Abstract
The aim of this study was to use magnetic resonance (MR) techniques to non-invasively compare the effects of the three leading vascular disrupting agents, namely combretastatin A-4 disodium phosphate (CA4DP), 5,6-dimethylxanthenone-4-acetic acid (DMXAA) and ZD6126. A C3H mouse mammary carcinoma grown in the right rear foot of female CDF1 mice was used and treatments performed when tumours had reached 200 mm3 in volume. Drugs were prepared fresh before each experiment and intraperitoneally injected into restrained non-anaesthetised mice. Tumour response was evaluated using 31P-MR spectroscopy and T1- and T2- weighted imaging with a 7-Tesla, horizontal bore magnet, before and up to 24 hours after treatment. All three drugs significantly decreased bioenergetic status and pH, and did so in a time and dose dependent fashion, but there were differences; the decrease by CA4DP occurred more rapidly than for DMXAA or ZD6126, while DMXAA had a narrow window of activity compared to CA4DP and ZD6126. Changes in T1 weighted images for all three agents suggested a dose dependent increase in tumour oedema within three hours after treatment, consistent with an increase in vessel permeability. Using T2 weighted images there was some evidence of haemaorrhagic necrosis by DMXAA, but such necrosis was limited following treatment with CA4DP or ZD6126.
It is now well established that the viability and continued growth of solid tumours depends critically on them developing their own blood supply, which they do from the pre-existing normal tissue vessels by the process of angiogenesis Citation[1]. The neovasculature that is formed is generally very different from the vessels from which it develops Citation[2]. It is primitive in nature, morphologically and functionally abnormal, and is unable to support the rapidly growing tumour cell mass. Thus, the vasculature becomes inadequate in meeting the nutrient and oxygen requirements of the tumour cells. This creates a microenviroment within the tumour that is nutrient deprived, oxygen deficient and highly acidic Citation[2], and the cells that exist in these adverse microenvironmental conditions have a significant impact on the response of that tumour to therapy, as well as influencing both the growth of the primary tumour and its metastatic spread Citation[3].
The importance of the tumour vasculature makes it an attractive target for therapy and substantial interest has been made in trying to develop treatments that specifically target this vasculature Citation[4]. One approach is to inhibit the angiogenic process itself Citation[5]. Strategies that have been tested include the use of drugs that interfere with the delivery or export of angiogenic stimuli, antibodies to inhibit or inactivate angiogenic factors after their release, drugs which inhibit receptor action, inhibitors of invasion, and agents that inhibit endothelial cell proliferation Citation[4]. Many of these approaches are currently in clinical testing Citation[6]. An alternative approach has involved the use of treatments that preferentially destroy the already established tumour vessels Citation[7]. Despite encouraging pre-clinical studies showing the potential of this latter approach, early attempts to achieve the same in humans were relatively unsuccessful and this was generally attributed to toxicity or lack of efficacy at the doses achievable in humans Citation[8]. Despite this a number of new drug-based vascular disrupting agents (VDAs) have been developed which are believed to be more efficient and less toxic and several of them are currently in clinical trials Citation[9].
Three of the leading VDAs that at present are under clinical investigation are combretastatin A-4 disodium phosphate (CA4DP), the colchicine analogue ZD6126 and the flavone acetic acid (FAA) derivative 5,6-dimethylxanthenone-4-acetic acid (DMXAA). CA4DP is a drug that was originally isolated from the African Bush Willow Combretum caffrum Citation[10]. It is a tubulin-binding agent that disrupts the cytoskeleton of dividing endothelial cells and as a result will induce vascular damage in a variety of animal solid tumours and human tumour xenografts Citation[11]. Unlike other VDAs that had previously been studied, CA4DP was found to induce vascular damage at doses that were far below those that are maximally tolerated Citation[12], Citation[13]. Phase I clinical testing of this agent has now been completed and phase II studies are in progress Citation[9]. ZD6126 is an N-acetylcochinol-O-phosphate derivative of colchicine that has recently been developed Citation[14], which from preliminary studies is also known to disrupt tubulin Citation[14] and thus induce vascular damage at well-tolerated doses in a range of tumour models Citation[15–17]. DMXAA is a more potent fused tricyclic analogue of FAA that is believed to cause vascular damage through a variety of mechanisms that again primarily target endothelial cells Citation[11], Citation[18]. As such, DMXAA has been shown to cause substantial vascular damage in a variety of murine tumours and human tumour xenografts Citation[11]. Unlike the tubulin-binding agents these effects with DMXAA tend to occur at doses that are close to the maximum tolerated, thus this drug has a narrow window of activity Citation[19–21]. Nevertheless it has still undergone preliminary clinical investigation Citation[9].
The aim of the study was to apply magnetic resonance spectroscopy and imaging for a non-invasive comparative evaluation of the vascular damaging effects of the three leading VDAs currently undergoing clinical testing. These studies were performed in our well-established C3H mouse mammary carcinoma in which all these agents have been shown to induce vascular damage Citation[13], Citation[17], Citation[22]. Primarily the study involved a comparison between spectroscopy changes using the biomarkers of 31P bioenergetics (ratio of the β-nucleoside triphosphate and inorganic phosphate peaks; β-NTP/Pi) and pH. In addition, T1 and T2-weighted images were studied to see if these approaches could be used to observe the anti-vascular effects. Preliminary studies in this tumour model using a single dose of one VDA (100 mg/kg CA4DP) have previously been presented Citation[23].
Materials and methods
Animal and tumour model
A C3H mouse mammary carcinoma grown in the right rear foot of 10–14 week-old female CDF1 mice was used in all experiments. This is a moderately differentiated carcinoma, which originally occurred spontaneously in our laboratory animals, and its derivation and maintenance has been described previously Citation[24]. New tumour material was taken from frozen stock every three months and transplanted on the flanks of host mice. To produce experimental tumours large flank tumours were excised, finely minced with scissors and 5–10 µl subcutaneously injected in the foot. Treatments were carried out when the tumours had reached about 200 mm3 in size, which generally occurred within 12–24 days after implantation. Tumour volume was estimated from the formula: D1×D2×D3×π/6, where the D values represent the three orthogonal diameters. All experiments were performed under National and European Union approved guidelines for animal welfare.
Drug preparation
All drugs were prepared fresh before each experiment. They were stored cold (4°C), protected from light, and used within one week. CA4DP was supplied by Oxigene Inc. (Boston, MA), DMXAA was obtained from Professor William Denny at the University of Auckland (New Zealand), and ZD6126 from Angiogene Pharmaceuticals (Aston Rowant, UK). They were all dissolved in saline (0.9% NaCl) and intraperitoneally (i.p.) injected at a constant volume of 0.02 ml/g mouse body weight. FAA was used as a positive control in our studies and was a gift from Lyonnaise Industrielle Pharmaceutique (LIPHA, Lyon, France). It was prepared by being dissolved in a 1% Na2CO3 solution and also i.p. injected at 0.02 ml/g. In our CDF1 mice the maximum tolerated dose (the maximum drug dose administered at which no animal deaths were observed) for each drug was found to be >400 mg/kg Citation[13], 25 mg/kg Citation[21], >300 mg/kg Citation[17] and 150 mg/kg Citation[25] for CA4DP, DMXAA, ZD6126 and FAA, respectively.
Experimental set-up
Non-anaesthetised tumour bearing mice were transferred to specially constructed lucite jigs which restrained individual animals, but allowed the tumour bearing legs to be exposed. This leg was then loosely attached to the jig with tape, without impairing blood supply to the foot. An i.p. line was then inserted into the animal and the restraining jig then attached to a plate which maintained the tumour beneath a 9-mm diameter, two-turn surface coil, which served as both the transmitter and the receiver. The whole set-up was then placed in an orthogonal position in the isocenter of a 7 Tesla, horizontal bore magnet (Varian, Palo Alto, CA). Temperature was maintained at about 37°C in the magnet tunnel using loops of tubing through which heated water was circulated. Mice were examined prior to and up to three hours after drug injection, without disturbing the position of the tumour in the magnet. Animals were returned to the magnet and re-examined at 24 hours after treatment.
MR spectroscopy
31P MR spectroscopy (pulse-acquire sequence using a 500 microsec sincos pulse; TR = 6000 millisecs; 160 excitations) was performed before treatment and in a consecutive manner after drug injection, thereby giving rise to a spectra every 16 minutes. Measurements included the entire tumour. It has been shown in this C3H mammary carcinoma Citation[26] that there is very little contamination of the tumour 31P spectrum from neighbouring tissues, with the exception of a small signal from phosphocreatine. Phosphorus spectra were obtained at 121.424 MHz with a spectral width of 8000 Hz. The endpoint selected to disclose changes in the bioenergetic status was the ratio of the peaks of inorganic phosphate (Pi) to β-nucleoside triphosphate (β-NTP). Estimation of intracellular pH was derived from changes in the chemical shift between β-NTP and Pi. Data analysis of 31P MR spectroscopy involved the VAPRO time domain fitting method Citation[27] using the MRUI analysis package running under Matlab (The Mathworks Inc, Natick, MA).
MR imaging
T1 weighted MR images were obtained with a slice thickness of 1 mm and in-plane resolution of 0.08 mm×0.08 mm (TR = 200 millisecs; TE = 20 millisecs). The images were analysed according to the disappearance of “hot spots”, seen as hyper-intense regions (e.g., white streaks on the images). These “spots” represent blood flowing into the area, thereby giving an estimation of the vascular shutdown. The biomarker for this test was a reduction of at least 20% in the number of hot spots. In every series, the pretreatment images were compared to the images obtained three hours after treatment. T2-weighted images were obtained with a slice thickness of 1 mm and in-plane resolution of 0.08 mm×0.16 mm (TR = 2000 millisecs; TE = 200 millisecs). Two approaches were employed to evaluate the magnitude of any changes in image intensity. The first involved estimating the median signal intensity in the tumour region, while the second was the percentage of voxels having an intensity of less than half the mean intensity of the entire tumour, the latter giving a crude measure of the proportion of relatively dark voxels and potentially the extent of hemorrhagic necrosis. For both methods a comparison was made between the pre-treatment values and those obtained 3 and 24 hours after treatment.
Statistical analysis
All data was statistically analysed using either a Student's t-test after testing for variance homogeneity using an F-test, or using a Wilcoxon signed-rank test. For either test a significance level of p < 0.05 was selected.
Results
Representative 31P MR spectra obtained in our C3H mouse mammary carcinoma are shown in . They are for measurements made in the same tumour for a 16 minute period before and 48–64 minutes after injection of 200 mg/kg ZD6126, and clearly illustrate not only the good quality of the spectra, but also the increase in the Pi peak after drug treatment. The relative changes in the ratio of β-NTP/Pi and subsequent changes in pH obtained in tumours as a function of time after treating mice with either saline or when using FAA (150 mg/kg) as a positive control, are shown in . For saline there was no significant change (Student's t-test; p < 0.05) in either the β-NTP/Pi ratio or pH over the 24 hour measurement period. The non-significant increase in the β-NTP/Pi ratio at 24 hours probably reflects the difficulty in re-positioning the tumour in the surface coil. With FAA, the β-NTP/Pi ratio decreased as a function of time after treatment, becoming significant by two hours and resulting in a maximum 40–60% reduction at three hours that was generally maintained for at least 24 hours. There was also a time dependent decrease in tumour pH from a starting value of 7.33, which again became significant two hours after FAA injection and was even further decreased after three hours. By 24 hours pH had fully recovered.
Figure 1. Representative 31P-MR spectra obtained in a C3H mouse mammary carcinoma. Measurements were made using a 7-Tesla magnet over a 16-minute period prior to injection of ZD6126 (200 mg/kg) and 48-64 minutes after administration. Labels show the inorganic phosphate (Pi) and β-nucleoside triphosphate (β-NTP) peaks.
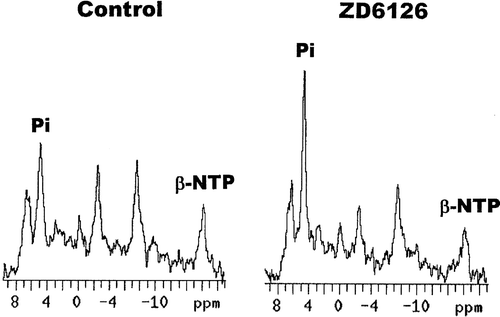
Figure 2. Drug induced changes in the β-NTP/Pi ratio or pH in a C3H mouse mammary carcinoma. Measurements were made prior to (0 hours) and up to 24-hours after treatment with either saline (○) or 150 mg/kg FAA (•). The β-NTP/Pi results are expressed relative to the pre-treatment values, while for pH the actual values are shown. All points represent the means (±1 S.E.) for 5-6 mice.
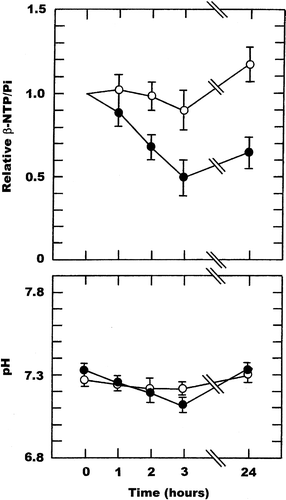
The effects of DMXAA, CA4DP and ZD6126 on the βNTP/Pi ratio and pH are shown in . For the FAA analogue, DMXAA, there was a clear time and drug-dose dependency. At a dose of 5 mg/kg there was no significant change in βNTP/Pi or pH, although for the latter a non-significant increase was seen at 24 hours. At a higher dose of 15 mg/kg, both βNTP/Pi and pH decreased. These decreases increased with time reaching significance by three hours, with the relative βNTP/Pi decreasing to 0.57 and pH going from a pre-treatment value of 7.39 to 7.17. By 24 hours both βNTP/Pi and pH had recovered. With the highest dose of 25 mg/kg a similar time dependent decrease was observed, but the changes in both parameters became significant earlier (at two hours) and were more pronounced. No values were obtained at 24 hours due to all the animals dying within that period.
Figure 3. Drug induced changes in the β-NTP/Pi ratio or pH in a C3H mouse mammary carcinoma. Measurements were made prior to (0 hours) and up to 24-hours after treatment with DMXAA given at doses of 5 (○), 15 (▵), or 25 (•) mg/kg. The β-NTP/Pi results are expressed relative to the pre-treatment values, while for pH the actual values are shown. All points represent the means (±1 S.E.) for 5-6 mice.
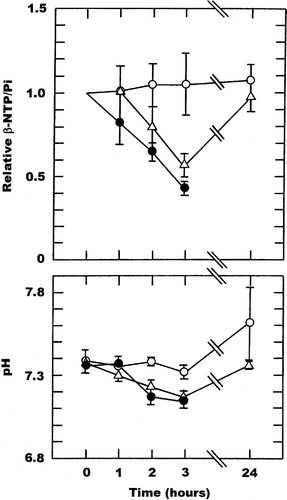
Figure 4. Drug induced changes in the β-NTP/Pi ratio or pH in a C3H mouse mammary carcinoma. Measurements were made prior to (0 hours) and up to 24-hours after treatment with CA4DP given at doses of 50 (○), 100 (▵), or 250 (•) mg/kg. The β-NTP/Pi results are expressed relative to the pre-treatment values, while for pH the actual values are shown. All points represent the means (±1 S.E.) for 4-7 mice.
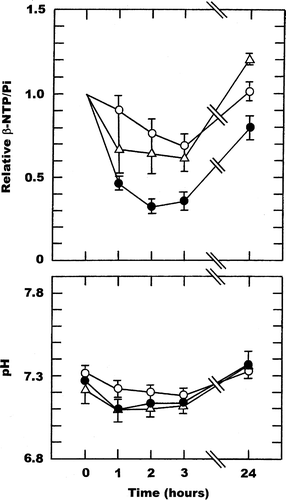
Figure 5. Drug induced changes in the β-NTP/Pi ratio or pH in a C3H mouse mammary carcinoma. Measurements were made prior to (0 hours) and up to 24-hours after treatment with ZD6126 given at doses of 100 (○) or 200 (•) mg/kg. The β-NTP/Pi results are expressed relative to the pre-treatment values, while for pH the actual values are shown. All points represent the means (±1 S.E.) for 4 mice.
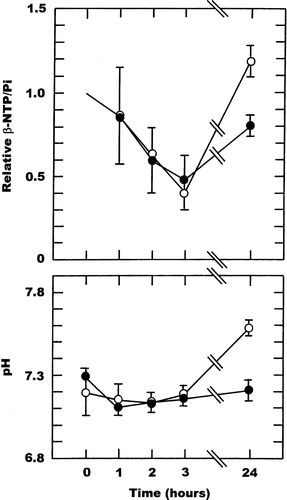
With CA4DP there were similar time and drug-dose dependent changes. At the lowest CA4DP dose of 50 mg/kg there was a slow decrease in βNTP/Pi over time reaching a significant 32% decrease by three hours, with full recovery at 24 hours. Tumour pH also decreased, reaching a maximum decrease of 0.15 units during the three hours following drug injection, and again had fully recovered by 24 hours. Using higher CA4DP doses of 100 and 250 mg/kg similar time course changes in βNTP/Pi and pH were obtained. For βNTP/Pi the degree of change was dose dependent, being 39% and 49% at three hours after giving 100 and 250 mg/kg, respectively. However, for tumour pH no drug dependency was apparent with the 100 and 250 mg/kg doses showing decreases that were of the same order as seen with the lower 50 mg/kg dose. Full recovery was also found by 24 hours.
ZD6126 also showed a time dependent decrease in β-NTP/Pi, but there was no apparent dose dependency, such that the decrease seen at three hours was 59% and 51% using doses of 100 and 200 mg/kg, respectively. Both of these changes were just under the significance level, which probably reflects the low numbers of animals. By 24 hours there was complete recovery at the 100 mg/kg dose and almost complete recovery with the 200 mg/kg dose. Interestingly, there was no significant change in tumour pH over the first three hours after treatment with 100 mg/kg and by 24 hours it had actually resulted in a significant increase. Whereas for the 200 mg/kg dose pH showed a substantial and significant decrease after one hour, after which time there was a slow recovery, although even by 24 hours it was still 0.08 units below the average starting value.
Representative T1 and T2-weighted images are shown in . In the pre-treatment T1-weighted image there are high intensity “hot-spots” which in general (as in this example) are mostly near the periphery, and three hours after treatment with 200 mg/kg ZD6126 they have disappeared. For the T2-weighted image there is a suggestion of an increased proportion of dark areas 24 hours after giving this same ZD6126 dose. Analysis of these T1 and T2-weighted images and those for all the other treatments used in this study are summarised in –. For the T1- weighted results the images recorded before drug administration are compared to those recorded after three hours (). Not all the mice in each treatment group were analysable for “hot spots” (i.e., no hot spot was seen prior to treatment), but for those that were the responders represented those tumours that showed a decrease of 20% or more in the number of hyper-intense regions. In the saline controls no responders were found, while in the FAA positive controls all the animals responded. For DMXAA, CA4DP and ZD6126, a general dose response relationship was observed with all the drugs, in that the higher the dose the greater the percentage of responders.
Figure 6. Representative changes in the T1 (upper panels) and T2 (lower panels) weighted images induced by ZD6126 (200 mg/kg) in a C3H mouse mammary carcinoma. For each set of images measurements were made before (left panels), or 3 (T1) and 24 (T2) hours after drug injection (right panels). The T1 weighted images show the “hot spots” which are lost after treatment, while the T2 weighted images show the increased portion of dark areas.
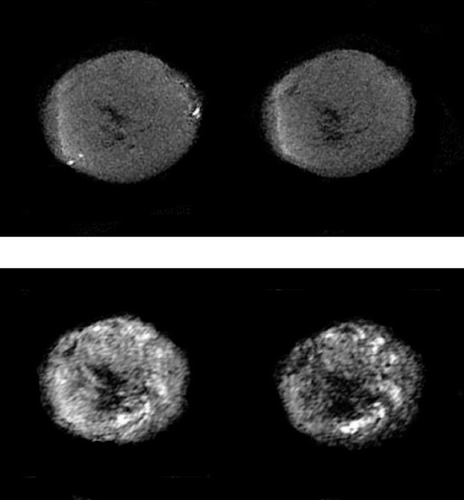
Table I. T1 weighted images: “hot spots”
The results of the T2-weighted image analyses are shown as either the percentage of dark pixels () or mean signal intensity (). With saline, absolutely no changes were seen at three and 24 hours using either parameter. Following treatment with FAA the percentage of dark pixels significantly increased at three hours and a slightly larger effect was seen after 24 hours, although this was not significant due to the larger variability. For the mean signal intensity estimates there were significant decreases at both three and 24 hours, with the latter time point showing the largest change. After treatment with the three VDAs the only significant change in percentage of dark pixels that was observed was an increase 24 hours following injection with the highest dose of ZD6126 (200 mg/kg). However, while little or no change was seen with any of the agents at three hours, at 24 hours there were clearly larger increases with a trend towards a dose response relationship. Using the mean signal intensity biomarker, significant decreases were found at three hours after treatment with 5 and 15 mg/kg DMXAA, but not at the highest 25 mg/kg dose. By 24 hours full recovery was apparent with all doses. With CA4DP decreases were seen at both time points with each dose, but only three hours after giving 100 mg/kg were these changes significant. For ZD6126, the greatest decreases were obtained at 24 hours after drug treatment, but again these changes were not significant.
Table II. T2 weighted images: percentage of dark pixels
Table III. T2 weighted images: mean signal intensity in tumour ROI
Discussion
The idea of preferentially damaging tumour vasculature as a therapeutic approach in not a new concept, but until recently no effective agents were available. Now a number of small molecule drugs have been identified that can produce substantial vascular damage in a variety of animal tumours without inducing corresponding effects in normal tissues Citation[9]. The lead compounds are CA4DP, ZD6126 and DMXAA and all three agents have moved into clinical testing Citation[9]. Although the vascular damage induced by VDAs in preclinical models can be extensive, the resulting effect on tumour growth has always been modest Citation[13], Citation[20], Citation[25], Citation[28], Citation[29]. This is because treatment with VDAs generally leaves a viable rim of cells from which the tumour can re-grow and it is for this reason that for the full clinical potential of VDAs to be realised they will need to be combined with more conventional therapies that target this outer rim Citation[9]. It also meant that in the early clinical trials where the VDAs were used as solitary agents, conventional tumour response endpoints were unlikely to show any effect. Thus, in those clinical studies dynamic contrast enhanced MRI was used as a non-invasive “biomarker” for activity Citation[30], Citation[31], since animal studies demonstrate this approach to be useful in detecting VDA induced changes Citation[32–36]. In the current study we have used MR approaches that do not require the administration of a contrast agent and so repeated measurements are possible, to further understand the interaction between these drugs and the tumour vasculature and to compare the time and dose dependency of these effects.
Using 31P-MRS we were able to demonstrate a drug-induced decrease in the bioenergetic status of a C3H mouse mammary carcinoma using all agents. For DMXAA no change was found with the lowest dose of 5 mg/kg, but at the higher doses a dose dependency was seen, with these decreases becoming greater as the time interval after treatment increased from 1–3 hours. These results are entirely consistent with perfusion data obtained using the RbCl uptake technique in the same tumour model Citation[21]. The time course response was also identical to that seen with the DMXAA structural analogue, FAA, used as a positive control and this too was consistent with our previous RbCl uptake data Citation[25]. By 24 hours the tumours treated with the 15 mg/kg dose had recovered. Exactly what happened at this time after the larger 25 mg/kg dose was not determined due to some animal lethality. But, RbCl data obtained with this 25 mg/kg dose of DMXAA showed that perfusion was still reduced at 24 hours Citation[21], so we would have expected the β-NTP/Pi ratio to also remain down, probably similar to what was seen with FAA at 24 hours. Using a slightly higher DMXAA dose of 27.5 mg/kg a decrease in bioenergetic status was also reported in two human colon adenocarcinomas, the HT29 and the LS1747 Citation[35]. Although there seemed to be differences in the degree of change observed in these two models, for both the effect was found to continually increase with time up to 180 minutes after treatment, exactly as was seen in our study.
The effect of CA4DP on bioenergetic status was also investigated in those two human colon adenocarcinoma models Citation[35]. It was found that a dose of 100 mg/kg had absolutely no influence on bioenergetic status in the HT29 tumour, but in the LS1747 an effect was seen that was actually identical to the DMXAA result, namely a slow continuous reduction. Other studies by this group have reported similar variable effects in different tumours, but in each case the change in bioenergetic status was slow and continuous up to the maximum time interval examined of 180 minutes Citation[34]. In our study, it was found that the decrease in β-NTP/Pi by CA4DP occurred more rapidly than with DMXAA; the nadir being reached within 1–2 hours. This again is consistent with the more rapid changes in perfusion observed with the RbCl uptake method in this C3H mouse mammary carcinoma Citation[21]. Indeed, studies by others using a variety of procedures for measuring the vascular effects of VDAs also support our findings that the CA4DP-induced changes occur more rapidly than those seen with DMXAA Citation[12], Citation[19], Citation[29], Citation[32]. These differences most likely reflect the different mechanisms by which vascular shut down occurs; direct tubulin binding for CA4DP and indirect effects of DMXAA through the induction of TNF Citation[11]. However, while they can help explain the obvious differences in our results, these different mechanisms are unlikely to account for the similarities seen for CA4DP and DMXAA in the study by Beauregard and colleagues Citation[35]. What is perhaps more surprising is the result with ZD6126 where the time dependent decrease in β-NTP/Pi resembles more closely DMXAA than CA4DP. Like CA4DP, ZD6126 is a tubulin binding agent and induces a rapid reduction in perfusion Citation[17]. However, unlike CA4DP in which perfusion recovers within 24 hours Citation[21] for ZD6126 this recovery is far more rapid and is complete within six hours Citation[17]. Thus, although both CA4DP and ZD6126 induce vascular shut down through a tubulin binding mechanism, the subsequent effects are clearly different and our estimates of bioenergetic status may be giving us clues to these differences.
One other aspect clearly shown by our 31P-MRS data is that for DMXAA there exist a narrow therapeutic window, with activity only seen when one goes above a dose of 5 mg/kg, yet at the 25 mg/kg dose substantial toxicity occurs. This has been reported previously by us using other biomarkers in this C3H mouse mammary carcinoma Citation[21], Citation[22], Citation[37], and by others using similar biomarkers Citation[19], Citation[20], as well as 31P-MRS Citation[38]. This is not the same for the tubulin-binding agents where the therapeutic window is much larger and substantial effects are seen at doses that are well below the maximum tolerated doses Citation[13], Citation[17], Citation[21], Citation[29].
In an earlier study in this C3H mammary carcinoma we attempted to demonstrate a relationship between bioenergetic status and hypoxia induced by a variety of treatments designed to reduce oxygen availability Citation[39]. It was found that bioenergetic status was a poor indicator of hypoxia, unless that hypoxia was induced by a decrease in tumour perfusion and only then if that reduced perfusion was in the order of around 75%. Comparing our current bioenergetic data with our previous estimates of tumour perfusion following treatment with the VDAs Citation[17], Citation[21], it appears that significant changes in bioenergetic status can be observed when perfusion is decreased by only 25–50%. What this may suggest is that the perfusion reductions by VDAs are not global changes, but rather reflect substantial reductions in small areas, with probably little or no changes in others. Thus, the use of 31P-MRS may be a useful approach for continuously monitoring such small, yet significant, changes induced by VDAs, both in pre-clinical and even clinical studies.
The effect of the three VDAs on tumour pH was also investigated. In general, the pH results followed the estimates of bioenergetic status, except for ZD6126 (200 mg/kg) where significant decreases in tumour pH were seen in the first hour after treatment despite their being no significant decrease in the β-NTP/Pi ratio. Apart from our earlier study with 100 mg/kg CA4DP Citation[23], only one other study has reported on the effects of VDAs on tumour pH Citation[40]. That was also performed using a 100 mg/kg dose of CA4DP and showed a significant pH decrease in the sarcoma F tumour within 30 minutes after treatment and some recovery by 150 minutes, a result that is not inconsistent with our findings.
These VDA induced changes in tumour pH have important implications, especially in situations where the VDAs are combined with other therapies in which pH plays an important role. There is evidence that under conditions of reduced pH tumour cells can be more sensitive to the cytotoxic action of certain chemotherapeutic drugs Citation[41] and hyperthermia Citation[42]. All three of the VDAs we studied have been combined with other forms of chemotherapy in preclinical studies and improved tumour responses seen, although this improvement in most cases may not have been greater than a simple additive effect of the VDA and cytotoxic drug Citation[43]. However, most of these drugs do not show any pH dependent activity. This is not the case for melphalan in which low pH is known to increase efficacy both in vitro and in vivo Citation[44] and using DMXAA the anti-tumour activity of melphalan was significantly enhanced in a mouse mammary tumour Citation[45]. Our data suggests that addition combinations of VDAs with other more pH sensitive agents (i.e., chlorambucil) should be considered. Hyperthermia is another therapy where substantial enhancements of tumour response have been reported after combination with VDAs (for review see Citation[8]) and again this clearly supports the concept of combining VDAs and pH sensitive therapies. This importance of VDA induced changes in tumour pH clearly needs further investigation specifically using MR based approaches.
The presence of hyper-intense regions (“hot spots”) in T1 weighted images probably reflects flow of blood within relatively large vessels into the image slice. This is supported by the observation that the hot spots are eliminated by pre-saturation of the adjacent thick slice (data not shown). Our analysis of the T1 weighted images showed a loss of “hot spots” after treatment with all three VDAs. Previous studies in another mouse mammary tumour, the T50/80, demonstrated an increase in both 1H T1 and T2 values at 4.7-Tesla 24 hours after treatment with photodynamic therapy Citation[46], with by far the greatest increase found in oedematous skin rather than the tumour itself. Such oedema is most likely to result from a drug-induced increase in vessel permeability. Indeed, additional studies have now shown that VDAs can cause such permeability changes Citation[34], Citation[47], Citation[48] and it has been suggested that an acute increase in vascular permeability would lead to oedema, causing an increase in interstitial fluid pressure, and resulting in vascular collapse Citation[11]. Although similar changes were anticipated in the present study, the impact of in-flow effects means that it is difficult to interpret our T1-weighted images in terms of the contribution from oedema. What is perhaps more surprising is that some 60% of tumours showed a response to DMXAA at a dose of 5 mg/kg, yet this dose has absolutely no effect on tumour perfusion Citation[21], bioenergetic status or pH (). Thus, a dose that is generally considered “inactive” in this tumour does have some effect on tumour vessels, but these changes in permeability are not sufficient to cause subsequent vessel collapse. This also suggests that adding some additional treatment to accentuate the effect of this 5 mg/kg dose on vessel permeability may make it an effective treatment and thus ultimately increase the therapeutic window for DMXAA which clearly would increase the clinical potential. In one study, DMXAA was combined with 5-HT and it was found that this latter agent not only enhanced the action of DMXAA, but DMXAA doses that had absolutely no activity became very effective Citation[20]. Whether this enhancement by 5-HT was the result of an increase in tumour vessel permeability or some other mechanism is not known.
Decreases in T2 weighted images are believed to be indicative of the development of haemorraghic necrosis, and several studies have shown that VDAs can not only induce haemorrhagic necrosis Citation[12], Citation[19], Citation[20], Citation[29], but that these changes can be detected with MRI approaches Citation[49]. Although T2-weighted MRI has not been shown to be a consistent indicator of haemorrhagic necrosis, Carano et al. Citation[50] identified low-T2 (but relatively high apparent diffusion coefficient) necrotic regions, assumed to contain paramagnetic molecules (deoxyhaemoglobin or methaemoglobin). Using our positive control drug, FAA, there were significant changes in both the percentage of dark pixels and mean signal intensity, but for its structural analogue, DMXAA, the only significant change was seen with mean signal intensity at three hours and only at the lower doses of 5 and 15 mg/kg, not the highest 25 mg/kg dose. Why no changes were seen at 24 hours in not clear. Additional histological studies on the induction of necrosis by DMXAA in this C3H mammary carcinoma clearly show a substantial increase by 24 hours after treatment Citation[22]. How quickly this necrosis develops in not known. With DMXAA it has been suggested that it takes several hours before any effect can be detected Citation[29]. This is certainly true for CA4DP, in which absolutely no increase in necrotic fraction was seen in this tumour model three hours after treatment, but this had significantly increased by 24 hours Citation[23]. There are two important factors that may explain the general lack of any significant effects seen with CA4DP in our C3H mouse mammary carcinoma. Firstly, the necrosis seen after treatment with CA4DP is typically not that large, with the necrotic fraction increasing with drug dose, but even at a dose of 400 mg/kg it is only increases from a control value of around 9 to 44% Citation[13], and secondly, the necrosis that does occur is typically not heamorrhagic in nature Citation[13]. ZD6126 also increases the necrotic fraction is this C3H mammary carcinoma Citation[17], but again even at the highest dose of 200 mg/kg that was tested, this necrosis only increased from a control value of 10% to 25% by 24 hours and again was not heamorrhagic Citation[17]. Of course, it is possible that any drug-induced decrease in signal intensity in the T2-weighted images may have been offset by possible T2 increases due to oedema.
Conclusion
Using the three most clinically relevant VDAs we were able to show changes in bioenergetic status of a C3H mouse mammary carcinoma that were different between the drugs, but were entirely consistent with data in this same tumour obtained using more invasive procedures. This demonstrates that 31P-MRS is an excellent method for monitoring the effects of VDAs. More importantly, it allows for measurements without the need for administration of a contrast agent, and thus continuous assessment is possible. By studying bioenergetics we are also actually looking at the response of the tumour cells following VDA treatment, and although the principal target of VDAs is the vasculature it is the effect this has on the tumour cells that ultimately influences outcome. It is now well established that despite the substantial vascular shutdown induced by VDAs and the subsequent ischemia, cell death and necrosis that develop, the use of VDAs as solitary agents in cancer therapy is limited Citation[4], Citation[9]. For their full clinical potential to be realised they need to be combined with more conventional therapies Citation[4], Citation[9]. Obtaining data that will aid in the selection of appropriate modalities to combine with the VDAs is important and again the fact that we can use the 31P-MRS data to observe drug induced changes in tumour pH is another potential benefit of this approach.
Using T1 and T2 weighted images we were also able to demonstrate drug-induced effects, but there were obvious inconsistencies. The T1 data suggested the induction of oedema as early as three hours after treatment, consistent with an increase in vessel permeability, suggesting that this is one of the earlier vascular changes occurring after VDA treatment and may be a precursor to the later vascular shutdown. Using this simple and easy method it was also possible to detect changes at drug doses that are not detected by other methods. With the T2 data it was possible to show changes using a VDA that can induce substantial necrosis that is haemorrhagic in nature, but for VDAs that induce limited, non-haemorrhagic necrosis its use may be limited. A combination of T2* MRI (sensitive to haemorrhagic effects) and diffusion weighted MRI (sensitive to increased interstitial space) is likely to provide a better approach to identify the contribution of haemorrhagic necrosis and oedema Citation[50]. This has been shown both pre-clinically Citation[49], Citation[51] and clinically Citation[52], but clearly this is an area that needs further investigation.
The authors would like to thank Mrs. Inger Marie Horsman and Ms. Dorthe Grand for excellent technical assistance. Financial support was obtained from the Danish Cancer Society.
References
- Folkman J. Role of angiogenesis in tumor growth and metastasis. Semin Oncol 2002; 29(Suppl 16)15–8
- Vaupel P, Kallinowski F, Okunieff P. Blood flow, oxygen and nutrient supply, and metabolic microenvironment of human tumors: A review. Cancer Res 1989; 49: 6449–65
- Horsman MR, Overgaard J. The oxygen effect and the tumour microenvironment. Basic Clinical Radiobiology for Radiation Oncologists, GG Steel. Edward Arnold, London 2002; 158–68
- Siemann DW, Warrington KH, Horsman MR. Targeting tumor blood vessels; an adjuvant strategy for radiation therapy. Radiother Oncol 2000; 57: 5–12
- Folkman J. Angiogenesis inhibitors: A new class of drugs. Cancer Biol Ther 2003; 2(4 Suppl 1)S127–33
- National Cancer Institute Website ( www.cancer.gov/clinicaltrials).
- Denekamp J. Vascular attack as a therapeutic strategy for cancer. Cancer Met Rev 1990; 9: 267–82
- Horsman MR, Murata R. Combination of vascular targeting agents with thermal or radiation therapy. Int J Radiat Oncol Biol Phys 2002; 54: 1518–23
- Siemann DW, Chaplin DJ, Horsman MR. Vascular targeting therapies for treatment of malignant disease. Cancer 2004; 100: 2491–9
- Pettit GR, Singh SB, Hamel E, Lin CM, Alberts DS, Garcia-Kendall D. Isolation and structure of the strong cell growth and tubulin inhibitor combretastatin A-4. Experientia 1989; 45: 209–11
- Tozer GM, Kanthou C, Baguley BC. Disrupting tumour blood vessels. Nat Rev Cancer 2005; 5: 423–35
- Dark GG, Hill SA, Prise VE, Tozer GM, Pettit GR, Chaplin DJ. Combretastatin A-4, an agent that displays potent and selective toxicity toward tumour vasculature. Cancer Res 1997; 57: 1829–34
- Murata R, Siemann DW, Overgaard J, Horsman MR. Interaction between combretastatin A-4 disodium phosphate and radiation in murine tumours. Radiother Oncol 2001; 60: 155–61
- Blakey DC, Westwood FR, Walker M, Hughes GD, David PD, Ashton SE, et al. Antitumor activity of the novel vascular targeting agent ZD6126 in a panel of tumor models. Clin Cancer Res 2002; 8: 1974–83
- Davis PD, Dougherty GJ, Blakey DC, Galbraith SM, Tozer GM, Holder AL, et al. ZD6126: Anovel vascular-targeting agent that causes selective destruction of tumor vasculature. Cancer Res 2002; 62: 7247–53
- Siemann DW, Rojiani AM. Enhancement of radiation therapy by the novel vascular targeting agent ZD6126. Int J Radiat Oncol Biol Phys 2002; 53: 164–71
- Horsman MR, Murata R. Vascular targeting effects of ZD6126 in a C3H mouse mammary carcinoma and the enhancement of radiation response. Int J Radiat Oncol Biol Phys 2003; 57: 1047–55
- Ching LM, Joseph WR, Crosier KE, Baguley BC. Induction of tumor necrosis factor alpha messenger RNA in human and murine cells by the flavone acetic acid analogue 5,6 dimethylxanthenone-4-acetic acid. Cancer Res 1994; 54: 870–2
- Laws AL, Matthew AM, Double JA, Bibby MC. Preclinical in vitro and in vivo activity of 5,6-dimethylxanthenone-4-acetic acid. Br J Cancer 1995; 71: 1204–9
- Lash CJ, Li AE, Rutland M, Baguley BC, Zwi LJ, Wilson WR. Enhancement of the anti-tumour effects of the antivascular agent 5,6-dimethylxanthenone-4-acetic acid by combination with 5-hydroxytryptamine and bioreductive drugs. Br J Cancer 1998; 78: 439–45
- Murata R, Overgaard J, Horsman MR. Comporative effects of combretastain A-4 disodium phosphate an 5,6-dimethylxanthenon-4-acetic acid on blood perfusion in a murine tumour and normal tissus. Int J Radiat Biol 2001; 77: 195–204
- Murata R, Overgaard J, Horsman MR. Potentiation of the anti-tumor effect of hyperthermia by combining with the vascular targeting agent 5,6-dimethylxanthenone-4-acetic acid. Int J Hyperthermia 2001; 17: 508–19
- Maxwell RJ, Nielsen FU, Breidahl T, Stødkilde-Jørgensen H, Horsman MR. Effects of combretastatin on murine tumours monitored by 31P MRS, 1H MRS and 1H MRI. Int J Radiat Oncol Biol Phys 1998; 42: 891–4
- Overgaard J. Simultaneous and sequential hyperthermia and radiation treatment of an experimental tumor and its surrounding normal tissue in vivo. Int J Radiat Oncol Biol Phys 1980; 6: 1507–17
- Horsman MR, Sampson LE, Chaplin DJ, Overgaard J. The in vivo interaction between flavone acetic acid and hyperthermia. Int J Hyperthermia 1996; 12: 779–89
- Nordsmark M, Maxwell RJ, Horsman MR, Bentzen SM, Overgaard J. The effect of hypoxia and hyperoxia on nucleoside triphosphate/inorganic phosphate pO2 and radiation response in an experimental tumour model. Br J Cancer 1997; 76: 1432–9
- van den Boogaart A, Van Hecke A, Van Huffel P, Graveron-Demilly S, van Ormondt D, de Beer R. MRUI: A graphical user interface for accurate routine MRS data analysis. In: Proceedings of the ESMRMB 13th annual meeting. Prague: 1996. p. 318.
- Hill SA, Sampson LE, Chaplin DJ. Anti-vascular approaches to solid tumour therapy: Evaluation of vinblastine and flavone acetic acid. Int J Cancer 1995; 63: 119–23
- Grosios K, Holwell SE, McGown AT, Pettit GR, Bibby MC. In vivo and in vitro evaluation of combretastatin A-4 and its sodium phosphate prodrug. Br J Cancer 1999; 81: 1318–27
- Evelhoch JL, LoRusso PM, He Z, DelProposte Z, Polin L, Corbett TH, et al. Magnetic resonance imaging measurements of the response of murine and human tumors to the vascular-targeting agent ZD6126. Clin Cancer Res 2004; 10: 3650–7
- Galbraith SM, Rustin GJ, Lodge MA, Taylor NJ, Stirling JJ, Jameson M, et al. Effects of 5,6-dimethylxanthenone-4-acetic acid on human tumor microcirculation assessed by dynamic contrast-enhanced magnetic resonance imaging. J Clin Oncol 2002; 20: 3826–40
- Galbraith SM, Maxwell RJ, Lodge MA, Tozer GM, Wilson J, Taylor NT, et al. Combretastatin A4 phosphate has tumor antivascular activity in rat and man as demonstrated by dynamic magnetic resonance imaging. J Clin Oncol 2003; 21: 2831–42
- Maxwell RJ, Wilson J, Prise VE, Vojnovic B, Rustin GJ, Lodge MA, et al. Evaluation of the anti-vascular effects of combretastatin in rodent tumours by dynamic contrast enhanced MRI. NMR Biomed 2002; 15: 89–98
- Beauregard DA, Hill SA, Chaplin DJ, Brindle KM. The susceptibility of tumors to the antivascular drug combretastatin A4 phosphate correlates with vascular permeability. Cancer Res 2001; 61: 6811–5
- Beauregard DA, Pedley RB, Hill SA, Brindle KM. Differential sensitivity of two adenocarcinoma xenografts to the anti-vascular drugs combretastatin A4 phosphate and 5,6-dimethylxanthenone-4-acetic acid, assessed using MRI and MRS. NMR Biomed 2002; 15: 99–105
- Robinson SP, McIntyre DJO, Checkley D, Tessier JJ, Howe FA, Griffiths JR, et al. Tumour dose response to the antivascular agent ZD6126 assessed by magnetic resonance imaging. Br J Cancer 2003; 88: 1592–7
- Murata R, Siemann DW, Overgaard J, Horsman MR. Improved tumor response by combining radiation and the vascular-damaging drug 5,6-dimethylxanthenone-4-acetic acid. Radiat Res 2001; 156: 503–9
- McPhail LD, Chung Y-L, Madhu B, Clarke S, Griffiths JR, Kelland LR, et al. Tumor dose response to the vascular disrupting agent, 5,6-dimethylxanthenone-4-acetic acid, using in vivo magnetic resonance spectroscopy. Clin Cancer Res 2005; 11: 3705–13
- Nordsmark M, Grau C, Horsman MR, Støkilde-Jørgensen H, Overgaard J. Relationship between tumour oxygenation, bioenergetic status and radiobiological hypoxia in an experimental model. Acta Oncol 1995; 34: 329–34
- Beauregard DA, Thelwall PE, Chaplin DJ, Hill SA, Adams GE, Brindle KM. Magnetic resonance imaging and spectroscopy of combretastatin A4 prodrug-induced disruption of tumour perfusion and energetic status. Br J Cancer 1998; 77: 1761–7
- Raghunand N, Gillies RJ. pH and chemotherapy. The Tumour Microenvironment: Causes and Consequences of Hypoxia and Acidity, JA Goode, DJ Chadwick. John Wiley and Sons, London 2001; 199–211
- Overgaard J, Bichel P. The influence of hypoxia and acidity on the hyperthermic response of malignant cells in vitro. Radiol 1977; 123: 511–4
- Shi W, Horsman MR, Siemann DW. Combined modality approaches using vascular disrupting agents. Vascular-targeted Therapies in Oncology, DW Siemann. John Wiley and Sons, London (in press)
- Chaplin DJ, Acker B, Olive PL. Potentiation of the tumor cytotoxicity of melphalan by vasodilating drugs. Int J Radiat Oncol Biol Phys 1989; 16: 1131–5
- Pruijn FB, van Daalen M, Holford NH, Wilson WR. Mechanism of enhancement of the antitumour activity of melphalan by the tumour-blood-flow inhibitor 5,6-dimethylxanthenone-4-acetic acid. Cancer Chemother Pharmacol 1997; 39: 541–6
- Dodd NJF, Moore JV, Poppitt DG, Wood B. In vivo magnetic resonance imaging of the effects of photodynamic therapy. Br J Cancer 1989; 60: 164–7
- Zwi LJ, Baguley BC, Gavin JB, Wilson WR. The morphological effects of the anti-tumor agents flavone acetic acid and 5,6-dimethyl xanthenone acetic acid on the colon 38 mouse tumor. Pathology 1994; 26: 161–9
- Ferrero E, Villa A, Ferrero ME, Toninelli E, Bender JR, Pardi R, et al. Tumor necrosis factor alpha-induced vascular leakage involves PECAM1 phosphorylation. Cancer Res 1996; 56: 3211–5
- Robinson SP, Kalber TL, Howe FA, McIntyre DJ, Griffiths JR, Blakey DC, et al. Acute tumor response to ZD6126 assessed by intrinsic susceptibility magnetic resonance imaging. Neoplasia 2005; 7: 466–74
- Carano RA, Ross AL, Ross J, Williams SP, Koeppen H, Schwall RH, et al. Quantification of tumor tissue populations by multispectral analysis. Magn Reson Med 2004; 51: 542–51
- Thoeny HC, De Keyzer F, Vandecaveye V, Chen F, Sun X, Bosmans H, et al. Effect of vascular targeting agent in rat tumor model: Dyamic contrast-enhanced versus diffusion-weighted MR imaging. Radiol 2005; 237: 492–9
- Padhani AR. MRI for assessing antivascular cancer treatments. Br J Radiol 2003; 76: S60–80