Abstract
Background and purpose. Compared with conventional 3D conformal radiotherapy (3D-CRT) the use of intensity-modulated radiation therapy (IMRT) has increased monitor units (MUs) in the delivery of prescribed dose to the patient and thus a potential risk of radiation-induced secondary cancer. Due to the elimination of the leaf-sequencing step in direct aperture based IMRT optimisation (DABO) the MUs in the beam delivery can be reduced. We compared MUs calculated by DABO with other IMRT techniques and 3D-CRT. Material and methods. Treatment plans for five head and neck cancer patients using dynamic IMRT technique (DMLC) and step-and-shoot (SMLC) technique (Varian Helios Cadplan), 3D-CRT (Varian Eclipse) and a home-made DABO were produced. The total number of MUs, dose coverage and standard deviation of prescribed dose in planning target volume (PTV) between different techniques were compared. Results. In all patients the PTV coverage and sparing of critical structures between the DABO, Helios DMLC and SMLC IMRT techniques was equivalent. Average MUs for beam delivery were 883 MU, 683 MU, 379 MU and 411 MU for DMLC, SMLC, DABO and 3D-CRT, respectively. Conclusions. The DABO IMRT technique is able to produce treatment plans equivalent in target coverage, dose uniformity and normal tissue sparing compared with the commercial IMRT techniques. The number of MUs with DABO were reduced with a factor of 2.3 (DMLC) and 1.8 (SMLC). The study suggests that with DABO IMRT technique the risk of secondary cancer can be reduced without compromise in the quality of treatment plans.
Intensity-modulated radiation therapy (IMRT) is becoming a routine technique in the treatment of several cancers due to improvements in dose conformity in the planning target volume (PTV) and normal tissue sparing. Unfortunately, the delivery of the IMRT treatment fields requires 2 to 5 times more monitor units (MUs) than 3D-conformal radiotherapy (3D-CRT) Citation[1], Citation[2]. An increase in the number of MUs is correlated with an increase in out-of-field radiation dose Citation[3]. Hall and Wuu Citation[4] estimated that if the number of MUs in IMRT is increased with a factor of 2–3 compared with 3D-CRT, IMRT is likely to almost double the incidence of second malignancies for patients surviving 10 years. The majority of second tumours are observed in the volume receiving <6 Gy Citation[5] thus in the areas that are usually not in the treatment field. Therefore, minimizing dose to distant sites could be significant in reducing the risk of second tumours Citation[6].
In most IMRT inverse planning systems the intensity distributions of the treatment fields are optimised. The intensity distributions are then reproduced with a multileaf collimator (MLC) using a separate leaf sequencing algorithm. Due to the separation of intensity optimisation and leaf sequencing, a large number of small subfields are required to realize the optimised beam intensities. Generally, as the number of subfields increases, the number of MUs and thus the out-of-field dose increases. A number of groups have investigated techniques to reduce the number of MUs needed for the MLC field segmentation Citation[7], Citation[8]. Also direct aperture based optimisation methods with IMRT have been studied in order to reduce the number of beam segments and number of MUs Citation[9–11].
We present a direct aperture based optimisation (DABO) where the number of MUs required to achieve comparable dose distribution with commercial IMRT techniques is greatly reduced. The technique has also the advantage that the calculation of intensity distributions as an intermediate step is not required Citation[12].
Material and methods
Direct aperture based optimisation (DABO)
The theoretical background of the present DABO technique has been already described Citation[12–15]. Briefly, the algorithm for the control of MLC is based on the use of an explicit dose function and inverse calculation based on the MLC parameters. Since the calculation of intensity distributions as an intermediate step is not needed the physical limitations of the MLCs can be implemented. Furthermore, the head scattering, leakage and “tongue and groove” effect can be accurately modelled by the DABO method Citation[12].
The DABO formalism is based on the use of pencil beam model for the dose calculation. The intensity distributions generated by the MLC are modified with the help of Heaviside function H. The dose model for one leaf pair can be modelled as
where x is a point in a patient space, D(x) the dose in the point x, w the width of the treatment field, δ t radiation beam-on time and h(x,u) the dose deposition kernel. H(u-a) and H(b-u) are the modified Heaviside functions for the left and right leaves Citation[12]. Superposition of all leaf-pairs is used to calculate the total dose.
Prior to the direct aperture optimisation the treatment fields are set and dose limits for the PTV and organs at risk (OAR) are defined. Additionally, the user determines the minimum amount of MUs for each subfield and the maximum number of subfields for one field. Before the start of the optimisation, the predefined shapes of the apertures are generated using the beams-eye-view (BEV) of the PTV and the OARs for each beam angle. During the optimisation the feasible solution for the MLC leaf positions and the irradiation times of the subfields are calculated. The dose deposition kernel is computed for the subfields using triple Gaussian pencil beam and MLC scattering models. Nonlinear inequalities for leaf positions and irradiation times are solved by using the quasi-Newton method (L-BFGS-B) Citation[12]. After the optimisation, a final dose calculation is performed using the optimised aperture shapes and aperture weights.
In the present investigation, a step-and-shoot MLC (SMLC) feature of the DABO optimisation was applied although the technique can be applied with the dynamic MLC (DMLC) treatment delivery Citation[16]. Also the tongue and groove effect was not modelled by the optimization in this study. The DABO optimisation was implemented in the commercially available treatment planning system (Cadplan, Varian, Palo Alto, CA).
Treatment planning
Dose distributions of five successive patients with head and neck cancer were optimised with DABO and calculated. The plans were compared with treatment plans generated by conventional 3D-CRT, SMLC and DMLC techniques from Cadplan Helios (LMC-version 6.3.5). The IMRT plans were generated using Cadplan treatment planning system (version 6.47) with 4 MV photons and MLC with 40 leaf-pairs.
In all IMRT treatment plans (DABO, Helios DMLC and SMLC) equal number of beams arranged with the same beam angles were used. The number of treatment fields per patient ranged from 6 to 8. The 3D-CRT plans were generated by using the standard technique of the clinic.
For dose objectives, the minimum dose to the PTV was set to be greater than 98% and the maximum dose less than 105% of the prescribed dose. The OARs specified were spinal cord, parotid glands, mandible, mucous membrane and lungs (if the target volume extended to the lower neck). The maximum dose for the spinal cord was set to 48 Gy. For other OARs dose volume constraints were used. The additional constraints in the DABO optimisation were a minimum of 3 MUs for one subfield and a maximum number of 9 subfields for a treatment field. The plans were generated using a leaf position tolerance of 2 mm and the minimum leaf gap of 5 mm. With Helios SMLC the leaf motion calculation was performed with 9 intensity levels to ensure the adequate agreement between optimal and actual fluences Citation[17], Citation[18].
Treatment plan analysis
Dose-volume histograms (DVH) of the PTV and the OARs were calculated to compare the dosimetric quality of the 3D-CRT and IMRT plans. PTV coverage was estimated with D95 (the minimum dose covering 95% of the PTV). The dose uniformity was assessed by calculating the standard deviation (SD) of the doses within the PTV. The number of total MUs obtained for each method was compared. Additionally, the number of subfields created by SMLC and DABO was examined.
Results
Dose distributions with different IMRT techniques were almost identical which can be seen in . The sparing of critical structures was also comparable as seen from DVHs in . For simplicity, the DVHs of SMLC are left out from the graph. The average maximum and the average mean PTVs doses for DMLC, SMLC, DABO and 3D-CRT were 108.0%, 111.4%, 109.3%, 111.1% and 99.2%, 99.7%, 99.0% and 99.3% respectively. All the planned doses were normalized so that the median dose was 100%, corresponding to 56 Gy.
Figure 1. Comparison of isodose distributions for a head and neck patient using DMLC, SMLC, DABO and 3D-CRT. The median doses of the PTVs were set to 56 Gy (normalized to 100%).
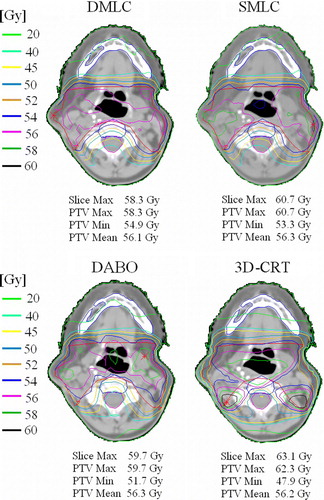
Figure 2. DVH for PTV, spinal cord and mandibula between DMLC (
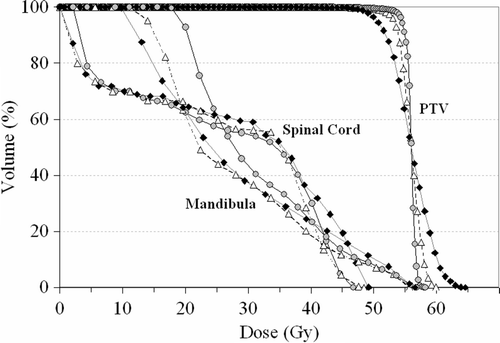
The total MUs of all the treatment plans are presented in . On average, the MUs created by DABO were reduced by a factor of 2.3 and 1.8 compared to Cadplan Helios DMLC and SMLC respectively. The number of MUs was smaller with DABO than with 3D-CRT by a factor of 1.1.
Table I. Comparison of the number of MUs needed to deliver a dose of 2 Gy to PTV and the corresponding SDs and D95s.
The evaluated dose uniformity (SD) and PTV coverage (D95) are illustrated in . The SD was smallest for DMLC as expected. The D95 values were comparable with the different IMRT techniques and largest for the 3D-CRT. The differences between the IMRT and 3D-CRT treatment plans were clear for the advantage of IMRT plans. The number of subfields generated by DABO was on the average 53% smaller than with Helios SMLC ().
Table II. Comparison between the number of subfields between SMLC and DABO.
Discussion
Although the risk of secondary malignancy after head and neck radiotherapy is small, the risk is increasing with longer survival times of the cancer patients, especially in children treated with radiotherapy. In the investigated commercial IMRT techniques the number of MUs was more than two-fold compared with conventional 3D-CRT and DABO (). The increase in MUs leads to higher head leakage and scatter Citation[3]. This is expected to increase radiation induced malignancies Citation[19]. The present investigation supports the view that with the DABO technique the MUs and related risk of secondary malignancy stay at the level typical with 3D-CRT.
The results indicate that all IMRT techniques yielded a more uniform coverage of PTV than a 3D-CRT technique. With respect to PTV coverage the DMLC technique performed better than the step-and-shoot delivery methods (SMLC and DABO) (). The main drawback of the DMLC technique is the highest MUs among the tested techniques. The reduction of MU (57%) by DABO was similar with other direct aperture based optimisations. Reductions of MUs by 80% Citation[11], 33% Citation[20] and 42% Citation[10] are reported when compared to Corvus and Eclipse treatment planning systems or pencil-beam based optimisation, respectively.
The average number of subfields with DABO technique was 32, which is markedly less than 67 with Cadplan Helios SMLC. This is due to the separation of the optimisation into the optimisation of the beam intensities and leaf sequencing step. With other direct aperture based optimisation methods the number of subfields has been 34 Citation[10] and 21 Citation[11] when investigated with a head and neck patient examples.
We conclude that the target coverage and the normal tissue sparing are comparable for DABO and conventional IMRT plans. However, the number of MUs are 45 and 57% lower for the DABO method when compared to SMLC and DMLC, respectively. A linear risk estimate would mean that a risk of radiation-induced second cancer is decreased with a factor of about 2 with DABO compared to the conventional IMRT techniques. Using the aperture based IMRT optimisation the risks of radiation induced second tumours can be kept at the level typical with 3D-CRT without compromise in the quality of treatment plans.
Acknowledgements
This research was supported by the Finnish Funding Agency for Technology and Innovation and Varian Medical Systems Finland Ltd.
References
- Kry SF, Salehpour M, Followill DS, Stovall M, Kuban DA, White RA, et al. The calculated risk of fatal secondary malignancies from intensity-modulated radiation therapy. Int J Radiat Oncol Biol Phys 2005; 62: 1195–203
- Zelefsky MJ, Fuks Z, Happersett L, Lee HJ, Ling CC, Burman CM, et al. Clinical experience with intensity modulated radiation therapy (IMRT) in prostate cancer. Radiother Oncol 2000; 55: 241–9
- Sharma DS, Animesh, Deshpande SS, Phurailatpam RD, Deshpande DD, Shrivastava SK, et al. Peripheral dose from uniform dynamic multileaf collimation fields: Implications for sliding window intensity-modulated radiotherapy. Br J Radiol 2006; 79: 331–5
- Hall J, Wuu S. Radiation induced second cancers: The impact of 3D-CRT and IMRT. Int J Radiat Oncol Biol Phys 2003; 56: 83–8
- Dörr W, Herrmann TJ. Cancer induction by radiotherapy: Dose dependence and spatial relationship to irradiated volume. Radiol Prot 2002; 22: A117–A121
- Lindsay KA, Wheldon EG, Deehan C, Wheldon TE. Radiation carcinogenesis modelling for risk of treatment-related second tumours following radiotherapy. Br J Radiol 2001; 74: 529–36
- Crooks SM, McAven LF, Robinson DF, Xing L. Minimizing delivery time and monitor units in static IMRT by leaf-sequencing. Phys Med Biol 2002; 47: 3105–16
- Kalinowski T. Reducing the number of monitor units in multileaf collimator field segmentation. Phys Med Biol 2005; 50: 1147–61
- De Gersem W, Claus F, De Wagter C, De Neve W. An anatomy-based beam segmentation tool for intensity-modulated radiation therapy and its application to head-and-neck cancer. Int J Radiat Oncol Biol Phys 2001; 51: 849–59
- Li Y, Yao J, Yao D. Genetic algorithm based deliverable segments optimisation for static intensity-modulated radiotherapy. Phys Med Biol 2003; 48: 3353–74
- Shepard DM, Earl MA, Li XA, Naqvi S, Yu C. Direct aperture optimisation: A turnkey solution for step-and-shoot IMRT. Med Phys 2002; 29: 1007–18
- Kolmonen P. The direct control of the multi-leaf collimator in the inverse problem of radiotherapy treatment planning. PhD thesis 2004; University of Kuopio.
- Kolmonen P, Tervo J, Lahtinen T. Use of the Cimmino algorithm and continuous approximation for the dose deposition kernel in the inverse problem of radiation treatment planning. Phys Med Biol 1998; 43: 2539–54
- Tervo J, Kolmonen P. A model for the control of a multileaf collimator in radiation therapy treatment planning. Inv Probl 2000; 16: 1875–95
- Tervo J, Kolmonen P, Lyyra-Laitinen T, Pinter JD, Lahtinen T. An optimisation-based approach to the multiple static delivery technique in radiation therapy. Ann Oper Res 2003; 119: 205–27
- Tervo J, Lyyra-Laitinen T, Kolmonen P, Boman E. An inverse treatment planning model for intensity modulated radiation therapy with dynamic MLC. Appl Math Comput 2003; 135: 227–50
- Chui CS, Chan MF, Yorke E, Spirou S, Ling CC. Delivery of intensity-modulated radiation therapy with a conventional multileaf collimator: Comparison of dynamic and segmental methods. Med Phys 2001; 28: 2441–9
- Nicolini G, Fogliata A, Cozzi L. IMRT with the sliding window: Comparison of the static and dynamic methods. Dosimetric and spectral analysis. Radiother Oncol 2005; 75: 112–9
- McKenzie A. Should treatment planning include risks of radiation-induced malignancies?. Radiother Oncol 2006; 81: S20–S21
- Bergman AM, Bush K, Milette M-P, Popescu IA, Otto K, Dunzenli C. Direct aperture optimisation for IMRT using Monte Carlo generated beamlets. Med Phys 2006; 33: 3666–79