Abstract
Purpose. To investigate the impact of using different radiation therapy techniques on contra-lateral breast (CB) dose, and also dose to other involved organs at risk such as heart and lungs following radiation therapy of breast and regional lymph nodes. Furthermore, to predict the risk for induced malignancies in CB using linear and non linear models. Material and methods. Eight patients with stage II-III breast cancer were included in this analysis. It was focused on three treatment techniques; conventional radiotherapy technique forwardly planed, IMRT and volumetric modulated arc (RapidArc) techniques, inversely planed. The CC algorithm was employed to calculate the standard treatment plans whereas for the IMRT and RapidArc treatment plans AAA algorithm was adopted. The dose results based on mostly DVH analysis were compared. The excess relative risk (ERR) for cancer induction in CB, employed both linear and non-linear models, was estimated. Results. A better homogeneity and conformation in PTV was observed in the RapidArc plans. The highest minimum dose to PTV was observed in the conventional plans while no difference was observed for minimum significant doses D98% and D99% where DX% is the dose received by X% of the PTV volume. In terms of organ sparing, the IMRT and RapidArc plans spare ipsilateral-lung better, but a 40% lower mean dose in the contra-lateral lung in the conventional plans is observed. The mean dose to the contra-lateral breast was lowest for the RapidArc plans as well as the V10Gy and the maximum dose. The mean predicted ERR for the eight patients were lower for the conventional and RA plans than for the IMRT plans assuming a linear dose-risk relationship. The mean predicted ERR when using a non linear model was lower for all the three techniques (with lowest ERR for RapidArc plans). Conclusions. From a clinical perspective, it should be concluded that all three solutions investigated in the study can offer high quality treatment of patients. Further comparative analysis of the two algorithms used in the present study, however, should be performed especially on the peripheral organ dose. The impact of CB exposure to a low-dose radiation on minimizing the risk of radiation induced malignancy in CB can be interpreted differently when using linear or non linear models to predict ERR. In general, no detriment was observed when using RapidArc compared to conventional treatments while a potentially higher risk could be associated to IMRT treatments with fixed gantry.
Radiotherapy to the breast/breast wall and regional lymph nodes using 4-5 field conventional technique is commonly given to breast cancer patients in stadium II-III. This treatment will also involve the irradiation of heart, lungs and contralateral breast (CB). One of the aims in modern radiotherapy is to avoid early and late side effects associated with the treatment; this can be achieved by steep dose gradients between the target volume and the surrounding normal tissues. One of the late side effects that have received increasing attention the last years is radiation induced malignancies. In patients treated for breast cancer, the most common secondary cancer is contralateral breast cancer Citation[1–3], accounting for approximately 50% of all second cancers Citation[4]. New treatment techniques are employed to minimize the radiation induced adverse effects without compromising the planned target volume (PTV) dose coverage. One of the relatively new developments in radiation treatment techniques used to improve target dose distribution and spare non-involved organs is intensity modulated radiation therapy (IMRT). IMRT is already applied to several sites such as prostate Citation[5], lung Citation[6], head and neck Citation[7] and breast Citation[8] primaries. Another intensity modulated radiation delivery method is intensity modulated arc therapy (IMAT) with infinite number of beam directions Citation[9–15]. In this article, radiotherapy treatment plans for eight breast cancer patients applying seven fields IMRT and RapidArc (RA), a single arc volumetric IMAT techniques Citation[9–15], are compared with four fields conventional breast radiotherapy technique. RA is a relative new planning and delivery technique based on an idea by K. Otto Citation[16]. It has been developed to at the same time: i) improve OARs and healthy tissue sparing compared to other IMRT solutions; ii) maintain or improve the same degree of target coverage; iii) reduce significantly the treatment time (beam on time) per fraction.
The aim of this comparative study is to investigate the impact of using different radiation therapy techniques on CB dose, as well as other involved organs at risk (OAR) such as heart and lungs. The data on CB dose is further used to predict the risk for induced malignancies in CB employed linear and non linear models as described in an earlier study Citation[17]. The estimated risk results based on dose data by the three techniques included in the present study are further compared and discussed.
Material and methods
Eight patients with stage II–III breast cancer undergoing 4-field post-operative radiotherapy of the primary breast and regional lymph nodes were included in this analysis. All the patients had ablatio mammae before radiotherapy. Radiotherapy was delivered to the chest wall, the infraclavicular, internal mammary and axillary nodes. Three of the included breast cancer patients were left-sided and the rest were right-sided. Radiation therapy planning was based on CT scans covering a region from the 6th cervical vertebra to the middle part of abdomen. The clinical target volume (CTV) and the organs at risk (OARs), such as ipsilateral lung (IPSI-L), heart, contralateral lung (CL) and contralateral breast (CB) were delineated in the planning CT images. The CTV is delineated with 5 mm margin under the body outline. The PTV includes the CTV with one cm margin. All the patients involved in this study received 25 fractions of 2 Gy to the regional lymph nodes and the chest wall, to a total dose of 50 Gy. All plans were designed to achieve the highest target coverage and the lowest dose possible to organs at risk. In practice, the goal was to achieve a maximum dose lower than 105% and minimum dose higher than 90% in planed target volume (PTV). Furthermore, the treatment plans aimed at achieving a dose lower than 20 Gy to the CL and lower than 15 Gy to the CB and the lowest dose possible to IPSI-L and heart.
This study focused on three treatment techniques; conventional radiotherapy technique forwardly planed, IMRT and RA, both inversely planed. The treatment techniques compared in the current study were planed respectively on the following treatment planning systems (TPS): Masterplan Oncentra by Nucletron (version 3.0 SP1) for the conventional plan, Eclipse by Varian for IMRT (release 7.5.14.3) and for RA (8.6.05). The photon beam energy was 6 MV for all the treatment plans, using a Varian Clinac 2100CD equipped with multileaf collimator (MLC) 80 leaves for the conventional plans and 6EX equipped with Millennium Multileaf Collimator with 120 leaves for IMRT and RA treatment techniques. The Collapsed Cone (CC) photon dose calculation algorithm Citation[18] was used to calculate conventional plans while the Anisotropic Analytical Algorithm (AAA) Citation[19–27] was used for IMRT and RA calculations. No bolus was applied. The dose calculation grid for the inversely and forward optimised dose plans was set to 3 mm (CC) and 2.5 mm (AAA). The slice thickness was 0.5 cm.
For PTV, the values of mean and minimum doses are reported together with the standard deviation over the eight included cases. In addition a PTV volume receiving at least 90% of the prescribed dose (V90%) is reported. The degree of conformality of the plans was measured with a conformity index, CI90%, defined as the ratio between the treated volume receiving at least 90% of the prescribed dose and the volume of the PTV. The standard deviation in PTV is used as a measure of the dose inhomogeneity. For OAR, the analysis included also the mean and maximum doses and a set of appropriate V-values for each OAR.
Maximum and minimum significant doses have been reported as D1%, D2%, D98% and D99% respectively where Dx% is the dose received by X% of the volume under consideration.
The effect of organ motion has not been considered in this study and all the plans were designed with no allowance of movement of the heart and chest wall.
To assess the differences among the three studied techniques (conventional versus IMRT plans and conventional versus RA plans), the paired-sample, SPSS (Statistical Package for the Social Sciences) paired t-test was applied. Data were considered statistically significant for p<0.05.
Conventional plans
This technique was used as the baseline reference in the present study. In the conventional treatment plans, the beam arrangement consisted of four half-beams with two tangential beams covering the caudal part of the target volume, and one anterior-posterior field (0°) and one oblique field, typically 110–115° from the anterior-posterior field, covering the cranial part. The beam angles, apertures, weights and dynamic wedges were optimized individually. The dose plans were normalized to the mean dose to PTV. The slice thickness was 0.5 cm.
IMRT plans
Intensity modulated plans were computed selecting the ‘conventional’ approach with fixed gantry and intensity modulated beams delivering the dose by means of the sliding window approach. In Eclipse, the optimisation engine of IMRT, computes optimal fluence maps from dose volume constraints derived from the general planning objectives. Optimal fluence maps are then converted by a leaf motion calculator into actual fluence maps which are deliverable using a multileaf collimator, for dynamic mode through the so-called ‘sliding window’ segmentation method. In the present study, IMRT plans were designed with seven fields equally spaced, with gantry from 30° to 210°, every 30°, describing an arc of 180° on the actual side of the patient as described in an earlier study Citation[28]. No extra margin to PTV outside the body contour was selected.
RA plans
At planning level, RA performs optimisation of the dose distribution based on dose-volume objectives, also accounting for the main features of the linac head (e.g. head scatter) and of the MLC (e.g. speed, transmission, rounded leaf tip and tongue and groove design). To achieve the desired level of modulation required, the optimiser is enabled to vary also the instantaneous dose rate, DR, from 0 to the maximum (600 or 1 000 MU/minute depending on the linac type), as well as the gantry rotational speed (from a maximum of ∼5.5 °/s). To minimise the contribution of tongue and groove effect during the arc rotation and to benefit from leaf trajectories non coplanar with respect to patient's axis, the collimator rotation in RapidArc is set to values different from zero. The entire gantry rotation is described in the optimisation process by a sequence of 177 control points (i.e. one control point (CP) every roughly 2°). The final dose calculation is performed in Eclipse by means of the AAA algorithm only. Further details about the RA optimisation process can be found in the earlier studies Citation[26–28].
Risk Prediction
Linear and non-linear models (Equations 1 and 4) as described in more details in an earlier study Citation[17] were employed to estimate excess relative risk (ERR) for breast cancer induction.1 where ERRD is ERR per dose (Gy) and with a suggested value of 0.86 Gy−1 for breast cancer induction caused by acute irradiation Citation[29] and
is the mean CB dose. In this study ERRD was reduced by a factor of 2 and set to 0.43 Gy−1 to account for fractionated radiation treatment Citation[17].
The non linear model is suggested by Dasu et al. Citation[30] and consists of two terms that effectively give the yield of pre malignant cells. The first term describes the induction of DNA mutations, and thus pre-malignant cells. The second term describes the cell survival:2 where D is each dose interval of the dose-volume histogram, n number of fractions, α1 and β1 are radiation induced mutation parameters, and α2 and β2 are cell survival parameters. Different parameters were investigated: α1=0.001–0.1 Gy−1, α2=0.25 Gy−1, and α1 /β1 and α2 /β2=2-8 Gy. The risk was estimated with parameter values α1/β1 and α2/β2=4 Gy, α1=0.002 Gy−1, α2=0.25 Gy−1 in this paper. However, the risk for induced malignancies is calculated for each dose interval of dose volume histogram using the non-linear model Citation[17]. Total effect model by Equation 3 was further used to integrate the result according to the dose distribution in CB:
3 where υi is the volume of tissue receiving dose Di given in n individual fractions. The final ERR product is estimated by 4 as explained in an earlier study Citation[17].
4 where K is the proportionality factor linking the yield of pre-malignant cells and excess relative risk Citation[17].
Results
A summary of DVH analysis can be found in where mean values over the cohort of eight patients are reported together with their standard deviation for the most interesting physical parameters. The mean and V90% in PTV were not statistically significant different among techniques. CI90% was on average nearly two times higher for conventional plans than for IMRT and RA plans. This difference was statistically significant (p < 0.01 for both IMRT versus conventional and RA versus conventional). The minimum dose to PTV was highest for the conventional plans. The difference was only statistically significant for the conventional plans versus IMRT plans with a p-value of 0.02. No statistically significant differences were observed in terms of minimum significant doses (D98% or D99%). Our data shows a more homogeneous dose distribution in PTV for the RA plans compared to the other two techniques. The statistically significant difference in inhomogeneity between the RA plans and the conventional technique was confirmed with a p-value <0.05 (0.012). For the maximum significant doses D1% and D2% in the PTV, the conventional and RA plans are statistically significant lower than the IMRT plans as well as the maximum dose to PTV is statistically significant lower in the RA plans versus conventional (p-value of 0.007) and versus IMRT with a p = 0.002.
Table I. Summary of DVH analysis for PTV and OAR for the cohort of 8 breast cancer patients included in this study, for 4 fields conventional, IMRT and RA treatment techniques. Data are shown as mean values plus or minus one standard deviation; in brackets the range. The patients were treated planned for 6 MV beams to a dose of 50 Gy in 25 fractions.
It has been previously shown that Dmax is correlated to cardiac toxicity following breast cancer irradiation Citation[31]. In our analysis no statistically significant difference in Dmax was found between the different plans. However, it should be noted that our patient population comprised only 3 left-sided breast cases and no conclusion can therefore be drawn.
The summary of findings on ipsilateral lung indicates that the difference in mean dose and V20Gy were significantly lower in the IMRT and RA plans compared to the conventional plans (p < 0.01). The maximum dose was significantly lower only in the RA plans versus conventional plans with a p value inferior to 0.01. The differences in the V10Gy to the IPSI-L between conventional plans versus IMRT and RA were not significant. Our data show that approximately an IPSI-L volume of 52% receiving a dose of 10 Gy for the conventional plans compared to an IPSI-L volume of 55% for both the IMRT and RA plans. In the case of V20Gy, both IMRT and RA showed values significantly inferior to conventional plans and consistent with about 22% V20GyCitation[32]. The significantly lowest D1% and D2% doses for IPSI-L were observed in the IMRT and RA plans (p = 0.00).
The contra-lateral lung received as expected statistically significant lower mean dose for the conventional plans (p = 0.00) than the IMRT and RA plans, with a relative reduction of more than 40% in the average mean CL dose. Nevertheless, for all techniques, low V10Gy was achieved (with RA showing the lowest level).
The mean dose to the contra-lateral breast was lowest for the RA plans versus conventional and IMRT plans. This difference was not statistically significant. V10Gy in the CB was found to be statistically significant lower for IMRT and RA plans compared to conventional (p = 0.009 for the IMRT versus conventional plans and p = 0.006 for the RA versus conventional plans). Our result also shows a statistically significant lower maximum point dose to CB in the RA plans versus conventional with a p-value of 0.016. The difference in maximum point dose between IMRT and conventional plans is not statistically significant; however, the average maximum dose is around 10% lower in the IMRT plans versus conventional plans. Maximum significant doses D1% and D2% for CB are statistically significant lowest in the IMRT and RA plans versus conventional plans with p values of 0.001, 0.00, 0.002 and 0.00, respectively.
The cumulative dose volume histograms for the PTV and CB for conventional, IMRT and RA plans are displayed in for each patient. This figure shows that the dose to the target between the eight patients included in the present study vary more distinctly in the conventional and IMRT plans than in the RA plans. A larger CB volume receiving a dose more than 10 Gy for the conventional and IMRT plans compared to the RA plans are also revealed in the same figure.
Figure 1. Dose volume histograms in cumulative form showing the dose distribution for CB and target using conventional treatment technique (upper panel), IMRT (middle panel) and RapidArc (lower panel).
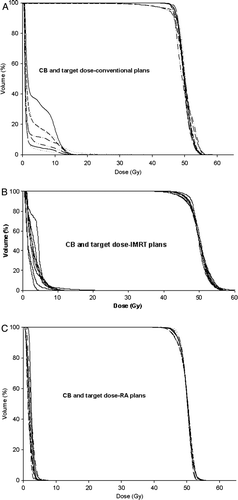
Mean dose to CB for each of the patients included in the analysis with three treatment techniques is shown in the . The average mean CB dose for the eight patients in the conventional, IMRT and RA plans is below 4.4%, nearly 5.7% and 4% of the prescribed dose, respectively. The mean CB dose, however, varies from approximately 1.3 to 4.3, 1.6 to 4.2 and 1.6 to 2.6 Gy between the patients, i.e. by a factor of approximately 3, 2.6 and 1.6 for the conventional, IMRT and RA plans, respectively.
Table II. Mean dose to CB and ERR calculated using the linear and non-linear models for the 8 patients using standard conventional treatment technique, IMRT and volumetric modulated arc techniques. The mean CB dose is used to predict the risk for radiation induced malignancy in CB using the linear model. Calculations applying the non-linear model are based on the patients’ individual dose volume histogram and assuming α1=0.002Gy−1, α2=0.25Gy−1 and both α1/β1=α2/β2=4Gy.
The calculated ERR, using the linear and non-linear models, as described in the 1 and 4, are shown in . presents graphically the same information. The mean predicted ERR for the eight patients included in the analysis was shown to be somewhat higher for the IMRT plans as compared with the two others when assuming a linear dose-risk relationship; this difference was however not statistically significant. When assuming a non-linear dose-risk relationship the mean predicted ERR was statistically significant lower for all the techniques; there were however no statistically significant differences in predicted mean ERR between the techniques. The calculated average ERR for the eight patients is approximately 2.3, 3.1 and 2.6 times higher when assuming a linear relationship as compared to a non-linear relationship for the conventional, IMRT and RA plans, respectively. The ERR of secondary cancer predicted by the linear model for the three techniques included in this analysis varies considerably between the eight patients as the mean CB dose varies (factor 3, 2.6 and 1.6) for the three techniques.
Discussion
Intensity modulation gives the possibility to generate concave dose distribution. The nearly concave shape of the PTV in breast cancer patients, make this patient group a relatively ideal choice for such treatment techniques. The present study compared two relatively new techniques; an IMRT technique with 5–9 fields together with a single arc volumetric IMAT technique (RA) against a well-established four field conventional treatment technique for radiotherapy of breast cancer with nodal involvements (infraclavicular and internal mammary chains). The major purpose of this comparison was to investigate the estimated dose to contra-lateral breast for each of the techniques included in the present study under comparable conditions, i.e. PTV coverage and aiming at the lowest possible involvement of organs at risk. It need to be mentioned that our results regarding the heart are relatively sparse and not sufficient to be discussed since only eight patients both left-and right-sided were included in the study.
Although, the minimum doses in PTV was significantly lower for IMRT plans and not significantly lower for RA plans versus the conventional plans as shown in , it is clear from V90% and from the DVHs of , that this underdosage observed for conventional plans is small and therefore clinically not relevant. The CI90% showed a better conformation in the IMRT and RA plans than the conventional ones implying consequently, an improved sparing of normal healthy tissues surrounding the mammary gland with these techniques compared to conventional approaches. Similarly, findings on standard deviation in PTV for RA plans, showed also a statistically significant improvement in homogeneity in the PTV. In addition, the larger variation in PTV dose distribution between the patients shown for conventional and IMRT plans () suggests that individual anatomical differences between the patients play a more crucial and potentially detrimental role, differently from what observed for RA data where interpatient variability has an almost marginal impact. This is consistent with what is reported by Wim et al. Citation[33] where several numbers of fields were proven to be needed when the inner radius of the concave PTV increases to avoid underdosage in PTV, whilst keeping OAR spared. The size of PTV varies considerably among breast cancer patients and therefore it could be advantageous if the number of fields is adjusted to the PTV size (conventional and IMRT cases). For RA, a single continuous arc of 360 degrees was used as described in the methods, representing the minimum number of “fields” defining a volumetric IMAT treatment.
It has been demonstrated Citation[34] that the mean lung dose and the percentage lung volume receiving more than 20 Gy (V20Gy) are good predictors for lung toxicity. Our results show a significant improvement in mean IPSI-L dose and V20Gy in IMRT and RA plans versus the conventional ones. In terms of CL dose, our data indicate no clinically significant difference in the three techniques except in mean and maximum CL dose which is lower in the standard and RA plans, respectively.
In the current study the lowest mean CB dose is observed in the RA plans. Our data also shows that a larger volume of CB, for most of the patients, receive a dose higher than 10 Gy ( and ) in the conventional plans. Landau et al. Citation[35] concluded in their study that in the case of breast radiotherapy, any variation from the conventional beam arrangement is likely to result in substantially increased low-dose irradiation to the contralateral breast and both lungs. This is partially confirmed for CB in the low dose region by the present results, but the substantially different shapes of DVH in the medium dose levels (with RA preferable to IMRT or conventional plans) makes it difficult to draw a generalised conclusion.
In fact, results reported for ERR estimates for secondary CB cancer employing linear and non-linear models suggest that conventional and RA data are highly consistent while a slight deterioration was observed for IMRT. The ERR predictions as shown in and , demonstrate similar estimations as presented in our recent study Citation[17]. It is reported Citation[17] that the linear model predicts a substantially higher ERR for the higher dose group, but similar ERR as for the low dose group (over 3-4 Gy) using a non linear model. The reason for this difference in predicted ERR by the two models for the high and low dose groups, respectively, are already discussed in our earlier study Citation[17]. According to the non-linear model further reduction in risk of radiation induced malignancy can best be achieved by reducing the dose to those regions where the dose already is low; whereas the linear model would recommend reducing the dose to those regions receiving the highest dose. Based on these considerations and the present results, the conventional treatment technique would be the one with lowest risk of radiation induced secondary CB malignancy, if a linear dose-risk relationship is assumed. The difference between techniques with respect to predicted ERR were, however, not statistically significant. On the contrary, when a more elaborated model is assumed as in the case of the two-component model to predict the risk of malignancy in CB, the most suitable techniques would be the volumetric modulated arc solution or the conventional technique. In fact, conventional dose distributions showed a larger CB volume receiving a medium-higher dose compared to RA compensating the smaller CB volume irradiated at very low-dose. IMRT plans present, on the contrary, larger involvement of the CB gland in both dose regions and this will result into a better minimizing the risk for radiation induced secondary CB cancer for RapidArc and conventional treatments compared to IMRT Citation[17].
A final note of caution shall be devoted to the photon dose calculation algorithms applied in the present study. The CC algorithm was employed to calculate the standard treatment plans whereas for the IMRT and RA treatment plans AAA algorithm was adopted. In an earlier study Citation[36] the calculation accuracy of CC and Pencil Beam, with special emphasis on peripheral dose, was investigated and compared with measured data. The study revealed a good agreement between CC calculations and measurements, superior to the corresponding for pencil beam. Similar investigations have been carried out recently to assess the behaviour of AAA (as well as CC and other algorithms) in the presence of heterogeneities in different geometrical or clinical conditions Citation[22–25], Citation[37] using as reference either measurements or Monte Carlo simulations. In general these studies proved that: i) AAA and CC have similar behaviour when applied to clinical conditions. Hasenbalg et al. Citation[37] showed a slightly superior quality of CC versus AAA based on a qualitative comparison of five clinical cases (lung and breast) benchmarked against Monte Carlo while, on the contrary, Knöös Citation[25] showed substantial equivalence. On a quantitative basis, Fogliata et al. Citation[23], Citation[24] investigated on geometrical phantoms and on clinical breast patients with different respiratory phases, the behaviour of the algorithms measured in terms of γ estimates, DVH analysis and correlations between tissue density and calculation accuracy. In these investigations, AAA and CC resulted equivalent to Monte Carlo simulations with some different pattern of accuracy depending on the localisation in the treated volumes but insufficient to claim superiority of any of the two algorithms. No peripheral dose calculation accuracy is, however, investigated in the study of Hasenbalg et al. Citation[37] while it was implicitly investigated in Fogliata et al. Citation[24] showing in this case a modest superiority of AAA but at the limit of statistical relevance. Further comparative analysis of the two algorithms used in the present study should be performed especially on the peripheral dose where calculations should be compared against measurements or Monte Carlo simulations with sufficient statistical power.
In conclusion, our data shows a better homogeneity and conformation in PTV in the RA plans versus conventional and IMRT plans. The highest minimum dose to PTV was observed in the conventional plans while no difference was observed for minimum significant doses. In terms of organ sparing, the IMRT and RA plans spare IPSI-L better, while the mean CL dose was, obviously given the essentially “tangential” geometry of the treatment, lower in the conventional plans. The cardiac sparing, for the same reason, is also better for the conventional plans versus the two other techniques for both the left-sided patients as well as the right-sided. The mean CB dose and the volume receiving medium-high dose levels resulted lowest for RA plans. IMRT and RA showed, as expected for the same reasons mentioned above, a slightly larger CB volume receiving a low-dose radiation. The impact of CB exposure to a low-dose radiation on minimizing the risk of radiation induced malignancy in CB can be interpreted differently when using linear or non linear models to predict ERR. In general, no detriment was observed when using RA compared to conventional treatments while a potentially higher risk could be associated to IMRT treatments with fixed gantry.
From a clinical perspective, it should be concluded that all three solutions investigated in the study can offer high quality of patient treatments and estimates of radiation induced malignancies do not truly differentiate among them. The selection of the more appropriate treatment should therefore depend on other factors like: i) minimisation of set-up and planning uncertainties (e.g. given by the usage of beam matching or field patching); ii) minimisation of treatment time; iii) maximisation of clinical throughput.
Acknowledgements
The work was supported by grants from the Norwegian Cancer Society and Norwegian Breast Cancer Society. Dr. L. Cozzi acted as Scientific Advisor to Varian Medical Systems and as Head of Research and Technological Development to Oncology Institute of Southern Switzerland, Bellinzona.
References
- Boice JD, Harvey EB, Blettner M, Stovall M, Flannery JT. Cancer in the contralateral breast after radiotherapy for breast cancer. N Engl J Med 1992; 326: 781–5
- Gao X, Fisher SG, Emami B. Risk of second primary cancer in the contralateral breast in women treated for early-stage breast cancer: A population-based study. Int J Radiat Oncol Biol Phys 2003; 56: 1038–45
- Unnithan J, Macklis RM. Contralateral breast cancer risk. Radiother Oncol 2001; 60: 239–46
- Harvey EB, Brinton LA. Second cancer following cancer of the breast in Connecticut, 1935–82. J Natl Cancer Inst Monogr 1985; 68: 99–112
- Damen EM, Brugmans MJ, Van der Horst A, Bos L, Lebesque JV, Mijnheer BJ, et al. Planning, computer optimization, and dosimetric verification of a segmented irradiation technique for prostate cancer. Int J Radiat Oncol Biol Phys 2001; 49: 1193–5
- Brugmans MJ, Van der Horst A, Lebesque JV, Mijnheer BJ. Beam intensity modulation to reduce the field sizes for conformal irradiation of lung tumors: A dosimetric study. Int J Radiat Oncol Biol Phys 1999; 43: 893–904
- Boyer AL, Geis P, Grant W, Carol M. Modulated beam conformal therapy for head and neck tumors. Int J Radiat Oncol Biol Phys 1997; 39: 227–36
- Evans PM, Donovan EM, Patridge M, Childs PJ, Convery DJ, Eagle S, et al. The delivery of intensity modulated radiotherapy to the breast using multiple static fields. Radiother Oncol 2000; 57: 79–89
- Yu CX, Li XA, Ma L, Chen D, Nagvi S, Shephard D, et al. Clinical implementation of intensity-modulated arc therapy. Int J Radiat Oncol Biol Phys 2002; 53: 453–63
- Yu CX. Intensity-modulated arc therapy with dynamic multileaf collimation: An alternative to tomotherapy. Phys Med Biol 1995; 40: 1435–49
- Cameron C. Sweeping-window arc therapy: An implementation of rotational IMRT with automatic beam-weight calculation. Phys Med Biol 2005; 50: 4317–36
- Cortuz C, Kappas C, Webb S. Intensity modulated arc therapy (IMAT) with centrally blocked rotational fields. Phys Med Biol 2000; 45: 2185–206
- Duthoy W, De Gersem W, Vergote K, Coghe M, Botenberf T, De Deene Y, et al. Whole abdominopelvic radiotherapy (WAPRT) using intensity-modulated arc therapy (IMAT): First clinical experience. Int J Radiat Oncol Biol Phys 2003; 57: 1019–32
- Earl M, Shepard D, Nagvi S, Li X, Zu CX. Inverse planning for intensity-modulated arc therapy using direct aperture optimization. Phys Med Biol 2003; 48: 1075–89
- MacKenzie MA, Robinson DM. Intensity modulated arc deliveries approximated by a large number of fixed gantry position sliding window dynamic multileaf collimator fields. Med Phys 2002; 29: 2359–65
- Otto K. Volumetric Modulated Arc therapy: IMRT in a single arc. Med Phys 2008; 35: 310–7
- Johansen S, Danielsen T, Olsen DR. Estimated risk for secondary cancer in the contra-lateral breast following radiation therapy of breast cancer. Acta Oncol 2008; 47: 391–6
- Ahnesjö A. Collapsed cone convolution of radiant energy for photon dose calculation in heterogeneous media. Med Phys 1989; 16: 577–92
- Ulmer W, Pyyry J, Kaissl W. A 3D photon superposition/convolution algorithm and its foundation on results of Monte Carlo calculations. Phys Med Biol 2005; 50: 1767–90
- Ulmer W, Harder D. A triple Gaussian pencil beam model for photon radiations in treatment planning. Med Phys 1995; 5: 25–30
- Ulmer W, Harder D. Applications of a triple Gaussian pencil beam model for photon beam treatment planning. Med Phys 1996; 6: 68–74
- Fogliata A, Nicolini G, Vanetti E, Clivio A, Cozzi L. Dosimetric validation of the anistropic Analytical Algorithm for photon dose calculation: Fundamental characterisation in water. Phys Med Biol 2006; 51: 1421–38
- Fogliata A, Vanetti E, Albers D, Brink C, Klivio A, Knöös T, et al. On the dosimetric behaviour of photon dose calculation algorithms in the presence of simple geometric heterogeneities: Comparison with Monte Carlo calculations. Phys Med Biol 2007; 52: 1363–85
- Fogliata A, Vanetti E, Clivio A, Nicolini G, Winkler P, Cozzi L. The impact of photon dose calculation algorithms on lung tissue under different respiratory phases. Phys Med Biol 2006; 51: 1421–38
- Knöös T, Wieslander E, Cozzi L, Brink C, Fogliata A, Albers D, et al. Comparison of dose calculation algorithms for treatment planning in external photon beam therapy for clinical situations. Phys Med Biol 2006; 51: 5785–807
- Fogliata A, Nicolini G, Alber M, Asell M, Dobler B, El-Haddad M, et al. IMRT for breast, a planning study. Radiother Oncol 2005; 76: 300–10
- Cozzi L, Dinshaw KA, Shivastava SK, Mahantshetty U, Engineer R, Deshpande DD, et al. A treatment planning study comparing volumetric arc modulation with RapidArc and fixed field IMRT for cervix uteri radiotherapy. Radiother Oncol 2008; 89: 180–91
- Fogliata, A, Clivio, A, Vanetti, E, Nicolini, G, Cozzi, L. Intensity modulation with photons for benign intracranial tumours. A planning comparison of volumetric single arc, helical arc and fixed gantry techniques. Radiother Oncol 2008 ( in press)
- Preston DL, Mattsson A, Holmberg E, Shore R, Hildreth NG, Boice JD, Jr. Radiation effects on breast cancer risk: A pooled analysis of eight cohorts. Radiat Res 2002; 158: 220–35
- Dasu A, Toma-Dasu I, Olofsson J, Karlsson M. The use of risk estimation models for the induction of secondary cancer following radiotherapy. Acta Oncol 2005; 44: 339–47
- Gagliardi G, Lax I, Söderström S, Gyenes G, Rutqvist LE. Prediction of excess risk of long-term cardiac mortality after radiotherapy of stage I breast cancer. Radiother Oncol 1998; 46: 63–71
- Graham MV, Purdy JA, Emami B, Harms W, Bosch W, Lockett MA, et al. Clinical dose-volume histogram analysis for pneumonitis after 3D treatment of non-small cell lung cancer (NSCLC). Int J Radiat Oncol Biol Phys 1999; 45: 323–9
- Wim D, De Gersem W, Vergote K, Boterberg T, Derie C, Smeets P, et al. Clincial implementation of intensity-modulated arc therapy (IMAT) for rectal cancer. Int J Radiat Biol Phys 2004; 60: 794–806
- Kwa S, Lebesque J, Theuws JC, Marks LB, Munley MT, Bentel G, et al. Radiation pneumonitis as a function of mean lung dose: An analysis of pooled data of 540 patients. Int J Radiat Oncol Biol Phys 1998; 42: 1–9
- Landau D, Adams E, Webb S, Ross G. Cardiac avoidance in breast radiotherapy: A comparison of simple shielding techniques with intensity-modulated radiotherapy. Radiother Oncol 2001; 60: 247–55
- Johansen S, Olsen DR, Danielsen T, Malinen E. Contralateral breast doses following radiotherapy of the breast and regional lymph nodes: Measurements and treatment planning calculations. Radiother Oncol 2007; 82: 332–6
- Hasenbalg F, Neuensschwander H, Mini R, Born EJ. Collapsed cone convolution and analytical anistropic algorithm dose calculations compared to VMC + +Monte Carlo simulations in clinical cases. Phys Med Biol 2007; 52: 3679–91