Abstract
Soil microorganisms are one of the primary driving factors of biogeochemical cycles in floodplain ecosystems. Vegetation type has an impact on the activities of soil microorganisms. However, it is currently unclear whether the composition and structure of soil bacterial and fungal communities in floodplain ecosystems have a consistent response pattern to vegetation communities. In this research, Illumina Mi-Seq sequencing technology was employed to study the soil fungal and bacterial communities covered by three typical vegetation communities in the Yellow River floodplain ecosystem. Our results showed that the underground biomass, total carbon (TC) and nitrogen (TN) were significantly different between the three vegetation communities. In the surface soil of summer, the Shannon index of fungi community was the highest in Tamarix chinensis (T. chinensis) and the lowest in Phragmites australis (P.australis). In autumn, the Shannon and Simpson indices of bacterial communities in surface soil were the highest in the P.australis and the lowest in the Sonchus arvensis (S.arvensis), but there was no significant difference in the β-diversity of bacterial community among the three vegetation communities. In autumn, the Chao1 index of fungal community in subsoil was the highest in P.australis and the lowest in S.arvensis, while there was no significant difference in the α-diversity of bacterial community among the three vegetation communities. In addition, there were considerable disparities in the fungal and bacteria community structure in various vegetation soils. Redundancy analysis (RDA analysis) showed that TN, TC, AP, pH, NO3-, NH4+ and moisture content were key factors driving bacterial community structure, while TP, NH4+, pH and moisture content were essential factors driving fungal community composition. These outcomes help to improve our understanding of the ecological model of soil fungal and bacterial community in floodplain ecosystems and give assistance in improving the role of an ecological barrier and floodplain service possibilities.
Keywords:
1. Introduction
A Floodplain ecosystem is an irregular and dynamic intertidal zone connecting the water area and the river bank (Cheong and Okada Citation2001; Yu et al. Citation2023), which is rich in species resources and is an important biological gene pool (Stevens et al. Citation2007). It contains abundant inorganic matter, organic matter and microorganisms, and its ecological environment is rich and diverse, which is critical for ecological balance. Meanwhile, the ecological environment of the beach is directly related to the ecological security of the basin and the region. It has good agricultural resource conditions and ecological environment advantages and plays a key role in the preservation of biodiversity and protecting various types of the ecosystem of the beach (Li et al. Citation2019; Zhao et al. Citation2020; Hill et al. Citation2021; Fan et al. Citation2023).
Microorganisms are one of the most active organisms in the Earth’s biosphere (Ravin et al. Citation2015). The floodplain is an important part of the earth biosphere; because of its special environmental conditions, the distribution of microorganisms in the floodplain is rich and diverse. They are important sources of organic matter and play a significant role in geochemical nutrient cycling, litter decomposition, ecosystem pollution remediation and biodiversity conservation (Hill et al. Citation2021). Soil microorganisms can not only decompose organic matter to provide nutrients for other biological factors but also transfer nutrients and information in the food web of the floodplain ecosystem. Bacteria is the most active microbial community in the soil ecosystem, which is abundant, widely distributed, and easy to acclimatize (Ravin et al. Citation2015). They are very sensitive to changing environments and react faster than other organisms, often reflecting the state of soil quality. Based on the characteristics of the soil bacterial community, it reflects the soil condition, making it a breakthrough to improve the floodplain ecosystem, which is a worthy research direction. Fungi play a vital role and an irreplaceable role in the soil ecosystem. Meanwhile, some fungi can form symbiotic relationships with corresponding vegetation communities, which has a beneficial influence in regulating floodplain ecosystem (Van Ryckegem et al. Citation2006; Citation2007; Li et al. Citation2016). However, far fewer has been reported about fungal communities than about bacterial communities.
Vegetation and soil microbial function changes are the key factors in evaluating the stability of the floodplain ecosystem. Soil microorganisms can promote plant growth and enhance the stress resistance of plants, on the contrary, plants also have a great influence on soil microbial community structure. Therefore, microbiological community structure and diversity found in soil affect plant growth and metabolic activities. The species and quantity of vegetation communities may alter the physicochemical features of soil through their litter and secretions and provide nutrients for soil microorganisms to alter the diversity and abundance of soil microorganisms (Behr et al. Citation2023; Molnár et al. Citation2023). A recent study has shown that higher vegetation diversity can increase soil fertility and improve soil nutrient cycling and plant nutrient use efficiency (Furey and Tilman Citation2021). Furthermore, a previous study related to ecosystem restoration showed that vegetal diversity and functional traits would also directly have an impact on the diversity and structure of microbial colonies in the soil, thereby affecting subsurface ecological processes and various ecosystem functions related to nutrient cycling (Wang et al. Citation2020). However, there are few studies on the community features of soil microorganisms under many types of vegetation in the floodplain ecosystem, and the correlation between them is also lacking.
In the present investigation, Illumina MiSeq sequencing technology was utilized to study the soil fungal and bacterial communities of different vegetation communities in the Yellow River floodplain ecosystem and explore the impacts of vegetation cover from the aspects of seasonal changes and different soil profile depths. We hypothesized that different vegetation in the floodplain ecosystem could lead to alterations in the composition and diversity of soil microbial communities by influencing the change of soil physical and chemical characteristics, and such effects change with the seasons. The aim of this study was to assess the effects of different vegetation communities on soil physicochemical properties and microbial community structure, so as to better to understand the soil microbial community in the floodplain ecosystem and give a reference for the management of the floodplain ecosystem.
2. Material and methods
2.1. Site of study
The experiment was carried out at the Yellow River shoal System Field Scientific Observation and Research Station (34°59’65"N, 113°25’05"E), which is located in Xingyang City, Henan Province China, in the middle and lower reaches of the Yellow River. The soil is mostly sandy soil with high salinization degree, loose soil, and high permeability. The vegetation growing on the natural land has the characteristics of saline-alkali resistance, drought resistance and flood resistance. The vegetation communities of three typical dominant species in the floodplain ecosystem were selected, namely Phragmites australis (P.australis), Tamarix chinensis (T. chinensis) and Sonchus arvensis (S.arvensis). For each vegetation community, four replicates were established, with a total of 12 plots, each measuring 4 m × 4 m.
2.2. Study design and collection of samples
Soil specimens were gathered in the summer of June 2020 and the autumn of November 2020. First, clean the litter, rocks, and other debris above the soil. Then, use the soil drill to drill the surface soil and the subsurface soil, two depths of the soil layer. Two soil depths were drilled: 0-10 cm (surface) and 10-20 cm (subsurface) correspondingly, and 3 points were taken and blended from each plot. Select three vegetation communities and set 4 quadrats for each vegetation community. The surface soil and subsurface soil specimens were gathered in summer and autumn, respectively, and a total of 48 soil specimens were gathered. The soil specimens were wrapped in sterile plastic bags and delivered to the laboratory on ice. The soil materials were separated into three groups. The 1st part was held at −80 °C to analyze the soil microbial community composition. The 2nd part was kept at 4 °C for further examination of the nitrate (NO3--N) and ammonium (NH4+-N) contents. The remaining sample was air-dried at room temperature, ground into powder and allowed to pass through a 0.25-mm mesh sieve for further chemical analysis.
2.3. Physicochemical examination of soil
A pH meter (Sartorius PT-21, Shanghai, China) with a soil-to-ddH2O ratio of 1:2.5 was employed to determine the soil pH. The PH of each sample was calculated 3 times. Soil total nitrogen (TN), total carbon (TC) and its contents were evaluated by the Vario Max CNS elemental analyzer (Elementar Analysensysteme GmbH, Hanau, Germany). To find out the soil ammonium nitrogen (NH4+) and nitrate nitrogen (NO3-), we take 10 g fresh soil sample, add 100 mL 2 M KCl solution, shake it in a shaker for 1 h, filter it with filter paper, and then determine it with a flow analyzer (SmartChem 200, AMS, France). Total phosphorus (TP) was evaluated by means of an Inductively Coupled Plasma Emission Spectrometer (Agilent 5100 ICP-OES). Following NaHCO3 extraction of soil samples, the available phosphorus (AP) was determined by means of the Mo-Sb colorimetry method (0.5 M). The weight loss of 15 mg of fresh soil dried at 105◦C was utilized to determine the soil moisture content after 48 h.
2.4. Plant sample collection and functional traits determination
Six plant functional characteristics were assessed: aboveground biomass, the total nitrogen and carbon contents (N, C) of aboveground, underground biomass, N and C content of root. These measurements were taken within a 1 × 1 m quadrat. We classified all of the quadrat’s above-ground living tissues based on species. The plants were then dried at 70◦C for 24 h and measured as aboveground biomass. All species’ 0-10 cm roots from the quadrat were gathered, dried at 70◦C for 24 h, and weight as underground biomass. Following weighing and drying, aboveground biomass and the underground biomass are ground and filtered them via a 0.25 mm filter to ascertain the TC and TN by means of a Vario MACRO Cube elemental analyzer (Elementar, Hanau, Germany).
2.5. Illumina MiSeq sequencing
An Omega Soil DNA Kit (Omega Bio-tek, M5635-02, Norcross, GA, USA) was employed to extract total DNA from the soil as per guidelines provided by the supplier. Illumina MiSeq High-throughput sequencing: For the sequencing of fungal and bacterial communities, the variable region of V3-V4 in the 16S rRNA gene of soil bacteria and the spacer region ITS1 gene in the internal transcription of fungi were selected as the detection objects, respectively. The variable region V3-V4 of the 16S rRNA gene of soil bacteria and the internal transcriptional spacer ITS1 of fungi were sequenced using the Illumina platform. The sequencing primers are as follows:
The sequences of bacteria’s V3-V4 hypervariable parts were amplified by using the primers 338 F (ACTCCTACGGGAGGCAGCAG) and 806 R (GGACTACHVGGGTWTCTAAT). The primers ITS5F (GGAAGTAAAAGTCGTAACAAGG) and ITS1R (GCTGCGTTCTTCATCGATGC) were used to amplify the Internal Transcribed Spacer 1 (ITS1) of fungi (Yu et al. Citation2023).
The entire sequence was completed by means of the Illumina MiSeq-PE300 platform (Illumina, San Diego, CA, USA). Microbiome bioinformatics was carried out using QIIME 2 (Bolyen et al. Citation2019). The raw readings with paired ends were demultiplexed with the demux plugin before being cut with the cut adapt plugin (Martin Citation2011). The sequences were denoised and quality-filtered utilizing the DADA2 method in the parameters (Callahan et al. Citation2016). To monitor sequence quality and eradicate all sequencing mistakes, we utilized the derepFastq, dada learnErrors, and mergePairs techniques with default parameters. The amplicon sequence variations (ASVs) of the singletons were then removed. Following that, the taxonomic identities of bacteria and fungi were identified using the Silva v132 database (http://www.arb-silva.de) (Quast et al. Citation2013), the UNITE database (Release 8.0)2 (Kõljalg et al. Citation2013), correspondingly.
The sequencing raw data were submitted to the National Centre for Biotechnology Information’s Sequence Read Archive (SRA) database, with the BioProject numbers: PRJNA876613, and PRJNA877715 for the bacteria and fungi, respectively.
2.6. Statistical assessment
The difference analysis of soil physical and chemical characteristics between various vegetation communities was carried out using ANOVA in SPSS (IBM Statistical Product and Service Solutions). Normal distribution and variance homogeneity test were performed on the data before analysis. Relevant graphics by genescloud (https://www.genescloud.cn) were used for drawing.
The α-diversity metrics was determined by seven indices that estimate the community’s richness (Observed_species and Chao1), total diversity (Shannon and Simpson index), evenness (Pielou_e), phylogenetic diversity (Faith_PD) and Goods_coverage. The larger the values of Chao1 and Observed species indices, the more species are reflected in the community. Shannon and Simpson refer to community diversity. The higher the Pielou_e index value, the higher the community uniformity. The higher the Goods_coverage index value, the wider the sample coverage, the fewer species that were not detected, and the more realistic the sample sequencing results were reflected. The microbial community structure of β-diversity was investigated by non-metric multidimensional scaling analysis (NMDS), which simplified the multidimensional analysis to two dimensions. The gap between sample points within the same group represented the repeatability of the samples, and the distance between sample points reflected the difference between groups. Using genescloud (https://www.genescloud.cn) redundancy analysis (RDA), the microbial community structure under different processing and testing of the correlation between soil properties.
Unless otherwise mentioned, all of the above statistical analyses were carried out in the R environment (v3.5.1; http://www.r-project.org).
3. Results
3.1. Plant biomass, carbon and nitrogen content and soil physicochemical properties
Analysis of variance indicates that there was no statistically significant difference in aboveground biomass and TC among the three vegetation types in summer, but there was a significant difference in TN (). There was no statistically significant change in aboveground biomass and TN among the three vegetation types in autumn, but there was a significant difference in TC (). There were considerable differences in belowground biomass and TC among the three vegetation types in the surface soil of summer (). There were significant differences in the subsurface biomass, TN and TC among the three vegetation types of the subsoil in summer (). There were significant differences in belowground biomass, TN, and TC in autumn surface soil of the three vegetation types (). There was significant differences in TN content in the subsurface soil of the three vegetation types in autumn (). In summer, there were considerable differences in NO3- and TP levels among different vegetation types in the surface soil (). In summer, there were statistically significant difference in TC, TP, AP, and pH among various vegetation types in subsurface soil (). In autumn, there were significant differences in TP and NO3- levels among several vegetation types in the surface soil (). In subsurface soil of autumn, there are significant differences in TN, TC, and NO3- among different vegetation types (). In summary, there are significant differences in aboveground and belowground biomass, TC, and TN between the three vegetation types in summer and autumn, as well as differences in TC, TN, AP, pH, NO3- and NH4+ in surface soil and in subsurface soil.
Figure 1. Biomass of aboveground and belowground, carbon content and nitrogen content in summer and autumn. A and B: Aboveground biomass (A. biomass), total carbon (TC) and total nitrogen (TN) of aboveground in summer and in autumn. C and D: Belowground biomass (B. biomass), total carbon (TC) and total nitrogen (TN) of belowground in summer (C: surface soil, D: subsoil.); E and F: Belowground biomass (B. biomass), total carbon (TC) and total nitrogen (TN) of below ground in autumn (E: surface soil, F: subsoil.). *p < 0.05, **p < 0.01, ***p < 0.001. (P: P. australis. T: T. chinensis. S: S.arvensis. J:June (summer). N:November (autumn). 1: surface soil 0–10 cm, 2: subsoil 10–20 cm.).
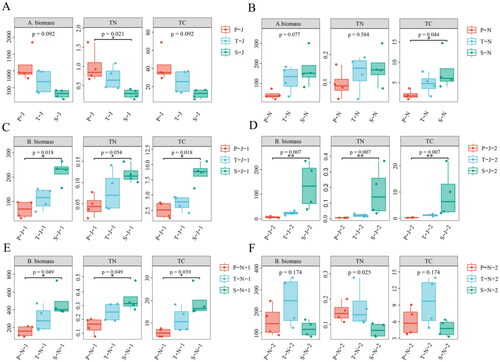
Figure 2. Soil physical, chemical in summer and autumn. A: Physical, chemical of surface soil in summer. B: Physical, chemical of subsurface soil in summer. C: Physical, chemical of surface soil in autumn. D: Physical, chemical of subsurface soil in autumn. (The total nitrogen (TN), total carbon (TC), and total phosphorus (TP); Available phosphorus (AP); Ammonium (NO3-); Nitrate (NH4+). P: P. australis. T: T. chinensis. S: S.arvensis. J:June (summer). 1: surface soil 0–10 cm, 2: subsoil 10–20 cm.). *P < 0.05, **P < 0.01, ***P < 0.001.
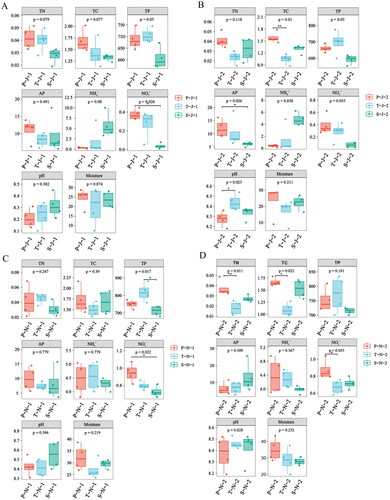
3.2. Characteristics of soil bacterial communities in different various types
Bacterial community structure in the surface soil during summer, the relative abundance of Pseudomonas in the P.australis community was the greatest, and that of Acinetobacter was the lowest. The relative presence of Subgroup_6 was the highest in the T. chinensis community, and SBR1031 was the lowest. Bacillus possessed the greatest relative abundance in the S.arvensis community and Pseudomonas was the lowest (). In summer, the α-diversity among the three vegetation types showed significant differences in the Pielou_e index between the surface soil bacterial communities of T. chinensis and S.arvensis community (Figure S1 A). In Summer, the Pielou_e indices of bacterial communities in the surface soil were the highest in the Tamarix and the lowest in the S.arvensis. NMDS analysis showed significant differences among the three vegetation types in summer (p = 0.005), and there were substantial disparities between P.australis and T. chinensis, P.australis and S.arvensis ( and Table S3). RDA study revealed that the 1st axis and the 2nd axis accounted for 59.02% of the variations in bacterial community structure in the surface soil of the three vegetation beds in summer, and NO3-, TN and TC were the main factors affecting the P.australis community (). AP was the key element influencing the bacterial community T. chinensis, while pH was the key component affecting the bacterial community S.arvensis.
Figure 3. The genus level of bacterial community abundance, the NMDS plots and the redundancy analysis (RDA) in summer. A: the genus level of bacterial community abundance of surface soil in summer. B: the NMDS plots of surface soil bacteria in summer. C: the redundancy analysis (RDA) of surface soil bacteria in summer. D: the genus level of bacterial community abundance of subsurface soil in summer. E: the NMDS plots of subsurface soil bacteria in summer. F: the redundancy analysis (RDA) of subsurface soil bacteria in summer.P: P. australis. T: T. chinensis. S: S.arvensis. J:June (summer). 1: surface soil 0–10 cm, 2: subsoil 10–20 cm.).
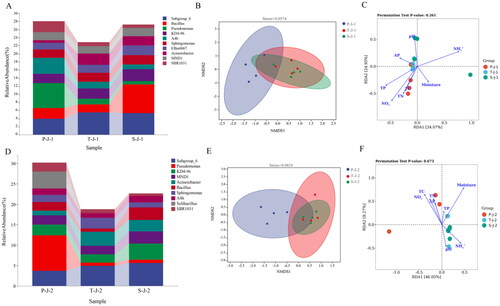
In subsoil during summer, the relative presence of Pseudomonas in the P.australis community was the highest, and that of Acinetobacter was the lowest. Subgroup_6 had the highest relative abundance in T. chinensis and S.arvensis types ( and Table S3). In summer, the α-diversity of bacteria in the subsoil of the three vegetation types showed no significant difference (Figure S1 B). In summer, NMDS analysis indicated that there was a significant difference (p = 0.002) among the three vegetation types in bacterial communities of the subsoil. There were significant differences between the soil bacteria groups of P.australis and T. chinensis (p = 0.029), and between the soil bacteria groups of P.australis and S.arvensis (p = 0.022) ( and Table S3). RDA study revealed that environmental variables contributed to 54.32% of the alterations in bacterial community structure in the surface soil of the summer three vegetation covers, and TN and AP were the key components impacting the bacterial community in the P.australis (). pH and moisture content primarily impacted the bacterial community of T. chinensis, while NH4+ mostly influenced the bacterial community composition of the soil of S.arvensis.
Bacterial community structure in the surface soil during autumn, Subgroup_6 possessed the greatest relative abundance in P.australis community while Aureibacillus was the lowest in the surface soil. The relative abundance of Subgroup_6 was greatest in T. chinensis community, and RB41 was the lowest. The relative abundance of Bacillus was highest in S.arvensis community and TRA3-20 was the lowest (). There were significant differences in Simpson, Shannon and Pielou_e indices among the soil bacteria groups of P.australis and T. chinensis (Figure S1 C). In autumn, the Simpson, Shannon and Pielou_e indices of bacterial communities in the surface soil were the highest in the P.australis and the lowest in the S.arvensis. NMDS analysis showed significant differences among the three vegetation types in autumn (p = 0.017). There were significant differences between soil bacteria groups of P.australis and S.arvensis (p = 0.028). There were no statistically significant differences in soil bacteria groups between T. chinensis and Phragmites australis, T. chinensis and S.arvensis communities ( and Table S3). RDA study revealed that environmental variables contributed to 51.37% of the alterations in the bacterial community structure of the surface soil of the autumn three vegetation types, TN and NH4+ were the main environmental factors impacting the bacterial community of P.australis (). TN, TP and NO3- were the main factors affecting soil bacterial community composition of S.arvensis.
Figure 4. The genus level of bacterial community abundance, the NMDS plots and the redundancy analysis (RDA) in autumn. A: the genus level of bacterial community abundance of surface soil in autumn. B: the NMDS plots of surface soil bacteria in autumn. C: the redundancy analysis (RDA) of surface soil bacteria in autumn. D: the genus level of bacterial community abundance of subsurface soil in autumn. E: the NMDS plots of subsurface soil bacteria in autumn. F: the redundancy analysis (RDA) of subsurface soil bacteria in autumn. (P: P. australis. T: T. chinensis. S: S.arvensis. J:June (summer). 1: surface soil 0–10 cm, 2: subsoil 10–20 cm.).
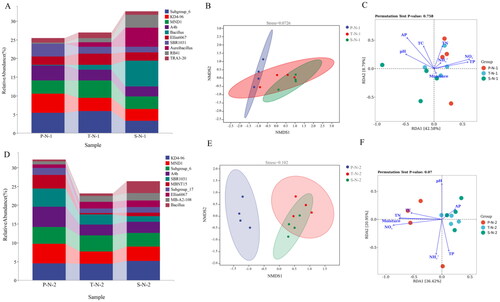
Composition of the bacterial community in subsoil during autumn, A4b had the greatest relative abundance in P.australis community and Bacillus had the lowest in subsoil. The relative prevalence of KD4-96 was the highest in T. chinensis community, and that of MBNT15 was the lowest. The relative presence of KD4-96 and MBNT15 was the highest in S.arvensis community (). There were no statistical differences in the α-diversity of bacteria in the subsoil of the three vegetation types in autumn (Figure S1 D). NMDS evaluation indicates that the overall difference in subsoil bacterial communities among the three vegetation types in autumn was significant (p = 0.002). There were significant changes observed in soil bacteria between P.australis and T. chinensis (p = 0.032). There was a significant statistical difference between the soil bacteria groups of P.australis and S.arvensis (p = 0.023). The differences observed in soil bacteria between T. chinensis and S.arvensis are statistically significant (p = 0.049). Therefore, there were significant differences between the subsoil bacterial community of the three vegetation types in autumn ( and Table S3). RDA study revealed that environmental variables contributed to 57.37% of the change in bacterial community structure in the subsoil of the three vegetation types cover in autumn, and NO3-, moisture content, TN and TC were the primary aspects of the environment influencing the bacterial community of P.australis (). AP mainly affected the soil bacterial community structure of T. chinensis and S.arvensis.
3.3. Characteristics of soil fungal communities in various vegetation types
Fungal community composition in the surface soil during summer, the relative abundance of Pyrenochaetopsis in P.australis community was the highest, and that of Acremonium was the lowest. The relative abundance of Pyrenochaetopsis was greatest in T. chinensis community and Aspergillus was the lowest. Fusarium has the greatest relative abundance in the T. chinensis community, whereas Sarocladium has the lowest (). The α-diversity of surface soil fungi in three vegetation types in summer revealed that the Shannon index of surface soil fungi communities in T. chinensis and P.australis communities was significantly different (Figure S2 A). In summer, the Shannon indices of fungi communities in the surface soil were the highest in the T. chinensis and the lowest in the P.australis. NMDS analysis indicated that there was no statistical difference among the three vegetation types in fungal communities of the surface soil.
Figure 5. The genus level of fungal community abundance, the NMDS plots and the redundancy analysis (RDA). A and B: the genus level of fungal community abundance of surface soil and subsurface soil in summer. C and F: the genus level of fungal community abundance of surface soil and subsurface soil in autumn. D and G: the NMDS plots of surface soil and subsurface soil fungus in autumn. E and H: the redundancy analysis (RDA) of fungus in surface soil and subsurface soil in autumn. (P: P. australis. T: T. chinensis. S: S.arvensis. J:June (summer). 1: surface soil 0-10 cm, 2: subsoil 10–20 cm.).
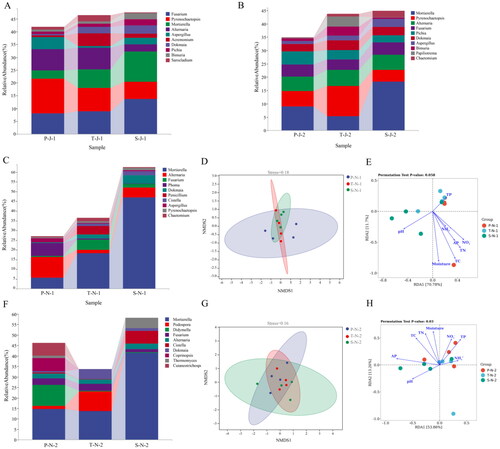
Fungal community composition in subsoil during summer, the relative abundance of Mortierella was greatest in P.australis community and Chaetomium was the lowest. The relative abundance of Pyrenochaetopsis was the greatest in T. chinensis community, and that of Chaetomium was the lowest. The relative abundance of Mortierella and Papiliotrema was the greatest in S.arvensis community, and Phoma was the lowest (). The variance analysis of α-diversity of subsoil fungal communities of the three vegetation types in summer showed no significant differences in Chao1, Goods-coverage, Simpson, Shannon, Pielou_e and observed species indices (Figure S2 B). NMDS analysis showed no significant difference in subsoil fungal community composition among the three vegetation types in summer.
Fungal community composition in the surface soil during autumn, the relative abundance of Alternaria was greatest in P.australis community and Penicillium was the lowest. Mortierella had the greatest relative abundance in T. chinensis community, and Aspergillus was the lowest. The relative abundance of Mortierella was greatest in S.arvensis community, and Phoma was the lowest (). The variance analysis of α-diversity of soil fungal community in the three vegetation types in autumn showed no significant statistical differences in the 6 indices (Figure S2 C). The NMDS study indicated that there were statistical differences among the three vegetation types in autumn (p = 0.006). The difference in soil fungi between P.australis and T. chinensis (p = 0.029) was significant. There was a statistical difference between the soil fungal community of P.australis and S.arvensis (p = 0.035) ( and Table S4). The RDA study revealed that environmental influences account for 82.48% of the alterations in the fungal community composition in the surface soil of the three vegetation types in autumn. Among them, TP, and TC mainly affect the fungal community composition of P.australis, TP mainly affects the fungal community of T. chinensis, and pH mainly affects the fungal community composition of S.arvensis ().
Fungal community composition in subsoil during autumn, Mortierella had the greatest relative abundance in P.australis community and Cistella was the lowest. The relative abundance of Mortierella was greatest in T. chinensis community. Mortierella’s relative abundance was the highest in S.arvensis community and Cutaneotrichosporon was the lowest (). The α-diversity of the subsoil fungal community of the three vegetation types in autumn showed that the subsoil fungal communities of P.australis and S.arvensis community in autumn had significant differences in Chao1, Goods_coverage and Observed_species indices (Figure S2 D). In autumn, the Chao1 and Observed_species indices of fungi communities in the surface soil were the highest in the P.australis and the lowest in the S.arvensis. But the Goods_coverage indices of fungi communities in the surface soil were the highest in the S.arvensis and the lowest in the Phragmites australis. NMDS analysis showed that the significant differences were found among the subsoil fungal communities of the three vegetation types in autumn (p = 0.01). The difference of soil fungi between P.australis and T. chinensis (p = 0.027) was significant. There was a statistical difference between the soil fungal community of P.australis and S.arvensis (p = 0.031) ( and Table S4). RDA analysis indicates that explains environmental influences 67.12% of the modifications in fungal community composition in the subsoil of three vegetation types in autumn. The P value obtained by the Monte Carlo replacement test was 0.01, indicating that the environmental factors tested had a significant effect on the fungal community. Among them, NO3-, NH4+ and TP mainly affected the community structure of P.australis soil fungi. Moisture content, NH4+ and TP mainly affected the soil fungal community composition of T. chinensis. AP and pH mainly affected the soil fungal community composition of S.arvensis ().
4. Discussion
The results of our investigation on aboveground biomass and belowground biomass of different vegetation types indicated that there were no significant variations in aboveground biomass of various vegetation types in summer and autumn (), but there were significant changes in subsurface biomass of surface soil and subsoil between Phragmites australis, T. chinensis and S.arvensis communities in summer and autumn (). As the depth of the soil increased, the contents of TN and TC in the belowground of the three vegetation types all changed (), and the proportion of microorganisms in different vegetation types was different in the corresponding period (). In the soil bacteria of the P.australis community, the relative abundance was greatest in the surface and subsoil layers, particularly Pseudomonas. Conversely, in the soil bacteria of the T. chinensis community, Subgroup_6 was the genus with the highest relative abundance in both the surface and subsoil layers of the soil. Among the soil bacteria of the T. chinensis community, Bacillus and Subgroup_6 had the highest relative abundance in the surface soil and subsoil layers, respectively (). The relative abundance of dominant bacteria in soil bacterial communities of different vegetation communities was different at genus level, so different vegetation communities had influence on soil bacterial composition. Shannon, Simpson and Pielou_e indices showed significant differences in α-diversity of surface soil bacterial community of P.australis community, T. chinensis community and S.arvensis in autumn, indicating that different vegetation affected diversity and evenness of surface bacterial community (Figures S 1). There were overall differences in bacterial β-diversity among the three vegetation communities, indicating that different vegetation communities had an effect on the structure of bacterial community. The alterations in bacterial composition might be because of a different litter of different vegetation, which leads to different belowground biomass, TN and TC contents (). Consistent with our findings, Previous studies have shown that vegetation types can have significant effects on the local soil ecosystem via various litter inputs (Walter et al. Citation2020). N and P addition do have a subtle, yet significant, effect on the root microbiota structure, an effect also observed in wheat. In that study, wheat plants were grown in natural soil with a long history of nutrient amendment and the microbiota differences were observed both for the roots but also for the bulk soil. The overall abundance of microbiota changes drastically in response to the nutritional status of the host plant (Tkacz et al. Citation2022). Fu found that vegetation change had a fundamental effect on soil microbial community structure and diversity, which might be directly connected to the soil’s physical and chemical characteristics (Fu et al. Citation2023). Therefore, the different content of belowground biomass, TN and TC may be one of the reasons for the different proportions of soil bacteria.
Microorganisms can boost plant development and improve plant stress resistance (Ding et al. Citation2021; Sarkar et al. Citation2022). Conversely, plants also have a significant impact on the structure, diversity and composition of microbial communities (Yang et al. Citation2022; Guo et al. Citation2023). Numerous investigations have revealed that there are many ecological factors affecting soil microbial communities, such as vegetation community type, soil moisture content, pH, carbon and nitrogen content and other physicochemical features (Wang et al. Citation2020; Zhang et al. Citation2021; Liang et al. Citation2022). Previous research demonstrated a link between microbial community organization and soil physical and chemical characteristics was analyzed based on the effects of alfalfa, oil sunflower, corn and ryegrass on soil physicochemical features, enzyme activities and the microbial community, diversity and composition. The results indicate that all vegetation types significantly reduced soil pH, total salinity and, bulk density, a rise of organic matter, TN, available nitrogen, TP, AP, and TK, and greatly decreased the number of nitrite bacteria (Li et al. Citation2022). Our results show a significant correlation between the bacterial α-diversity (Shannon, Simpson, and Pielou_e) index and the determined soil parameters (TP, AP, pH, NO3-, TN, TC, and moisture content) (, ). The effect of TN on the reed community in natural environment is relatively consistent, but other soil parameters (TP, AP, pH, NO3-, TN, TC, and moisture content) have different effects on the three different vegetation communities, which is consistent with other studies on different wetlands. Soil pH is believed to be a powerful driver that shapes the structure of soil bacterial communities in a variety of ecosystems, which may affect the soil microbial physiology, morphology, and metabolism. Soil water affects the availability of oxygen, soil respiration, nutrient diffusion in the soil and the breakdown of litter, which, in turn, has an impact on the structure of microbial communities in wetland ecosystems, especially those related to nitrogen and carbon cycling. Similar to our results, research on coastal wetlands in the Yellow River Delta found that there were changes in soil microbial abundance and diversity under various vegetation cover areas such as bare land, P.australis, galea and alkali canopy (Li et al. Citation2021). In conclusion, the changes of belowground biomass and carbon and nitrogen content in different vegetation types affected the physicochemical features of soil, thus it can affect the composition of soil bacteria to some extent.
Under similar environmental conditions at a certain spatial scale, different vegetation cover types initially determined the makeup of microbial communities. The characteristics of vegetation litter and its root exudates are different, the impacts on soil microorganisms are different, and the structure and composition of soil microbial communities vary (Lan et al. Citation2019; Ehlers et al. Citation2020; Tang et al. Citation2023). To further confirm the relationship between the physicochemical characteristics of soil and microorganisms, soil fungi in various vegetation types were detected. The results of our study showed that there were significant differences in the diversity of surface soil fungal communities of T. chinensis community and S.arvensis community in summer (Shannon indices), indicating that the composition of fungal communities was affected by different vegetation types. However, in autumn, the fungal communities of different vegetation communities in the surface soil tend to be consistent in terms of α-diversity, while in the subsoil, the richness of different vegetation fungal communities (Chao1 index) and sample coverage indices (Goods_coverage index) are significantly different. These results indicated that soil depth had greater influence on α-diversity of fungal community than different vegetation communities. Therefore, different vegetation communities and soil physicochemical properties have certain effects on fungal community diversity ( and S2 C-D). In addition, fungi can degrade complex molecules containing cellulose and lignin components in vegetation litter, but they need soil phosphorus for degradation, which may confirm the important impact of soil TP content on the structure of the fungal communities. In line with our study, a study pointed out that root exudation-mediated microbial interactions play a critical role in health status, soil fertility, and plant growth and development (Eisenhauer et al. Citation2012). A study showed that vegetation Jerusalem artichoke can not only promote soil microbial richness but also soil quality and nitrogen fixation (Shao et al. Citation2019). A study demonstrated that SMC and TOC were the most critical factors for modifying the structure of the bacterial community in soil, whereas SMC and TP were the major factors influencing soil fungus community structure change (Fu et al. Citation2023). In summary, soil physicochemical properties and plant biomass distribution characteristics of various vegetation types affect the composition and structural characteristics of soil fungi in different vegetation types.The different living characteristics of bacterial and fungal communities also lead to differences in their response patterns to different vegetation communities.
5. Conclusions
Our results provide evidence that vegetation type influences the composition and diversity of the soil’s microbial community and provide a personalized study of the important soil environmental parameters that explain these variations. The organization of soil microbial communities was mostly influenced by plant type but almost not affected by soil depth. In addition, we found that the abundance and diversity of bacteria and fungi have different response patterns to different vegetation communities. TN, TC, AP, pH, NO3-, NH4+, and moisture content are the primary elements that impact the makeup of bacterial community in various vegetation types. Unlike bacteria, TP, NH4+, pH and moisture content are the primary determinants of fungal community. In conclusion, different soil physical and chemical properties and plant characteristics (Plant biomass, carbon and nitrogen content) of different typical vegetation communities in the floodplain ecosystem of the Yellow River should be an important reason for the change of soil microbial community composition and structural characteristics. In addition, bacterial communities and fungal communities have different response patterns to different vegetation types.
Authors’ contributions
Author contributions Yanyan Yu conceived the presented idea and received important feedback from Jinyong Gao, Qianyun Yu, Anwei Su and Mingli Zang. Binghai Lei and Yaru Nie carried out the field management. Anwei Su, Mingli Zang, Shengyao Cai, Wenwen Zhang and Tingting Fang carried out the sampling collections, DNA preparation, and chemical measurement. Binghai Lei and Yaru Nie carried out the MiSeq sequencing process and data analysis. Jinyong Gao, wrote the manuscript with help from Yanyan Yu and Qianyun Yu.
Supplemental Material
Download MS Word (887.3 KB)Acknowledgments
We thank Cong Wang, Di Xi and Xiaodong Wang for their participation in the laboratory experiments and Wenke Cheng, Dingjun Hu, Minghao Chang and Haojie Li for their contributions to the field works.
Disclosure statement
No potential conflict of interest was reported by the author(s). The authors declare that we have no known competing financial interests or personal relationships that could have appeared to influence the work reported in this paper.
Additional information
Funding
References
- Behr JH, Kampouris ID, Babin D, Sommermann L, Francioli D, Kuhl-Nagel T, Chowdhury SP, Geistlinger J, Smalla K, Neumann G, et al. 2023. Beneficial microbial consortium improves winter rye performance by modulating bacterial communities in the rhizosphere and enhancing plant nutrient acquisition. Front Plant Sci. 14:1. doi: 10.3389/fpls.2023.1232288.
- Bolyen E, Rideout JR, Dillon MR, Bokulich NA, Abnet CC, Al-Ghalith GA, Alexander H, Alm EJ, Arumugam M, Asnicar F, et al. 2019. Reproducible, interactive, scalable and extensible microbiome data science using QIIME 2. Nat Biotechnol. 37(8):852–16. doi: 10.1038/s41587-019-0209-9.
- Callahan BJ, McMurdie PJ, Rosen MJ, Han AW, Johnson AJ, Holmes SP. 2016. DADA2: high-resolution sample inference from Illumina amplicon data. Nat Methods. 13(7):581–583. doi: 10.1038/nmeth.3869.
- Cheong CJ, Okada M. 2001. Effects of spilled oil on the tidal flat ecosystem–evaluation of wave and tidal actions using a tidal flat simulator. Water Sci Technol. 43(2):171–177. doi: 10.2166/wst.2001.0087.
- Ding Y, Chen Y, Lin Z, Tuo Y, Li H, Wang Y. 2021. Differences in soil microbial community composition between suppressive and root rot-conducive in tobacco fields. Curr Microbiol. 78(2):624–633. doi: 10.1007/s00284-020-02318-3.
- Ehlers BK, Berg MP, Staudt M, Holmstrup M, Glasius M, Ellers J, Tomiolo S, Madsen RB, Slotsbo S, Penuelas J, et al. 2020. Plant secondary compounds in soil and their role in belowground species interactions . Trends Ecol Evol. 35(8):716–730. doi: 10.1016/j.tree.2020.04.001.
- Eisenhauer N, Scheu S, Jousset A. 2012. Bacterial diversity stabilizes community productivity . PLOS One. 7(3):e34517. doi: 10.1371/journal.pone.0034517.
- Fan X, Zhang L, Yuan L, Guo B, Zhang Q, Wang Y, Wu Q. 2023. Loss of tidal creek ecosystem vitality caused by tidal flat narrowing on the central Jiangsu coast, China. Sci Total Environ. 864:161216. doi: 10.1016/j.scitotenv.2022.161216.
- Fu L, Xie R, Ma D, Zhang M, Liu L. 2023. Variations in soil microbial community structure and extracellular enzymatic activities along a forest-wetland ecotone in high-latitude permafrost regions. Ecol Evol J. 13(6):e10205. doi: 10.1002/ece3.10205.
- Furey GN, Tilman D. 2021. Plant biodiversity and the regeneration of soil fertility. Proc Natl Acad Sci U S A. 118(49):e2111321118. doi: 10.1073/pnas.2111321118.
- Guo L, Tóth T, Yang F, Wang Z. 2023. Effects of different types of vegetation cover on soil microorganisms and humus characteristics of soda-saline land in the Songnen Plain. Front Microbiol. 14:1163444. doi: 10.3389/fmicb.2023.1163444.
- Hill NK, Woodworth BK, Phinn SR, Murray NJ, Fuller RA. 2021. Global protected-area coverage and human pressure on tidal flats. Conserv Biol. 35(3):933–943. doi: 10.1111/cobi.13638.
- Kõljalg U, Nilsson RH, Abarenkov K, Tedersoo L, Taylor AFS, Bahram M, Bates ST, Bruns TD, Bengtsson-Palme J, Callaghan TM, et al. 2013. Towards a unified paradigm for sequence-based identification of fungi. Mol Ecol. 22(21):5271–5277. doi: 10.1111/mec.12481.
- Lan LY, Yang WQ, Wu FZ, Liu YW, Yang F, Guo CH, et al. 2019. Effects of soil fauna on microbial community during litter decomposition of Populus simonii and Fargesia spathacea in the subalpine forest of western Sichuan, China. . Ying Yong Sheng Tai Xue Bao. 30:2983–2991.
- Li F, Guo Y, Wang Z, Mu Y. 2022. Influence of different phytoremediation on soil microbial diversity and community composition in saline-alkaline land]. Int J Phytoremediation. 24(5):507–517. doi: 10.1080/15226514.2021.1955240.
- Li HL, Ma B, Zhang XL, Zhang QQ, Guo XH, Fan P, et al. 2016. Diversity of culturable endophytic fungi of common reed (Phragmites australis) in coastal wetland. Ying Yong Sheng Tai Xue Bao. 27:2066–2074.
- Li J, Chen Q, Li Q, Zhao C, Feng Y. 2021. Influence of plants and environmental variables on the diversity of soil microbial communities in the Yellow River Delta Wetland, China. Chemosphere. 274:129967. doi: 10.1016/j.chemosphere.2021.129967.
- Li PD, Jeewon R, Aruna B, Li HY, Lin FC, Wang HK. 2019. Metabarcoding reveals differences in fungal communities between unflooded versus tidal flat soil in coastal saline ecosystem. Sci Total Environ. 690:911–922. doi: 10.1016/j.scitotenv.2019.06.473.
- Liang P, Jingan X, Liying S. 2022. The effects of reclaimed water irrigation on the soil characteristics and microbial populations of plant rhizosphere. Environ Sci Pollut Res Int. 29(12):17570–17579. doi: 10.1007/s11356-021-16983-9.
- Martin M. 2011. CUTADAPT removes adapter sequences from high-throughput sequencing reads. EMBnet j. 17(1):10–12. doi: 10.14806/ej.17.1.200.
- Molnár Z, Solomon W, Mutum L, Janda T. 2023. Understanding the mechanisms of Fe deficiency in the rhizosphere to promote plant resilience. Plants . 12(10):1945. doi: 10.3390/plants12101945.
- Quast C, Pruesse E, Yilmaz P, Gerken J, Schweer T, Yarza P, Peplies J, Glöckner FO. 2013. The SILVA ribosomal RNA gene database project: improved data processing and web-based tools. Nucleic Acids Res. 41(Database issue):D590–6. doi: 10.1093/nar/gks1219.
- Ravin NV, Mardanova AV, Skryabin KG. 2015. Metagenomics as a Tool for the Investigation of Uncultured Microorganisms. Genetika. 51:519–528.
- Sarkar S, Kamke A, Ward K, Hartung E, Ran Q, Feehan B, Galliart M, Jumpponen A, Johnson L, Lee STM, et al. 2022. Pseudomonas cultivated from Andropogon gerardii rhizosphere show functional potential for promoting plant host growth and drought resilience. BMC Genomics. 23(1):784. doi: 10.1186/s12864-022-09019-0.
- Shao T, Gu X, Zhu T, Pan X, Zhu Y, Long X, Shao H, Liu M, Rengel Z. 2019. Industrial crop Jerusalem artichoke restored coastal saline soil quality by reducing salt and increasing diversity of bacterial community. Appl Soil Ecol. 138:195–206. doi: 10.1016/j.apsoil.2019.03.003.
- Stevens H, Brinkhoff T, Rink B, Vollmers J, Simon M. 2007. Diversity and abundance of Gram positive bacteria in a tidal flat ecosystem . Environ Microbiol. 9(7):1810–1822. doi: 10.1111/j.1462-2920.2007.01302.x.
- Tang Q, Li Q, Tong L, Wu R, Xu J. 2023. Rhizospheric soil organic carbon accumulated but its molecular groups redistributed via rhizospheric soil microorganisms along multi-root Cerasus humilis plantation chronosequence at the karst rocky desertification control area. Environ Sci Pollut Res Int. 30(28):72993–73007. doi: 10.1007/s11356-023-27588-9.
- Tkacz A, Ledermann R, Martyn A, Schornack S, Oldroyd GED, Poole PS. 2022. Nodulation and nitrogen fixation in Medicago truncatula strongly alters the abundance of its root microbiota and subtly affects its structure. Environ Microbiol. 24(11):5524–5533. doi: 10.1111/1462-2920.16164.
- Van Ryckegem G, Gessner MO, Verbeken A. 2007. Fungi on leaf blades of Phragmites australis in a brackish tidal marsh: diversity, succession, and leaf decomposition. Microb Ecol. 53(4):600–611. doi: 10.1007/s00248-006-9132-y.
- Van Ryckegem G, Van Driessche G, Van Beeumen JJ, Verbeken A. 2006. The estimated impact of fungi on nutrient dynamics during decomposition of Phragmites australis leaf sheaths and stems. Microb Ecol. 52(3):564–574. doi: 10.1007/s00248-006-9003-6.
- Walter J, Buchmann CM, Schurr FM. 2020. Shifts in plant functional community composition under hydrological stress strongly decelerate litter decomposition. Ecol Evol. 10(12):5712–5724. doi: 10.1002/ece3.6310.
- Wang J, Wang Y, He N, Ye Z, Chen C, Zang R, Feng Y, Lu Q, Li J. 2020. Plant functional traits regulate soil bacterial diversity across temperate deserts. Sci Total Environ. 715:136976. doi: 10.1016/j.scitotenv.2020.136976.
- Wang W, Wang Z, Yang K, Wang P, Wang H, Guo L, Zhu S, Zhu Y, He X. 2020. Biochar application alleviated negative plant-soil feedback by modifying soil microbiome. Front Microbiol. 11:799. doi: 10.3389/fmicb.2020.00799.
- Yang Y, Liu H, Yang X, Yao H, Deng X, Wang Y, An S, Kuzyakov Y, Chang SX. 2022. Plant and soil elemental C: n: p ratios are linked to soil microbial diversity during grassland restoration on the Loess Plateau, China. Sci Total Environ. 806(Pt 1):150557. doi: 10.1016/j.scitotenv.2021.150557.
- Yu Y, Liu H, Zhang L, Sun Z, Lei B, Miao Y, Chu H, Han S, Shi Y, Zheng J, et al. 2023. Distinct response patterns of plants and soil microorganisms to agronomic practices and seasonal variation in a floodplain ecosystem. Front Microbiol. 14:1094750. doi: 10.3389/fmicb.2023.1094750.
- Zhang ZF, Yu QG, Wang H, Liu HH, Zhao YC, Xie XY. 2021.Effects of plant community and soil properties on soil bacterial community in Bitahai Wetland, Southwest China]. Ying Yong Sheng Tai Xue Bao. 32:2199–2208.
- Zhao Y, Liu Q, Huang R, Pan H, Xu M. 2020. Recent evolution of Coastal Tidal flats and the impacts of intensified human activities in the Modern Radial Sand Ridges, East China. Int J Environ Res Public Health. 17(9):3191. doi: 10.3390/ijerph17093191.