account for dissimilar heat sensitivity of different (tumor) tissues, varying synergy between chemotherapeutic agents and heat and other relevant clinical effects from hyperthermia. Alternative thermal dose parameters have been studied, including TRISE (a product median temperature rise >37 °C and duration of treatment for all sessions, normalized to 450 min), area under the curve (AUC), Tmean, Tmax, Tmin or percentiles T90 T50 and T10, but these also do not cover the previously mentioned issues. Lastly, it is the question whether there is a strong dose-response relationship between thermal dose and outcome in the clinical setting. Issels et al. proposed six hallmarks of hyperthermia: induction of an immune response, enhanced drug delivery, blocking cell survival, evading DNA repair, inducing a cellular stress response and sensitization to chemotherapy or radiotherapy [Citation37]. Induction of an immune response and enhanced drug delivery to tumor tissue caused by increased perfusion and drug extravasation presumably require exceeding a low temperature threshold of 39–40 °C and do not advance much with increasing thermal dose [Citation15]. The subsequent four biological effects may get larger with increasing thermal dose but might as well achieve saturation when exceeding a certain thermal dose. Since the median temperature of multiple treatments per patient was 40.93 °C and intra-patient variability of temperature was 0.38 °C (IQR 0.29–0.52), a thermal dose threshold of 40.5 °C or lower would be impossible to detect. However, this temperature range showed a significant correlation with the occurrence of bladder spams, which may be unrelated to the thermal dose effect on NMIBC.
Results of previously published research regarding thermal dose-effect on cancer outcome are not consistent and have a retrospective design. For radiotherapy plus hyperthermia, a few studies found a clear thermal dose-effect relationship with cancer outcome for cervical carcinoma or breast carcinoma: the higher the thermal dose, the better the outcome [Citation23,Citation25]. Some authors demonstrated improved outcome above certain thermal dose cutoff points for cervical, breast and prostate carcinoma [Citation24,Citation32,Citation38,Citation39]. In contrast, other breast cancer trials could not identify a thermal dose–effect relationship [Citation40–42]. Datta et al. evaluated 18 MIBC patients, who were deemed unfit for radical cystectomy and were alternatively treated with radiotherapy and hyperthermia followed by TURBT [Citation34]. The author concluded that the two patients with local recurrence had significantly lower CEM43 and AUC than patients without recurrence. Only few studies using thermochemotherapy investigated thermal dose related to cancer outcome. Three reports included soft tissue sarcoma patients who received neoadjuvant thermochemotherapy [Citation28,Citation43] or thermochemotherapy alternatively to surgery [Citation44]. Hereof two studies showed higher thermal dose in responders than non-responders (T50 40.8 °C vs. 39.4 °C, p = 0.006 [Citation44]; and T50 40.9 °C vs. 40.3 °C, p = 0.04 [Citation28]), while one study showed no correlation between thermal dose and outcome [Citation43]. Moreover, patients with peritoneal carcinomatosis treated with hyperthermic intraperitoneal chemotherapy (HIPEC) had significantly better OS and PFS if a temperature of >40 °C for at least 40 min was reached [Citation29].
This study demonstrates that an acceptable and reproducible thermal dose was achieved in this patient cohort. In vitro, the cytotoxic effect of MMC and epirubicin on bladder cancer cells is increased when it is combined with mild hyperthermia at 43 °C, which is slightly higher than the clinical temperatures achieved in this study [Citation13]. The thermal dose presented in other clinical studies is within the same range as in the current study. Much higher clinical thermal dose is possibly difficult to achieve without inducing significant side effects. Following a recent review, a reasonable clinical thermal dose with limited toxicity is 40–42 °C for 30–60 min, that is, equivalent to a CEM43 of 1–15 min [Citation15]. Despite that in the current study no thermal dose effect was found, RCTs have shown that NMIBC patients benefit from RF-CHT with MMC, compared to MMC instillations without hyperthermia [Citation12,Citation19] and compared to BCG [Citation20]. We hypothesize that thermal dose achieved in these studies including NMIBC patients are in the same range as achieved in the current study as the treatment protocol is similar. As a possible therapeutic thermal dose effect may include overcoming a cutoff value below the achieved range in the current study, we advise to continue treating patients with a thermal dose of >40.5 °C for >45 min, which was achieved in 97.2% of the patients in this cohort.
Previously, a few studies with very small sample sizes reported thermal dose achieved by deep regional hyperthermia combined with intravesical chemotherapy for NMIBC. One pilot study demonstrated a median bladder temperature of 41.6 °C (40.6–42.1) in 18 NMIBC patients, while heating duration was not mentioned [Citation45]. Another study reported that 42 °C for 10–12 min was achieved in 15 NMIBC patients included in the study; however, more details of thermal dose were not presented [Citation46]. A third study described a median temperature of 40.3 ± 1.2 °C in the bladder lumen of NMIBC patients, while duration was not mentioned [Citation47]. Lastly, a study discussed detailed thermal dosimetry of 14 NMIBC patients and showed a bladder tissue temperature of 40 °C or higher for 61.9 ± 11.4 min and a mean thermal dose of 21.3 ± 16.5 CEM43 per session [Citation48]. The thermal dose presented in these studies using external RF with are similar to somewhat higher (the latter study) compared to the present study. Besides the method of heating (external RF vs. intravesical RF), also the location of thermometry probes differed. Unfortunately, the thermal dose reported in these small studies was not correlated with NMIBC outcome.
To our knowledge, no study reported thermal dose achieved by intravesical RF or heat conduction in NMIBC patients so far. Thus, thermal dose achieved in the bladder wall by external RF, intravesical RF or intravesical heat conduction has also not been directly compared. In a porcine bladder cancer model, the bladder wall is inhomogeneously heated after both intravesical RF and intravesical heat conduction [Citation33]. Mean temperature was higher in the bladder neck after intravesical RF compared to intravesical heat conduction, whereas the mean temperature in the other parts of the bladder were not significantly different comparing both heating techniques. A recent RCT showed that instillation of MMC combined with intravesical hyperthermia by conduction was not superior to MMC instillation without hyperthermia [Citation49]. In contrast, two RCTs demonstrated increased effect from RF-CHT with MMC compared to instillation of MMC alone [Citation12,Citation19], suggesting that hyperthermia induced by intravesical radiofrequency might be superior to conductive heating. External RF is not routinely used against NMIBC or MIBC. External RF may result in more complete bladder wall heating, but limitations of external RF are that complex technology and extensive support (such as more extensive thermal monitoring or patient specific deep heating planning) are required, and importantly, a significant amount of benign tissue surrounding the bladder is included in the heated region [Citation50].
The dose-limiting effect from the occurrence of bladder spasms can be explained by the fact that the temperature is manually lowered in case bladder spasms occur during hyperthermia. Bladder spasms may be induced by stimulation of sensitive sensory neurons in the bladder epithelium which influence the micturition reflex [Citation51]. Therefore, patients that do not have bladder spasms are more likely to receive a higher thermal dose. Individual tolerability, including bladder spasms, must be respected.
Strengths of this study are that, we are the first to study the association between thermal dose and NMIBC outcome. Reasonable thermal dose was achieved in the vast majority of treatment sessions.
Limitations of the current study are those associated with a retrospective study design, such as risk of selection and information bias, and underreporting of side effects. Treatment intervals and number of treatments differed between patients. Thermometry was restricted to measurements of three thermocouples close to the distal catheter tip. Moreover, we had limited access to detailed thermometry results and used averaged thermal dose parameters.
In conclusion, no significant association between thermal dose as and NMIBC outcome was found. This could be explained by the relatively uniform temperature range patients were treated with, access to only averaged thermal dose data, or by our hypothesis that a thermal dose effect might involve passing a certain threshold value which might already have been reached in patients in the current study. On the other hand, occurrence of bladder spasms had a dose-limiting effect. We advise to treat patients with a temperature of >40.5 °C for at least 45 min while respecting individual tolerability, including occurrence of bladder spasms. Future research on a thermal dose effect relationship requires high quality thermometry, strict treatment schedules with fixed intervals and number of treatment sessions.
Supplemental Material
Download PDF (321 KB)Acknowledgments
The authors thank Sophie Witjes, Yvonne Wimper and Tom Arends for support with database.
Disclosure statement
No potential conflict of interest was reported by the author(s).
References
- GLOBOCAN estimated cancer incidence, mortality, and prevalence worldwide in 2018. 2018 [accessed 2022 Dec]. Available from: http://globocan.iarc.fr
- Mossanen M, Gore JL. The burden of bladder cancer care: direct and indirect costs. Curr Opin Urol. 2014;24(5):487–491.
- Babjuk M, Burger M, Compérat E, et al. EAU Guideline Non-muscle-invasive Bladder Cancer. Presented at the EAU Annual Congress Amsterdam. 2020. http://uroweb.org/guidelines/compilations-of-all-guidelines/
- Cambier S, Sylvester RJ, Collette L, et al. EORTC nomograms and risk groups for predicting recurrence, progression, and disease-specific and overall survival in Non-Muscle-invasive stage Ta-T1 urothelial bladder cancer patients treated with 1-3 years of maintenance bacillus Calmette-Guérin. Eur Urol. 2016;69(1):60–69.
- Guallar-Garrido S, Julián E. Bacillus Calmette-Guérin (BCG) therapy for bladder cancer: an update. Immunotargets Ther. 2020;9:1–11.
- Aziz A, May M, Burger M, PROMETRICS 2011 research group, et al. Prediction of 90-day mortality after radical cystectomy for bladder cancer in a prospective european multicenter cohort. Eur Urol. 2014;66(1):156–163.
- Nielsen ME, Mallin K, Weaver MA, et al. Association of hospital volume with conditional 90-day mortality after cystectomy: an analysis of the national cancer data base. BJU Int. 2014;114(1):46–55.
- Hautmann RE, de Petriconi RC, Volkmer BG. Lessons learned from 1,000 neobladders: the 90-day complication rate. J Urol. 2010;184(3):990–994; quiz 1235.
- Parker WP, Smelser W, Lee EK, et al. Utilization and outcomes of radical cystectomy for high-grade non-muscle-invasive bladder cancer in elderly patients. Clin Genitourin Cancer. 2017; S1558-7673(17)30208-2.
- Issels RD, Lindner LH, Verweij J, European Organization for the Research and Treatment of Cancer-Soft Tissue and Bone Sarcoma Group and the European Society for Hyperthermic Oncology, et al. Effect of neoadjuvant chemotherapy plus regional hyperthermia on long-term outcomes among patients with localized High-Risk soft tissue sarcoma: the EORTC 62961-ESHO 95 randomized clinical trial. JAMA Oncol. 2018;4(4):483–492.
- van Driel WJ, Koole SN, Sikorska K, et al. Hyperthermic intraperitoneal chemotherapy in ovarian cancer. N Engl J Med. 2018;378(3):230–240.
- Colombo R, Salonia A, Leib Z, et al. Long-term outcomes of a randomized controlled trial comparing thermochemotherapy with mitomycin-C alone as adjuvant treatment for non-muscle-invasive bladder cancer (NMIBC). BJU Int. 2011;107(6):912–918.
- van der Heijden AG, Verhaegh G, Jansen CF, et al. Effect of hyperthermia on the cytotoxicity of 4 chemotherapeutic agents currently used for the treatment of transitional cell carcinoma of the bladder: an in vitro study. J Urol. 2005;173(4):1375–1380.
- van Valenberg FJP, van der Heijden AG, Lammers RJM, et al. Intravesical radiofrequency induced hyperthermia enhances mitomycin C accumulation in tumour tissue. Int J Hyperthermia. 2017;34:988–993.
- van Rhoon GC, Franckena M, Ten Hagen TLM. A moderate thermal dose is sufficient for effective free and TSL based thermochemotherapy. Adv Drug Deliv Rev. 2020;163-164:145–156.
- Arends TJ, Falke J, Lammers RJ, et al. Urinary cytokines in patients treated with intravesical mitomycin-C with and without hyperthermia. World J Urol. 2015;33(10):1411–1417.
- van Valenberg H, Colombo R, Witjes F. Intravesical radiofrequency-induced hyperthermia combined with chemotherapy for non-muscle-invasive bladder cancer. Int J Hyperthermia. 2016;32(4):351–362.
- Liem EI, Crezee H, de la Rosette JJ, et al. Chemohyperthermia in non-muscle-invasive bladder cancer: an overview of the literature and recommendations. Int J Hyperthermia. 2016;32(4):363–373.
- Colombo R, Da Pozzo LF, Salonia A, et al. Multicentric study comparing intravesical chemotherapy alone and with local microwave hyperthermia for prophylaxis of recurrence of superficial transitional cell carcinoma. J Clin Oncol. 2003;21(23):4270–4276.
- Arends TJ, Nativ O, Maffezzini M, et al. Results of a randomised controlled trial comparing intravesical chemohyperthermia with mitomycin C versus bacillus Calmette-Guerin for adjuvant treatment of patients with intermediate- and high-risk Non-Muscle-invasive bladder cancer. Eur Urol. 2016;69(6):1046–1052.
- Tan WS, Panchal A, Buckley L, et al. Radiofrequency-induced thermo-chemotherapy effect versus a second course of bacillus Calmette-Guérin or institutional standard in patients with recurrence of non-muscle-invasive bladder cancer following induction or maintenance bacillus Calmette-Guérin therapy (HYMN): a phase III, open-label, randomised controlled trial. Eur Urol. 2019;75(1):63–71.
- Brummelhuis ISG, Wimper Y, Witjes-van Os H, et al. Long-Term experience with Radiofrequency-Induced hyperthermia combined with intravesical chemotherapy for Non-Muscle invasive bladder cancer. Cancers (Basel). 2021;13(3):377.
- Franckena M, Fatehi D, de Bruijne M, et al. Hyperthermia dose-effect relationship in 420 patients with cervical cancer treated with combined radiotherapy and hyperthermia. Eur J Cancer. 2009;45(11):1969–1978.
- Refaat T, Sachdev S, Sathiaseelan V, et al. Hyperthermia and radiation therapy for locally advanced or recurrent breast cancer. Breast. 2015;24(4):418–425.
- Kroesen M, Mulder HT, van Holthe JML, et al. Confirmation of thermal dose as a predictor of local control in cervical carcinoma patients treated with state-of-the-art radiation therapy and hyperthermia. Radiother Oncol. 2019;140:150–158.
- Sapareto SA, Dewey WC. Thermal dose determination in cancer therapy. Int J Radiat Oncol Biol Phys. 1984;10(6):787–800.
- Bakker A, van der Zee J, van Tienhoven G, et al. Temperature and thermal dose during radiotherapy and hyperthermia for recurrent breast cancer are related to clinical outcome and thermal toxicity: a systematic review. Int J Hyperthermia. 2019;36(1):1024–1039.
- Wendtner CM, Abdel-Rahman S, Krych M, et al. Response to neoadjuvant chemotherapy combined with regional hyperthermia predicts long-term survival for adult patients with retroperitoneal and visceral high-risk soft tissue sarcomas. J Clin Oncol. 2002;20(14):3156–3164.
- Schaaf L, van der Kuip H, Zopf W, et al. A temperature of 40 °C appears to be a critical threshold for potentiating cytotoxic chemotherapy in vitro and in peritoneal carcinomatosis patients undergoing HIPEC. Ann Surg Oncol. 2015;22 Suppl 3: s758–s765.
- Bacillus Calmette-Guérin-Unresponsive Nonmuscle Invasive Bladder Cancer: Developing Drugs and Biologics for Treatment. Available at: https://www.fda.gov/regulatory-information/search-fda-guidance-documents/bacillus-calmette-guerin-unresponsive-nonmuscle-invasive-bladder-cancer-developing-drugs-and. (Accessed 6th of September)
- Datum van overlijden van personen die ingeschreven staan in de GBA. Available at: https://www.cbs.nl/.
- Ohguri T, Harima Y, Imada H, et al. Relationships between thermal dose parameters and the efficacy of definitive chemoradiotherapy plus regional hyperthermia in the treatment of locally advanced cervical cancer: data from a multicentre randomised clinical trial. Int J Hyperthermia. 2018;34(4):461–468.
- van Valenberg FJP, Witjes JA, Aklan B, et al. Inducing intravesical hyperthermia of the ex-vivo porcine bladder wall: radiofrequency-induction versus recirculation using a custom-made device. Int J Hyperthermia. 2018;35(1):323–329.
- Datta NR, Marder D, Datta S, et al. Quantification of thermal dose in moderate clinical hyperthermia with radiotherapy: a relook using temperature-time area under the curve (AUC). Int J Hyperthermia. 2021;38(1):296–307.
- Dewhirst MW, Viglianti BL, Lora-Michiels M, et al. Basic principles of thermal dosimetry and thermal thresholds for tissue damage from hyperthermia. Int J Hyperthermia. 2003;19(3):267–294.
- Field SB, Morris CC. The relationship between heating time and temperature: its relevance to clinical hyperthermia. Radiother Oncol. 1983;1(2):179–186.
- Issels R, Kampmann E, Kanaar R, et al. Hallmarks of hyperthermia in driving the future of clinical hyperthermia as targeted therapy: translation into clinical application. Int J Hyperthermia. 2016;32(1):89–95.
- Bakker A, Tello Valverde CP, van Tienhoven G, et al. Post-operative re-irradiation with hyperthermia in locoregional breast cancer recurrence: temperature matters. Radiother Oncol. 2022;167:149–157.
- Nakahara S, Ohguri T, Kakinouchi S, et al. Intensity-Modulated radiotherapy with regional hyperthermia for High-Risk localized prostate carcinoma. Cancers (Basel). 2022;14(2):400.
- de Bruijne M, van der Holt B, van Rhoon GC, et al. Evaluation of CEM43 degrees CT90 thermal dose in superficial hyperthermia: a retrospective analysis. Strahlenther Onkol. 2010;186(8):436–443.
- Oldenborg S, Griesdoorn V, van Os R, et al. Reirradiation and hyperthermia for irresectable locoregional recurrent breast cancer in previously irradiated area: size matters. Radiother Oncol. 2015;117(2):223–228.
- Linthorst M, Baaijens M, Wiggenraad R, et al. Local control rate after the combination of re-irradiation and hyperthermia for irresectable recurrent breast cancer: results in 248 patients. Radiother Oncol. 2015;117(2):217–222.
- Stahl R, Wang T, Lindner LH, et al. Comparison of radiological and pathohistological response to neoadjuvant chemotherapy combined with regional hyperthermia (RHT) and study of response dependence on the applied thermal parameters in patients with soft tissue sarcomas (STS). Int J Hyperthermia. 2009;25(4):289–298.
- Issels RD, Prenninger SW, Nagele A, et al. Ifosfamide plus etoposide combined with regional hyperthermia in patients with locally advanced sarcomas: a phase II study. J Clin Oncol. 1990;8(11):1818–1829.
- Geijsen ED, de Reijke TM, Koning CC, et al. Combining mitomycin C and regional 70 MHz hyperthermia in patients with nonmuscle invasive bladder cancer: a pilot study. J Urol. 2015;194(5):1202–1208.
- Inman BA, Stauffer PR, Craciunescu OA, et al. A pilot clinical trial of intravesical mitomycin-C and external deep pelvic hyperthermia for non-muscle-invasive bladder cancer. Int J Hyperthermia. 2014;30(3):171–175.
- Fatehi D, van der Zee J, Notenboom A, et al. Comparison of intratumor and intraluminal temperatures during locoregional deep hyperthermia of pelvic tumors. Strahlenther Onkol. 2007;183(9):479–486.
- Juang T, Stauffer PR, Craciunescu OA, et al. Thermal dosimetry characteristics of deep regional heating of non-muscle invasive bladder cancer. Int J Hyperthermia. 2014;30(3):176–183.
- Tan WS, Prendergast A, Ackerman C, et al. Adjuvant intravesical chemohyperthermia versus passive chemotherapy in patients with intermediate-risk non-muscle-invasive bladder cancer (HIVEC-II): a phase 2, open-label, randomised controlled trial. Eur Urol. 2022; S0302-2838(22)02552-0.
- Stauffer PR, van Rhoon GC. Overview of bladder heating technology: matching capabilities with clinical requirements. Int J Hyperthermia. 2016;32(4):407–416.
- Gonzalez EJ, Merrill L, Vizzard MA. Bladder sensory physiology: neuroactive compounds and receptors, sensory transducers, and target-derived growth factors as targets to improve function. Am J Physiol Regul Integr Comp Physiol. 2014;306(12):R869–878.