Abstract
Once bone metastasized and androgen independent, prostate cancer is often associated with skeletal morbidity and disability. New treatment modalities that can palliate symptoms from the skeleton and inhibit further progression are warranted. In this study, the antitumoral effects following treatment with a combination of docetaxel and the new generation bisphosphonate, zoledronic acid, were investigated on two hormone-refractory prostate cancer cell lines: PC3 and DU145. The prostate cancer cells were treated with increasing concentrations of zoledronic acid in the absence or presence of docetaxel. Toxicity was measured using fluorometric microculture cytotoxic assay technique. A concentration of 25 µM, zoledronic acid reduced the viable cell number to 68% and 98% for PC3 and DU145 cells respectively. Docetaxel, on the other hand, at a concentration of 0.1 ng/ml, had no effect on the viability. However, a combination of zoledronic acid and docetaxel reduced the cell number to 60% and 81% respectively. Furthermore, zoledronic acid in the concentration range 12.5 µM–50 µM enhanced the antitumoral effects of docetaxel (0.01–1 ng/ml) in an additive and/or synergistic manner for both cell lines. These data support the hypothesis that zoledronic acid, in addition to having bone resorption inhibiting properties, also exhibits anti-tumoral effects. It also appears that combined treatment with docetaxel causes additive and/or synergistic cytostatic effects on prostate cancer cells.
Introduction
Prostate cancer is the most common cancer in men and the second leading cause of death among men in Western countries. The survival rate for early stage prostate cancer is high but for relapsing tumors there is no adequate salvage therapy. Androgen deprivation constitutes first-line therapy for metastatic prostate cancer with a median survival of approximately three years after diagnosis of metastasis. As a rule, however, the disease progresses from an androgen-dependent to an androgen-independent state, thus rendering hormonal therapy less effective. At this stage the treatment options are limited and in the presence of bone metastases the disease is often associated with severe skeletal morbidity. New treatment modalities that can palliate skeletal symptoms and inhibit further progression are thus warranted.
Bisphosphonates (BPs) constitute a new medical therapeutic option for bone metastatic disease. The therapeutic effects have mainly been related to inhibitory effects on osteoclasts, which explains why BPs essentially have been used to treat pain and prevent skeletal complications from patients with lytic disease such as breast cancer and multiple myeloma Citation[1]. It is hypothesized however, that every metastatic cancer in bone begins with osteolysis Citation[2] and sclerotic metastases may in fact have increased activities of both osteoclasts and osteoblasts Citation[3]. Several studies have demonstrated clinical benefits, such as pain relief and reduction of skeletal related events following treatment with BPs, including in patients with advanced prostate cancer Citation[4], Citation[5].
It has been discussed whether the effects of BPs in part could be related to direct anti-tumoral effects. The mechanisms behind BPs effects on cancer cells are not completely clear, but activation of caspases and inhibition of farnesyl disphosphate synthase, a key enzyme in the mevalonate pathway, are suggested mechanisms of action Citation[6–8]. The latter leads to reduced prenylation of small GTP-binding proteins, which are essential for normal cell function and survival. In vitro, nitrogen containing-BPs (N-BPs) in submicromolar concentrations have been shown to inhibit tumor cell adhesion and furthermore to reduce invasion through the extracellular matrix. At higher concentrations, antiproliferative and proaptotic effects have been reported. Several in vitro studies have, indeed, demonstrated varying anti-proliferative and cytostatic effects on myeloma derived cell lines Citation[9–11] breast cancer cell lines Citation[12], Citation[13] and prostate cancer cell lines Citation[8], Citation[14–16]. By use of flow cytometry, Lee et al. Citation[15] demonstrated that pamidronate treatment (100 µM) induced cell death in the three prostate cancer cell lines PC3, DU 145, and LNCaP, while zoledronic acid (ZOL) treatment instead exerted dramatic effects on cell proliferation. Corey et al. Citation[16] confirmed these antitumoral effects and also demonstrated in vivo inhibitory effects on osteoblastic and osteolytic lesions in SCID mice following treatment with ZOL. Moreover, Dumon et al. Citation[14] showed that the anti-tumoral effects are related to factors such as dose and exposure time and that the effects vary among BPs with aminobisphosphonates being the most efficient. Synergistic cytotoxic effects in breast cancer cells have been shown when combining ZOL with paclitaxel Citation[17] or docetaxel Citation[18] and also for myeloma cells when combining ZOL and dexamethasone Citation[19].
Docetaxel (DOC) belongs to the taxoid family and acts primarily by inhibiting microtubular depolymerization and has been found to counter the effects of bcl-2 and bcl-x gene expression. It is believed that DOC concentrates within cells making it a potent intracellular stabilizer and, finally, polymerized microtubules cannot be dissembled in the presence of DOC. Cells arrest in the G2M phase of the cell cycle, ultimately leading to apoptosis. In earlier phase II trials of DOC-based regimens in patients with hormone refractory prostate cancer, decreased PSA levels and measurable disease have been observed. Recently, the results were presented of a phase III study comparing mitoxantrone + prednisone versus weekly or intermittent DOC + prednisone for patients with advanced prostate cancer. An improved survival and favorable pain and PSA responses were shown in the intermittent DOC arm, indicating that DOC might be an useful drug for this group of patients Citation[20].
The aim of this study was to further explore the anti-tumoral potential of ZOL, more specifically to see if additive and super-additive anti-tumoral effects can be achieved on DU145 and PC3 cells following combined treatment with DOC.
Material and methods
Cell lines and culture conditions
The human, hormone refractory, metastatic prostate carcinoma cell lines DU145 and PC3 cell lines were cultured in 5 ml dishes (Falcon) with RPMI 1640 (GIBCO); 2 mM glutamine (GIBCO), 10% fetal calf serum (Life Technologist) was added to the media. The cells were kept in a 37°C incubator with humidified air and 5% CO2. The culture medium was changed twice a week. Cells were removed from the dishes by treatment trypsin-EDTA (Sigma, USA). Both cell lines were obtained from American Type Culture Collection (ATCC, USA).
Drugs
Different concentrations of ZOL (Novartis, Basel, Switzerland) (6.25–100 µM) and/or DOC (Aventis Pharmaceutical, NJ) (0.01–100 ng/ml) were used for the FMCA and morphological studies. For a comparison, peak plasma values of DOC reach 3.7 µg/ml following one-hour infusion of the drug when used clinically, at a dose of 100 mg/m2. Clinical ZOL concentrations in serum are discussed in the discussion section, please see below.
Fluorometric microculture cytotoxic assay (FMCA)
The FMCA method was adopted from Larsson et al. Citation[21]. On day one, 200 µl/ well of the tumor cell preparation (10 000 cells/well) were seeded in triplicates into 96 well microtiter plates. Thus, the tumor cells were allowed to attach to the bottom of the plate before the drugs were added. The culture plates were then incubated at 37°C in humidified atmosphere containing 95% air and 5% CO. At the end of 72 hours’ incubation, the culture medium was washed away, the 96 well plates rinsed three times in phosphate buffer saline pH 7.4, and followed by addition of 200 µl/well of physiological buffer containing 10 µg/ml fluorescence-diacetate (FDA). After incubation for 30 min at 37°C, the fluorescence from each well was read in a Fluoroscan 2 (Lab-systems OY, Helsinki, Finland). Technically, the fluorescence generated by hydrolysis of FDA to fluorescin by viable cells is measured.
The FMCA assay was used to measure the remaining viable cell number of PC3 and DU145 cells following treatment with DOC or ZOL alone or in different combinations. An additive cytostatic effect is referred to as an effect that significantly reduces the number of viable cells more than either of these drugs alone, while a synergistic effect has been defined as an effect that reduces the viable cell number more than the sum of the reduction caused by either drug alone. The dose–response experiments were done six times and the combined treatment experiments four times. Average values are presented.
Cell preparation with DAPI
On day one the cells were seeded on 4 well glasses (Nunc) with 50 000/well in 500 µl media. After 24 h, ZOL in concentrations of 25, 50, and 100 µM and DOC in concentrations 0.1 and 1.0 ng/ml were added. Combinations of ZOL and DOC were also done in all concentrations. As a positive control for induction of apoptotic cells, DOC at a concentration of 10 ng/ml was used. After 72 h, the wells were rinsed three times with PBS and first fixed in 4% formaldehyde for 5 min at room temperature, rinsed in PBS and finally fixed in methanol at −20°C for 20 minutes. The glasses were mounted with DAPI mounting medium (Vector). DAPI (4’, 6-diamidino-2-phenylindole, dihydrochloride) is a DNA staining and binds to the grooves at the surface of the DNA helix. The fluorophore has a peak absorbance at 358 nm and emission at 461 nm. It is best excited by an UV laser, 356 nm.
Statistical analysis
The unpaired t-test was used to compare differences in the mean values. To test the assumption that data were normally distributed a Normal plot was performed in each group of variables; p-values less then 0.05 were considered statistically significant. All significant differences were recalculated according to the non-parametric Mann–Whitney U test, with no alteration in the conclusions. The tests were performed with Statistica™ Release 4.1 software for Macintosh.
Results
Anti-tumoral effects following ZOL and DOC treatment
Incubation with ZOL for 72 h caused a dose-dependent decrease in the viable cell number for both PC3 cells (A) and DU145 cells (Figure 1B). PC3 cells showed a high sensitivity to ZOL with a decrease of viable cells from 90% at 6.25 µM to 44% at 100 µM, as compared with controls (p < 0.05) (Figure 1A). DU145 cells were less sensitive to ZOL than PC3 cells, since 97% and 71% viable cells were detected at 6.25 µM and 100 µM, respectively and to achieve a statistically significant reduction (p < 0.05) of the viable DU145 cell number, a concentration of 50 µM or higher was required (Figure 1B). The sensitivity to DOC was inverted for these two cell lines with DU145 cells being the most sensitive. In concentrations below 0.1 ng/ml, only a tendency to cytostatic effects was seen for both cell lines but at 1 ng/ml and higher concentrations, the effects were pronounced.
Figure 1. (A) and (B): As compared with untreated control cells, treatment with ZOL in concentrations from 6.25 µM or higher showed a statistically significant and dose-dependent lower number of viable PC3 cells. For the DU145 cells, higher concentrations of ZOL, i.e. 50 µM and 100 µM, were needed to achieve statistically significant cytostatic effects. Error bars indicate standard deviations.
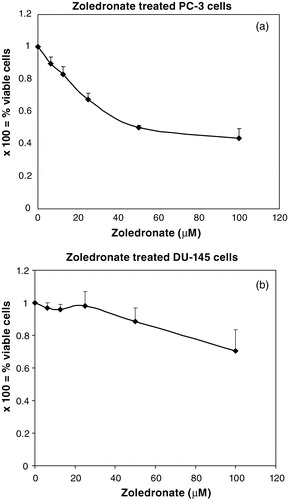
Treatment with DOC only at a concentration of 1 ng/ml caused a reduction of viable cells to 74% and addition of 25 µM ZOL reduced significantly the viable cell number to 48% (p < 0.05). Thus, an additive effect was observed for PC3 cells when combining DOC at 1 ng/ml and ZOL at 25 µM (A). At lower DOC concentrations the effects from this drug were negligible and mainly from ZOL and vice versa; at a DOC concentration of 10 ng/ml or higher, the effects were mainly from DOC and the additive effects only a trend without statistical significance (Figure 2A). Synergistic anti-tumoral effects on DU145 cells were demonstrated for the combined treatment (Figure 2B). At a DOC concentration of 0.1 ng/ml the viable cell number was 95% and at a ZOL concentration of 25 µM the viable cell number was 98%, as compared with controls (Figure 2B). At these concentrations, the combined treatment demonstrated a significant reduction of the viable cell number to 81% and by increasing ZOL to 50 µM, 71% viable cells were detected (p < 0.05). At a lower DOC concentration (0.01 ng/ml), this effect was also significant in combination with 50 µM ZOL but not with 25 µM ZOL (p = 0.08). The additional cytostatic effects from ZOL were low when combined with DOC in concentrations of 1 ng/ml and higher, and without significance (Figure 2B).
Figure 2. (A): At a DOC concentration of 1 ng/ml and a ZOL concentration of 25 µM, an additive cytostatic effect is obtained since the number of viable PC3 cells following this combined treatment is lower than with treatment with either ZOL or DOC alone. Error bars indicate standard deviations. (B): At a concentration of DOC of 0.1 ng/ml, addition of 25 µM or 50 µM ZOL causes a cytostatic effect on DU145 cells which significantly differs from treatment with either of these drugs alone and furthermore is synergistic. This effect is abundant also at a DOC concentration of 0.01 ng/ml in combination with ZOL 50 µM. Error bars indicate standard deviations.
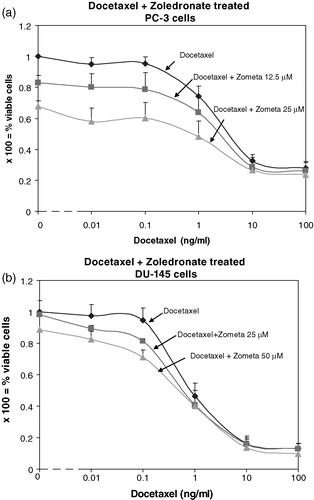
Identification of mitotic and apoptotic cells through changes in morphological features
Morphological studies of both cell lines following treatment with ZOL and DOC were performed using DAPI. Evaluation was done following treatment for 72 h, irrespective of treatment combination. For PC3 cells, a visual dose-dependent decrease of the number of mitoses was observed but, as for the combined treatment with DOC, no apoptotic cells were seen. The same pattern was observed for DU145 cells, as seen in A–3C. These figures show representative morphological features of DU145 cells following the most interesting treatment combination, i.e. that caused the most pronounced synergistic effect in the FMCA measurements. As seen in Figure 3A, mitotic cells are abundant following treatment with DOC at a concentration of 0.1 ng/ml. Following treatment with ZOL at a concentration of 50 µM, mitotic cells were also frequently represented (Figure 3B). When these two drugs were combined, however, the number of mitotic cells was reduced (Figure 3C) but apoptotic cells, as seen in the positive control (DOC 10 ng/ml), were still rare (Figure 3D).
Figure 3. (A) and (B): Representative examples of the morphological evaluation following DAPI preparation of the cells are shown. In (A), treatment with DOC (0.1 ng/ml) only is shown. The arrow indicates a mitotic cell; mitoses were abundant at this DOC concentration. Following single treatment with ZOL (50 µM), mitotic cells were also frequent (the arrow indicate such an example). The combined treatment (C) reduced the number of mitotic cells, an observation in accordance with decreased proliferation. Apoptotic cells, as seen in the positive control (DOC 10 ng/ml) (D), were, though, still rare (apoptotic cells indicated by arrows).
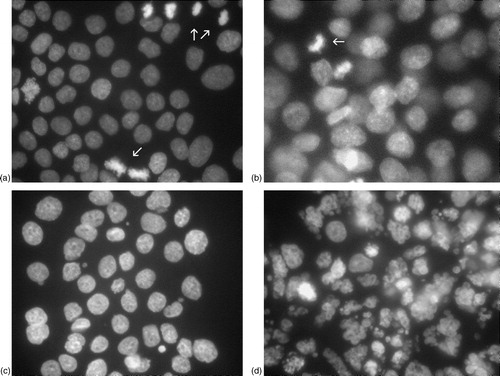
Discussion
More than 80% of men with advanced prostate cancer develop bone metastases and these are often associated with morbidity such as pain, pathological fractures, spinal cord compression, and other skeletal complications Citation[4], Citation[9], Citation[22]. As for breast cancer patients, there is increasing evidence that addition of BPs to standard therapy for patients with bone-metastasized hormone-refractory prostate cancer reduces the number of skeletal related events (SRE's) Citation[5], Citation[23]. The clinical utility of BPs relates to powerful inhibitory effects on various functional aspects of osteoclasts including generation and activity Citation[24–26] but also by shortening their life span by inducing apoptosis Citation[12]. As a consequence, bone resorption is decreased. A number of experimental studies, in vitro Citation[8], Citation[14–16] and in vivo Citation[16], indicate however, that clinical benefits exerted by BP treatment may be attributed to direct anti-tumoral effects. These effects on cancer cells may include reduced cell adhesion to non-mineralized bone matrices and cortical bone slices but also cytostatic effects Citation[8], Citation[14–16], Citation[27], Citation[28]. Furthermore BPs have been shown to act synergistically with other anti-neoplastic agents for breast cancer-and myeloma cell lines Citation[13], Citation[17], Citation[19].
In this study we have investigated the anti-tumoral properties of ZOL in terms of proliferation inhibition and apoptotic induction but also the potential of ZOL and DOC to exert super-additive anti-tumoral effects on two hormone-refractory prostate cancer cell lines; PC3 and DU145. The concentration range of ZOL was 6.25–100 µM and the incubation time 72 h, values that typically have been used by others Citation[14–16]. A dose-dependent decrease in the viable cell number was observed for both cell lines but the sensitivity differed markedly as 25 µM ZOL was capable of causing a significant reduction of the viable cell number for PC3 cells, but higher concentrations were required for DU145 cells. Morphological studies verified that the anti-tumoral effects can mainly be attributed to proliferation inhibition, as the number of mitotic cells visually decreased with increasing concentrations of ZOL. Only a minimal number of apoptotic cells were observed. Our data confirm the results by Lee et al. Citation[15], who found that the anti-tumoral effects of ZOL on PC3 cells were on the basis of cytostasis rather than induction of cell death. On the other hand, Corey et al. Citation[16] showed induction of apoptosis on PC3 cells following treatment with 68 µM ZOL. The percentage of apoptotic cells as determined by TUNEL assay was, however, low (1.8–4.1%) with the highest number on day 1 after treatment and the lowest on day 3 Citation[16]. These results are thus in line with our observations that only a sporadic fraction of the ZOL treated PC3 and DU145 cells show apoptotic features. Using a complementary technique to measure earlier events of apoptosis than DNA fragmentation, namely compromised cells, Corey et al., however, found higher absolute values of apoptotic cells Citation[16]. Moreover, Dumon et al. recently showed that dose of BPs and exposure time significantly influence the anti-tumoral effects exerted by BPs Citation[14]. With regard to dose dependency of the anti-tumoral effects exerted by BPs, our results are consistent with those of Dumon et al., as the viable cell number clearly decreases with increasing concentration of ZOL (see Figures 1A–1B).
The present study demonstrates for the first time additive and synergistic cytostatic effects on prostate cancer cells following treatment with ZOL and another anti-neoplastic agent, DOC. These effects were abundant for DU145 cells, as seen in Figure 2B. For example, combined treatment with DOC at a concentration of 0.1 ng/ml and ZOL at 50 µM caused a higher reduction of the viable cell number than expected, considering the results achieved using the drugs one by one. For the PC3 cells, which in general were found to be more sensitive to ZOL and less sensitive to DOC than DU145 cells, additive cytostatic effects were observed in the concentration range 12.5–25 µM and 0.01–1 ng/ml for ZOL and DOC respectively. Clearly, PC3 and DU145 cells respond differently to ZOL and to DOC but also to the combined regime, which may reflect the heterogeneity of prostate cancer cells. The concentration range of ZOL used in this study was 1–100 µM. Approximately 10-fold lower levels of BPs have been detected in serum during treatment of patients Citation[29–31]. However, it has been suggested that the effective local concentration of BPs at sites of active bone resorption is considerably higher than the serum levels, due to the affinity of BPs to bone mineral Citation[32]. Combined treatment regimes with potential to yield synergistic anti-tumoral effects without increasing the side effects correspondingly are tempting, especially for patients with lowered performance status but still in need of palliative therapy.
By increasing the concentration of ZOL in combined treatment for DU145 cells, it is possible that even more pronounced cytostatic effects can be achieved. Also, the sequence in which ZOL and DOC are administered may perhaps influence the anti-tumoral effects. Although the present study was not designed to elucidate the underlying mechanisms of the synergistic effects, such studies should be of particular interest. Theoretically, however, as discussed by Jagdev Citation[17], who found synergistic effects on breast cancer cells following treatment with ZOL and another taxan, paclitaxel, inhibition of the mevalonate pathway by ZOL and prevented chromosome segregation and cell division by a taxan may cause a cumulative apoptotic insult. Several placebo-controlled clinical trials, in various cancer types, have demonstrated benefits following BP treatment in terms of reduced number of SREs and pain reduction Citation[4], Citation[5], Citation[33], Citation[34]. Moreover, use of BPs in combination with chemotherapy has been shown to reduce the number of visceral metastases from breast cancer and to improve survival compared with those receiving standard antineoplastic treatment alone Citation[35]. Recently, Vordos et al. reported data from a pilot study of patients with hormone-refractory PC receiving combined DOC and ZOL treatment. A mean survival of at least 14 months was reported and the authors suggested a potential additive or synergistic effect on tumor cells following the combined treatment regime, although no mechanistic explanation was given Citation[36]. Our data, although preliminary, support this clinical observation on a cellular level but to what extent antitumoral effects of BPs in general have contributed to the clinical benefits observed so far still remains an open question.
In summary, our data support the hypothesis that ZOL exert anti-tumoral effects on prostate cancer cells. Furthermore we show for the first time that combined treatment with DOC causes additive and/or synergistic cytostatic effects in vitro. In vivo studies, both experimental and clinical trials, are warranted to evaluate the benefits of these observations.
The authors would like to acknowledge a Swedish Cancer Society Grant, King Gustav V's Jubilee Fund, and the Cancer Society in Stockholm.
References
- Falkmer U, Jarhult J, Wersall P, Cavallin-Stahl E. A systematic overview of radiation therapy effects in skeletal metastases. Acta Oncol 2003; 42: 620–33
- Roland S. Calcium studies in ten cases of osteoblastic prostatic metastases. J Urol 1958; 79: 339–42
- Clarke NW, McClure J, George NJ. Morphometric evidence for bone resorption and replacement in prostate cancer. Br J Urol 1991; 68: 74–80
- Saad F, Gleason DM, Murray R, Tchekmedyian S, Venner P, Lacombe L, et al. A randomized placebo-controlled trial of zoledronic acid in patients with hormone-refractory metastatic prostate carcinoma. J Natl Cancer Inst 2002; 94: 1458–68
- Saad F. Zoledronic acid significantly reduces pathologic fractures in patients with advanced-stage prostate cancer metastatic to bone. Clin Prostate Cancer 2002; 1: 145–152
- Shipman CM, Croucher PI, Russell RG, Helfrich MH, Rogers MJ. The bisphosphonate incadronate (YM175) causes apoptosis of human myeloma cells in vitro by inhibiting the mevalonate pathway. Cancer Res 1998; 58: 5294–5297
- Coxon FP, Benford HL, Russell RG, Rogers MJ. Protein synthesis is required for caspase activation and induction of apoptosis by bisphosphonate drugs. Mol Pharmacol 1998; 54: 631–8
- Oades GM, Senaratne SG, Clarke IA, Kirby RS, Colston KW. Nitrogen containing bisphosphonates induce apoptosis and inhibit the mevalonate pathway impairing Ras membrane localization in prostate cancer cells. J Urol 2003; 170: 246–52
- Aparicio A, Gardner A, Tu Y, Savage A, Berenson J, Lichtenstein A. In vitro cytoreductive effects on multiple myeloma cells induced by bisphosphonates. Leukemia 1998; 12: 220–9
- Shipman CM, Rogers MJ, Apperley J, Graham R, Russell G, Croucher PI. Anti-tumour activity of bisphosphonates in human myeloma cells. Leuk Lymphoma 1998; 32: 129–38
- Shipman CM, Rogers MJ, Vanderkerken K, Van Camp B, Graham R, Russell G, Croucher PI. Bisphosphonates––mechanisms of action in multiple myeloma. Acta Oncol 2000; 39: 829–35
- Fromigue O, Lagneaux L, Body JJ. Bisphosphonates induce breast cancer cell death in vitro. J Bone Miner Res 2000; 15: 2211–21
- Senaratne SG, Pirianov G, Mansi JL, Arnett TR, Colston KW. Bisphosphonates induce apoptosis in human breast cancer cell lines. Br J Cancer 2000; 82: 1459–68
- Dumon JC, Journe F, Kheddoumi N, Lagneaux L, Body JJ. Cytostatic and apoptotic effects of bisphosphonates on prostate cancer cells. Eur Urol 2004;45:521–8; discussion 528–9.
- Lee MV, Fong EM, Singer FR, Guenette RS. Bisphosphonate treatment inhibits the growth of prostate cancer cells. Cancer Res 2001; 61: 2602–8
- Corey E, Brown LG, Quinn JE, Poot M, Roudier MP, Higano CS, Vessella RL. Zoledronic acid exhibits inhibitory effects on osteoblastic and osteolytic metastases of prostate cancer. Clin Cancer Res 2003; 9: 295–306
- Jagdev SP, Coleman RE, Shipman CM, Rostami HA, Croucher PI. The bisphosphonate zoledronic acid induces apoptosis of breast cancer cells: evidence for synergy with paclitaxel. Br J Cancer 2001; 84: 1126–34
- Witters LM, Crispino J, Fraterrigo T, Green J, Lipton A. Effect of the combination of docetaxel zoledronic acid and a COX-2 inhibitor on the growth of human breast cancer cell lines. Am J Clin Oncol 2003; 26: S92–97
- Tassone P, Forciniti S, Galea E, Morrone G, Turco MC, Martinelli V, et al. Growth inhibition and synergistic induction of apoptosis by zoledronate and dexamethasone in human myeloma cell lines. Leukemia 2000; 14: 841–4
- Eisenberger MA, De Wit R, Berry W, et al A multicenter phase III comparison of docetaxel (D) + prednisone (P) and mitoxantrone (MTZ) + P in patients with hormone refractory prostate cancer(HRPC), ASCO Abstract 4 Genitourinary cancer category prostate cancer subcategory 2004.
- Larsson R, Kristensen J, Sandberg C, Nygren P. Laboratory determination of chemotherapeutic drug resistance in tumor cells from patients with leukemia using a fluorometric microculture cytotoxicity assay (FMCA). Int J Cancer 1992; 50: 177–85
- Carlin BI, Andriole GL. The natural history skeletal complications and management of bone metastases in patients with prostate carcinoma. Cancer 2000; 88: 2989–94
- Saad F, Gleason DM, Murray R, Tchekmedyian S, Venner P, Lacombe L, et al. Long-term efficacy of zoledronic acid for the prevention of skeletal complications in patients with metastatic hormone-refractory prostate cancer. J Natl Cancer Inst 2004; 96: 879–82
- Rogers MJ, Watts DJ, Russell RG. Overview of bisphosphonates. Cancer 1997; 80: 1652–60
- Rodan GA. Mechanisms of action of bisphosphonates. Annu Rev Pharmacol Toxicol 1998; 38: 375–88
- Flanagan AM, Chambers TJ. Inhibition of bone resorption by bisphosphonates: interactions between bisphosphonates osteoclasts and bone. Calcif Tissue Int 1991; 49: 407–15
- Van der Pluijm G, Vloedgraven H, van Beek E, van der Wee-Pals L, Lowik C, Papapoulos S. Bisphosphonates inhibit the adhesion of breast cancer cells to bone matrices in vitro. J Clin Invest 1996; 98: 698–705
- Boissier S, Magnetto S, Frappart L, Cuzin B, Ebetino FH, Delmas PD, Clezardin P. Bisphosphonates inhibit prostate and breast carcinoma cell adhesion to unmineralized and mineralized bone extracellular matrices. Cancer Res 1997; 57: 3890–4
- Berenson JR, Rosen L, Vescio R, Lau HS, Woo M, Sioufi A, et al. Pharmacokinetics of pamidronate disodium in patients with cancer with normal or impaired renal function. J Clin Pharmacol 1997; 37: 285–90
- Body JJ, Gaich GA, Scheele WH, Kulkarni PM, Miller PD, Peretz A, et al. A randomized double-blind trial to compare the efficacy of teriparatide [recombinant human parathyroid hormone (1–34)] with alendronate in postmenopausal women with osteoporosis. J Clin Endocrinol Metab 2002; 87: 4528–35
- Reid IR, Brown JP, Burckhardt P, Horowitz Z, Richardson P, Trechsel U, et al. Intravenous zoledronic acid in postmenopausal women with low bone mineral density. N Engl J Med 2002; 346: 653–61
- Sato M, Grasser W, Endo N, Akins R, Simmons H, Thompson DD, et al. Bisphosphonate action, alendronate localization in rat bone and effects on osteoclast ultrastructure. J Clin Invest 1991; 88: 2095–105
- O'Rourke N, McCloskey E, Houghton F, Huss H, Kanis JA. Double-blind placebo-controlled dose–response trial of oral clodronate in patients with bone metastases. J Clin Oncol 1995; 13: 929–34
- Hortobagyi GN, Theriault RL, Porter L, Blayney D, Lipton A, Sinoff C, et al. Efficacy of pamidronate in reducing skeletal complications in patients with breast cancer and lytic bone metastases. Protocol 19 Aredia Breast Cancer Study Group. N Engl J Med 1996; 335: 1785–91
- Diel IJ, Solomayer EF, Costa SD, Gollan C, Goerner R, Wallwiener D, et al. Reduction in new metastases in breast cancer with adjuvant clodronate treatment. N Engl J Med 1998; 339: 57–63
- Vordos D, Paule B, Vacherot F, Allory Y, Salomon L, Hoznek AY, et al. Docetaxel and zoledronic acid in patients with metastatic hormone-refractory prostate cancer. BJU Int 2004; 94: 524–7