ABSTRACT
Purpose: Choroidal neovascularization (CNV) is a common vision-threatening complication associated with many fundus diseases. The retinal pigment epithelial (RPE) cell junction barrier has critical functions in preventing CNV, and oxidative stress can cause compromise of barrier integrity and induce angiogenesis. Rap1, a small guanosine triphosphatase (GTPase), is involved in regulating endothelial and epithelial cell junctions. In this work, we explored the function and mechanism of Rap1 in CNV in vivo.
Methods: A laser-induced rat CNV model was developed. Rap1 was activated through intravitreal injection of the Rap1 activator 8CPT-2ʹ-O-Me-cAMP (8CPT). At 14 days after laser treatment, CNV size in RPE/choroid flat mounts was measured by fluorescein isothiocyanate-dextran staining. Expression of vascular endothelial growth factor (VEGF) and cell junction proteins in RPE/choroid tissues were analyzed by western blots and quantitative real-time PCR assays. Reactive oxygen species (ROS) in RPE cells were detectedbydichloro-dihydro-fluorescein diacetate assays. The antioxidant apocynin was intraperitoneally injected into rats.
Results: Activating Rap1 by 8CPT significantly reduced CNV size and VEGF expression in the rat CNV model. Rap1 activation enhanced protein and mRNA levels of ZO-1 and occludin, two tight junction proteins in the RPE barrier. In addition, reducing ROS generation by injection of apocynin, a NADPH oxidase inhibitor, inhibited CNV formation. Rap1 activation reduced ROS generation and expression of NADPH oxidase 4.
Conclusions: Rap1 activation inhibits CNV through regulating barrier integrity and ROS generation of RPE in vivo, and selectively activating Rap1 may be a way to reduce vision loss from CNV.
Introduction
Choroidal neovascularization (CNV) is a major vision-threatening complication associated with several common retinal degenerative and inflammatory diseases, such as age-related macular degeneration (AMD), central exudative chorioretinopathy, pathological myopia, angioid streaks, ocular histoplasmosis syndrome, and chorioretinitis.Citation1 CNV often damages the macula, leading to exudation or hemorrhage and scar forming that causes visual loss.Citation2 Activated choroidal endothelial cells (CECs) originating from the choriocapillaris migrate through the Bruch membrane toward the retinal pigment epithelium (RPE), and severe vision loss occurs most often when they transmigrate the RPE into the neurosensory retina to proliferate into CNV.Citation3 The RPE is thought to be critical for preventing CNV development. Normally, the RPE forms a monolayer connected by tight junctions that create the outer blood-retinal barrier and breakdown is a step in CNV pathogenesis.Citation4 However, in aging eyes, oxidative stress, metabolic stress, hypoxia, and inflammation compromise the RPE barrier and induce angiogenesis.Citation5 Specifically, excessive reactive oxygen species (ROS) induce dysregulation of functional networks and deleterious changes in the RPE that result in CNV.Citation6
Rap1 is a member of the Ras guanosine triphosphatase (GTPase) superfamily. It has two isoforms, Rap1a and Rap1b, that share 95% amino acid identity.Citation7 Like all GTPases, Rap1 acts as a molecular switch, cycling between an active (GTP-bound) and an inactive (GDP-bound) form through guanine nucleotide exchange factors and GTPase-activating proteins (GAPs). Cumulative evidence shows that activation of Rap1 strengthens cell junction integrity and reduces cell migration by affecting actin cytoskeleton remodeling.Citation8–Citation10 Both Rap1a and Rap1b are expressed in RPE. Previous studies have showed that Rap1 expression and activity are involved in the formation and development of CNV.Citation11 In this work, we developed a rat laser-induced CNV model and activated Rap1 in vivo by a cAMP analog 8CPT-2ʹ-O-Me-cAMP (8CPT) to explore the mechanism of Rap1 regulating CNV. 8CPT specifically activates Rap1 via effects on upstream exchange factors (Epac1 and Epac2), but does not activate other cAMP-responsive pathways, e.g., Ras and ERK.Citation12 Our results show that Rap1 activation reduces CNV size and ROS generation of RPE, and enhances RPE cell junction in a rat laser-induced CNV model.
Materials and methods
Animals
Brown Norway (BN) rats (male, aged 8–10 weeks, 180–200 g) were from Vital River Laboratory Animal Technology Co., Ltd (Beijing, China). Prior to experiments, slit-lamp and ophthalmoscopic examinations were performed to exclude abnormalities in eyes. The study protocols were approved by the Ethical Committee of Affiliated Second Hospital of Hebei Medical University.
Laser-induced CNV model and injections
BN rats were anesthetized with intraperitoneal injections of 10% chloral hydrate (0.35 ml/kg), and pupils were dilated with 1% tropicamide and 1% mydriacyl. Using a 532 nm laser (Coherent Novus Omni, Palo Alto, CA, USA), a slit-lamp delivery system, and a coverslip as a contact lens, six spots (300 mW, 200 μm, and 50 ms) were placed in each eye. Lesions were 2–3 disk diameters from the optic nerve head. Development of a bubble under laser radiation confirmed rupture of Bruch’s membrane. Lesions with hemorrhage were excluded. Right after laser injury, a 1 μl intravitreal injection of 8CPT (20.5 μM; Calbiochem, Darmstadt, Germany) was delivered to activate Rap1 in vivo. In separate rats, phosphate buffer saline (PBS) intravitreal injection was the control. Ten rats were used in each group, and both eyes of each rat were lasered and injected with the same reagent. To inhibit ROS generation in vivo, an intraperitoneal injection of apocynin (10 mg/kg/d, Sigma–Aldrich, St. Louis, MO, USA) was delivered daily for 14 days. PBS injection was the control.
Measurement of CNV and CNV lesions
At 14 days after laser treatment, animals were perfused with 1 ml PBS containing 50 mg/ml fluorescein isothiocyanate-labeled dextran (FITC-dextran, average MW 2 × 106; Sigma–Aldrich) before sacrifice. Eyes were harvested and fixed for 1 h in 10% phosphate-buffered formalin. Cornea, lens, and vitreous were removed. Posterior eyecups of the RPE, choroid, and sclera were dissected and flat-mounted on microscope slides by cutting radial incisions. CNV images were captured using a laser scanning confocal microscope (Bio-Rad, Hercules, CA, USA), and images at different focal planes were stacked and flattened. Lesions with obvious hemorrhage or bridging were excluded. The fluorescent area of lesion spot was quantified with ImageJ software (National Institutes of Health, Bethesda, MD, USA). Ten lesions from at least three rats per group were analyzed.
Western blots
RPE/choroid tissues were triturated, lysed on ice in RIPA buffer, and boiled in SDS loading buffer. Protein extracts were subjected to 6–12% SDS-PAGE and processed with a standard protocol. Primary antibodies for GTP-Rap1 (1:1000, NewEast Biosciences, King of Prussia, PA, USA), vascular endothelial growth factor (VEGF, 1:1000, Santa Cruz Biotechnology, Santa Cruz, CA, USA), zonula occludens-1 (ZO-1, 1:2000, Santa Cruz Biotechnology), occludin (1:2000, Santa Cruz Biotechnology), NADPH oxidase 4 (NOX4, 1:1000, Abcam, Cambridge, UK), and β-actin (1:4000, Abclone, Wuhan, China) were used.
Quantitative real-time PCR assays
Total RNA was extracted from RPE/choroid tissues and reverse transcribed to cDNA. Quantitative real-time PCR (qPCR) analysis was performed with SYBR Premix Ex Taq™ kits (TAKARA Bio, Dalian, China) on an ABI7500 Real-Time PCR System (Applied Biosystems, Foster City, CA, USA). Relative gene expression was normalized to GAPDH with the 2−ΔΔCt method. The qPCR primer sequences are in .
Table 1. Primers for qPCR.
Measurement of ROS productions
Rat RPE cells were isolated according to the method of Heller et al.Citation13 Isolated cells were immediately incubated in 5 μM 2,7-dichlorodihydro-fluorescein diacetate (DCFH-DA, Invitrogen, Carlsbad, CA, USA) in serum-free medium for 30 min at 37°C and washed twice with PBS. DCFH-DA is cell-permeable and hydrolyzed intracellularly to the DCFH carboxylate anion; this is retained in cells, and induced by ROS to form a fluorescent product, dichlorofluorescein (DCF). ROS-induced DCF fluorescence was measured with a fluorescent plate reader with excitation 485 nm and emission 520 nm. Simultaneously, fluorescence emitted by cells on slides was observed with a confocal microscope. Cells incubated with 10 μM H2O2 were the positive control.
Statistical analysis
All experiments were performed in triplicate. Data are means ± SEM, and differences between groups were analyzed with independent-sample t-tests (two-tailed, GraphPad Prism 5, GraphPad Software, Inc., La Jolla, CA, USA). P < 0.05 was considered statistically significant. One representative set of data from western blots and fluorescent images were shown.
Results
Rap1 activation inhibits laser-induced CNV
To explore the effect of Rap1 on CNV, 8CPT, a known Rap1 activator, was used in a rat CNV model. We confirmed that 8CPT treatment activated Rap1 in rats. Western blots showed that active Rap1 increased after 8CPT intravitreal injection (). We observed the response of rats to laser injury after 8CPT treatment. CNV was induced by photocoagulation using an argon laser, and all spots were positive for CNV at 14 days after laser treatment. Confocal analyses of RPE-choroid-scleral flat mounts by FITC-dextran revealed significantly smaller CNV lesion areas in 8CPT-injected groups than in PBS control groups (). Western blots showed VEGF expression in RPE/choroid tissue was significantly decreased in 8CPT-injected rats compared with control rats ().
Figure 1. Rap1 activation inhibits laser-induced CNV in rats.
A. Western blot of GTP-Rap1 in RPE/choroid tissues from CNV rats with PBS and 8CPT injection. B. Quantification of results in A. Protein levels normalized against Rap1, n = 3. **P < 0.01. C. Representative confocal images of RPE/choroid flat mounts stained with FITC-dextran in PBS and 8CPT groups. Scale bar = 100 μm. D. Quantification of CNV area. n = 10 lesions. ***P < 0.001. E. Western blot of VEGF in RPE/choroid tissues from CNV rats with PBS and 8CPT injection. F. Quantification of results in E. Protein levels normalized against β-actin, n = 3. **P < 0.01.
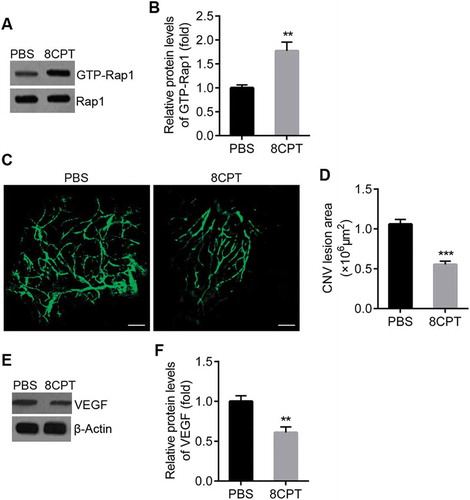
Rap1 activation strengthens RPE cell junctions
Previous reports implicated Rap1 in cell junction regulation. We detected expression of ZO-1 and occludin, components of the tight junction complex, after 8CPT treatment. Western blots showed that ZO-1 and occludin protein in RPE/choroid tissue was significantly increased in 8CPT-injected rats compared with control rats (). qPCR indicated that ZO-1 and occludin mRNA also increased with 8CPT ()).
Figure 2. Rap1 activation enhances expression of RPE cell junction proteins.
A. Western blot of ZO-1 and occludin in RPE/choroid tissues from CNV rats with PBS and 8CPT injection. B. Quantification of results in A. Protein normalized against β-actin. C. Quantitative real-time PCR for ZO-1 and Occludin mRNA in two groups, n = 3. *P < 0.05. **P < 0.01. ***P < 0.001.
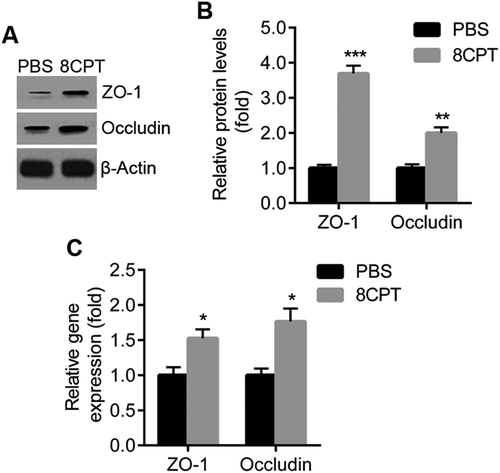
Inhibition of ROS generation reduces CNV
To confirm the effect of ROS in CNV, we used apocynin, an NADPH oxidase inhibitor, to reduce ROS generation in CNV. After apocynin injection, NOX4 expression was reduced in rat RPE/choroid by western blot (). ROS generation was measured as DCF fluorescence in the RPE cells of rats treated with intraperitoneal apocynin or PBS. Fluorescence was reduced in the apocynin-injected group (). Administration of apocynin reduced CNV lesion size in rats ().
Figure 3. Inhibiting ROS generation with apocynin reduces CNV.
A. Western blot of NOX4 in RPE/choroid tissues from CNV rats with PBS and apocynin injection. B. Quantification of results in A. Protein normalized against β-actin, n = 3. **P < 0.01. C. Representative image of ROS generation in RPE cells from CNV rats with PBS and apocynin injection. RPE cells were isolated and cultured in DCFH-DA, and DCF fluorescence from cells on slides observed by confocal microscope. Scale bar = 20 μm. D. Quantification of ROS generation. ROS-induced DCF fluorescence measured with a fluorescence plate reader with excitation 485 nm and emission 520 nm, n = 3. *P < 0.05. E. Representative confocal images of RPE/choroid flat mounts stained with FITC-dextran in PBS and apocynin groups. Scale bar = 100 μm. F. Quantification of CNV area, n = 10 lesions. ***P < 0.001.
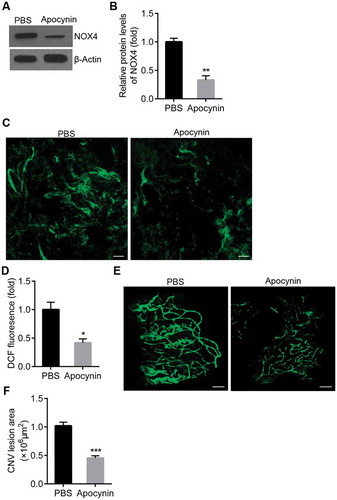
Rap1 activation reduces ROS generation in the RPE at CNV lesions
To determine if activation of Rap1 reduced CNV area by inhibiting ROS generation, ROS in the RPE cells was measured in rats treated with intravitreal 8CPT or control. The strength of DCF fluorescence in the RPE cells was significantly decreased in rats that received 8CPT injections compared to PBS (). Moreover, 8CPT injection caused a decrease in NOX4 protein and mRNA by western blot and qPCR (). These results suggested that activation of Rap1 could reduce CNV formation by inhibiting ROS generation in the RPE.
Figure 4. Rap1 activation reduces ROS generation in RPE at CNV lesions.
A. Representative image of ROS generation in RPE cells from CNV rats with PBS and 8CPT injection. RPE cells were isolated and cultured in DCFH-DA, and DCF fluorescence emitted by cells on slides was observed by confocal microscope. Scale bar = 20 μm. B. Quantification of ROS generation. ROS-induced DCF fluorescence was measured with a fluorescence plate reader with excitation 485 nm and emission 520 nm, n = 3. *P < 0.05. C. Western blot of NOX4 in RPE/choroid tissues from CNV rats with PBS and 8CPT injection. D. Quantification of results in C. Protein normalized against β-actin, n = 3. *P < 0.05. E. Quantitative real-time PCR of NOX4 mRNA in two groups, n = 3. *P < 0.05.
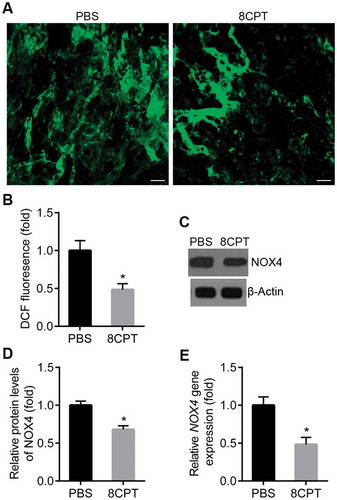
Discussion
In this work, we showed that activating Rap1 by 8CPT, a known Rap1 activator, reduced CNV size and VEGF expression in a rat laser-induced CNV model. Rap1 activation enhanced expression of ZO-1 and occludin, two tight junction proteins in the RPE barrier. Furthermore, Rap1 activation reduced NOX4 expression and ROS generation. These results demonstrated that Rap1 activation inhibited CNV through regulating barrier integrity and ROS generation in the RPE ().
Figure 5. Schematic of Rap1 in regulating CNV. Rap1 inhibits CNV through enhancing cell junctions and decreasing ROS generation in RPE cells. An alternative mechanism may exist in which Rap1 inhibits CNV by regulating these function of CECs.
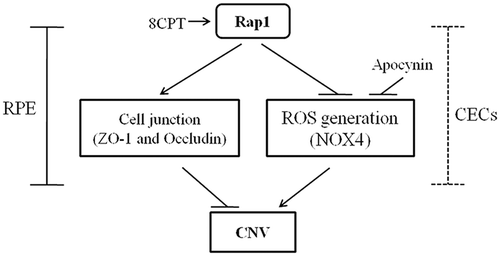
The RPE maintains the outer blood-retinal barrier. Intact barrier integrity is important for the health and function of the retinal photoreceptors and choriocapillaris.Citation14 The barrier comprises a single layer of epithelium cells that have tight junctions. Impairment of junctional regulation interferes with compartmentalization of angiogenic agonists normally secreted from the RPE basal aspect, and inhibitors secreted from the apical aspect, by permitting movement of factors to other compartments.Citation15 This change in concentrations of angiogenic factors such as VEGF activates motile CECs. Tight junctions consist of multiprotein complexes including transmembrane protein components, such as occludin and cadherins, and cytoplasmic protein components, such as ZO-1 and β-, α-, and p120-catenin. Some of these bind to the actin cytoskeleton.Citation16 Of the signaling proteins involved in junctional regulation, small GTPases are focused because of their ability to cycle between active and inactive states. Small GTPases of the Rho family regulate cell junctions by affecting actin cytoskeleton remodeling.Citation17,Citation18
For the small GTPase Rap1, Hartnett ME and colleagues reported that in the RPE line ARPE-19, knockdown or inhibition of Rap1 activity impairs barrier functions. This result was shown with reduced transepithelial electrical resistance and electrical impedance, mislocalization of cadherins, formation of gaps within the monolayer, and higher transmigration of CECs.Citation15 These results suggest Rap1 regulates RPE junctional barrier function and might potentiate CNV. For the specific functions of Rap1a and Rap1b isoforms, in vitro, Rap1a short hairpin RNA (shRNA) educes steady-state barrier integrity, whereas Rap1b shRNA affects dynamic junctional responses. In vivo, Rap1b−/− mice have larger CNV volumes compared to wild-type or Rap1a−/−.Citation11 In addition, increasing Rap1a activity specifically in RPE cells by self-complementary adeno-associated virus 2 vectors reduces CNV volume in vivo and inhibits CEC transmigration across RPE monolayers in vitro.Citation19 Our data showed activating Rap1 reduced CNV size and VEGF expression, and thus inhibited CNV development in a rat laser-induced CNV model. Activating Rap1 also enhanced the RPE barrier by increasing protein and mRNA for ZO-1 and occludin, two tight junction proteins. Our conclusion was consistent with previous reports from the Hartnett laboratory. We used a rat model and different experiments to further confirm the critical function of Rap1 in CNV formation.
The barrier function of vascular endothelial cells maintained by adherens and tight junctions is central for regulating angiogenesis and inflammation.Citation20,Citation21 In microvascular endothelial cells, Rap1 regulates the accumulation of adherens and tight junction proteins to the cell–cell contact sites.Citation17,Citation21,Citation22 In CNV, besides the RPE barrier, Rap1 may also regulate the barrier function of CECs to affect angiogenesis.Citation15,Citation23 Therefore, an alternative interpretation to increased ZO-1 and occludin expression by 8CPT is that CECs have more stable tight junctions, and thus they are less likely to remodel and migrate (). This could yield an inhibition to CNV lesion. We need further data to identify the role of Rap1 in RPE and CECs.
Multiple risk factors, including obesity, hypertension, smoking, and light exposure, are reported to contribute to AMD pathogenesis through increasing oxidative stress generated by several mechanisms, including constant exposure to light and ROS.Citation24 ROS cause cell damage directly but can also trigger intracellular signal transduction that leads to various outcomes in different cell types involved in AMD.Citation25 Hartnett et al. demonstrated that in CECs, overexpressing active Rap1a by 8CPT inhibits TNF-α-induced CEC migration by inhibiting NADPH oxidase-dependent NF-kB and Rac1 activation. This result suggests that Rap1a de-escalates CNV development by interfering with ROS-dependent signaling.Citation23 Similarly, in RPE inhibition of Rap1 by Rap1GAP increases ROS generation, whereas activation of Rap1 by 8CPT reduces ROS by interfering with NADPH oxidase assembly.Citation26 Our results in a rat laser-induced CNV model showed that reducing ROS generation by antioxidant apocynin treatment inhibited CNV formation in rats. Rap1 activation decreased NOX4 expression and ROS generation, suggesting that Rap1 affected CNV by regulating oxidative stress. Our results confirmed and extended the conclusion of Wang et al.Citation26
In conclusion, our findings indicated that Rap1 GTPase is important in CNV through regulating RPE junctional barrier function and ROS generation in vivo. Our results suggested that selectively activating Rap1 may be a way to reduce vision loss from CNV and improve clinical outcomes.
Declaration of interest
The authors report no conflicts of interest. The authors alone are responsible for the content and writing of this article.
Additional information
Funding
References
- de Jong P. Mechanisms of disease: age-related macular degeneration. N Engl J Med. 2006;355(14):1474–85. doi:10.1056/NEJMra062326.
- Kwak N, Okamoto N, Wood JM, Campochiaro PA. VEGF is major stimulator in model of choroidal neovascularization. Invest Ophthalmol Vis Sci. 2000;41(10):3158–64.
- Grossniklaus HE, Green WR. Choroidal neovascularization. Am J Ophthalmol. 2004;137(3):496–503. doi:10.1016/j.ajo.2003.09.042.
- Gehrs KM, Anderson DH, Johnson LV, Hageman GS. Age-related macular degeneration-emerging pathogenetic and therapeutic concepts. Ann Med. 2006;38(7):450–71. doi:10.1080/07853890600946724.
- Penn JS, Madan A, Caldwell RB, Bartoli M, Caldwell RW, Hartnett ME. Vascular endothelial growth factor in eye disease. Prog Retin Eye Res. 2008;27(4):331–71. doi:10.1016/j.preteyeres.2008.05.001.
- Li Q, Dinculescu A, Shan Z, Miller R, Pang J, Lewin AS, Raizada MK, Hauswirth WW. Downregulation of p22phox in retinal pigment epithelial cells inhibits choroidal neovascularization in mice. Mol Ther. 2008;16(10):1688–94. doi:10.1038/mt.2008.164.
- Hilbi H, Kortholt A. Role of the small GTPase Rap1 in signal transduction, cell dynamics and bacterial infection. Small GTPases. 2017;1–7. doi:10.1080/21541248.2017.1331721.
- Boettner B, Van Aelst L. Control of cell adhesion dynamics by Rap1 signaling. Curr Opin Cell Biol. 2009;21(5):684–93. doi:10.1016/j.ceb.2009.06.004.
- Liu JJ, Stockton RA, Gingras AR, Ablooglu AJ, Han J, Bobkov AA, Ginsberg MH. A mechanism of Rap1-induced stabilization of endothelial cell–cell junctions. Mol Biol Cell. 2011;22(14):2509–19. doi:10.1091/mbc.E11-02-0157.
- Pannekoek WJ, Post A, Bos JL. Rap1 signaling in endothelial barrier control. Cell Adh Migr. 2014;8(2):100–07. doi:10.4161/cam.27352.
- Ljubimov AV, Wittchen ES, Nishimura E, McCloskey M, Wang H, Quilliam LA, Hartnett ME, Ljubimov AV. Rap1 GTPase activation and barrier enhancement in RPE inhibits choroidal neovascularization in vivo. PLoS ONE. 2013;8(9):e73070. doi:10.1371/journal.pone.0073070.
- Enserink JM, Christensen AE, de Rooij J, van Triest M, Schwede F, Genieser HG, Døskeland SO, Blank JL, Bos JL. A novel Epac-specific cAMP analogue demonstrates independent regulation of Rap1 and ERK. Nat Cell Biol. 2002;4(11):901–06. doi:10.1038/ncb874.
- Heller JP, Kwok JC, Vecino E, Martin KR, Fawcett JW. A method for the isolation and culture of adult rat Retinal Pigment Epithelial (RPE) cells to study retinal diseases. Front Cell Neurosci. 2015;9:449. doi:10.3389/fncel.2015.00449.
- Bhutto I, Lutty G. Understanding age-related macular degeneration (AMD): relationships between the photoreceptor/retinal pigment epithelium/Bruch’s membrane/choriocapillaris complex. Mol Aspects Med. 2012;33(4):295–317. doi:10.1016/j.mam.2012.04.005.
- Wittchen ES, Hartnett ME. The small GTPase Rap1 is a novel regulator of RPE cell barrier function. Invest Ophthalmol Vis Sci. 2011;52(10):7455–63. doi:10.1167/iovs.11-7295.
- Hartsock A, Nelson WJ. Adherens and tight junctions: structure, function and connections to the actin cytoskeleton. Biochim Biophys Acta. 2008;1778(3):660–69. doi:10.1016/j.bbamem.2007.07.012.
- Spindler V, Schlegel N, Waschke J. Role of GTPases in control of microvascular permeability. Cardiovasc Res. 2010;87(2):243–53. doi:10.1093/cvr/cvq086.
- Terry S, Nie M, Matter K, Balda MS. Rho signaling and tight junction functions. Physiology (Bethesda, Md). 2010;25(1):16–26.
- Wang H, Han X, Bretz CA, Becker S, Gambhir D, Smith GW, Samulski RJ, Wittchen ES, Quilliam LA, Chrzanowska-Wodnicka M, et al. Retinal pigment epithelial cell expression of active Rap 1a by scAAV2 inhibits choroidal neovascularization. Mol Ther Methods Clin Develop. 2016;3:16056. doi:10.1038/mtm.2016.56.
- Schlegel N, Waschke J. cAMP with other signaling cues converges on Rac1 to stabilize the endothelial barrier- a signaling pathway compromised in inflammation. Cell Tissue Res. 2014;355(3):587–96. doi:10.1007/s00441-013-1755-y.
- Tawa H, Rikitake Y, Takahashi M, Amano H, Miyata M, Satomi-Kobayashi S, Kinugasa M, Nagamatsu Y, Majima T, Ogita H, et al. Role of Afadin in vascular endothelial growth factor- and Sphingosine 1-phosphate-induced angiogenesis. Circ Res. 2010;106(11):1731–42. doi:10.1161/CIRCRESAHA.110.216747.
- Cullere X. Regulation of vascular endothelial barrier function by Epac, a cAMP-activated exchange factor for Rap GTPase. Blood. 2005;105(5):1950–55. doi:10.1182/blood-2004-05-1987.
- Wang H, Fotheringham L, Wittchen ES, Hartnett ME. Rap1 GTPase inhibits tumor necrosis factor-α–induced choroidal endothelial migration via NADPH Oxidase– and NF-κB–dependent activation of Rac1. Am J Pathol. 2015;185(12):3316–25. doi:10.1016/j.ajpath.2015.08.017.
- Nishimura Y, Hara H, Kondo M, Hong S, Matsugi T. Oxidative stress in retinal diseases. Oxid Med Cell Longev. 2017;2017:4076518. doi:10.1155/2017/4076518.
- Ye F, Kaneko H, Hayashi Y, Takayama K, Hwang SJ, Nishizawa Y, Kimoto R, Nagasaka Y, Tsunekawa T, Matsuura T, et al. Malondialdehyde induces autophagy dysfunction and VEGF secretion in the retinal pigment epithelium in age-related macular degeneration. Free Radic Biol Med. 2016;94:121–34. doi:10.1016/j.freeradbiomed.2016.02.027.
- Wang H, Jiang Y, Shi D, Quilliam LA, Chrzanowska-Wodnicka M, Wittchen ES, Li DY, Hartnett ME. Activation of Rap1 inhibits NADPH oxidase-dependent ROS generation in retinal pigment epithelium and reduces choroidal neovascularization. Faseb J. 2014;28(1):265–74. doi:10.1096/fj.13-240028.