Abstract
Purpose. To evaluate the effect of the vascular disrupting drugs combretastatin A-4 disodium phosphate (CA4DP) and 5,6-dimethylxanthenone-4-acetic acid (DMXAA) on the intra/extracellular volume fraction of water and blood perfusion in tumours using MRI. Methods and materials. Mice with C3H mammary carcinomas underwent repeated MRI T2-weighted imaging, water-diffusion and perfusion measurements before and up to 6-hours following CA4DP and DMXAA treatment. Results. CA4DP treatment caused an increase in water diffusion in those tumour areas that presented the lowest blood perfusion, however this appeared only after five hours. The blood perfusion in highly perfused tumour regions decreased immediately after administration of CA4DP. DMXAA treatment caused an early decrease in water diffusion in the low-perfused tumour segments and followed by a subsequent decrease in the remaining part of the tumour. The blood perfusion decreased early in all parts of the tumour. Conclusion. The effect of CA4DP and DMXAA on tumour blood flow was comparable. The reduction in regional blood flow caused by CA4DP in the whole tumour segment occurred early, however, changes in ADC after DMXAA appeared more extended and earlier than after CA4DP treatment, especially in tumour areas already suffering from a low blood perfusion.
Formation of new blood vessels is a prerequisite for tumour growth in order to satisfy an increased demand of oxygen and nutrients. When tumour growth rate exceeds the capacity of the local blood supply, growth factors which stimulate angiogenesis are released. As a result, the surrounding mature vessels will begin sprouting new capillaries that grow toward and infiltrate the tumour, and thus initiate an unrestricted expansion Citation[1]. As tumour vasculature is generally very different from normal tissue vessels Citation[2], the tumour blood vessels are potentially a target for therapy Citation[3] either by anti-angiogenetic drugs preventing formation of new blood vessels or by vascular disrupting drugs destroying already formed tumour blood Citation[4].
Combretastatin A-4 disodium phosphate (CA4DP) and 5,6-dimethylxanthenone-4-acetic acid (DMXAA) are two vascular disrupting drugs widely used. CA4DP is a tubulin-binding agent that destabilizes the cytoskeleton tubulin polymers of dividing endothelial cells and has been shown to cause a rapid, extensive and irreversible vascular shutdown concomitant with a reduction in the vascular volume Citation[5–8]. Similarly, DMXAA has shown remarkable anti-tumour effects Citation[9], Citation[10], reducing the tumour blood supply by activation of the tumour necrosis factor (TNF) Citation[11]. Both CA4DP and DMXAA can compromise the established tumour vasculature, causing a decrease in regional blood flow.
As a consequence of vascular shutdown by CA4DP and DMXAA, cell swelling will occur together with changes in the balance between intra- and extra-cellular water. However, because of different mechanisms of the CA4DP and DMXAA the effect on tumour blood vessels on tumour cell viability could be different.
Identification of physiological changes in cells subjected to ischemia can be done with a number of techniques. However, magnetic resonance imaging or spectroscopy provides non-invasively a number of parameters for quantification of cellular functional changes in tumours, thus being suited for longitudinal studies. Water diffusion and regional blood perfusion are key parameters in identifying tumour segments suffering from ischemia. The apparent diffusion coefficient (ADC) is dependent on the ratio between the intra cellular and extra cellular fluid compartment. This means that ADC will change accordingly to the cell swelling caused by ischemia; ADC will change in case the cells swell. Thus the aim of this study was to evaluate differences in ADC and regional blood perfusion in response CA4DP and DMXAA by MRI based measurements of regional blood perfusion and water diffusion.
Materials and methods
Animal model
C3H mouse mammary carcinomas were transplanted on the right rear foot of 10–14 weeks old female CDF1 mice. Experiments were carried out when tumours reached a volume of 200 mm3, approximately 3 weeks after implantation. The tumour volume was determined by the formula: D1×D2×D3×π/6, where D1, D2 and D3 represent three orthogonal diameters. Thirty-two mice were randomly divided into three groups: 1) Saline treated group (n = 3) received ip injection of 0.4ml saline, 2) CA4DP treated group (n = 13) received ip injection of 250 mg/kg CA4DP (Oxigene Europe AB, Lund, Sweden), and 3) DMXAA treated group (n = 16) received ip injection of 25 mg/kg DMXAA (provided by Dr William Denny, University of Auckland, New Zealand). CA4DP and DMXAA were dissolved in saline before injection. The employed doses of CA4DP and DMXAA were selected as these have been shown to induce a maximal reduction in the tumor blood perfusion Citation[12].
Animals were awake during the complete procedure which lasted nearly six hours. They were restrained in a plastic jig and left in the magnet during the whole experiment. This allowed for the interpolation of tumour image segments between different image recordings during an experiment. An 18 G venflon connected with a polythene tube (Ø: 0.38 mm) was intravenously inserted for administration of CA4DP or DMXAA. A 27 G butterfly connected with a polythene tube (Ø: 0.38 mm) was inserted in the tail vein for bolus injection of an MR contrast agent. To maintain constant temperature (37±0.5°C measured rectal temperature) throughout the experiment a plastic tube with circulating warm water was wrapped around the body. The experimental study was approved by the local animal research committee.
Magnetic Resonance Imaging
MRI was performed with a 7 Tesla horizontal bore magnet (Oxford Instruments, Oxford, UK), interfaced to a Unity Inova console (Varian Inc, Palo Alto, CA), and equipped with a 12.5 Gauss/cm gradient system (Tesla Engineering Limited, West Sussex, UK). A home-built 9 mm diameter double-turned surface coil served both as a transmitter and receiver.
ADC was estimated from diffusion weighted images recorded with a Stejskal-Tanner spin-echo imaging sequence using the following parameters: TR = 2300 ms, TE = 50 ms, slice thickness of 2 mm, 128×128 data matrix, coronal orientation and field-of-view of 4×4 cm2. One slice through the centre of tumour was acquired. The diffusion weighting was set by the b-value given by b = γ2δ2(Δ-δ/3)G2, where γ is the gyromagnetic constant, δ is the duration of the individual gradient pulses, Δ is the time separation between the leading edges of the two gradient pulses and G is the strength of the diffusion gradient, which was applied in the read-out direction. Diffusion gradients equivalent to b-values of 0 and 1370×10−3 s/mm2 were employed using gradient pulse widths of δ = 15 ms and Δ = 30 ms. ADC was calculated with the equation: ADC = ln[S(0)/S(b)]/b, where S(b) is the signal intensity at diffusion-weighting factor b and S(0) is the signal intensity at b = 0. DWI was performed at 0, 60, 120, 180, 240, 300, and 360 min.
Blood perfusion weighted images were acquired with a dynamic contrast enhanced T1-weighted gradient-spoiled gradient-echo sequence using the following MRI parameters: TR = 30 ms, TE = 3 ms, flip-angle of 40°, slice thickness of 2 mm, 64×64 data matrix, coronal orientation and field-of-view of 4×4 cm2. One image slice was acquired through the centre of the tumour, and a total of 150 images were collected in each sequence. For estimations of the relative blood perfusion, Gd-DTPA (Magnevist; Bayer Schering Pharma, Berlin, Germany) was adminstered with a dosage of 0.1 mmol/kg. Earlier studies have demonstrated that the administered Gd-DTPA necessary for blood flow estimations affects neither the tumour blood flow nor the extra/intracellular volume fraction of water Citation[13]. Quantitative perfusion values were given as the relative blood flow (rBF) with units of ml/s/cm3. Blood perfusion was estimated at −20, 80, 140, 200, 260, 320, 380 min following administration of drugs.
Third, a T2-weighted imaging sequence was applied to obtain morphologically representative images of the tumour, performed with spin echo imaging sequence using the following MRI parameters: TR = 2300 ms, TE = 50 ms, slice thickness of 2 mm, 128×128 data matrix, coronal orientation and field-of-view of 4×4 cm2, one slice through the centre of tumour was acquired. T2 weighted imaging was acquired at −10, 70, 130, 190, 150, 310, 370 min.
ROI selection
Image post-processing of DWI and PWI was performed using the MIStar software (Apollo Imaging Technology Pty, Melbourne, Australia). The segmentation of the tumours started with outlining the circumference of the whole tumour in the T2 image. This area was transferred to the perfusion image. Within the outline of the whole tumour in image areas with highest and lowest perfusion values were pointed out and the segmentation software was allowed to expand the area until a gray tone shift was obtained. An example of this semi-automatic segmentation is shown in . The segmentation was performed on the images acquired before administration of drugs. The identified segment coordinates were transferred onto the following images and duplicated onto the ADC maps.
Figure 1. Selection of regions of interests (ROIs). The tumor was transplanted on the right rear foot of 10–14 weeks old female CDF1 mice. The animals were restrained in a plastic jig. The right foot was exposed and secured with tape, and the tail was put through a hole in the rear of the jig, helping to secure the mouse in a fixed position. The tumor was positioned adjacent to a radiofrequency surface-coil Citation[13]. DWI, PWI and T2 weighted imaging was acquired with a coronal slice, which has been stated in the text. This means that the slice orientation was mostly parallel to the surface of the skin. The area in images representing tumour tissue was divided into three regions of interests: 1) whole tumour segment, a ROI covering the whole tumour area, 2) high perfusion segments, and 3) low perfusion segments. The tumour areas with the lowest blood perfusion were primarily located in the central parts of the tumour, whereas higher levels of blood perfusion were found in the periphery close to the surrounding healthy cells.
![Figure 1. Selection of regions of interests (ROIs). The tumor was transplanted on the right rear foot of 10–14 weeks old female CDF1 mice. The animals were restrained in a plastic jig. The right foot was exposed and secured with tape, and the tail was put through a hole in the rear of the jig, helping to secure the mouse in a fixed position. The tumor was positioned adjacent to a radiofrequency surface-coil Citation[13]. DWI, PWI and T2 weighted imaging was acquired with a coronal slice, which has been stated in the text. This means that the slice orientation was mostly parallel to the surface of the skin. The area in images representing tumour tissue was divided into three regions of interests: 1) whole tumour segment, a ROI covering the whole tumour area, 2) high perfusion segments, and 3) low perfusion segments. The tumour areas with the lowest blood perfusion were primarily located in the central parts of the tumour, whereas higher levels of blood perfusion were found in the periphery close to the surrounding healthy cells.](/cms/asset/6e796d8d-03ca-4a1d-98d6-a77bdc4932f9/ionc_a_276967_f0001_b.gif)
Statistics
Statistical analysis was performed with STATA 9.0 (StataCorp LP, College Station, TX) using univariate repeated ANOVA. A statistical significance level of 0.05 was used.
Results
In animals receiving saline, no significant changes were observed in neither ADC (A) nor in the blood perfusion (B). The low-perfused tumour segments constituted 10–20% and the high-perfused segments 30–40% of the whole tumour area. The tumour areas with the lowest blood perfusion were primarily located in the central parts of the tumour, whereas higher levels of blood perfusion were found in the periphery close to the surrounding healthy cells.
Figure 2. Time dependent effects of saline (0.4 ml ip), CA4DP (250 mg/kg ip) and DMXAA (20 mg/kg ip) on ADC (2A, 2C, 2E) and relative blood flow (rBF) (2B + 2D + 2F). Results are shown following treatment with saline (2A, 2B), CA4DP (2C, 2D) or DMXAA (2E, 2F) for high-perfused (⋄), low-perfused (□) and whole tumour areas (Δ). Values that are significantly different compared to baseline values are filled with black color. Data are shown as mean±sem.
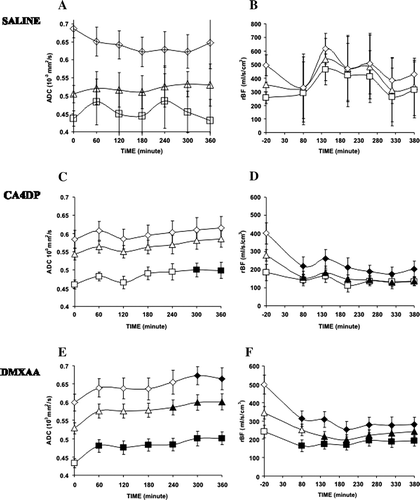
For mice receiving CA4DP, no change in ADC was found in neither the whole tumour nor in the high-perfused segments, respectively, compared to the levels before injection of CA4DP (C). In low-perfused segments, however, a significant increase was observed at 5 hours after injection of CA4DP (p = 0.047). The blood perfusion decreased significantly in the CA4DP group in both the whole tumour (p = 0.027) area and in the high-perfused tumour areas (p = 0.025) (D). This fall appeared within the first hour after CA4DP injection both in the high-perfused segments (p = 0.026) and in the whole tumour segment (p = 0.034). Blood perfusion remained low throughout the remaining 6 hours. In the low perfused tumour segment, blood perfusion did not show a significant further reduction.
For the DMXAA group, ADC increased significantly in whole tumour (p = 0.03), high perfused segments (p = 0.013) and low perfused segment (p = 0.034) (E). However, this increase only appeared after 4 and 5 hours in the high-perfused tumour segments (p = 0.037) and within the whole tumour area (p = 0.032), respectively. In contrary to the changes in ADC observed in the CA4DP group, the increase in ADC of the low-perfused segments was significant within the first hour in this DMXAA group (p = 0.036). The DMXAA treated animals also showed a significant decrease in blood perfusion in whole tumour area (p = 0.005) and in the high perfused segments (p = 0.019) and in the low perfused segments (p = 0.028) (F). In both the high-perfused and low-perfused segments, the reduction appeared already in the first hour after drug injection and remained significantly lower than baseline values for the entire six hours. However, looking at the whole tumour a significant decrease in blood perfusion was not observed before two hours after injection.
Discussion
Blood vessels within a tumour are unevenly distributed, and the present study confirmed that the effect of vascular disrupting drugs differed in different parts of the tumour Citation[14]. We have previously demonstrated spatial differences using T2-weighted MRI analysed with image texture parameters Citation[15]. The present study confirmed these structural differences, not only in relation to blood perfusion, but also in the measured ADC. Furthermore, differences in both spatial and temporal effect of CA4DP and DMXAA on blood perfusion and on ADC were demonstrated.
The segmentation of the ADC maps into low and high perfusion areas was based on a semi-automatic segmentation procedure. An automatic and thus fully non-biased segmentation method could have been applied; however, the practical experience with such algorithms was that this method was too unstable with major errors that needed manually corrections anyway. The following transfer of the high and low perfused segments to the ADC maps was possible because the tumors were not allowed to move during the whole procedure.
The saline group did not show significant differences in either ADC or in blood perfusion. The standard deviations varied more than in the CA4DP and the DMXAA group, however, this might be caused by the rather low number of mice entering this group. The results following CA4DP and DMXAA are inside the intrinsic variation we found in the saline group.
The ADC is considered to be dependent on both the intra-cellular water space and the extra-cellular space Citation[16]. As the water molecule movements are more restricted in the intra-cellular compartment compared to the extra-cellular compartment, a shift in ADC may indicate a shift in the distribution of water between the two compartments. This can be observed in tissue undergoing cellular swelling Citation[17] and could be an early indication of therapeutic response in tumours Citation[18–20]. Particularly, it has been shown that viable tumour cells segments can be differentiated from tumour necrosis by DWI Citation[21], Citation[22], and that ADC mapping can be successfully used to assess an early tumour response after chemotherapy and radiation therapy Citation[23]. Buijs et al. found after transarterial chemo-embolization for metastatic breast cancer (14 patients), the mean tumor ADC increased, but the ADC values in the non-tumorous liver, spleen, and kidney remained unchanged Citation[24].
CA4DP exerts its effect mainly by destabilizing tubulin polymers of proliferating endothelial cells, and the accompanying reduction in vascular volume results in partial or total vascular shutdown Citation[5–8]. In areas where blood perfusion is already low, further vascular shutdown results in red cells aggregating and an increased viscous resistance Citation[25]. While CA4DP caused a pronounced reduction in blood perfusion in those areas that already had a high blood perfusion, the blood perfusion remained unchanged in the low-perfused areas. This finding may be explained by tumour blood perfusion in the low-perfused areas already is at the boundary for cells to survive and further reduction in blood supply results in necrosis; a hypothesis that is supported by the present results in which CA4DP treatment caused an increased ADC, mainly in areas already suffering from a low blood perfusion.
DMXAA can partially dissolve the actin filaments of endothelial cells to cause a rapid shutdown of tumour blood vessels Citation[25] through the induction of TNF Citation[26–28]. Despite the unevenly distributed blood vessels within tumours, DMXAA can cause a spatially uniform shutdown in blood perfusion. This effect was confirmed in our study, where the blood perfusion decreased in all parts of the tumour within the first hour after DMXAA treatment. This acute reduction in blood flow is probably related to the multiple mechanisms of DMXAA: like release of cytokines such as TNF and interferons, stimulation of tumour macrophages, and an increased formation of lymphokine-activating killer cells Citation[29]. Compared to the CA4DP group, ADC (or the intra/extracellular volume ratio) increased in all regions in the DMXAA treated group over time. This pattern was different in the CA4DP-treated group, in which an increased ADC was only detected in low-perfused areas.
The findings of this experimental study may advantageously have been corroborated with ex-vivo microscopy (or other invasive modalities). Unfortunately, it was difficult to identify the same tumour segments with microscopy as those used in the MRI analyses. Further, other invasive measurements of tumour physiological or structural parameter compromise the tissue and thus impede the benefit of non-invasive MRI in a longitudinal study.
In conclusion, CA4DP and DMXAA both showed spatial and temporal differences in their ability to significantly affect the vascular conditions in tumours. The reduction in regional blood flow caused by CA4DP in the whole tumour segment occurred early in both drugs. However, the changes in ADC after DMXAA appeared more extended and earlier than after CA4DP treatment, especially in tumour areas already suffering from a low blood perfusion.
Acknowledgements
The authors would like to thank Ms I.M. Horsman, Ms P. Schjerbeck and Ms D. Grand for technical help. This study was funded in part by the Danish Medical Research Council (Grant #22-05-0519 BMP-MP), Karen Elise Jensens Foundation and the Danish Cancer Society. Michael R. Horsman is a consultant for Oxigene, Europe AB, Lund, Sweden.
References
- Kerbel RS. Tumor angiogenesis: Past, present and the near future. Carcinogenesis 2000; 21: 505–15
- Vaupel P, Kallinowski F, Okunieff P. Blood flow, oxygen and nutrient supply, and metabolic microenvironment of human tumors: A review. Cancer Res 1989; 49: 6449–65
- Siemann DW, Warrington KH, Horsman MR. Targeting tumor blood vessels: An adjuvant strategy for radiation therapy. Radiother Oncol 2000; 57: 5–12
- Murata R, Overgaard J, Horsman MR. Combretastatin A-4 disodium phosphate: A vascular targeting agent that improves that improves the anti-tumor effects of hyperthermia, radiation, and mild thermoradiotherapy. Int J Radiat Oncol Biol Phys 2001; 51: 1018–24
- Pettit GR, Singh SB, Hamel E, Lin CM, Alberts DS, Garcia-Kendall D. Isolation and structure of the strong cell growth and tubulin inhibitor combretastatin A-4. Experientia 1989; 45: 209–11
- Woods JA, Hadfield JA, Pettit GR, Fox BW, McGown AT. The interaction with tubulin of a series of stilbenes based on combretastatin A-4. Br J Cancer 1995; 71: 705–11
- Dark GG, Hill SA, Prise VE, Tozer GM, Pettit GR, Chaplin DJ. Combretastatin A-4, an agent that displays potent and selective toxicity toward tumor vasculature. Cancer Res 1997; 57: 1829–34
- Galbraith SM, Maxwell RJ, Lodge MA, Tozer GM, Wilson J, Taylor NJ, et al. Combretastatin A4 phosphate has tumor antivascular activity in rat and man as demonstrated by dynamic magnetic resonance imaging. J Clin Oncol 2003; 21: 2831–42
- Zwi LJ, Baguley BC, Gavin JB, Wilson WR. The morphological effects of the anti-tumor agents flavone acetic acid and 5,6-dimethyl xanthenone acetic acid on the colon 38 mouse tumor. Pathology 1994; 26: 161–9
- Ching LM, Goldsmith D, Joseph WR, Korner H, Sedgwick JD, Baguley BC. Induction of intratumoral tumor necrosis factor (TNF) synthesis and hemorrhagic necrosis by 5,6-dimethylxanthenone-4-acetic acid (DMXAA) in TNF knockout mice. Cancer Res 1999; 59: 3304–7
- Beauregard DA, Pedley RB, Hill SA, Brindle KM. Differential sensitivity of two adenocarcinoma xenografts to the anti-vascular drugs combretastatin A4 phosphate and 5,6-dimethylxanthenone-4-acetic acid, assessed using MRI and MRS. NMR Biomed 2002; 15: 99–105
- Murata R, Overgaard J, Horsman MR. Comparative effects of combretastatin A-4 disodium phosphate and 5,6-dimethylxanthenone-4-acetic acid on blood perfusion in a murine tumour and normal tissues. Int J Radiat Biol 2001; 77: 195–204
- Chen G, Jespersen SN, Pedersen M, Pang Q, Horsman MR, Stodkilde-Jorgensen H. Intravenous administration of Gd-DTPA prior to DWI does not affect the apparent diffusion constant. Magn Reson Imaging 2005; 23: 685–9
- Zhao D, Jiang L, Hahn EW, Mason RP. Tumor physiologic response to combretastatin A4 phosphate assessed by MRI. Int J Radiat Oncol Biol Phys 2005; 62: 872–80
- Chen G, Jespersen S, Pedersen M, Pang Q, Horsman MR, Stodkilde-Jorgensen H. Evaluation of anti-vascular therapy with texture analysis. Anticancer Res 2005; 25: 3399–405
- Gass A, Niendorf T, Hirsch JG. Acute and chronic changes of the apparent diffusion coefficient in neurological disorders--biophysical mechanisms and possible underlying histopathology. J Neurol Sci 2001; 186(Suppl 1)S15–S23
- Le BD. Molecular diffusion, tissue microdynamics and microstructure. NMR Biomed 1995; 8: 375–86
- Zhao M, Pipe JG, Bonnett J, Evelhoch JL. Early detection of treatment response by diffusion-weighted 1H-NMR spectroscopy in a murine tumour in vivo. Br J Cancer 1996; 73: 61–4
- Chenevert TL, Mckeever PE, Ross BD. Monitoring early response of experimental brain tumors to therapy using diffusion magnetic resonance imaging. Clin Cancer Res 1997; 3: 1457–66
- Poptani H, Puumalainen AM, Grohn OH, Loimas S, Kainulainen R, Yla-Herttuala S, et al. Monitoring thymidine kinase and ganciclovir-induced changes in rat malignant glioma in vivo by nuclear magnetic resonance imaging. Cancer Gene Ther 1998; 5: 101–9
- Lyng H, Haraldseth O, Rofstad EK. Measurement of cell density and necrotic fraction in human melanoma xenografts by diffusion weighted magnetic resonance imaging. Magn Reson Med 2000; 43: 828–36
- Lang P, Wendland MF, Saeed M, Gindele A, Rosenau W, Mathur A, et al. Osteogenic sarcoma: Noninvasive in vivo assessment of tumor necrosis with diffusion-weighted MR imaging. Radiology 1998; 206: 227–35
- Vossen JA, Buijs M, Kamel IR. Assessment of tumor response on MR imaging after locoregional therapy. Tech Vasc Interv Radiol 2006; 9: 125–32
- Buijs M, Kamel IR, Vossen JA, Georgiades CS, Hong K, Geschwind JF. Assessment of metastatic breast cancer response to chemoembolization with contrast agent enhanced and diffusion-weighted MR imaging. J Vasc Interv Radiol 2007; 18: 957–63
- Tozer GM, Kanthou C, Baguley BC. Disrupting tumour blood vessels. Nat Rev Cancer 2005; 5: 423–35
- Galbraith SM, Rustin GJ, Lodge MA, Taylor NJ, Stirling JJ, Jameson M, et al. Effects of 5,6-dimethylxanthenone-4-acetic acid on human tumor microcirculation assessed by dynamic contrast-enhanced magnetic resonance imaging. J Clin Oncol 2002; 20: 3826–40
- Jameson MB, Baguley BC, Kestell P, Zhao L, Paxton JW, Thompson PI, Waller S Pharmacokinetics of 5,6-dimethylxanthenone-4-acetic acid (AS1404), a novel vascular disrupting agent, in phase I clinical trial. Cancer Chemother Pharmacol 2007;59:681–687.
- Rustin GJ, Bradley C, Galbraith S, Stratford M, Loadman P, Waller S, et al. 5,6-dimethylxanthenone-4-acetic acid (DMXAA), a novel antivascular agent: Phase I clinical and pharmacokinetic study. Br J Cancer 2003; 88: 1160–7
- Zhou S, Kestell P, Baguley BC, Paxton JW. 5,6-dimethylxanthenone-4-acetic acid (DMXAA): A new biological response modifier for cancer therapy. Invest New Drugs 2002; 20: 281–95