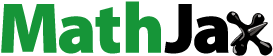
Abstract
Background
Augmented reality (AR) has become a promising tool in neurosurgery. It can minimise the anatomical challenges faced by conventional endoscopic or microscopic transsphenoidal reoperations and can assist in intraoperative guidance, preoperative planning, and surgical training.
Objectives
The aims of this systematic review are to describe, compare, and evaluate the use of AR in endoscopic and microscopic transsphenoidal surgery, incorporating the latest primary research.
Methods
A systematic review was performed to explore and evaluate existing primary evidence for using AR in transsphenoidal surgery. A comprehensive search of MEDLINE and EMBASE was conducted from database inception to 11th August 2021 for primary data on the use of AR in microscopic and endoscopic endonasal skull base surgery. Additional articles were identified through searches on PubMed, Google Scholar, JSTOR, SCOPUS, Web of Science, Engineering Village, IEEE transactions, and HDAS. A synthesis without meta-analysis (SWiM) analysis was employed quantitatively and qualitatively on the impact of AR on landmark identification, intraoperative navigation, accuracy, time, surgeon experience, and patient outcomes.
Results
In this systematic review, 17 studies were included in the final analysis. The main findings were that AR provides a convincing improvement to landmark identification, intraoperative navigation, and surgeon experience in transsphenoidal surgery, with a further positive effect on accuracy and time. It did not demonstrate a convincing positive effect on patient outcomes. No studies reported comparative mortalities, morbidities, or cost-benefit indications.
Conclusion
AR-guided transsphenoidal surgery, both endoscopic and microscopic, is associated with an overall improvement in the areas of intraoperative guidance and surgeon experience as compared with their conventional counterparts. However, literature on this area, particularly comparative data and evidence, is very limited. More studies with similar methodologies and quantitative outcomes are required to perform appropriate meta-analyses and to draw significant conclusions.
Introduction
The clinical use of augmented reality (AR) in neurosurgery was first described by Kelly et al. and Roberts et al. in the 1980s,Citation1,Citation2 laying the developmental foundations of novel neurosurgical AR devices and culminating in the commercialisation of the head-up display (HUD) microscopes in the 1990s.Citation3 Forty years after Roberts & Kelly, AR has become a promising tool in neurosurgery that is growing in popularity, enhancing safety and efficiency.Citation4,Citation5 Studies have shown that AR successfully improves the optical image produced by endoscopes and microscopes through virtual overlays that provide a real-time 3D (3 dimensional) anatomical model. In doing so, AR aids the surgeon in the correct localisation of surgical landmarks and intra-operative navigation, particularly in more complex neurosurgical cases with a narrow visual field such as minimally invasive skull base surgery. Intrinsically, the development of neurosurgical AR systems contain components that assist in preoperative planning to varying degrees.Citation6 On one end, the manual marking on imaging for rendering AR allows the surgeon better familiarisation with the operative anatomy prior to the procedure. On the other end, the combination of AR with a hands-on hybrid model allows for enhanced preparation and planning of a specific procedure. Such simulations have significant scope in training systems also and are increasing in popularity.Citation6
One of the most commonly performed minimally invasive neurosurgical procedures is transsphenoidal endoscopic endonasal surgery (ETS) and microscopic transsphenoidal surgery (MTS), used commonly for the resection of pituitary tumours.Citation7–9 However, despite the evident postoperative benefits that come with performing procedures in a minimally invasive manner, transsphenoidal surgery (TS) still faces numerous perioperative and postoperative hurdles, especially as compared with an open transcranial surgical (OTCS) approach. The technically demanding nature (whereby the operator must navigate through a significantly dense area whilst avoiding critical neurovascular structures that are in close proximity to the pathology of interest) suggests that TS may be the ideal candidate for AR guidance. As the identification of key landmark structures is critical throughout the entirety of a transsphenoidal procedure, utilising AR and the benefits that it may bring to structure visualisation provides an opportunity to improve patient safety and may be less disruptive to the surgical workflow than the current adjuncts (such as microvascular Doppler ultrasonography or neuronavigation systems).
However, the limited line of sight of transsphenoidal procedures that results from the minimally invasive approach remains a critical hurdle that is yet to be adequately overcome. It can hinder the operator in being able to visualise structural margins sufficiently, which has the potential to lead to ETS and MTS having lower rates of gross tumour resection with regards to tumours with a high recurrence rate, namely giant or locally invasive pituitary macroadenomas.Citation9–11 In fact, research has suggested that in many cases, ETS and MTS are unable to resect larger pituitary tumours without leaving residuals, resulting in repeat surgeries. From this stems a further significant challenge in that both transsphenoidal techniques remain unable to overcome the anatomical challenges imposed by repeat transsphenoidal surgeries: adhesions, fibrosis and severe distortions of surgical landmarks. Given the difficulties of incomplete resection resulting from a limited line of sight and the anatomical disruption from larger tumours; the subsequent challenges of repeat surgeries; and the technically demanding nature of transsphenoidal approaches, the enhancement of ETS and MTS by means of AR navigation and guidance tools is arguably the next promising step in the evolution of minimally invasive TS, which may enable it to overcome its current limitations.
The last significant systematic reviews on AR in neurosurgery were performed Cho et al.,Citation12 Lopez et al.,Citation13 and Meola et al..Citation14 Lopez et al. and Meola et al. concluded that the use of AR was likely safe and enhanced outcomes when operating on different neurosurgical pathologies.Citation13,Citation14 Cho et al.Citation12 positively advocated for further research to be conducted within the use of AR in neurosurgery, particularly within the scope of improved accuracy of registration systems.Citation12 However, all three studies only marginally addressed transsphenoidal surgery and did not explore the use of AR in endoscopic surgery adequately. Therefore, this systematic review aims to describe, compare, and evaluate the use of AR in endoscopic and microscopic transsphenoidal surgery incorporating the latest primary research.
Methods
Literature search
This systematic review was conducted following the Cochrane Collaboration guidelinesCitation15 and Preferred Reporting Items for Systematic Reviews and Meta-Analyses (PRISMA).Citation16 A comprehensive search of MEDLINE and Excerpta Medica dataBASE (EMBASE) was conducted from 1860 to August 2021. The search string consisted of the search term search string “((augmented reality OR virtual reality OR extended reality OR mixed reality) AND (transsphenoidal or endoscopic or endonasal or anterior skull base or skull base or transsphenoidal))”. Additional articles were identified through manual searches on PubMed, Google Scholar and JSTOR. Furthermore, SCOPUS, Web of Science, Engineering Village, IEEE transactions, Healthcare Databases Advanced Search (HDAS), PubMed, and Google Scholar were searched for AR in neurosurgery and sinus surgery using keywords “augmented reality and neurosurgery” and “augmented reality and sinus surgery”.
Study inclusion and exclusion criteria
All original articles in the English language, including those within biomedical engineering literature, reporting on the use of AR within ETS in ENT or neurosurgery were included. The sole inclusion criterion was studied reporting the use of transsphenoidal surgery with AR in humans in the context of ventral skull base surgery and sinus surgery, including patients (in vivo), cadaveric simulation models (ex vivo) or experimental models (in vitro). The exclusion criteria included: surgical simulation in virtual reality (VR) setting, the language of publication not English, the field of application not ventral skull base or sinus surgery, studies discussing commentaries, reviews, and abstracts. Studies reporting on the use of AR in simulation training for endoscopic endonasal surgery or using AR solely in conjunction with surgical robots were excluded as they were deemed to be outside the scope of this review.
Eligibility assessment, data extraction and quality assessment
Studies were evaluated for eligibility independently by two reviewers (SGT & RV). Disagreements were resolved by consensus after discussion with a third reviewer (ARA). Data were extracted using the Covidence data collection tool.Citation17 All randomised control trial studies were critically appraised using the revised tool for risk of bias in randomized trials (ROB2 tool),Citation18 non-randomised studies were appraised using the risk of bias in non-randomized studies - of interventions (ROBINS-I tool).Citation19 The following data points were extracted from each study: study author(s); date of publication; study design; sample size and type; assessed pathology of interest; AR methodology; AR technology; landmark identification reporting; accuracy results (physical, objective measurement of true vs AR-identified location in the three-dimensional space); intraoperative navigation results and reporting; operative and preoperative timings; patient complications; patient outcomes; and the surgeon experience.
Synthesis and analysis
In line with Cochrane Collaboration Guidelines, a synthesis without meta-analysis (SWiM) was employed.Citation20–22 Microscopic and endoscopic, then in vivo, ex vivo and in vitro were stratified according to the risk of bias (1 = critical risk, 2 = high risk, 3 = moderate risk, 4 = low risk). Finally, the direction of effect of each study was determined by investigators independently, after which a vote-counting based was conducted fully-blinded by three reviewers (SGT, RV, ARA), always unanimously reaching consensus.
Results
A total of 1847 abstracts papers were identified using our literature search strategy, of which 377 papers underwent full-text review and 17 studies were included (). The PRISMA study selection process is shown in . The ROBINS-I tool scored all included non-randomised studies (n = 14): nine studies at low risk, five studies at moderate risk, one study at serious risk, and no study at critical risk of bias overall ().Citation23–28,Citation30,Citation32–34,Citation36–39 The ROB2 tool scored all included randomised studies (n = 3) at low to moderate risk with no study scoring high risk of bias overall ().Citation29,Citation31,Citation35 In total, there were three studies that focussed only or partly on the use of AR-assisted microscopy,Citation24–26 and 14 studies for pure AR-assisted endoscopy.Citation23,Citation27–39 Furthermore, there were seven in vivo studies,Citation24,Citation25,Citation30,Citation32,Citation35,Citation37,Citation39 five ex vivo studies,Citation28,Citation29,Citation31,Citation34,Citation38 four in vitro studies,Citation26,Citation27,Citation33,Citation36 one mixed in vitro and ex vivo study.Citation23 Notably, four of these studies particularly discussed revision surgeries.Citation24,Citation25,Citation35,Citation37
Figure 1. Preferred Reporting Items for Systematic Reviews and Meta-Analyses (PRISMA) flowchart outlining the study selection process.

Figure 2. In (a) risk of bias summary plot for non-randomized studies with a bar chart of the distribution of risk-of-bias judgments for all included studies (n = 14) across the domains of the ROBINS-I tool, shown in percentages (%) is shown. At the bottom, the overall risk of bias, which represents the collated risk-of-bias judgements for all domains, is depicted. In (b) ROB2 risk of bias summary plot for randomized studies with a bar chart of the distribution of risk-of-bias judgments for all included studies (n = 3) across the domains of the ROB2 tool, shown in percentages (%) is shown. At the bottom, the overall risk of bias, which represents the collated risk-of-bias judgements for all domains, is depicted.

Table 1. Studies included assessing the use of AR in transsphenoidal surgery.
Augmented reality technology
Imaging
The most utilised imaging modality was computed tomography (CT), with six studies reporting exclusively using CT either pre- or postoperatively, or both.Citation23,Citation28,Citation30,Citation31,Citation34,Citation35 Two studies, namely Pennacchietti et al. and Onishi et al., reported using magnetic resonance imaging (MRI) as the sole imaging modality, which was fused by means of AR with the endoscopic view in both studies.Citation35,Citation36 Four studies reported the use of both MRI and CT.Citation24,Citation25,Citation32,Citation39 Notably among them, Caversaccio et al. and Carl et al. used CT or MRI angiography in addition, with Carl et al. furthermore using radiographic fluoroscopy. Cone beam computed tomography (CBCT) was employed by three studies, all of them reporting the additional use of C-arm technology.Citation31,Citation33,Citation38
Registration
Fiducial registration (FR) was the most commonly used registration method (n = 7).Citation24,Citation27,Citation30,Citation31,Citation34,Citation39 Of those, only Carl et al. additionally employed automated registration, using automatic intraoperative CT (iCT)-based registration. Four studies used surface registration as the sole registration method.Citation23,Citation26,Citation35,Citation36 Interestingly, Pennacchietti et al. employed a hybrid approach, combining surface-matching registration and anatomical registration. Being the only studies to employ each registration method, Citardi et al. and Prisman et al. used contour-based registration and in-house deformable CBCT registration, respectively. Caversaccio et al. registered the position of the head manually by means of using a needle pointer.
Segmentation
Four of the included studies, all non-in vivo studies, utilised manual segmentation as the sole segmentation technique, employing a variety of software.Citation29,Citation31,Citation33,Citation38 Dixon et al.Citation29 and Dixon et al.Citation31 used the ITK-SNAP 2.0 software,Citation38 whereas Lai et al. used a self-developed AR surgical navigation system. Four studies used purely automated segmentation,Citation23,Citation25,Citation26,Citation32 with Lapeer et al. using the ARView system to achieve this.Citation26 Two studies employed a combined automated and manual segmentation.Citation24,Citation39 Using the Brainlab software, Carl et al. used manual segmentation for vascular risk structures, but automated segmentation to visualise nerve risk structures. Being the only studies to employ each, Pennacchietti et al. used complex pixel volume segmentation and Dixon et al.Citation30 used contour-based segmentation.
Tracking
Twelve of the 18 included studies chose optical tracking,Citation25–27,Citation29–31,Citation33,Citation34,Citation36–39 with six studies all utilizing the NDI Polaris Optical Tracking System (OTS).Citation25,Citation26,Citation30,Citation31,Citation34,Citation38 Being an exception to this, Caversaccio et al. used the Optotrak™ 3020, Zeiger et al. used Brainlab's Cranial Navigation software, and Lai et al. used their own OTS. Only two studies used electromagnetic tracking (EMT) as the sole method,Citation24,Citation28 with Citardi et al. choosing the Scopis Hybrid Navigation. Taking a comparative study approach, Linxweiler et al. utilised both the NAV1 SinusTracker software with an EMT unit and the NAV1 OTS. Similarly, Mirota et al. evaluated and compared both pointer-based navigation (Optotrak pointer and StealthStation Pointer) and tracker-system-based endoscopy against their own video-based tracking system.
Display
The majority of studies (n = 10) used a hybrid endoscopic-AR display, in which AR images are projected onto the endoscopic view.Citation23,Citation25,Citation27,Citation28,Citation33–37,Citation39 For this, Pennacchietti et al. used the neuronavigation workstation NovaPlan (version 2.6.10, Scopis, Germany), Zeiger et al. the Endoscope Surgical Navigation Advanced Platform (EndoSNAP), Mirota et al. the OptoTrak and the remaining studies employed their own technology. Two studies used a sub-monitor,Citation29,Citation31 with Dixon et al.Citation31 and Dixon et al.Citation29 projecting AR as mesh and CT images as tri-planar onto the sub-monitor. A 3D wire-frame model was projected on the endoscopic view by Kawamata et al. using a Pentium III-based personal computer. Dixon et al.Citation30 was the only study to use wall-down display. Overall, three studies used a microscope-AR hybrid display instead of endoscopic,Citation24–26 with Carl et al. being the only one to use a head-up display of the microscope additionally.
Intraoperative performance
Landmark identification
With regards to the number and type of structures identified using AR-overlay, ten studies discussed AR-assisted navigation and intraoperative identification of anatomical landmarks,Citation24,Citation28–34,Citation37–39 with the majority of studies reporting visualising the carotid arteries (n = 8),Citation28–30,Citation32,Citation37–39 optic nerves (n = 7),Citation24,Citation28–30,Citation32,Citation37,Citation38 and bony landmarks (n = 7).Citation28,Citation29,Citation32,Citation34,Citation37–39 Only three studies, all using optical tracking and an endoscopic-AR display, were able to detect and report on bony structures posterior to the cranial wall of the sphenoid sinus.Citation34,Citation37,Citation39 Nonetheless, six studies, including three microscopic studies, were able to localise and report on structures anterior to the cranial cavity.Citation28,Citation29,Citation32,Citation34,Citation38,Citation39 Kawamata et al. was the only study to report on localisation of the vidian nerve. Seven studies reported that AR-assisted navigation had positive effects on landmark identification ().Citation27,Citation28,Citation30,Citation32,Citation37–39 Four studies reported significant usefulness in the identification of neurovascular landmarks for increasing surgical safety, as well as detecting skull anatomical variations.Citation27,Citation28,Citation38,Citation39 Although Prisman et al. noted minimal benefit of AR in ventral landmark identification, it reported, alongside Zeiger et al. and Bong et al., an increase in spatial anatomical understanding with the use of AR.
Figure 3. The effects of AR navigation technology on transsphenoidal surgery in terms of (a) landmark identification, (b) intraoperative navigation, (c) accuracy, (d) time, (e) surgeon experience, and (f) patient outcomes are visualized as harvest plot. The effects are stratified into three columns: negative effect, no effect, and positive effect. A rectangle represents an individual study, unless at the base of the rectangle a number is specified to represent multiple studies i.e. 2x (= two studies). The colours of the rectangles correspond to the study setting: black (in vivo), grey (ex vivo), white (in vitro). The number on top of the rectangle specifies the risk of bias in outcome measurement (in line with the risk of bias analysis in , with 4 implying very robust outcome measurement or no risk of bias, 3: moderate risk, 2: high risk, and 1: critical risk, according to the risk of bias analysis results. The height of the rectangle directly correlates to the risk of bias in outcome measurement and the aforementioned number on top of a rectangle. The symbol

Intraoperative overlays & navigation
Five studies reported the overlay of the pituitary gland and/or the associated dura in the landmark identification process.Citation29,Citation30,Citation32,Citation38,Citation39 Three of these studies additionally reported being able to overlay respective target tumours intraoperatively.Citation24,Citation32,Citation39 Notably, Zeiger et al. was the only study to furthermore overlay pituitary tumours, meningiomas and Rathke’s cleft cyst. Interestingly, in the realm of microscope-AR, Carl et al. reported that overlay of landmarks increased intraoperative orientation markedly ().Citation24 Pennacchietti et al. and Kawamata et al. reported that AR overlays improved target lesion visualisation and more accurate estimations of the distance between endoscope and lesion.Citation33,Citation37
Accuracy
15 studies reported on a variety of accuracy parameters,Citation23–28,Citation30–38 assessing the precision of structure and target AR-overlay. Pennacchietti et al. and Dixon et al.Citation30 reported that accurate localisation of anatomical landmarks was objectively and subjectively improved using AR-assisted endoscopy.Citation30,Citation37 Using purely quantitative data, three studies reported that target registration error was markedly reduced using AR (, ).Citation27,Citation30,Citation32 Of these, Bong et al. reported a significant decrease of error events decreased from 4.86 ± 1.22 to 1.71 ± 1.38 (p = 0.0073). Similarly, 11 studies reported on target registration errors (TRE),Citation23–26,Citation28,Citation29,Citation31–36,Citation38 with mean TREs ranging from 0.55 mmCitation33 to 2.36 mm.Citation23 Using manual error registration, Caversaccio et al. recorded a mean overlay error (MOE) of 0.7mm. Employing fiducial registration instead, Carl et al. yielded a higher TRE of 2.33 ± 1.30 mm. However, Linxweiler et al. and Li et al. reported no significant difference in accuracy between AR-navigation and conventional 2D (2 dimensional) navigation. Notably, Carl et al. reported that iCT-based registration significantly (p < 0.001) increased AR accuracy to TRE = 0.83 ± 0.44 mm.
Figure 4. A pooled effect size analysis forest plot on the use of AR navigation in transsphenoidal surgery compared to non-AR methods is shown (n = 3 studies).Citation23,Citation24,Citation44 Eligibility criterion was having comparative study designs and presenting the appropriate statistical data to calculate an effect size. The size of the grey square of the SMD visual correlates to the study sample size and the straight line indicated the confidence interval. The diamond at the bottom indicates the overall pooled effect. The red bar below it indicates the prediction interval. Heterogeneity is indicated by the chi-squared statistic (I2) with an associated p-value. The 95% confidence intervals (CI) are shown in squared bracket ([ ]). There was no significant treatment effect size noted for AR navigation in transsphenoidal surgery noted.
![Figure 4. A pooled effect size analysis forest plot on the use of AR navigation in transsphenoidal surgery compared to non-AR methods is shown (n = 3 studies).Citation23,Citation24,Citation44 Eligibility criterion was having comparative study designs and presenting the appropriate statistical data to calculate an effect size. The size of the grey square of the SMD visual correlates to the study sample size and the straight line indicated the confidence interval. The diamond at the bottom indicates the overall pooled effect. The red bar below it indicates the prediction interval. Heterogeneity is indicated by the chi-squared statistic (I2) with an associated p-value. The 95% confidence intervals (CI) are shown in squared bracket ([ ]). There was no significant treatment effect size noted for AR navigation in transsphenoidal surgery noted.](/cms/asset/d05d6ef8-6043-4609-8274-48227464f6bf/ibjn_a_2057435_f0004_c.jpg)
Time
Seven studies reported on operative time,Citation25,Citation27,Citation29,Citation34,Citation35,Citation37,Citation39 with six studies discussing the effects of AR-assisted navigations tool on total operative time ().Citation25,Citation27,Citation34,Citation35,Citation37,Citation39 Two studies reported a significant reduction in time when using AR navigation systems;Citation25,Citation34 Caversaccio et al. detected an average reduction in the operating time of 10–25 minutes in in vivo endoscopic endonasal and microscopic skull base surgery (n = 406) whilst Li et al. reported a significant reduction in cadaveric surgical simulation time (AR: 88.27 ± 20.45 vs Conventional: 104.93 ± 24.61, p < 0.05). However, two studies, Bong et al. and Dixon et al.Citation29 described the contrary. Bong et al. noted that the time to task completion [localisation of eleven points sequentially in a simulated endonasal operation] was increased when using AR navigation to conventional methods (18.26 ± 7.82s vs 13.75 ± 6.30s) and Dixon et al.Citation29 noted that most subjects did not feel a reduction in the cadaver-based surgical simulation time. However, Dixon et al.Citation29 nonetheless described that participants subjectively felt that the in vivo operation time is likely to be reduced with the use of AR, outside of a cadaveric setting. Interestingly, one study, Linxweiler et al. reported no significant time difference between the conventional and AR navigation systems, although a significantly longer time period with preoperative image analysis and image-based planning of the surgical procedure reported in the AR-navigational system. Moreover, Zeiger et al. reported that the operative planning time when using AR ranged from ten minutes to one hour depending on the image quality and surgical pathology.
Clinical outcomes
Surgeon experience
Twelve studies found that surgeons positively experienced and perceived using AR-navigation tools in transsphenoidal surgery overall ().Citation24,Citation27–32,Citation34,Citation35,Citation37–39 In addition, a better-perceived performance and increased confidence in decision making were reported by three studies,Citation27,Citation29,Citation30 particularly in difficult and complex cases involving critical structures, which lead to the surgeons subjectively performing a more ‘complete’ surgery. Interestingly, Dixon et al.Citation31 reported the preference and effectiveness of auditory over visual signals as proximity warnings to inform the surgeon whilst keeping distraction to a minimum. Six studies unanimously reported that AR-navigation provides a statistically significant reduction in mental demand,Citation27,Citation29–31,Citation34,Citation39 five of which report a further reduction in surgeon effort (p < 0.02), frustration (p < 0.02), and other relevant NASA task load index (NASA-TLX) subscales when compared with conventional navigation.Citation29–32,Citation39
Patient outcomes
Four studies reported on post-operative complications,Citation25,Citation35,Citation37,Citation39 with two studies reporting on medium to long-term patient outcomes when using AR-navigation in transsphenoidal surgery.Citation37,Citation39 However, only Linxweiler et al. compared the effect of an AR navigation tool on patient outcomes with those of conventional transsphenoidal surgery ().Citation35 Pennacchietti et al. reported the complete remission of eight patients post-operatively, four stable diseases, and no postoperative neurological deficits after AR-navigation endoscopic-assisted endonasal skull-base operations; however, four patients required revision surgery, two of which were due to cerebrospinal fluid (CSF) leaks.Citation37 Linxweiler et al. reported only minor operative complications (eight minor bleeds and five local wound infections) and no surgical revisions post-operation after AR-assisted endoscopic sinus surgery, with no significant difference between the AR and the non-AR group being reported in terms of the postoperative condition.Citation35 Employing both AR-assisted microscopy and endoscopy for tumour resections and chronic sinus surgery resections, respectively, Caversaccio et al. reported 26 small haematomas due to the use of transcutaneous needles for registration purposes.Citation25
After qualitative analysis, we found strong methodological differences between studies as they were using completely different AR technologies, different study settings and surgical fields, and having different outcome measurements. This heterogeneity was further proven statistically with Higgin’s I2 scoring 82% (). Due to this, as well as insufficient comparative data is available to calculate sufficient standard effect size (SES), in line with Cochrane Collaboration Guidelines,Citation23 a synthesis without meta-analysis (SWiM) was conducted instead of a meta-analysis. 13 studies were included in the SWiM,Citation24,Citation25,Citation27–32,Citation35,Citation37–39 with the inclusion criteria being that they included a clear evaluative statement regarding the advantage or disadvantage of AR-guided TS compared to non-AR-guided TS. Graphical evaluation of quantitative SWiM as harvest plots using voting on directional effect can be seen in .
Discussion
Minimally invasive techniques of ETS and MTS are regarded as superior to conventional OTCS approaches in attaining more favourable post-operative outcomes and ultimately reducing collateral tissue damage through the inherently shorter operative pathways and smaller surgical sites. ETS, in particular, was shown to have significantly higher rates of post-operative visual improvement than OTCS and MTS, although both minimally invasive techniques perform less well when compared to OTCS in treating tumours with high recurrence, namely larger or locally invasive pituitary macroadenomas, and are more prone to CSF leakage.Citation10–12,Citation39,Citation40 Within this continuous drive to reduce tissue disruption and functional trauma with ETS and MTS, the technical issues of intraoperative instrument localisation, anatomical evaluation and understanding, and management of intraoperative complications are largely persistent in minimally invasive neurosurgery, if not exacerbated. In fact, ETS and MTS face significant difficulty in overcoming the anatomical challenges posed by repeat transsphenoidal surgeries, such as adhesions, fibrosis, and severe distortions of surgical landmarks due to the inherently complex and challenging nature of such procedures. Furthermore, ETS and MTS somewhat limited in their line of sight, making complete tumour margin visualisation even more challenging. These current limitations of conventional ETS and MTS, therefore, warrant the assessment and exploration of technologies that can assist in the surgical guidance and structural identification, given the promising nature of minimally invasive skull base surgery in improving patient outcomes. This review, therefore, evaluated the use of AR in TS as an aid to overcoming challenging anatomy identification and intraoperative guidance.
The SWiM analysis found that AR guidance improved landmark identification and intraoperative navigation when employing AR-ETS compared to conventional ETS (), although these small number of studies were mostly non-comparative with relatively small-medium sample sizes.
This is in line with existing literature, with studies on the positive effect of AR guidance on surgical orientation having been reported in several different surgical fields.Citation34 In particular, this review found that AR guidance enables the surgeon to recognise anatomical variations and vascular risk structures more accurately. This is a salient finding in terms of patient safety. Existing literature shows that conventional ETS, although less so than conventional MTS, is associated with significant risks, namely CSF leaks due to the breach of the arachnoid membrane, as well as endocrine disturbances, particularly diabetes insipidus.Citation41 Although the mortality of even OTCS overall does not exceed 1%, the morbidity of the complications may have significant impacts on the quality of life of the patients and hence AR guidance could be the decisive factor to further reduce complication risk associated with conventional methods.Citation42 Moreover, Pennacchietti et al., Linxweiler et al. and Caversaccio et al. all employed AR-guided TS to operate on revision surgeries (n = 46) and reported successful outcomes with low complication rates, indicating that patient safety is also not compromised but perhaps rather ensured using AR guidance, even in situations with complex anatomical obstacles.Citation25,Citation35,Citation37 To solidify this finding, future studies must aim to compare conventional TS to AR-guided TS purely in revision surgery cases.
Furthermore, conventional ETS shares an important technical limitation with OTCS and MTS in its lack of adequate intraoperative navigation in complex cases, further increasing the reliance on sound anatomical knowledge and operative experience but adding to the mental workload and reduced confidence in decision making. This review found that AR guidance enhances surgeon confidence and performance, especially when completing complex and difficult surgical tasks, potentially due to reduced mental demand and enhanced sight.Citation34 Given this, after accounting for adequate surgical skill and anatomical skill, it is highly probable that surgeons perform safer surgery when given AR guidance as an adjunct.Citation43 Without AR guidance, surgeons have to manually transform two-dimensional radiological images into a three-dimensional anatomical mental visual, as well as often look at separate displays, whilst simultaneously handling instruments in the surgical field.Citation44 This form of multi-tasking has been shown to lead to errors by multiple studies.Citation45,Citation46 With AR guidance surgeons are enabled to focus on risk structures and operative obstacles without reduced mental distraction, leading to safer surgeries for patients, even in difficult operative scenarios. However, studies exploring surgeons’ experience qualitatively, particularly Dixon et al.,Citation31 pointed out that specific modalities of AR guidance introduce distractions during operations, especially visual warning systems. Although this is a critical point of weakness of AR guidance, it may be easily mitigated by replacing visual cues with auditory or tactile ones. That said, interestingly the role of the visual distraction of AR displays has been discussed previously. Marcus et al. explored this issue in a preclinical randomised study with 50 novice surgeons trying to identify a basilar tip aneurysm in a validated head model with varying degrees of AR guidance.Citation47 It was reported that whilst an always-on AR solid overlay was associated with the greatest inattentional blindness, all image guidance displays, regardless of the degree of AR guidance and overlay, resulting in a considerable and statistically significant inattentional blindness. Possibly, this may have arisen due to attentional tunneling, increased visual clutter, and an additional camouflage effect because of the AR assistance. However, simultaneously, it is important to emphasise that a salient weakness of conventional ETS is the need for the surgeon to switch between his visual field and the screen display or move the display to allow for adequate line-of-sight alignment. This manual and time-consuming process is a most likely at least equally significant source of operative error, and AR guidance using overlay displays minimises this to a minimum. Marcus et al., therefore, suggested that greater importance should be attributed to the cognitive load of each display modality as opposed to the AR guidance itself.Citation47 It was suggested that the employment of an on-demand overlay, as opposed to always-on-overlay, may best maximise intra-operative accuracy. This may also be particularly useful in emergencies, where turning overlays off may be beneficial to reduce visual distractions.
In terms of operative accuracy, this review found that AR-ETS and AR-MTS are at least equal and in some cases superior (). In line with existing literature, the qualitative analysis showed that AR guidance overall has marginal to moderate positive effect on operative accuracy ().Citation48 AR-ETS was reported to yield an accuracy of 0.55 mmCitation33–2.36 mmCitation23 which is deemed widely to be at least sufficient for clinical application. However, it must be noted that many of the included studies were cadaveric or in vitro studies, and hence assessed accuracy in an artificial setting which is unlikely to completely match the operative environment in a clinical setting, and therefore might have positively overestimated the accuracy of AR-ETS. None of these studies assessed the accuracy of AR-ETS in a dynamic setting, even though certain risk structures of transsphenoidal surgery, particularly the vasculature of the brain, are dynamic organs. Given this, the exact quantitative performance of AR-ETS in terms of clinical accuracy remains elusive. Ideally, the aim of future non-in vivo studies must be to stimulate the dynamic interfaces of the brain to give an accurate real-to-life estimate of accuracy. That said, existing literature indicates that AR is very capable to account for motion by using dynamic registration, hence this error may not hold much weight in the accuracy of AR-ETS, however, this certainly must be investigated further.Citation49
Similarly, the results of this review are not conclusive regarding the exact extent and significance of time reduction, as well as patient outcomes when using AR guidance. Whilst it is reported that the surgical preparation time increased with the use of AR guidance, the SWiM analysis yielded a mixed evaluation of effects on time when using AR-guided surgery. An improvement in time, namely a reduction in overall operative time, may potentially result from the increased confidence of the surgeons, knowing that they are a safe distance away from critical structures; naturally, operative progress slows situations of uncertainty and real-time AR guidance overlaying critical structures with adequate information about depth and distance minimises the sense of uncertainty. However, two studies reported that the use of AR guidance did lead to an increase in overall treatment time. This most likely is due to lack of familiarity of the surgical team with the technology, prolonging particularly the pre-operative phase.Citation27,Citation29 However, further studies are needed to confidently assess the effect of AR on pre- and intra-operative time. Patient outcomes when using AR-guided TS were only evaluated and compared to conventional methods by a single study, finding no effects, neither positive nor negative. This study, by Linxweiler et al., was a relatively large scale (n = 100) randomised control trial, suggesting that their findings can be regarded as valid.Citation35 On the one hand, this is a relatively positive sign in terms of patient safety and indicates that the improved landmark identification, accuracy, and surgeon experience at worst does no harm to the patient, and at best retains the strength of conventional methods but adds positive benefits, particularly for the surgeon. Ultimately, no long-term follow-up studies have been performed as of now, and these will be needed to make valid statements on the effect of AR guidance on patient outcomes after TS.
Finally, it is pivotal for neurosurgeons to know which specific technological modalities and options are most favourable to achieve optimal surgical performance. Interestingly, most studies that reported positive landmark identification outcomes utilising AR guidance used an OTS and a hybrid endoscopic-AR display (),Citation27,Citation28,Citation37–39 which might suggest that those are favourable over other tracking and display modalities. However, the only study that performed a direct comparison of different tracking and display modalities, namely Linxweiler et al., reported that EMT-guided operations were evaluated more positively by surgeons than OTS-guided operations.Citation35 That said, given that only the EMT group was operated on with AR guidance and OTS control group with conventional methods in this study, the comparison is biased and hence cannot be deemed valid. Nonetheless, existing literature overall does indeed state that EMT is slightly superior to OTS in terms of accuracy, stating the most likely reasons for this to be greater proximity to critical structures, lack of need for patient immobilisation, and no line-of-sight obstruction.Citation50 However, in specific situations, namely those where bend forces are involved, due to the inherent flexibility of EMT instruments, they may be more error-prone than OTS.Citation50 Whether this plays a role in OTS being most used is subject to further research. Overall, more studies that compare the two different tracking systems in the setting of transsphenoidal surgery are needed to derive clear conclusions regarding which modality is superior. To continue with the other technological variables, namely displays, this review found that most studies used hybrid endoscopic-AR, however sub-monitors, wall-down displays and HUD, though less frequently used, were also associated with positive improvements in accuracy.Citation24,Citation29–31 Hence, more research is needed comparing different display modalities, to state which one should be given preference. Similarly, no clear pattern of connection between specific imaging, registration and segmentation techniques and relatively superior outcomes could be established by this review, hence future studies must explore this aspect further.
In current wide-spread surgical practice, alternative non-AR-based technological adjuncts are used to aid in intraoperative guidance and structural identification, such as neuronavigation systems and, to a lesser extent, microvascular Doppler ultrasonography, Particularly in relation to iatrogenicinternal carotid artery injury, a very worrying complication of TS, the literature suggests that intra-operative Doppler usage prior to a dural opening happen before all TS, aiding in the timely detection of the internal carotid artery.Citation51,Citation52 It would be appropriate that future research directly compare such widespread intraoperative guidance adjuncts with AR guidance, particularly with regards to the effects on operative time, landmark identification and target accuracy, and the potential disruption to the operators’ workflow. Ideally, such research could lay the foundations for a useful cost-effectiveness analysis on this subject. Finally, whilst AR guidance and identification is a promising technological frontier in enhancing transsphenoidal surgery, artificial intelligence has been reported to be beneficial for pituitary surgery diagnosis and pre-operative management, particularly by means of magnetic resonance imaging-based radiomics and machine learning.Citation53 Albeit still in a pre-clinical developmental stage, robotic surgery may also further enhance outcomes in transsphenoidal surgery. Particularly of interest, is the “Concentric Tube Robot”, a surgical robot specifically designed for operations in the anatomically restrictive anterior skull base.Citation54,Citation55 The multitude of possible combinations of AR guidance with these other technological breakthroughs may yield enhancements, capabilities, and outcomes that could transcend our current understanding of the entire field of TS, but this will most certainly require significant research, further pioneering innovations in these technological fields, and a great deal of willpower and resources to during each step of the way.
This review revealed significant limitations of the existing literature on the use of augmented reality in transsphenoidal surgery. Firstly, none of the non-randomised studies (n = 14) objectively compared either non-AR vs AR or different AR technologies with each other in a statistically non-biased manner, namely by ensuring that both control and non-control groups were, apart from the examined variable (i.e. OTS vs EMTs), all else equal (“ceteris paribus”). Hence, it is difficult to make unbiased comparisons and derive valid conclusions. Additionally, many studies were found to be non-in vivo, which limits the generalisability of findings to a real-life setting. Hence, in the future, all studies must seek to follow set protocol reducing skewing independent variables to a minimum, as well as ideally being randomised and in vivo. Furthermore, it will be vital for healthcare providers to know how cost-effective AR-guided TS is compared to conventional TS, however, no study exists on this topic to date and future research must explore this using appropriate benchmarks such as the NICE cost-effectiveness threshold.Citation56
Limitations
The key limitations of this study are particularly due to the inherent limitations of the literature itself. Firstly, given the aforementioned lack of unbiased methodology, as well as the non-in vivo study design of the numerous included studies, implies that our deductions and conclusions may have been negatively impacted. This was tried to account for in the SWiM analysis by incorporating the risk of bias grading and the study design (in vivo vs non-in vivo) in the weighting of each study. Furthermore, given the lack of unbiased and similar study designs, as well as robust statistical data on treatment efficiency of AR-guided TS, this study was unable to conduct a meta-analysis. Including a meta-analysis, instead of a purely qualitative synthesis, would have enhanced the validity of our findings. In the future, more studies must compare AR-guided TS to non-AR-guided TS, and provide results of their statistical analyses, particular treatment effect size, to allow for a meta-analysis to be performed and for publication bias to be assessed.
Conclusion
AR-guided TS, including AR-ETS and AR-MTS, are, relative to their non-AR counterparts, found to be associated with improved reported outcomes in landmark identification, intraoperative navigation and surgeon experience. Accuracy was found to be on par to superior to conventional approaches when using AR overlay, however, time consumption is potentially increased by the use of AR. Post-operative complications were found to be low and reduced by AR guidance. Findings on the choice of technological modalities show that OTS and overlay displays are most commonly used, however, it remains elusive as to which modalities should be given preference. In the future, more studies with similar methodologies and quantitative outcomes are needed in order to perform meta-analyses and draw more valid conclusions.
Ethical approval
Ethical approval was not required as this a review article
Disclosure statement
The authors declare that they have no financial or other interest relating to products, distributors, or manufacturers associated with the following review study. All authors declare no conflict of interest in the pursuit of this study. All authors participated in the coordination and drafting of this text. All authors read and approved the final manuscript.
References
- Kelly PJ, Alker GJ, Goerss S. Computer-assisted stereotactic microsurgery for the treatment of intracranial neoplasms. Neurosurgery 1982;10:324–31.
- Roberts DW, Strohbehn JW, Hatch JF, Murray W, Kettenberger H. A frameless stereotaxic integration of computerized tomographic imaging and the operating microscope. J Neurosurg 1986;65:545–9.
- Mascitelli JR, Schlachter L, Chartrain AG, et al. Navigation-linked heads-up display in intracranial surgery: early experience. Oper Neurosurg 2018;15:184–93.
- Inoue D, Cho B, Mori M, et al. Preliminary study on the clinical application of augmented reality neuronavigation. J Neurol Surg A Cent Eur Neurosurg 2013;74:71–6.
- Kockro RA, Tsai YT, Ng I, et al. Dex-ray: augmented reality neurosurgical navigation with a handheld video probe. Neurosurgery 2009;65:795–807. discussion 807–8.
- Coelho G, Rabelo NN, Vieira E, et al. Augmented reality and physical hybrid model simulation for preoperative planning of metopic craniosynostosis surgery. Neurosurg Focus 2020;148:E19.
- Darbar A, Mustansir F, Hani U, Sajid MI. A review of common endoscopic intracranial approaches. Asian J Neurosurg 2020;15:471–8.
- Møller MW, Andersen MS, Glintborg D, et al. Endoscopic vs. microscopic transsphenoidal pituitary surgery: a single centre study. Sci Rep 2020;10:21942.
- Batista RL, Trarbach EB, Marques MD, et al. Nonfunctioning pituitary adenoma recurrence and its relationship with sex, size, and hormonal immunohistochemical profile. World Neurosurgery 2018;120:e241–e246.
- Pasquini E, Zoli M, Frank G. Endoscopic endonasal surgery: new perspectives in recurrent and residual pituitary adenomas. World Neurosurg 2012;77:457–8.
- Cavallo LM, Solari D, Tasiou A, et al. Endoscopic endonasal transsphenoidal removal of recurrent and regrowing pituitary adenomas: experience on a 59-patient series. World Neurosurg 2013;80:342–50.
- Cho J, Rahimpour S, Cutler A, Goodwin CR, Lad SP, Codd P. Enhancing reality: a systematic review of augmented reality in neuronavigation and education. World Neurosurg 2020;1139:186–95.
- López WO, Navarro PA, Crispin S. Intraoperative clinical application of augmented reality in neurosurgery: a systematic review. Clin Neurol Neurosurg 2019;1177:6–11.
- Meola A, Cutolo F, Carbone M, Cagnazzo F, Ferrari M, Ferrari V. Augmented reality in neurosurgery: a systematic review. Neurosurg Rev 2017;40:537–48.
- Cochrane. Handbook for systematic reviews of interventions. 2021 Training.cochrane.org. https://training.cochrane.org/handbook.
- Moher D, Liberati A, Tetzlaff J, Altman DG. Preferred reporting items for systematic reviews and meta-analyses: the PRISMA statement. Int J Surg 2010;8:336–41.
- Covidence (2020) Covidence – Better systematic review management. Available from: https://www.covidence.org.
- Sterne JAC, Savović J, Page MJ, et al. RoB 2: a revised tool for assessing risk of bias in randomised trials. BMJ 2019;366:l4898.
- Sterne JAC, Hernán MA, Reeves BC, et al. ROBINS-I: a tool for assessing risk of bias in non-randomised studies of interventions. BMJ 2016;355:i4919.
- Campbell M, McKenzie JE, Sowden A, et al. Synthesis without meta-analysis (SWiM) in systematic reviews: reporting guideline. BMJ 2020;368:l6890.
- Core RT, R: A language and environment for statistical computing. R Foundation for Statistical Computing 2013;1–523.
- McKenzie JE, Brennan SE. Synthesizing and presenting findings using other methods. Cochrane Handbook for Systematic Reviews of Interventions 2019;321–47.
- Mirota DJ, Wang H, Taylor RH, Ishii M, Gallia GL, Hager GD. A system for video-based navigation for endoscopic endonasal skull base surgery. IEEE Trans Med Imaging 2012;31:963–76.
- Carl B, Bopp M, Voellger B, Saß B, Nimsky C. Augmented reality in transsphenoidal surgery. World Neurosurg 2019;125:e873–e883.
- Caversaccio M, Langlotz F, Nolte L-P, Häusler R. Impact of a self-developed planning and self-constructed navigation system on skull base surgery: 10 years experience. Acta Otolaryngol 2007;127:403–7.
- Lapeer R, Chen MS, Gonzalez G, Linney A, Alusi G. Image-enhanced surgical navigation for endoscopic sinus surgery: evaluating calibration, registration and tracking. Int J Med Robot 2008;4:32–45.
- Bong JH, Song H-J, Oh Y, Park N, Kim H, Park S. Endoscopic navigation system with extended field of view using augmented reality technology. Int J Med Robot 2018;14: e1886.
- Citardi MJ, Agbetoba A, Bigcas J-L, Luong A. Augmented reality for endoscopic sinus surgery with surgical navigation: a cadaver study. Int Forum Allergy Rhinol 2016;6:523–8.
- Dixon BJ, Chan H, Daly MJ, Vescan AD, Witterick IJ, Irish JC. The effect of augmented real-time image guidance on task workload during endoscopic sinus surgery. Int Forum Allergy Rhinol 2012;2:405–10.
- Dixon BJ, Daly MJ, Chan H, Vescan A, Witterick IJ, Irish JC. Augmented image guidance improves skull base navigation and reduces task workload in trainees: a preclinical trial. Laryngoscope 2011;121:2060–4.
- Dixon BJ, Daly MJ, Chan H, Vescan A, Witterick IJ, Irish JC. Augmented real-time navigation with critical structure proximity alerts for endoscopic skull base surgery. Laryngoscope 2014;124:853–9.
- Kawamata T, Iseki H, Shibasaki T, Hori T. Endoscopic augmented reality navigation system for endonasal transsphenoidal surgery to treat pituitary tumors: technical note. Neurosurgery 2002;50:1393–7.
- Lai M, Skyrman S, Shan C, Babic D, Homan R. Fusion of augmented reality imaging with the endoscopic view for endonasal skull base surgery; a novel application for surgical navigation based on intraoperative cone beam computed tomography and optical tracking. PLOS One 2020;15(2):e0229454.
- Li L, Yang J, Chu Y, et al. A novel augmented reality navigation system for endoscopic sinus and skull base surgery: a feasibility study. PLOS One 2016;11:e0146996.
- Linxweiler M, Pillong L, Kopanja D, et al. Augmented reality-enhanced navigation in endoscopic sinus surgery: a prospective, randomized, controlled clinical trial. Laryngoscope Investig Otolaryngol 2020;5:621–9.
- Onishi K, Fumiyama S, Miki Y, Nonaka M, Koeda M, Noborio H. Study on the development of augmented-reality navigation system for transsphenoidal surgery. human-computer interaction. human values and quality of life. Cham, Switzerland: Springer International Publishing, 2020; pp. 623–638.
- Pennacchietti V, Stoelzel K, Tietze A, et al. First experience with augmented reality neuronavigation in endoscopic assisted midline skull base pathologies in children. Childs Nerv Syst 2021;37:1525–34.
- Prisman E, Daly MJ, Chan H, Siewerdsen JH, Vescan A, Irish JC. Real-time tracking and virtual endoscopy in cone-beam CT-guided surgery of the sinuses and skull base in a cadaver model. Int Forum Allergy Rhinol 2011;1:70–7.
- Zeiger J, Costa A, Bederson J, Shrivastava RK, Iloreta AMC. Use of mixed reality visualization in endoscopic endonasal skull base surgery. Oper Neurosurg 2020;19:43–52.
- Cappabianca P, Solari D. The endoscopic endonasal approach for the treatment of recurrent or residual pituitary adenomas: widening what to see expands what to do? World Neurosurg 2012;77:455–6.
- Cappabianca P, Cavallo LM, Colao A, de Divitiis E. Surgical complications associated with the endoscopic endonasal transsphenoidal approach for pituitary adenomas. J Neurosurg 2002;97:293–8.
- Black PM, Zervas NT, Candia GL. Incidence and management of complications of transsphenoidal operation for pituitary adenomas. Neurosurgery 1987;20:920–4.
- Irugu DV, Stammberger HR. A note on the technical aspects and evaluation of the role of navigation system in endoscopic endonasal surgeries. Indian J Otolaryngol Head Neck Surg 2014;66:307–13.
- Guha D, Alotaibi NM, Nguyen N, Gupta S, McFaul C, Yang VXD. Augmented reality in neurosurgery: a review of current concepts and emerging applications. Can J Neurol Sci 2017;44:235–45.
- Raban MZ, Walter SR, Douglas HE, Strumpman D, Mackenzie J, Westbrook JI. Measuring the relationship between interruptions, multitasking and prescribing errors in an emergency department: a study protocol. BMJ Open 2015;5:e009076.
- Westbrook JI, Woods A, Rob MI, Dunsmuir WT, Day RO. Association of interruptions with an increased risk and severity of medication administration errors. Arch Intern Med 2010;170:683–90.
- Marcus HJ, Pratt P, Hughes-Hallett A, et al. Comparative effectiveness and safety of image guidance systems in neurosurgery: a preclinical randomized study. JNS 2015;123:307–13.
- Vávra P, Roman J, Zonča P, et al. Recent development of augmented reality in surgery: a review. J Healthc Eng 2017; 2017:4574172.
- Azuma RT, Bishop G. Improving static and dynamic registration in an optical see-through HMD. Proceedings of the 21st annual conference on Computer graphics and interactive techniques. New York: ACM; 1994.
- Koivukangas T, Katisko JP, Koivukangas JP. Technical accuracy of optical and the electromagnetic tracking systems. Spriner Plus 2013;2:1–7.
- Dusick JR, Esposito F, Malkasian D, Kelly DF. Avoidance of carotid artery injuries in transsphenoidal surgery with the doppler probe and micro-hook blades. Operative Neurosurgery 2007;1:ONS–322.
- Yamasaki T, Moritake K, Hatta J, Nagai H. Intraoperative monitoring with pulse doppler ultrasonography in transsphenoidal surgery: technique application. Neurosurgery 1996;38:95–8.
- Dai C, Sun B, Wang R, Kang J. The application of artificial intelligence and machine learning in pituitary adenomas. Front Oncol 2021;11:5433.
- Soldozy S, Young S, Yağmurlu K, et al. Transsphenoidal surgery using robotics to approach the Sella turcica: integrative use of artificial intelligence, realistic motion tracking and telesurgery. Clin Neurol Neurosurg 2020;197:106152.
- Gilbert H, Hendrick R, Remirez A, Webster IIR. A robot for transnasal surgery featuring needle-sized tentacle-like arms. Expert Rev Med Devices 2014;11:5–7.
- McCabe C, Claxton K, Culyer AJ. The NICE cost-effectiveness threshold: what it is and what that means. Pharmacoeconomics 2008;26:733–44.