Abstract
Materials and methods. The aim was to retrospectively investigate correlations between potential predictive parameters and the occurrence of radiation-induced lung injury in patients with primary or secondary lung tumours treated with stereotactic body radiation therapy (SBRT). Sixty patients (63 tumours) underwent SBRT, with a dose of 45 Gy in 3 fractions over 5 days or 26 Gy in single fraction. The following parameters were tested for correlation with Radiation Therapy Oncology Group (RTOG) lung toxicity score: planning target volume (PTV), tumour location, primary vs. metastatic tumour, and Mean Lung Dose (in 2 Gy fractions, MLD2). Normal Tissue Complication Probability (NTCP) values were then estimated. Results. The median follow-up time was 30.9 months (range 6.7–56.7). RTOG grade 0–1 toxicity was observed in 54/63 (85.7%) and grade 2–3 in 9/63 (14.3%) cases. Mean values of MLD2 for RTOG grade 0–1 and 2–3 were respectively 11.2 Gy (95% Confidence Interval (CI) 10.1–12.3 Gy) and 20.3 Gy (95% CI 16.6–23.9 Gy). NTCP mean values for RTOG grade 0–1 and 2–3 were respectively 4% (95% CI 2–5.9%) and 37% (95% CI 11.6–62.3%). Univariate analysis, performed with t-Student test, showed a statistically significant difference between MLD2 values in the two groups (t = 5.93 and p ≤ 0.001). Logistic regression analysis showed a good correlation between MLD2 and toxicity scores 2–3 (p = 0.008, odds ratio 1.5). From logistic regression relationship between the observed rates of grade 2–3 and MLD2, a D50=19.8 Gy and a γ50=2.2 were obtained. From the sigmoid-shaped dose-response relationship between NTCP and MLD2, a D50=22.4 Gy and γ50=2.2 were derived. Discussion. MLD2 is strongly associated to the risk of lung injury. Higher NTCP values are associated with a higher risk, but when comparing the expected to the observed toxicity rate, NTCP seems to underestimate the risk.
Dose-escalation studies using three-dimensional conformal radiation therapy (3D-CRT) as well as other retrospective series clearly suggest a benefit in terms of tumour control probability when higher radiation doses are delivered in non-small cell lung cancer (NSCLC) Citation[1].
In conventionally fractionated 3D-CRT, major limitations to the use of higher doses are represented by the prolonged overall treatment time and the higher risk of deleterious effects on healthy tissues, such as radiation-induced lung injury. In recent years, these considerations have led to the design of aggressive hypo-fractionated stereotactic body radiation therapy (SBRT) schedules in treating extra-cranial neoplasms, mainly represented by pulmonary tumours. Currently, these approaches are usually reserved for selected early stage NSCLC patients, medically or functionally unfit for surgery, or to patients with lung metastases from different primary tumour sites. Preliminary results from several clinical series in this setting showed excellent outcomes, with local control rates in the range of 80 – 100% Citation[2–7]. 3D conformal planning combined with stereotactic localization using specifically designed body frames allows an adequate localization of tumours. In turn, set-up errors are significantly reduced allowing for smaller treatment fields and a good physical sparing of normal tissues, with a very sharp gradient of dose. Nevertheless, the risk of pulmonary toxicity in this subgroup of patients, employing previously uninvestigated high doses, remains an open issue. In estimating the risk, the potential selection bias of these patients, who are often not amenable to surgery due to reduced pulmonary function or medical comorbidities, also has to be taken into account. Moreover, an interstitial fibrosis is more likely to occur after radiation pneumonitis (RP), with a subsequent further impairment of pulmonary function. Together with the progressive development of advanced planning and delivery processes to improve radiation treatment conformality, the identification of predictive factors for lung injury in these patients is of significant clinical interest. In this paper, we retrospectively analysed the incidence and grade of lung toxicity in patients treated with SBRT at our Institution, in the context of a mono-institutional study in primary and secondary lung tumours. A statistical analysis was then performed to investigate the potential correlation between different parameters (mainly dosimetric) and lung toxicity.
Materials and methods
Patients’ selection
From May 2003 to June 2006, 60 patients (63 tumours) with primary NSCLC or lung metastases were treated with SBRT. The treatment technique was based on the method first described by Blomgren and colleagues Citation[2]. The eligibility criteria for SBRT were stage I NSCLC (IA and IB less than 5 cm) not amenable with surgery for medical contraindications or patient's refusal, and “oligo” lung metastases (≤ 3), ECOG performance status ≤ 2, and no prior radiation therapy to the site of SBRT. Lesions located less than 2 cm away from major airways or less than 1 cm from major blood vessels were considered not eligible for SBRT. Diagnostic work-up included CT scan (thorax, abdomen, brain) with intravenous contrast medium and 18FDG-PET-CT scan to better evaluate disease extent. Thirty-eight of 41 patients affected by primary NSCLC were assessed by a thoracic surgeon and deemed medically or functionally inoperable because of the presence of significant comorbidities and high surgical risk; three patients refused the proposed surgical approach. The most common clinical comorbidities included chronic obstructive pulmonary disease and age. Twenty-five patients had histologically proven NSCLC. In 16 patients, in whom a fine-needle biopsy was unsuccessful or could not be performed, a clinical proof of malignancy was presumed due to a growing lesion on sequential CT scans, and/or due to positive 18FDG-PET findings (SUV values > 2.5). Nineteen patients had a diagnosis of lung metastases, from different primary tumour sites. Three of 19 had a diagnosis of bilateral disease. Patients and tumours characteristics are listed in . All patients were included in the study and treated according to the recommendations of Helsinki Declaration and subsequent amendments; a written informed consent was obtained from every patient (this study was a part of a prospective phase II trial ongoing at our Institution on thoracic SBRT).
Table I. Patients and tumours characteristics
Treatment technique
Each patient was immobilized in a supine position with an individually fitted vacuum pillow and a stereotactic body frame (SBF, ELEKTA® Oncology System). Set-up was checked on SBF using a laser system and permanent chest and legs skin markers. Tumour breathing motion was studied by fluoroscopy. An abdominal compression device was employed in 13 patients (21.6%), when target volume shifting exceeded 10 mm in a cranio-caudal direction; if the tumour was not visible, we evaluated the diaphragm motion as a surrogate for tumour motion.
Patients underwent a planning CT scan of the entire thorax using 2.5 mm thick slices without intravenous contrast media. Each CT slice was scanned with an acquisition time of 3 seconds to include the whole phase of one respiratory cycle. The target was outlined in sequential axial CT images and the gross tumour volume (GTV) was contoured using a CT lung window setting (1600-400 Hounsfield units) and including parenchymal spiculae. Clinical target volume (CTV) corresponded to GTV, as previously reported Citation[6]. With the help of an automatic 3D contour generation tool (OTP, Nucletron®, The Netherlands), the planning target volume (PTV) was generated adding to the CTV a margin of 5 mm in the axial plane and of 10 mm in the longitudinal direction. Adjacent organs-at-risk (oesophagus, heart, spinal cord, both lungs) were outlined. OTP version 1.5 software (Nucletron®, The Netherlands) was employed for treatment planning. SBRT was delivered with an Elekta Precise® Linear Accelerator using 6 to 8 static non-opposing, non-coplanar shaped photon beams of 6 MV and 10 MV. Three fractions of 15 Gy in 1 week (48 hrs interval, Monday through Friday) prescribed to the PTV-enclosing 80% isodose with normalization to 100% at the isocenter (18.75 Gy) were administered to 41 primary and 17 metastatic tumours, and one fraction of 26 Gy was delivered to five metastatic tumours (32.5 Gy to the isocenter). To take lung heterogeneity into account, the dose distribution was calculated by collapsed cone algorithm. Isocenter location was verified before each treatment session by a visual comparison between two orthogonal (0° and 90°/ 270°) electronic portal images (5x5 cm2 field size) and the corresponding digitally reconstructed radiographs obtained from the treatment planning system. If the set-up error was estimated to be 3 mm or more in any direction, patients were repositioned and portal images acquired and verified again. The mean time for each session was approximately 45 minutes.
Radiation-induced lung injury scoring
Lung toxicity was graded using the RTOG acute radiation toxicity score (for events occurring between day 1 and 90 from the end of radiation treatment) and RTOG late radiation toxicity score (for events occurring after day 90). RTOG scoring system is reported in details in . We did not consider as toxicity the presence of radiological changes without symptoms. All patients have been assessed by the same Radiation Oncologists’ Team during routine clinical and radiological follow-up. All records were accurately evaluated to avoid the risk to confound RT-related toxicities with COPD exacerbations and /or infections. In patients with documented co-morbidities (majority of patients with primary NSCLC and deemed medically inoperable) we considered the diagnosis of RP only if a deterioration of the previous clinical status was clearly evident and another possible diagnosis reasonably excluded.
Table II. RTOG lung toxicity scoring system
Follow-up in the first year included clinical examination, thorax CT scan 45 days after treatment and then every 3 months. After the first year a CT scan every 6 months was planned. CT-PET scan was done 3 and 9 months after treatment in order to add some potential functional information to morphological imaging, mainly in terms of SUV modifications as potential predictors of outcome (this will be part of another investigational research protocol) or as potential contribution in terms of differential diagnosis between tumour re-growth and treatment-induced pulmonary fibrosis.
Mean Lung Dose (MLD) and Normal Tissue Complication Probability (NTCP)
Mean Lung Dose was calculated from ipsilateral lung DVH excluding CTV. The Equivalent Dose in 2 Gy fractions (MLD2) was obtained from the differential DVH's, and all doses to the partial volumes re-scaled using Biological Effective Dose (BED) formula derived from LQ model (with an α/β = 3 Gy for normal lung tissue) Citation[8].
Normal Tissue Complication Probability (NTCP) values for lung were estimated for each case in order to evaluate the validity of NTCP model in SBRT, for comparison with MLD2 and for potential future use in radiobiological modelling.
NTCP was calculated taking into account only the ipsilateral lung. This choice was justified by the low dose received by the contralateral lung in SBRT (steep dose gradient of SBRT plans) in our patients. Lung was thus defined as ipsilateral lung minus CTV. For NTCP calculation a Matlab® program developed at our Institute was employed. The 3D dose and volume matrix from normalized lung DVHs elaborated by Masterplan Treatment Planning System (Nucletron®, The Netherlands) were extracted and NTCP values calculated according to Lyman-Kutcher-Burman (LKB) model Citation[9] for each plan. Assuming an α/β = 3 Gy for the ipsilateral normal lung, we re-scaled the dose axis of the DVH to the Equivalent Dose in 2 Gy fractions according to the LQ model and the method described by Van den Heuvel Citation[7]. To calculate the NTCP value (ipsilateral lung) the following parameters were used: TD50=24.5 Gy, n = 0.87, m = 0.18 Citation[9].
A binary logistic regression analysis was also performed in order to correlate the observed toxicities (grade 0–1 vs. 2–3) with the MLD2 values, according to the method described by Willner and colleagues Citation[10]; from this curve the D50 (dose for 50% complication probability rate) and γ50 (normalized slope of the sigmoid dose-response curve) values were obtained. At this point a gaussian fit was performed in order to correlate the predicted toxicities (by NTCP) and the MLD2 values. The corresponding predicted values of D50 and γ50 were extracted also from this curve Citation[10].
Statistical analysis
The following predictive parameters were considered for the statistical analysis and tested for correlation with RTOG lung toxicity score: PTV, primary vs. metastatic tumours, tumour location (central vs. peripheral, lower vs. median vs. upper lobe), MLD2. Tumour location was defined as peripheral when the CTV external margin was located at a distance of less than 1 cm from the chest wall (pulmonary mantle lesions) and as central in all other cases (excluding lesions close to mediastinal structures as previously specified).
Univariate analysis was performed with 2-way contingency tables using χ2 test; multivariate analysis was performed with logistic regression. A p-value ≤ 0.05 was considered as statistically significant. In the statistical analysis, we did not consider NTCP as separate predictive parameter due to its strong dependence on MLD2.
STATA® Statistical Software (College Station, TX) was employed for data analysis.
Results
All 60 patients (63 tumours) included in the study protocol were considered for analysis. The median follow-up time was 30.9 months (range 6.7–56.7).
RTOG grade 0–1 pulmonary toxicity was observed globally in 54/63 lesions (85.7%), while RTOG grade 2–3 pulmonary toxicity was observed in 9/63 (14.3%); median interval of occurrence of grade 2–3 pulmonary toxicity was 4.8 months (range 1.9–22.4 months) from the treatment.
On the whole cohort, the mean MLD2 was 11.3 Gy (range 3.7–28.5 Gy) and NTCP quartiles were respectively Q25=0.05%, Q50=1% and Q75=9.5% (range 0.05–92%). Mean values of MLD2 in grade 0–1 and 2–3 lung toxicity were respectively 11.2 Gy (95% Confidence Interval (CI) 10.1–12.3 Gy) and 20.3 Gy (95% CI 16.6–23.9 Gy). Univariate analysis, performed with t-Student test, showed a statistically significant difference between MLD2 mean values in the two groups (grade 0–1 vs. grade 2–3), with t = 5.93 and p ≤ 0.001. Mean values of the predicted NTCP in RTOG grade 0–1 and 2–3 lung toxicity were respectively 4% (95% CI 2–5.9%) and 37% (95% CI 11.6–62.3%) ().
Table III. MLD2 and NTCP mean values according to RTOG lung toxicity score
Pearson's test did not show any correlation between tumour location (peripheral vs. central, χ2=4.7, p = 0.2, and upper vs. medium vs. lower lobe, χ2=9.12, p = 0.17) and the occurrence of RTOG grade 2–3 lung toxicity. Similar results were found when investigating a potential correlation between primary vs. metastatic tumours (χ2=2.08, p = 0.55) and RTOG grade 2–3 pulmonary toxicity.
Logistic regression analysis () showed a good correlation between MLD2 and RTOG lung toxicity scores 2–3 (p = 0.008, odds ratio 1.52), and no other statistically significant correlation with other parameters.
Table IV. Logistic regression analysis (correlation with RTOG grade 2-3 pulmonary toxicity)
The graph shown in represents the observed grade 2–3 lung toxicity frequency, divided in 3 Gy MLD2 intervals; the dotted line represents the logistic regression relationship between the observed rates of grade 2–3 lung toxicity and MLD2. D50 and γ50 calculated values were 19.8 Gy and 2.2.
Figure 1. MLD2, NTCP and observed lung toxicity for ipsilateral lung. The histogram shows the percentage of RTOG grade 2–3 toxicity (the values in the bars indicate the number of patients with grade 2–3 toxicity over the total number of patients at risk within the same MLD range), while the dotted line the binary logistic fitting of observed data; the continuous line represents the gaussian fit between NTCP and MLD2.
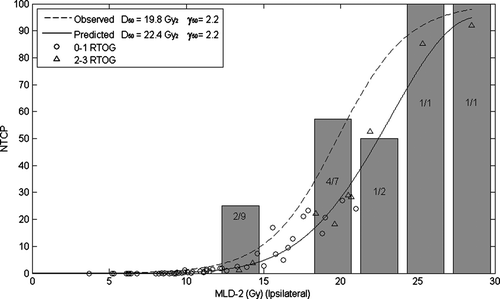
From the sigmoid-shaped dose-response relationship between NTCP (predicted toxicity) and MLD2, the D50 and γ50 calculated values were 22.4 Gy and 2.2.
Discussion
Radiation pneumonitis is a relatively common event in conventionally fractionated 3D-CRT for lung cancer; when delivering total doses up to 60–66 Gy, its reported incidence is in the range 10–20%. In SBRT, the achievable high grade of conformality drastically reduces the amount of irradiated normal lung, but the aggressive hypo-fractionation schedules generally employed could potentially influence the risk of toxicity. Extremely heterogeneous regimens have been tested and different toxicity scales (NCI-CTC, SWOG, and RTOG) employed in phase II trials, with a consequent difficult comparison between different published studies Citation[3–7].
In a recent series by Guckenberger and colleagues Citation[11], clinically symptomatic radiation pneumonitis (graded with NCI-CTC score) was observed in 10% of patients, even if only 5/10 required a specific therapy.
As pointed out by Kocak and colleagues Citation[12], in thoracic radiation therapy (conformal or stereotactic) there are some concerns in defining radiation lung injury, mainly because of the common presence of confounding medical conditions. The time onset of radiation acute/sub-acute clinical toxicity seems to be different between SBRT and 3D-CRT (longer after SBRT, as confirmed by our report, with a median interval of occurrence of 4.8 months), while the radiological presentation is similar. An interesting finding of the previously cited study Citation[11] is that, after 6 months, the proportion of patients with no evidence of radiological changes after SBRT is only 22%, suggesting a lack of correlation between radiological changes and the occurrence of clinically symptomatic lung injury.
Considering lung function, in a phase I study by Timmerman and colleagues Citation[6], including 37 patients in a dose-escalation protocol, ten patients treated with SBRT showed a 10% decline in at least one of the evaluated pulmonary function tests (FEV1, FVC, DLCO), while six patients showed clinical symptoms related to radiation-induced lung injury. Ohashi and colleagues did not demonstrate an alteration in pulmonary function one year after SBRT when considering TLC, FVC, FEV 1.0, and DLCO Citation[13].
Paludan and colleagues did not show any worsening of clinical symptoms after SBRT in patients with pulmonary comorbidities, with a minimal impact on dyspnea aggravation Citation[14].
To our knowledge, a few data are currently available on the correlation between dose-per-fraction, total dose, dose-volumetric predictive factors and radiation-induced lung injury in patients treated with SBRT. NTCP, MLD, and dose-volume parameters (Vdose) have instead been intensively investigated in 3D-CRT.
In a systematic review by Rodrigues and colleagues Citation[15] on 3D-CRT toxicity in lung cancer, MLD, NTCP, and Vdose resulted to be predictive, even with low sensitivities, in the range of 50–75% for Vdose, 19–36% for MLD, and 0 – 44% for NTCP. MLD was significantly associated with RP risk in studies by Yorke and colleagues (ipsilateral, lower lung, and total lung) Citation[16] and by Hernando and colleagues, where a MLD < 10 Gy was associated to a 10% radiation pneumonitis rate, 11–20 Gy to 16%, 21 – 30 Gy to 27%, and > 30 Gy to 44.5% Citation[17]. In the analysis performed by Graham and colleagues, which considered the lungs as a paired organ, an increasing rate of toxicity was associated to progressively higher values of MLD (without a statistically significant correlation), while V20 was selected as the most significant dosimetric predictive parameter Citation[18].
In 3D-CRT, NTCP is usually estimated by considering the lungs as a paired organ excluding CTV or PTV, with the assumption that NTCP follows a sigmoid dose-response relationship. Rancati and colleagues did not detect a significant correlation between NTCP and RP in univariate analysis Citation[19], and a recent update of the Memorial Sloan-Kettering Cancer Centre dose-escalation trial indicates that MLD and NTCP, as whole-lung dosimetric variables, are predictive of lung injury Citation[20]. Hernando and colleagues Citation[17] and Armstrong and colleagues Citation[21] detected a significant correlation between NTCP and lung injury risk, and a NTCP value of 12% was defined as cut-off. Marks reported that higher sensitivity and accuracy are achievable for NTCP when patients with very poor pre-RT pulmonary function are excluded from analysis Citation[22]. A possible explanation is that patients with very low pre-RT pulmonary function are more likely to develop non-radiation-related symptoms Citation[19].
A Vdose parameter equivalent to V20 or V13 in 3D-CRT has not been previously defined for SBRT, and there are still few data available on the predictive value of MLD and NTCP when highly hypo-fractionated regimens are employed. Song and colleagues reported retrospectively that when NTCP values (calculated on both lungs excluding CTV) are below 20%, the risk of pulmonary toxicity is negligible Citation[23], and personal communication]. Kontrisova and colleagues, comparing lung dosimetry in SBRT in different respiration conditions (free breathing, deep inspiration and expiration breath hold), estimated NTCP with LKB model and correction for fractionation, similarly to us, and generally obtained very low NTCP values (only 5 patients of 13 with NTCP > 1%) Citation[24].
In our series, we did not observe any severe pulmonary toxic event when MLD2 was below 12 Gy. Higher NTCP values were associated with a higher risk, but when comparing the predicted toxicity rate with the observed rate, the corresponding NTCP model seemed to underestimate the risk (D50 22.4 Gy vs.19.8 Gy), while the steepness of the two dose-response relationships (γ50 values) resulted to be equal. Probably if we considered for the NTCP calculation both lungs, NTCP values would have been further lower, increasing the risk of underestimation. These results are partially in contrast with those obtained by Willner and colleagues on MLD and NTCP (calculated on ipsilateral lung) as predictive factors in conventionally fractionated 3D-CRT Citation[9]. In their study NTCP overestimated the risk of lung injury, but, very interestingly, the predicted D50 and γ50 values obtained from dose-response relationships were very similar to ours.
With little clinical data currently available, MLD2 seems to be a useful parameter in predicting risk of lung injury in the clinical decision making process, appearing as a well suited parameter to be used at the stage of treatment planning. The possibility to fit clinical toxicity data with MLD2, generating an experimental dose-response relationship for SBRT could be useful in comparing results from previously published studies and in obtaining parameters for toxicity risk prediction in SBRT. Due to the low number of major toxic events, the related uncertainty of this prediction is still relatively large, and we think that to confirm our findings and to definitively validate MLD2 as a standard predictive parameter an analysis of toxicity data from other prospective trials in thoracic SBRT is needed. In any case, in our clinical activity, we are currently employing MLD2 as dose constraint for pulmonary toxicity.
Acknowledgements
The Authors would like to thank Giovanna Gagliardi, at Karolinska University Hospital, Stockholm, for her precious suggestions and for helping in reviewing the manuscript. The Authors declare that they do not have any potential conflict of interest in connection with the material described.
References
- Kong FM, Ten Haken RK, Schipper MJ, Sullivan MA, Chen M, Lopez C, et al. High-dose radiation improved local tumour control and overall survival in patients with inoperable/unresectable non-small cell lung cancer: Long-term results of a radiation dose escalation study. Int J Radiat Oncol Biol Phys 2005; 63: 324–33
- Blomgren H, Lax I, Naslund I, Svanstrom R. Stereotactic high dose fraction radiation therapy of extracranial tumours using an accelerator; clinical experience on the first thirty-one patients. Acta Oncol 1995; 34: 861–70
- Wulf J, Haedinger U, Oppitz U, Thiele W, Mueller G, Flientje M. Stereotactic radiotherapy for primary lung cancer and pulmonary metastases: A non-invasive treatment approach in medically inoperable patients. Int J Radiat Oncol Biol Phys 2004; 60: 186–96
- Onishi H, Araki T, Shirato H., Nagata Y, Hiraoka M, Gomi K, et al. Stereotactic hypofractionated high-dose irradiation for stage I non small cell lung carcinoma. Cancer 2004; 101: 1623–31
- Nagata Y, Takayama K, Matsuo Y, Norihisa Y, Mizowaki T, Sakamoto T, et al. Clinical outcomes of a phase I/II study of 48 Gy of stereotactic body radiotherapy in 4 fractions for primary lung cancer using a stereotactic body frame. Int J Radiat Oncol Biol Phys 2005; 63: 1427–31
- Timmerman R, Papiez L, McGarry R, Likes L, DesRosiers C, Frost S, et al. Extracranial stereotactic radioablation: Results of a phase I study in medically inoperable stage I non-small cell lung cancer. Chest 2003; 124: 1946–55
- Zimmermann FB, Geinitz H, Schill S, Thamm R, Nieder C, Schratzenstaller U, et al. Stereotactic hypofractionated radiotherapy in stage I (T1-2 N0 M0) non-small-cell lung cancer (NSCLC). Proceedings of the 3rd Acta Oncologica Symposium on Stereotactic Body Radiotherapy, June 15-17, 2006, Copenhagen, Denmark. Acta Oncol 2006; 45: 771–994
- Van den Heuvel F. Decomposition analysis of differential dose volume histograms. Med Phys 2006; 33: 297–307
- Burman C, Kutcher GJ, Emami B, Goitein M. Fitting of normal tissue tolerance data to analytic function. Int J Radiat Oncol Biol Phys 1991; 21: 123–35
- Willner J, Jost A, Baier K, Flentje M. A little to a lot or a lot to a little? An analysis of pneumonitis risk from dose-volume histogram parameters of the lung in patients with lung cancer treated with 3D conformal radiotherapy. Strahlenther Onkol 2003; 179: 548–59
- Guckenberger M, Heilman K, Wulf J, Mueller G, Beckmann G, Flentje M. Pulmonary injury and tumour response after stereotactic body radiotherapy (SBRT): Results of a serial follow-up CT study. Radiother Oncol 2007; 85: 435–42
- Kocak Z, Evans E, Zhou S, Miller KL, Folz RJ, Shafman TD, et al. Challenges in defining radiation pneumonitis in patients with lung cancer. Int J Radiat Oncol Biol Phys 2005; 62: 635–8
- Ohashi T, Takeda A, Shigematsu N, Kunieda E, Ishizaka A, Fukada J, et al. Differences in pulmonary function before vs. 1 year after hypofractionated stereotactic radiotherapy for small peripheral lung tumours. Int J Radiat Oncol Biol Phys 2005; 62: 1003–8
- Paludan M, Traberg Hansen A, Petersen J, Grau C, Hoyer M. Aggravation of dyspnea in stage I non-small cell lung cancer patients following stereotactic body radiotherapy: Is there a dose-volume dependency? Proceedings of the 3rd Acta Oncologica Symposium on Stereotactic Body Radiotherapy, June 15-17, 2006, Copenhagen, Denmark. Acta Oncol 2006; 45: 771–994
- Rodrigues G, Lock M, D'Souza D, Yu E, Van Dyk J. Prediction of radiation pneumonitis by dose-volume histogram parameters in lung cancer – a systematic review. Radiother Oncol 2004; 71: 127–38
- Yorke ED, Jackson A, Rosenzsweig KE, Braban L, Leibel SA, Clifton Ling C. Dose-volume factors contributing to the incidence of radiation pneumonitis in non-small cell lung cancer patients treated with three-dimensional conformal radiation therapy. Int J Radiat Oncol Biol Phys 2002; 54: 329–39
- Hernando ML, Marks LB, Bentel JC, Zhou SM, Hollis D, Das SK, et al. Radiation induced pulmonary toxicity: A dose-volume histogram analysis in 201 patients with lung cancer. Int J Radiat Oncol Biol Phys 2001; 51: 650–9
- Graham M, Purdy J, Emami B, Harms W, Bosch W, Lockett MA, et al. Clinical dose-volume histogram analysis for pneumonitis after 3D treatment for non-small cell lung cancer (NSCLC). Int J Radiat Oncol Biol Phys 1999; 45: 323–9
- Rancati T, Ceresoli GL, Gagliardi G, Schipani S, Cattaneo GM. Factors predicting radiation pneumonitis in lung cancer patients: A retrospective study. Radiother Oncol 2003; 67: 275–83
- Yorke ED, Jackson A, Rosenzweig KE, Braban L, Leibel SA, Ling CC. Correlation of dosimetric factors and radiation pneumonitis for non-small cell lung cancer patients in a recently completed dose-escalation study. Int J Radiat Oncol Biol Phys 2005; 63: 672–82
- Armstrong J, Raben A, Zelefsky M, Burt M, Leibel S, Burman C, et al. Promising survival with three dimensional conformal radiation therapy for non-small cell lung cancer. Radiother Oncol 1997; 44: 17–22
- Marks LB. Dosimetric predictors of radiation-induced lung injury. Int J Radiat Oncol Biol Phys 2002; 54: 340–7
- Song DY, Benedict SH, Cardinale RM, Chung TD, Chang MG, Schmidt-Ullrich RK. Stereotactic body radiation therapy of lung tumours: Preliminary experience using normal tissue complication probability-based dose limits. Am J Clin Oncol 2005; 28: 591–6
- Kontrisova K, Stock M, Dieckmann K, Bogner J, Potter R, Georg D. Dosimetric comparison of stereotactic body radiotherapy in different respiration conditions: A modelling study. Radiother Oncol 2006; 81: 97–104