Abstract
Radiation-induced impairment of renal function is preceded by capillary endothelial cell damage, which initiates a cascade of inflammatory and thrombotic events. Accumulation of leukocytes in the irradiated kidney, especially in areas surrounding the glomeruli, has been clearly demonstrated. The chemokine fractalkine has recently been identified as a key mediator of leukocyte adhesion that functions without the requirement of integrins or selectin-mediated rolling. In this study we investigate the possible involvement of fractalkine in the inflammatory response of the irradiated kidney. Mouse kidneys were irradiated with single doses of 16 or 0 Gy, and protein and mRNA levels of fractalkine and PECAM-1 were examined after 10 to 40 weeks. These changes were correlated with the progressive increase and distribution of leukocytes in the irradiated kidneys. Increased fractalkine immunoreactivity was seen at glomerular sites 30 to 40 weeks after irradiation. This fractalkine expression was strongly associated with the presence of leukocytes surrounding the Bowman's capsule of the same glomeruli. No significant changes in mRNA levels of fractalkine were seen in whole kidney extracts after irradiation, but expression levels were not determined for isolated glomeruli. PECAM-1 protein levels did not change with time after irradiation, although a significant decrease in mRNA expression was seen at 10 weeks. This study is the first demonstration of increased fractalkine after irradiation and the results suggest that fractalkine may be an important mechanism of leukocyte trafficking in the development of a radiation induced inflammatory response.
The kidney is one of the critical organs in abdominal irradiation, particularly where large volumes of both kidneys are included in the radiation field. Impairment of renal function is not usually detectable until about 4 to 6 months after irradiation, although cellular changes occur much earlier. A key process in the cascade of events occurring after irradiation is endothelial cell (EC) swelling and detachment from the basement membrane, followed by progressive cell loss. Remaining ECs exhibit increased permeability leading to platelet adhesion, thereby initiating the formation of mural microthrombi. If unopposed, subintimal proliferation occurs with subsequent thickening of the basement membrane and progressive enlargement of platelet-rich thrombi, which may lead to total vessel occlusion, thereby jeopardizing organ function Citation[1–5].
A prominent feature associated with vascular changes seen after irradiation is leukocyte attachment, which is likely to occur at sites of platelet activation and is a characteristic component of both acute and chronic inflammation. Accumulation of leukocytes has been demonstrated after kidney irradiation in the cortex, glomeruli and around the Bowmans capsule of glomeruli, where leukocytes form crescent-shaped clusters Citation[1], Citation[2], Citation[6], Citation[7].
Migration of leukocytes into extravascular tissues involves a cascade of molecular events, including the production and secretion of chemotactic and cell adhesion factors. Fractalkine (CX3CL1) is a recently discovered chemokine with several unique features. In addition to its chemotactic properties as a soluble chemokine, fractalkine is also expressed on activated endothelial cells in a membrane-bound form, which acts as an adhesion molecule, capturing fractalkine receptor-positive leukocytes without requiring selectin-mediated rolling or activation of integrins. The presence of a functional fractalkine receptor (CX3CR1) has recently been demonstrated on platelets, thereby providing evidence for a functional role of fractalkine in platelet activation Citation[8]. We hypothesize that these unique features make fractalkine well suited for platelet activation and leukocyte recruitment in tissues with high blood flow, such as in the renal glomerulus.
Platelet endothelial cell adhesion molecule 1 (PECAM-1, CD31) is highly expressed in the vasculature with approximately one million copies on the surface of each EC. PECAM-1 is involved in the initial formation and stabilization of cell-cell contacts, and maintenance of the vascular permeability barrier Citation[9]. It has also been implicated in neutrophil recruitment in vivo Citation[10] and leukocytes adhesion and transendothelial migration in vitro Citation[11], Citation[12].
Preventing the influx of inflammatory cells could help to reverse or inhibit the progression of renal injury after irradiation. The aim of the present study is to investigate the effects of ionizing radiation on the expression of key inflammatory markers involved in directing leukocyte infiltration at sites of inflammation, thereby elucidating their possible role in the pathogenesis of radiation nephropathy.
Materials and methods
Irradiation procedures
Female C3H mice (substrain HenAf-nu+) were irradiated at 12–14 weeks of age (body weight 23–27 g). Food and acidified water were provided ad libitum. Experiments were approved by the local animal welfare committee and were in accordance with the national regulations for animal experiments.
Non-anesthetized mice were sham-irradiated or irradiated with a single dose of 16 Gy via two lateral opposed tangential fields on both kidneys. Irradiation was performed with 250 kV x-rays, operating at 15 mA and filtered with 0.5 mm Cu. The dose rate at the position of the kidney was 2.35 Gy per minute and mice were rotated 180° halfway through each irradiation in order to ensure a homogeneous dose distribution.
Immunohistochemistry
Groups of 4–7 irradiated and age-matched control animals were sacrificed at intervals of 10 to 40 weeks after irradiation. Immediately before killing, mice were anesthetized and kidneys were perfused with 5 ml 0.9% NaCl. Kidneys were excised, frozen in liquid nitrogen and stored at −80°C. Cryosections (4 µm) were cut for immunohistochemistry, fixed for 10 min in cold acetone and incubated with specific primary antibodies () in 1% bovine serum albumine (BSA)/PBS (w/v) at room temperature for 60 min. After washing in PBS, sections were incubated with specific biotinylated secondary antibodies for 30 min, followed by peroxidase labeled avidin-biotin complex (Vector Laboratories, Burlingame, USA). The reactions were visualized using 3,3′diaminobenzidine (Vector Laboratories, Burlingame, USA) as the chromogen (brown reaction product). Sections were lightly counterstained with hematoxylin to discriminate the glomeruli from the surrounding tissue. The immunostaining conditions were optimized for each antibody reagent. Omission of primary antibodies served as negative controls. Tissue sections were analyzed without knowledge of the treatment.
Table I. Specific primary antibodies against proteins of interest.
Semi-quantitative scoring
The number of fractalkine positive glomeruli was determined as a percentage of the total number of glomeruli per section using a semi-quantitative scoring system based on the following criteria: ring formation or relative surface coverage of <30% or >30% (). Data are expressed as mean±S.D (n = 6–7 mice per group).
mRNA expression
Total RNA (free of genomic DNA) from irradiated and age matched control kidneys (n = 4/group) was isolated with RNAsol B according to the manufacturer's instructions (Bioteck Laboratories, Houston, USA). RNA concentrations were measured spectrophotometrically at 260 nm and purity was confirmed with 260/280 OD spectrophotometer readings.
Pooled RNA from four individual animals (total 5 µg) was reverse transcribed with Superscript II Reverse transcriptase (Invitrogen) and random hexamers in a total volume of 20 µl, according to the manufacturer's protocol. After cDNA synthesis, the volume was adjusted to 100 µl with water.
Real time PCR (RT-PCR) assays were performed to quantify levels of RNA transcripts of fractalkine, and PECAM-1. Specific primers for the genes of interest were designed using Primer Express 2.0 software (Applied Biosystems) and sequences are presented in . Samples were subjected to 45 cycles of amplification of 15 s at 95°C followed by 1 min at 60°C, using an ABI PRISM 7700 detection system and SYBR Green buffer according to the manufacturer's instruction with minor modification (Applied Biosystems). Water and RNA that had not been subjected to reverse transcription reactions were included to ensure specificity. Integrity was assessed for each sample through analysis of amplification plots and dissociation curves. In addition, the size of the PCR products was checked by agarose gel electrophoresis. The relative quantification was performed using the standard curve method with sham-irradiated kidney as a calibrator according to User Bulletin #2 (Applied Biosystems). To establish the reproducibility of the PCR in different runs, all amplifications were carried out in duplicate. Expression levels were normalized for the expression of GAPDH, a housekeeping gene.
Table II. Primersequences.
Statistical analysis of mRNA expression
Values are presented as relative mRNA levels compared to sham-irradiated controls, after normalization for a housekeeping gene, GAPDH. The Student's t-test was used to compare mean values (±S.D.) of sham-irradiated control and irradiated groups. A p-value < 0.05 was considered significant.
Results
Immunohistochemistry
shows kidney sections stained for the presence of leukocytes, fractalkine and PECAM-1 at 40 weeks after irradiation or sham treatment. Previous studies using quantitative immunohistochemistry demonstrated a progressive increase in leukocyte invasion in the mouse kidney from 10–40 weeks after irradiation Citation[7]. This was confirmed in the current study. At early time intervals (10–20 weeks) after irradiation, leukocytes were distributed randomly throughout the interstitium (data not shown), whereas at later times (peri)glomerular accumulation of leukocytes was found (A&B).
Figure 2. Photographs of mouse kidney sections stained immunohistochemically for leukocytes (A–B), fractalkine (C–D) and PECAM-1 (E–F) at 40 weeks after irradiation with 0 Gy or 16 Gy. All photomicrographs were taken at an original magnification of 400×. Sections were counterstained with hematoxylin and eosin.
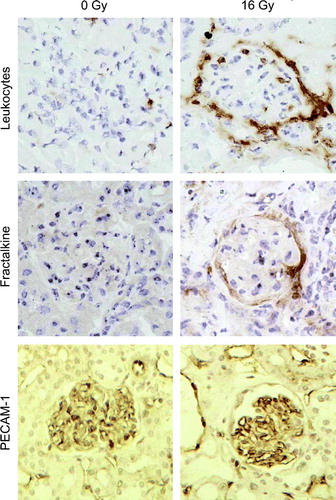
There was no detectable fractalkine immunoreactivity in normal sham-irradiated kidneys (C) or at 10–20 weeks after irradiation (data not shown). Increased expression of fractalkine was specifically found in and/or around the glomeruli at 30 and 40 weeks after irradiation (D). The immunohistochemical staining patterns for fractalkine ranged from ring formation to surface coverage of <30 or >30% (). Semi-quantitative scoring demonstrated a significant increase in the number of fractalkine immunopositive glomeruli as a percentage of the total number of glomeruli at 30 and 40 weeks after irradiation (). Staining of serial sections demonstrated that periglomerular fractalkine expression at late times after irradiation correlated with leukocytes surrounding the Bowmans capsule of those glomeruli (B,D). In contrast, leukocyte invasion of the renal cortex, which has been demonstrated with time after radiation was not associated with fractalkine expression (data not shown).
Figure 3. Semi-quantitative analysis of the number of fractalkine positive glomeruli as a percentage of the total number of glomeruli at different time intervals after irradiation or sham-treatment. Glomeruli were evaluated separately with surface coverage ranging from ring formation to < or >30%. Data are expressed as mean±S.D.
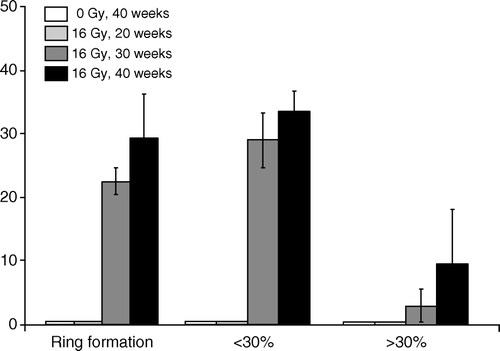
PECAM-1 immunoreactivity was prominent in ECs of both the renal cortex and glomeruli and there was no apparent difference between expression levels in irradiated and unirradiated sections (E,F). Previous studies using quantitative image analysis Citation[13] had shown no significant change in the amount of glomerular PECAM-1 protein with time after irradiation.
mRNA expression
Messenger RNA levels of genes of interest were quantified using real time PCR at different time intervals after irradiation. Single PCR products of the expected length were amplified (confirmed by agarose gel electrophoresis) from cDNA samples of both irradiated and control kidneys; no amplification was observed with the use of water or RT negative controls. For all genes of interest, correlation coefficients of standard curves from sham-irradiated kidneys were consistently >0.95 (mean R2±S.D.=0.98±0.04).
Transcripts of fractalkine were constitutively expressed during the observation period. There were no significant differences in mRNA expression between irradiated kidneys and sham-irradiated controls (A). In contrast, mRNA expression for PECAM-1 was significantly decreased at 10 weeks after irradiation, with partial recovery towards control levels at later times (B).
Discussion
Our previous studies showed that radiation-induced impairment of renal function in mice was preceded by a progressive increase in leukocytes, mainly in the cortex Citation[13]. Here, we provide evidence for the molecular mechanism that may mediate these inflammatory changes. Our data demonstrate that fractalkine present in the glomeruli of irradiated kidneys was strongly associated with leukocytes surrounding these glomeruli. This is the first demonstration of increased renal fractalkine after irradiation, although increased expression has also been reported in glomerular endothelium upon induction of anti-glomerular basement membrane glomerulonephritis Citation[14].
The most convincing evidence of a causal role for fractalkine in leukocyte recruitment was obtained from experiments using neutralizing antibodies for fractalkine or its receptor (CX3CR1) during the generation of inflammatory responses in experimental animals. In a model system of crescent glomerulonephritis, viral chemokine with antagonistic activity for CC-, CXC- and CX3C-chemokine receptors significantly reduced the infiltration of leukocytes and attenuated proteinuria Citation[14]. Other studies demonstrated that daily injections of anti-CX3CR1 antibody blocked the infiltration of leukocytes into the glomeruli, prevented crescent formation and improved renal function, suggesting a role for fractalkine in the pathogenesis of the glomerulonephritis Citation[15].
In kidneys of mice with bovine serum albumin overload proteinuria, fractalkine mRNA levels were approximately two fold higher than those in controls Citation[16]. Fractalkine expression was also induced in tubular epithelial cells in this model. In contrast, we were not able to detect any increased expression of fractalkine mRNA in extracts from whole irradiated kidneys, suggesting post-transcriptional regulation of the protein synthesis. The increased protein levels seen after in vivo irradiation may be due to increased release from damaged ECs, rather than a direct increase in transcription such as occurs after in vitro stimulation with cytokines (such as TNF alpha). Alternatively, a local increase in fractalkine mRNA in the glomeruli could remain undetected when analysing expression levels in whole kidney extract.
The discovery of fractalkine has provided new insights into the mechanisms of platelet activation, leukocyte recruitment and organ damage at sites of inflammation. While soluble chemokines activate platelets rather unspecifically, membrane-anchored fractalkine may represent a more specific mechanism by focussing activated platelet to the original site of EC damage Citation[8]. Changes in fractalkine protein levels might also attract mononuclear cells directly into the tissue and enhance their adhesion property, which in turn would favour inflammation and disease progression.
Taken together, these reports suggest that radiation-induced fractalkine might be an important mechanism for platelet adhesion and leukocyte trafficking at the endothelium in the high-flow glomerular circuit and, in turn, implicates its receptor as a prime drug target for therapeutic intervention of endothelium-related inflammatory renal disease after irradiation.
Due to the association between cell-cell adhesion and cell growth and migration, we also studied the expression and localization of EC adhesion molecule PECAM-1. We did observe a decrease in PECAM-1 mRNA, which was significant at 10 weeks. This might be associated with a reduction of the adhesion interaction between adjacent ECs, thereby enhancing their permeability Citation[9]. However, there was no apparent change in the immunoreactivity of PECAM-1 in irradiated kidneys, either in the present study or in a previous study Citation[13]. The role of PECAM-1 in the inflammatory processes in the irradiated kidney therefore remains uncertain.
In conclusion, irradiation has been shown to increase fractalkine expression, which may regulate periglomerular leukocyte invasion in irradiated mouse kidney. These changes may be part of the tissues’ attempt to protect itself or may contribute to tissue damaging processes. It is important to understand the potential limitations of extrapolating data from mice to humans. Therefore, the challenge remains how to convert this knowledge into potential therapies of radiation nephropathy.
References
- Stewart FA, Te Poele JAM, Van der Wal AF, Oussoren YG, Van Kleef EM, Kuin A, et al. The link between functional damage and vascular mediated inflammatory and thrombotic changes. Acta Oncol 2001; 40: 952–7
- Jaene RS, Robbins MEC, Bywaters T, Whitehouse E, Rezvani M, Hopewell JW Capillary endothelium. Target site of renal radiation injury. Lab Invest 1993;68:396–405.
- Stephens LC, Robbins ME, Johnston DA, Thames HD, Price RE, Peters LJ, et al. Radiation nephropathy in the rhesus monkey: Morphometric analysis of glomerular and tubular alterations. Int J Radiat Oncol Biol Phys 1995; 31: 865–73
- Robbins ME, Bonsib SM. Radiation nephropathy: A review. Scanning Microsc 1995; 9: 535–60
- Fajardo LF. The pathology of ionizing radiation as defined by morphologic patterns. Acta Oncol 2005; 44: 13–22
- Van Kleef E, Verheij M, Te Poele H, Dewit L, Stewart F. In vitro and in vivo expression of endothelial von Willebrand Factor and leukocyte accumulation after fractionated irradiation. Radiat Res 2000; 154: 375–81
- Van Kleef EM, Te Poele JAM, Oussoren Y, Verheij M, Van de Pavert I, Braunhut SJ, et al. Increased expression of glomerular von Willebrand Factor after irradiation of the mouse kidney. Radiat Res 1998; 150: 528–34
- Schafer A, Schulz C, Eigenthaler M, Fraccarollo D, Gawaz M, Ertl G, et al. Novel role of the membrane bound chemokine fractalkine in platelet activation and adhesion Blood 2003; 103: 407–12
- Ferrero E, Ferrero ME, Pardi R, Zocchi MR. The platelet endothelial cell adhesion molecule–1 (PECAM-1) contributes to endothelial barrier function. FEBS Lett 1995; 374: 323–6
- Vaporciyan AA, DeLisser HM, Yan HC, Mendiguren II, Thom SR, Jones ML, et al. Involvement of platelet-endothelial cell adhesion molecule-1 in neutrophil recruitment in vivo. Science 1993; 262: 1580–2
- Gaugler MH, Squiban C, Mouthon MA, Gourmelon P, van der Meeren A. Irradiation enhances the support of haemopoietic cell transmigration, proliferation and differentiation by endothelial cells. Br J Haematol 2001; 13: 940–50
- Gaugler MH, Vereycken-Holler V, Squiban C, Aigueperse J. PECAM-1 (CD31) is required for interactions of platelets with endothelial cells after irradiation. J Thromb Haemost 2004; 2: 2020–6
- Kuin A, Citarella F, Oussoren YG, Van der Wal AF, Dewit LGH, Stewart FA. Increased glomerular Vwf after kidney irradiation is not due to increased biosynthesis or endothelial cell proliferation. Radiat Res 2001; 156: 20–7
- Chen S, Bacon KB, Li L, Garcia GE, Xia Y, Lo D, et al. In vivo inhibition of CC and CX3C chemokine-induced leukocyte infiltration and attenuation of glomerulonephritis in Wistar-Kyoto (WKY) rats by vMIP-II. J Exp Med 1998; 188: 193–8
- Feng L, Chen S, Garcia GE, Xia Y, Siani MA, Botti P, et al. Prevention of crescentic glomerulonephritis by immunoneutralization of the fractalkine receptor CX3CR1. Kidney Int 1999; 56: 612–20
- Donadelli R, Zanchi C, Morigi M, Buelli S, Batani C, Tomasoni S, et al. Protein overload induces fractalkine upregulation in proximal tubular cells through nuclear factor kappaB- and p38 mitogen-activated protein kinase-dependent pathways. J Am Soc Nephrol 2003; 14: 2436–46