Abstract
Purpose. To clarify the radiosensitivity of intratumor total and quiescent (Q) cells in vivo to accelerated carbon ion beams compared with γ-ray irradiation. Materials and methods. SCC VII tumor-bearing mice received a continuous administration of 5-bromo-2′-deoxyuridine (BrdU) to label all intratumor proliferating (P) cells. Then they received 290 MeV/u carbon ions or γ-rays. Immediately or 12 hours after the irradiation, the radiosensitivity of Q cells was assessed in terms of the micronucleus frequency using immunofluorescence staining for BrdU. That of the total (=P+Q) tumor cells was determined from the BrdU non-treated tumors based on the micronucleus frequency and clonogenic cell survival. Results. The apparent difference in radiosensitivity between total and Q cell populations under γ-ray irradiation was markedly reduced with carbon ion beam, especially with a higher linear energy transfer (LET) value. Clearer repair in Q cells than total cells through delayed assay under γ-ray irradiation was efficiently inhibited with carbon ion beams, especially with a higher LET. Conclusion. In terms of tumor cell-killing effect as a whole, including intratumor Q cells, carbon ion beams, especially with higher LET values, were very useful for suppressing the dependency on the heterogeneity within solid tumors as well as depositing radiation dose precisely.
It is thought that heavy charged particle beams can offer an improved dose conformation to the target volume as compared with photon radiotherapy, with better sparing of normal tissue structures close to the target Citation[1]. In addition, beams of ions heavier than helium exhibit a strong increase in linear energy transfer (LET) in the Bragg peak as compared with the entrance region Citation[1]. These physical properties are much more advantageous than those of photon radiotherapy.
Meanwhile, high-LET radiation, except for protons, is more effective Citation[2] than low-LET x- or γ-radiation at inducing biological damage. High-LET radiation gives a higher relative biological effectiveness (RBE) value for cell killing Citation[3], a reduced oxygen effect Citation[3], and a reduced dependence on the cell-cycle Citation[4], Citation[5], making it potentially superior to low-LET radiation in the treatment of cancer. Thus, particle radiation therapy has gained increasing interest worldwide, and many clinical centers are considering introducing radiation therapy with charged particles heavier than protons. However, almost all these radiobiological characteristics of high-LET charged particle beams were based on the response of total tumor cell populations as a whole using in vitro cell cultures or in vivo solid tumors Citation[1].
Many cells in solid tumors are quiescent in situ but are still clonogenic Citation[6]. These quiescent (Q) tumor cell populations have been thought to be more resistant to low-LET radiation because of larger hypoxic fractions and greater capacity to repair potentially lethal damage (PLD) than proliferating (P) tumor cells, mainly based on the characteristics of plateau-phase cultured cells in vitro Citation[6]. So far, employing our method for selectively detecting the response of intratumor Q cell populations in vivo, we have already showed that all these characteristics could also be applied to Q state cells in solid tumors in vivo Citation[7].
In this study, we analyzed the micronucleus formation in the total (=P+Q) and Q cell populations in solid tumors, and the clonogenic cell survival in the total cell population through irradiation to the solid tumors with 290 MeV/u carbon ion beams of various LET values in a-6-cm spread-out Bragg peak (SOBP), compared with cobalt-60 γ-ray beams, using our method for selectively detecting the response of Q cells in solid tumors Citation[7]. The carbon ion beams were produced by a heavy-ion accelerator installed at the National Institute of Radiological Sciences (NIRS) in Chiba, Japan. The accelerator was originally set up for radiation therapy for refractory malignant solid tumors Citation[8].
Materials and methods
Mice and tumors
SCC VII squamous cell carcinomas (Department of Radiology, Kyoto University) derived from C3H/He mice were maintained in vitro in Eagle's minimum essential medium supplemented with 12.5% fetal bovine serum. Cells were collected from exponentially growing cultures, and 1.0×105 cells were inoculated subcutaneously into the left hind legs of 8- to 11-week-old syngeneic female C3H/He mice (Japan Animal Co., Ltd., Osaka, Japan). Fourteen days after the inoculation, each tumor had reached approximately 1 cm in diameter. At treatment, the body weight of the tumor-bearing mice was 22.1±2.3 (Mean±SD) g. Mice were handled according to the Recommendations for Handling of Laboratory Animals for Biomedical Research, compiled by the Committee on Safety and Ethical Handling Regulations for Laboratory Animal Experiments, Kyoto University and NIRS. All experimental procedures mentioned here were in accordance with institutional guidelines for the care and use of laboratory animals in research. Incidentally, the p53 of the SCC VII tumor cells is the wild type Citation[9].
Labeling with 5-bromo-2′-deoxyuridine (BrdU)
Nine days after the tumor cell inoculation, mini-osmotic pumps (Durect Corporation, Cupertino, CA) containing BrdU dissolved in physiological saline (250 mg/ml) were implanted subcutaneously to label all P cells for 5 days. Administration of BrdU did not change the tumor growth rate. The tumors were 1 cm in diameter on treatment. The labeling index after continuous labeling with BrdU was 55.3±4.5%, and reached a plateau at this stage. Therefore, we regarded tumor cells not incorporating BrdU after continuous labeling as Q cells. Needless to say, during the 5-day labeling period, cells that early incorporate BrdU may become Q cells later. However, cells that cannot incorporate BrdU for the 5-day labeling period are thought to be certainly Q status cells.
Irradiation
After the labeling with BrdU, tumor-bearing mice received γ-ray irradiation or accelerated carbon ion beam irradiation. Irradiation was carried out with tumor-bearing mice held in a specially designed device made of acrylic resin with the tail and both the arms and legs firmly fixed with an adhesive tape. γ-ray irradiation was performed with a cobalt-60 γ-ray irradiator at a dose rate of 2.75 Gy/min. Carbon-12 ions were accelerated up to 290 MeV/u by the synchrotron of the Heavy Ion Medical Accelerator in Chiba (HIMAC). Irradiation was conducted using horizontal carbon beams with a dose-rate of approximately 1 Gy/min. The LET of the 290 MeV/u carbon beam with the 6-cm SOBP ranges from 14 keV/µm to greater than 200 keV/µm, depending on depth. The SOBP employed here is composed of various LET components with different weighting factors at each depth, which were obtained from penetration of mono-energetic charged particles through a different thickness of bar-ridge filters Citation[10]. Desired LET beams could be obtained almost continuously by selecting the depth along the beam path through regulating the thickness of a Lucite range shifter Citation[10]. Carbon beams with 18, 43, 50 and 74 keV/µm LET were obtained at the middle of the plateau, upstream within the 6-cm SOBP, at the middle of the SOBP, and downstream within the SOBP, respectively. A desired irradiation field was obtained by the simultaneous use of an iron collimator and a brass collimator.
Each irradiation group also included mice that were not pretreated with BrdU. The tumors were then excised immediately and 12 h after irradiation.
Immunofluorescence staining of BrdU-labeled cells and micronucleus (MN) assay
Tumors excised from the mice given BrdU were minced and trypsinized [0.05% trypsin and 0.02% ethylenediamine-tetraacetic acid (EDTA) in phosphate-buffered saline (PBS), 37°C, 15 min]. Tumor cell suspensions thus obtained were incubated for 72 h in tissue culture dishes containing complete medium and 1.0 µg/ml of cytochalasin-B to inhibit cytokinesis while allowing nuclear division, and the cultures were then trypsinized and cell suspensions were fixed. After the centrifugation of fixed cell suspensions, the cell pellet was resuspended with cold Carnoy's fixative (ethanol:acetic acid=3:1 in volume). The suspension was then placed on a glass microscope slide and the sample was dried at room temperature. To enable detection of BrdU in P cells, the slides were treated with 2 M hydrochloric acid for 60 min at room temperature to dissociate the histones and partially denature the DNA. The slides were then immersed in borax-borate buffer (pH 8.5) to neutralize the acid. BrdU-labeled tumor cells were detected by indirect immunofluorescence staining using monoclonal anti-BrdU antibody (Becton Dickinson, San Jose, CA) and fluorescein isothiocyanate (FITC)-conjugated antimouse IgG antibody (Sigma, St. Louis, MO). To observe the double staining of tumor cells with green-emitting FITC and red-emitting propidium iodide (PI), cells on the slides were treated with PI [2 µg/ml in phosphate-buffered saline (PBS)] and monitored under a fluorescence microscope.
The MN frequency in cells not labeled with BrdU could be examined by counting the micronuclei in the binuclear cells that showed only red fluorescence. The MN frequency was defined as the ratio of the number of micronuclei in the binuclear cells to the total number of binuclear cells observed Citation[7].
The ratios obtained in tumors not pretreated with BrdU indicated the MN frequency at all phases in the total (P + Q) tumor cell population. More than 400 binuclear cells were counted to determine the MN frequency. The respective MN frequencies in the unirradiated condition were 0.056±0.005 and 0.081±0.011 for total tumor and Q cell populations.
Clonogenic cell survival assay
The clonogenic cell survival assay was also performed in the mice given no BrdU using an in vivo-in vitro assay method. Tumors were excised from the mice, minced and disaggregated by stirring for 20 min at 37°C in PBS containing 0.05% trypsin and 0.02% EDTA. Through these procedures, single tumor cell suspensions were obtained from the whole tumor. The cell yield was (4.5±1.1)×107/g tumor weight. Appropriate numbers of viable tumor cells from the single cell suspension were plated on 60 or 100-mm tissue culture dishes, and, 12 days later, colonies were fixed with ethanol, stained with Giemsa, and counted. The plating efficiency at 0 Gy was 52.0±4.5%.
The radiosensitivity of Q cells was assessed in terms of the MN frequency using immunofluorescence staining for BrdU. That of the total (=P + Q) tumor cells was determined from the BrdU non-treated tumors based on the MN frequency and clonogenic cell survival.
Three mice were used to assess each set of conditions and each experiment was repeated twice. To examine the differences between pairs of values, Student's t-test was used when variances of the two groups could be assumed to be equal; otherwise the Welch t-test was used. p-values are from two-sided tests.
Results
The clonogenic cell survival curves for total tumor cell populations immediately and 12 h after γ-ray irradiation or carbon ion beam irradiation with various LET values are shown in the left and right panels of , respectively. Apparently, whether immediately or 12 h after irradiation, the surviving fractions (SFs) were much smaller for carbon ions than for γ-rays. Whether immediately or 12 h after carbon ion beam irradiation, as the LET value increased, the SFs decreased.
Figure 1. Cell-survival curves for total tumor cell populations as a function of radiation dose immediately after irradiation and 12 hours after irradiation are shown in the left and right panel, respectively. Open triangles, open squares, solid triangles, and solid squares represent the surviving fractions after irradiation with carbon ion beams having an LET of 18, 43, 50, and 74 keV/µm, respectively. Solid circles represent the surviving fractions after γ-ray irradiation. Bars represent standard errors.
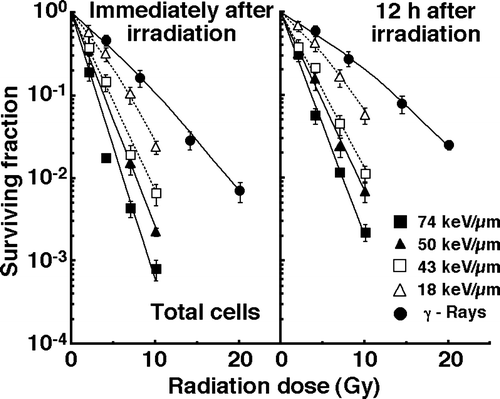
For baseline correction, we used the normalized MN frequency to exclude the MN frequency in non-irradiated control tumors. The normalized MN frequency was the MN frequency in the irradiated tumors minus that in the non-irradiated tumors. The normalized MN frequencies of total or Q cell populations for cells plated immediately and 12 h after γ-ray irradiation or carbon ion beam irradiation with various LET values are shown in and , respectively. In both and 3, the data for total and Q cells are shown in the left and right panels, respectively. Overall, the normalized MN frequencies were lower for Q cells than for the total cell population under all irradiation conditions. In both total and Q cell populations, whether plated immediately or 12 h after irradiation, the normalized MN frequencies were significantly larger for carbon ions than for γ-rays (p<0.05).
Figure 2. Dose-response curves of normalized micronucleus (MN) frequency for total and quiescent cell populations as a function of radiation dose immediately after irradiation are shown in the left and right panel, respectively. Open triangles, open squares, solid triangles, and solid squares represent the normalized MN frequencies after irradiation with carbon ion beams having an LET of 18, 43, 50, and 74 keV/µm, respectively. Solid circles represent the normalized MN frequencies after γ-ray irradiation. Bars represent standard errors.
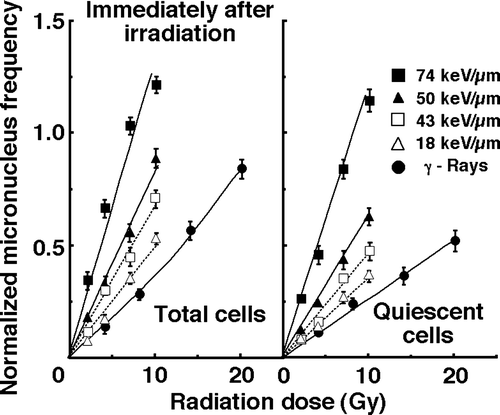
Figure 3. Dose-response curves of normalized micronucleus (MN) frequency for total and quiescent cell populations as a function of radiation dose 12 hours after irradiation are shown in the left and right panel, respectively. Open triangles, open squares, solid triangles, and solid squares represent the normalized MN frequencies after irradiation with carbon ion beams having an LET of 18, 43, 50, and 74 keV/µm, respectively. Solid circles represent the normalized MN frequencies after γ-ray irradiation. Bars represent standard errors.
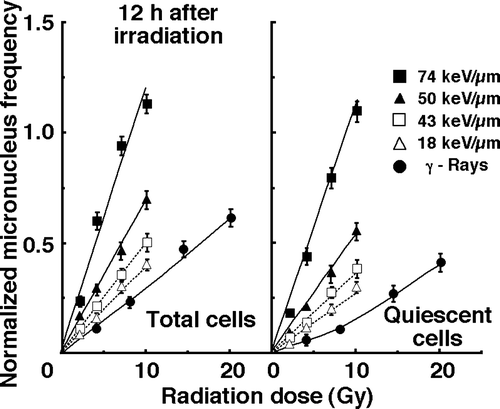
To evaluate the relative biological effectiveness (RBE) of the carbon ion beams with various LET values in both total and Q cell populations compared with γ-rays, the data given in were used (). On the whole, the values of RBE for Q cells and 12 h after irradiation were larger than those for the total cell population and immediately after irradiation, respectively. In both total and Q cell populations, as the LET value increased, namely, as the irradiated point became deeper within the SOBP of the carbon ion beam, the values of RBE also increased.
Table I. Relative biological effectiveness of carbon ion beams* compared with γ-rays in total and quiescent populations.
To assess the degree of the repair from damage induced by γ-ray or carbon ion beam irradiation in total and Q cell populations during the 12 h between the end of irradiation and the beginning of the assay, dose-modifying factors (DMFs) were calculated in both cell populations at various endpoints using the data given in (). In general, whichever form of radiation was employed, the repair was more marked in Q cells than in the total cell population. However, carbon ion beams, especially with high LET values, namely, deep within the SOBP, efficiently suppressed the repair in both total and Q cell populations. Actually, the 43, 50 and 74 keV/µm carbon ion beams gave significantly lower DMFs than the γ-rays (p<0.05) (Table II).
Table II. Dose-modifying factors after repair of potentially lethal damage.*
To examine the difference in sensitivity between the total and Q cell populations, the DMFs under each set of irradiation conditions, which compare the radiation doses necessary to obtain a normalized MN frequency of 0.25 in Q cells with those in total cells, were calculated using the data in and (). Carbon ion beams, especially with high LET values, namely, deep within the SOBP, efficiently reduced the difference in sensitivity. Actually, the 50 and 74 keV/µm carbon ion beams gave significantly lower DMFs than the γ-rays (p<0.05) (Table III). This decrease in the difference was comparatively more remarkable at 12 hours after irradiation than immediately after irradiation.
Table III. Dose-modifying factors for quiescent cells relative to total tumor cells.*
Discussion
It is true that the values of RBE may be substantially depend on radiation quality (LET), radiation dose, number of dose fractions, dose rate and biologic system or end-point, including the kind of irradiated cells, tumors and tissues Citation[11]. However, concerning the RBE values obtained in this study, as a whole, those of carbon ion beams in Q cells were significantly larger than those in total cells (Table I), reflecting the finding that Q cells showed significantly and relatively lower sensitivity than total cells under γ-ray irradiation and under carbon ion beams, respectively. Twelve hours had already been shown to be long enough to repair the initial radiation-induced damage after γ-ray irradiation, and the capacity for PLD repair was also shown to be greater in Q cells than in the total cell population Citation[12]. Therefore, in both cell populations, the RBE values of carbon ion beams at 12 h after irradiation were larger than those immediately after irradiation. Furthermore, not only in total cells but also in Q cells, since the sensitivity to carbon ion beams became higher as the LET value increased, that is, as the irradiated point became deeper in the SOBP of the carbon ion beam, the RBE values also became larger with the LET value. This means precise treatment planning for targeting tumors is essentially required. Otherwise, severe complications are potentially brought about in normal tissues around the target tumors.
The current study showed that the difference in radiosensitivity between the total and Q cells populations was markedly reduced by using carbon ion beams, especially when high LET beams were given (Table III). Although our previous report showed that Q cells have a much larger hypoxic fraction than the total tumor cell population Citation[7], the sensitivity to carbon ion beams of the total tumor cell population was similar to that of Q cells. That is to say, it follows that oxygenated and hypoxic cells in SCC VII solid tumors have almost the same radiosensitivity to carbon ion beams. Further, in this study, PLD repair by Q cells was suppressed strongly with carbon ion beams, especially high LET beams (Table II). Because of less difference in radiation sensitivity among cell cycles and a lesser capacity for PLD repair when carbon ion beams, especially high LET beams, were used, the values of RBE in delayedly excised Q tumor cells are the largest in . These newly revealed radiobiological advantages concerning the responses of intratumor Q cell populations provided us with another rationale for high LET radiation therapy. Including these biological advantages, since a very precise dose of radiation can be deposited in deep-seated tumors using SOBP Citation[1], carbon ion beam radiotherapy has been tried in the clinic since 1994 in Japan Citation[8]. The clinical trials revealed that carbon ion radiotherapy provided definite local control and offered a survival advantage without unacceptable morbidity in a variety of refractory tumors that were hard to cure using conventional treatment modalities Citation[8].
On the other hand, there is clearly heterogeneity in the response of solid tumor tissue to conventional low LET radiation therapy Citation[6]. Factors which cause the heterogeneity in laboratory systems include: the distribution of inherent cellular radiation sensitivity; sensitivity of cells to irradiation in vivo as affected by the distribution of pO2 and other metabolites; cellular capacity to repair radiation damage; cell proliferation kinetics; immune-basal rejection by the host against tumor; extent of loss of tumor clonogens by exfoliation from the surface or into the vascular spaces, etc Citation[6]. Furthermore, it is thought that the quiescent state of cells in solid tumors arises because of oxygen and nutrient deprivation caused by the heterogeneity in vivo within solid tumors Citation[6]. In this study, the radiation with carbon ion beams potentially reduced the difference in radiosensitivity between total and Q cell populations in solid tumors. That is to say, carbon ion beam radiotherapy has the potential to overcome the heterogeneity in solid tumors very efficiently because of its biological advantages Citation[11].
Incidentally, in this study, 74 keV/µm was the largest LET value. When helium is used as an ion beam, it was shown that the RBE value is largest when the LET value is around 100 keV/µm Citation[11]. In other words, the RBE value decreases as the LET value increases over 100 keV/µm. Thus, in future, we would like to check the change in RBE with the increase in LET over 100 keV/µm when carbon ion beams are employed. In addition, we also plan to examine the responses of intratumor Q cell populations to much lower dose-rate irradiation under conditions where sublethal damage is supposed to be repaired during irradiation, compared with those of total tumor cell populations.
Solid tumors, especially human tumors, are thought to contain a high proportion of Q cells Citation[6]. The presence of these cells is probably due, in part, to hypoxia and the depletion of nutrition in the tumor core, and this is another consequence of poor vascular supply Citation[6]. This might promote the formation of micronuclei in Q tumor cells in non-irradiated control tumors. Due to the findings that Q cell population has less sensitivity, a greater PLD repair capacity, and a larger hypoxic fraction than P cell population in vivo Citation[7], more Q cells can survive after radiation therapy than P cells. Meanwhile, it should also be noted that the role of Q cells as determinants of treatment success or failure is not fully established, and their impact may vary from tumor to tumor. It is thought that during fractionated radiotherapy, the Q cell population substantially decreases because of recruitment from Q to P status in vivo due to preferential death of P cells Citation[13]. However, even after fractionated radiotherapy, Q cell population still remains as long as solid tumors cannot fully regress to an extent where Q status cannot be present. Consequently, the control of Q cells has a great impact on the outcome of radiation therapy. As shown here, as the irradiation point was deeper within the SOBP, namely, as the LET value was larger, the difference in sensitivity between the total and Q cell populations decreased, and the capacity for PLD repair and the RBE value in both total and Q cell populations decreased and increased, respectively. In terms of the tumor cell-killing effect as a whole, including intratumor Q cell control, carbon ion beam radiotherapy can be regarded as a very promising treatment modality for deep-seated refractory tumors because of its very efficient cytotoxic effect on intratumor Q cell populations particularly at a deeper point within the SOBP of the carbon beams and its advantageous potential to deposit radiation dose very precisely using SOBP.
Acknowledgements
This study was supported, in part, by a Grant-in-aid for Scientific Research (C) (18591380) from the Japan Society for the Promotion of Science.
References
- Schulz-Ertner D, Jaekel O, Schlegel W. Radiation therapy with charged particles. Semin Oncol 2006; 16: 249–59
- Todd P, Wood JC, Walker JT, Weiss SJ. Lethal, potentially lethal, and nonlethal damage induction by heavy ions in cultured human cells. Radiat Res 1985; Suppl 8: S5–S12
- Blakely EA, Tobias CA, Yang TC, Smith KC, Lyman JT. Inactivation of human kidney cells by high-energy monoenergetic heavy- ion beams. Radiat Res 1979; 80: 122–60
- Palayoor ST, Humm JL, Atcher RW, Hines JJ, Macklis RM. G2M arrest and apoptosis in murine T lymphoma cells following exposure to 212Bi alpha particle irradiation. Nucl Med Biol 1993; 20: 795–805
- Kagawa K, Inoue T, Tokino T, Nakamura Y, Akiyama T. Overexpression of GML promotes radiation-induced cell cycle arrest and apoptosis. Biochem Biophys Res Commun 1997; 241: 481–5
- Vaupel P. Tumor microenvironmental physiology and its implications for radiation oncology. Semin Radiat Oncol 2004; 14: 197–275
- Masunaga S, Ono K. Significance of the response of quiescent cell populations within solid tumors in cancer therapy. J Radiat Res 2002; 43: 11–25
- Tsujii H, Mizoe JE, Kamata T, Baba M, Kato S, Kato H, et al. Overview of clinical experiences on carbon ion radiotherapy at NIRS. Radiother Oncol 2004; 73(Suppl 2)S41–S49
- Masunaga S, Ono K, Suzuki M, Nishimura Y, Kinashi Y, Takagaki M, et al. Radiosensitization effect by combination with paclitaxel in vivo including the effect on intratumor quiescent cells. Int J Radiat Oncol Biol Phys 2001; 50: 1063–72
- Torikoshi M, Minohara S, Kanematsu N, Komori M, Kanazawa M, Noda K, et al. Irradiation system for HIMAC. J Radiat Res 2007; 48(Suppl)A15–A25
- Hall EJ. Linear energy transfer and relative biologic effectiveness. Radiobiology for the Radiologist6th ed, EJ Hall. Lippincott Williams & Wilkins. 2006; 106–116
- Masunaga S, Ono K, Abe M. Potentially lethal damage repair by quiescent cells in murine solid tumors. Int J Radiat Oncol Biol Phys 1992; 22: 973–8
- Masunaga S, Ono K, Mitsumori M, Abe M. The alteration of radiosensitivity of quiescent cell populations in murine solid tumors irradiated twice at various intervals with x-rays. Jpn J Cancer Res 1993; 84: 1130–5