Abstract
Background
The causal association between particulate matter 2.5 (PM2.5) and Alzheimer’s disease (AD) remains inconclusive, and the mediators of the association have yet to be explored.
Aims
We aimed to assess the potential causal relationship between PM2.5 and AD, and to investigate the mediating role of dehydroepiandrosterone sulphate (DHEAS).
Subjects and methods
We implemented a two-sample Mendelian randomisation (MR) study to examine the genetic predisposition to PM2.5 exposure and its association with AD. The inverse-variance weighted (IVW) method served as the primary analytical tool to estimate the odds ratio (OR) and 95% confidence interval (95% CI).
Results
There were 6 and 4 genetic variants associated with DHEAS and PM2.5, respectively. Based on the multivariable MR analysis, we found that after adjusting for DHEAS, each standard deviation increase in PM2.5 was associated with the risk of AD (OR: 2.96, 95% CI: 1.33, 6.58, p = 0.00769). The MR Egger intercept test did not detect horizontal pleiotropy for PM2.5 (P-pleiotropy = 0.879) and DHEAS(P-pleiotropy = 0.941). According to the results of the mediation analysis, DHEAS accounted for 18.3% of the association between PM2.5 and AD.
Conclusion
Our findings affirm a significant causal association between PM2.5 exposure and AD, with DHEAS playing a mediating role in this relationship.
Introduction
Ambient air pollution is a major environmental concern for the health of the population in both urban and rural areas worldwide (Zhao et al. Citation2021). Fine particulate matter with a diameter of less than 2.5 micrometres (PM2.5) has emerged as a significant and widespread air pollutant, and it represents a new environmental risk factor for accelerated brain ageing (Underwood Citation2017), as well as being linked to an increased risk of multiple chronic diseases (Tainio et al. Citation2021) including Alzheimer’s disease (AD) (Younan et al. Citation2020). A systematic review and meta-analysis involving nearly 12 million older individuals revealed that a 10 μg/m3 increase in PM2.5 concentration was positively associated with AD (Tsai et al. Citation2019). Another meta-analysis indicated that the probability of developing AD increased by 95% for every 10 μg/m3 increase in PM2.5 concentration (Fu and Yung Citation2020). A recent meta-analysis of epidemiological studies identified exposure to PM2.5 as a significant risk factor for AD, with a pooled hazard ratio of 1.08 (Dhiman et al. Citation2022). It is noteworthy that earlier meta-analyses encompassed studies employing various AD diagnostic evaluation techniques and different exposure assessment methodologies (Dhiman et al. Citation2022; Fu and Yung Citation2020; Tsai et al. Citation2019). Moreover, epidemiological studies are inherently challenged by potential confounding shifts and reverse causality (Nørgaard et al. Citation2017; Sattar and Preiss Citation2017), necessitating sophisticated research methods to elucidate the causal relationship between PM2.5 and AD. Importantly, certain confounding or mediating factors, particularly biological indicators closely related to the onset of neurological diseases, play a crucial role in assessing their interrelationship.
Dehydroepiandrosterone sulphate (DHEAS), a prevalent endocrine hormone produced mainly by the adrenal gland, holds significant value in assessing neurological disorder-related diseases (Leowattana Citation2001). Research has demonstrated that environmental pollution exposure can disrupt homeostasis and affect hypothalamic-pituitary-gonadal axis hormone levels, including DHEAS (Rodriguez-Carrillo et al. Citation2023). DHEAS is involved in critical brain functions such as neuronal plasticity, survival, cognition, and behaviour, it has also shown preventative and therapeutic potential in various neuropsychiatric and neurodegenerative disorders, including AD (Strac et al. Citation2020). A meta-analysis of thirty-one studies suggested that a decrease in DHEAS concentration could signal an increased risk of AD (Pan et al. Citation2019). Furthermore, DHEAS may hinder the development and pathophysiology of AD by counteracting natural killer cell overactivity in response to cytokines, due to its immunomodulatory role in the physiological activity of these cells (Solerte et al. Citation1999). This antagonistic effect of DHEAS on natural killer cell overactivity could alleviate certain neuroimmune elements associated with the aetiology and progression of AD (Solerte et al. Citation1999). However, the causal link and potential role of DHEAS in AD remain unconfirmed, primarily due to the inherent potential confounding in epidemiological studies (Nørgaard et al. Citation2017).
Mendelian randomisation (MR), a method for inferring causality using genetic variability as a proxy for exposure, functions similarly to conducting a natural randomised control trial. This approach helps mitigate confounding bias and reverse causality often encountered in observational studies (Lawlor et al. Citation2008). MR’s design inherently prevents reverse causation, as allele assignment during meiosis is random and precedes disease onset (Zheng et al. Citation2017). These advantages extend to mediation analysis, where MR ensures the absence of unmeasured confounding among the exposure, mediator, or outcome – a challenge seldom overcome by traditional observational methods (Carter et al. Citation2021). The recent proliferation of genome-wide association studies (GWAS) data, characterised by large sample sizes, has improved the estimation of single-nucleotide polymorphisms (SNPs) and, consequently, facilitated MR research. Thus, our study aims to explore the independent causal associations of PM2.5 and DHEAS with AD, and to ascertain the mediating role of DHEAS in the aetiology of PM2.5-related AD.
Materials and methods
Study design
This study employed a two-sample MR approach to elucidate the causal relationship between PM2.5, DHEAS, and AD, focusing particularly on the mediating role of DHEAS. In adherence to the Strengthening the Reporting of Observational Studies in Epidemiology using Mendelian Randomisation (STROBE-MR) guidelines, this MR investigation was meticulously conducted (Skrivankova et al. Citation2021). A detailed flow chart outlining the study’s methodology is depicted in . Given that the analysis was based on summary-level data from publicly available sources, the need for informed consent and ethical approval was circumvented. Nonetheless, the Ethics Committee of the Guangdong Provincial People’s Hospital endorsed the study, providing a solid ethical foundation for our analysis (KY-Q-2021-244-01).
Data sources
In this MR study, we sourced our data for exposures, mediator, and outcome variables from the summary-level data available in the IEU OpenGWAS database (https://gwas.mrcieu.ac.uk/). The primary exposures investigated were PM2.5 and DHEAS, with DHEAS also serving as the mediating variable, and AD being the outcome variable. For PM2.5, summary-level genetic data was retrieved from the UK Biobank, encompassing 423,796 individuals and 9,851,867 SNPs, under the trait code: ukb-b-10817. AD-related summary-level data was sourced from the IEU OpenGWAS database, featuring 54,162 individuals and 7,055,882 SNPs, identified by the trait code: ieu-a-97. Additionally, DHEAS-related summary-level genetic data was obtained from the EBI GWAS database, which included 9,722 individuals and 21,770,677 SNPs, associated with the trait of ebi-a-GCST004941. All datasets were derived from European populations. A comprehensive summary of the traits included is provided in .
Table 1. Summary of the included traits in the Mendelian randomisation study.
Instrumental variable selection
Three key assumptions have to be met in order to derive causal conclusions from this MR study: (1) the genetic variations were significantly linked with PM2.5; (2) the genetic variants were not associated with potential confounders; and (3) the genetic variants were not associated with AD except via the PM2.5 route. For the selection of all SNPs, a genome-wide significant level (p < 5 × 10−8), a lack of linkage disequilibrium (LD) at an R2<0.001 threshold, and a clumping distance more than 10,000 kb were necessary. The relevance assumption was often validated in the literature on instrumental variables by an F-statistic value greater than 10. If an exposure SNP was missing from an outcome dataset, proxy SNPs with a minimum LD R2 value greater than 0.8 and a minor allele frequency (MAF) threshold for aligning palindromes more than 0.3 were used instead.
Assessment of ambient PM10 and DHEAS
To assess ambient air pollution levels, a Land Use Regression (LUR) model developed within the framework of the European Study of Cohorts for Air Pollution Effects (ESCAPE) (http://www.escapeproject.eu/) was employed. This model estimated the annual average concentration of PM2.5 at each participant’s address by leveraging Geographic Information System (GIS)-derived variables, including traffic intensity, land use patterns, and topographical features. The LUR models adeptly captured the spatial variability of yearly average air pollutant concentrations around the homes of the participants. Meanwhile, the United Kingdom Household Longitudinal Study—a comprehensive longitudinal survey involving 40,000 households across the United Kingdom (comprising England, Wales, Scotland, and Northern Ireland)—yielded summary statistics for DHEAS. This study involved 9722 participants (4308 men and 5414 women)(Prins et al. Citation2017). The measurement of DHEAS levels in serum samples was conducted using a competitive immunoassay on the Roche E module analyser. Subsequently, the results were log-transformed and adjusted for age and gender differences, thus ensuring that the SNPs accurately reflected a log-transformed unit (mol/L) increase in DHEAS levels (Nounu et al. Citation2022; Prins et al. Citation2017).
Statistical analysis, sensitivity analysis and mediation effect analysis
For the primary univariable MR analysis, we employed the inverse-variance weighted (IVW) method. Cochran’s Q-statistic was used to measure heterogeneity, which was a potential indicator of horizontal pleiotropy. We used the weighted median and mode-based estimators, MR-Egger regression, and MR-PRESSO (Pleiotropy RESidual Sum and Outlier) to test for potential bias from horizontal pleiotropy. The leave-one-out sensitivity analysis was also employed to examine how the magnitude of associations changes by removing individual SNPs. To evaluate heterogeneity, the MR-Egger and IVW methods were applied. If horizontal pleiotropy existed in IVs, the MR-Egger intercept was nonzero and statistically significant (p < 0.05). Each method relaxed a different set of MR presumptions, making a consistent effect across all ways more resistant to bias from horizontal pleiotropy. For multivariable MR analysis, we used the IVW method, and DHEAS level was adjusted. The results were shown using scatter plots, forest plots, and funnel plots. Forest plots were used to illustrate each SNP’s influence. MR Egger analysis was also shown in the forest plots. A leave-one-out analysis was performed to ascertain how each instrument contributed to the overall effect. To express the MR estimates of causality between PM2.5 and AD risk, odds ratios (OR) and related 95% confidence intervals (CI) were utilised.
For mediation MR analyses, a 2-step MR was performed to assess whether DHEAS has a mediating effect between PM2.5 and AD. The first stage was to calculate the causal influence of genetically determined PM2.5 on the mediator DHEAS (A), and the second was to calculate the DHEAS’s causal effect on AD (B). The proportion of DHEAS’s total effect on AD was estimated by dividing the indirect effect, which was obtained by multiplying the results from the two phases (A × B) by the total effect. The delta method was used to calculate standard errors from effect estimates obtained from two-sample MR analysis (Burgess et al. Citation2015). The R packages of TwoSampleMR, Mendelian Randomisation, MR-PRESSO, and MVMR were used to carry out all MR analyses. The MR analysis was carried out using the R software (version 4.0.3, available at http://www.r-project.org) and the "TwoSampleMR" R package (version 0.5.5, available at https://mrcieu.github.io/TwoSampleMR/). All reported P values were two-sided and significance was expressed in p < 0.05.
Results
Selection of instrumental variables
In this MR investigation, a rigorous selection process for instrumental variables related to PM2.5 and DHEAS in the context of AD resulted in the identification of 6 independent genetic variants for PM2.5 and 4 for DHEAS. These variants were selected based on predefined screening criteria that emphasised their relevance to AD. presented detailed information on these instrumental variables, including the SNP names, standard errors, effect alleles, effect allele frequencies, and beta coefficients, providing a comprehensive overview of their characteristics and potential impact on AD.
Table 2. Detailed information on genetic instruments associated with Alzheimer’s disease.
MR analysis of PM2.5 level and AD
In the univariate MR analysis, genetically predicted PM2.5 levels were linked to an increased risk of AD (OR: 2.88, 95% CI: 1.01, 8.18, p = 0.00475), and were significantly associated with DHEAS levels (OR:1.90, 95% CI: 1.14, 3.17, p = 0.014) for each 1-standard deviation (SD) increment in PM2.5, as determined by the IVW method (). In the multivariable MR framework, after adjusting for DHEAS levels, it was found that each SD increase in PM2.5 was associated with an increased risk of AD (OR: 2.96, 95% CI: 1.33, 6.58, p = 0.00769) (). The results of the IVW analysis were visually summarised in a forest plot ().
Figure 2. Association of the genetically determined PM2.5 and dehydroepiandrosterone sulphate with Alzheimer’s disease.
CI: confidence interval; OR: odds ratio; PM: particulate matter; DHEAS: dehydroepiandrosterone sulphate; MR-PRESSO: Mendelian randomisation pleiotropy residual sum and outlie; MVMR: multivariable Mendelian randomisation.
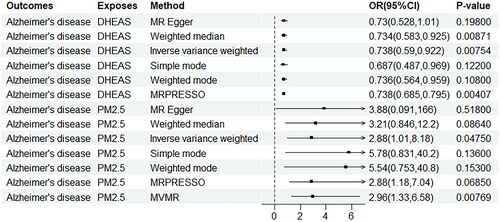
Figure 3. Forest plot of PM2.5 and dehydroepiandrosterone sulphate associated with risk of Alzheimer’s disease. The x-axis showed the MR effect size for PM2.5 and dehydroepiandrosterone sulphate on Alzheimer’s disease: (A)PM 2.5, (B) dehydroepiandrosterone sulphate. The y-axis showed the analysis for each of the SNPs.
PM: particulate matter; SNP: single nucleotide polymorphism; MR: Mendelian randomisation.
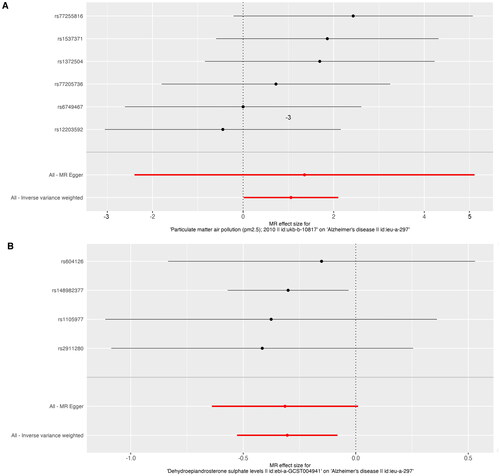
Table 3. Mendelian randomisation assessing the causal association between expose/mediator and Alzheimer’s disease.
MR analysis of DHEAS level and mediation effects of AD
In the univariate MR analysis, genetically predicted DHEAS levels were found to be associated with a 26.2% decrease in the risk of AD for each 1-SD increase in DHEAS, as analysed by the IVW method (OR: 0.738, 95% CI: 0.685, 0.795, p = 0.00407) (). The IVW analysis results were effectively visualised in a forest plot depicted in . According to , the beta value representing the total effect of PM2.5 on AD was 1.06 (95% CI: 0.0118, 2.1000). The beta value for the direct effect A, indicating the impact of PM2.5 on DHEAS levels, was 0.641 (95% CI: 0.129, 1.150). Meanwhile, the beta value for direct effect B, which reflects the influence of DHEAS levels on AD, was −0.304 (95% CI: −0.528, −0.0811). These effects were all determined through IVW analysis. The mediation effect was calculated using the delta method, revealing that the mediation effect of PM2.5 on AD via DHEAS accounted for 18.3% of the total effect.
Table 4. Mediation analysis of the mediation effect of PM2.5 on Alzheimer’s disease via dehydroepiandrosterone sulphate levels.
Pleiotropy, heterogeneity and sensitivity analysis
To validate our findings, we performed sensitivity analyses using a variety of MR methods, including Simple mode, MR Egger, Weighted median, and Weighted mode. illustrated that horizontal pleiotropy, which could potentially bias our results, was not detected in either PM2.5 (P-pleiotropy = 0.879) or DHEAS levels (P-pleiotropy = 0.941) as evidenced by the MR Egger intercept test. Additionally, both the MR Egger and IVW methods yielded P-values greater than 0.05 in Cochran’s Q-test (), indicating the absence of significant heterogeneity among the PM2.5 genetic variants in the AD GWAS dataset. Despite some sensitivity analyses not showing statistical differences, the relationship between PM2.5 and DHEAS with AD remained consistent with the primary IVW findings. The results from these sensitivity analyses were comprehensively visualised in . Moreover, the leave-one-out analysis conducted for each instrumental variable (IV) demonstrated that the causal connections remained significant across all calculated IVs. This suggests that no single IV dominated the observed associations between PM2.5 and DHEAS with AD, reaffirming the robustness and validity of the two-sample MR findings ().
Figure 4. A scatter plot is used to visualise the causal effect association of PM2.5 and dehydroepiandrosterone sulphate level with Alzheimer’s disease: (A)PM 2.5, (B) dehydroepiandrosterone sulphate. The x-axis showed the SNP effect and SE on each PM2.5 anddehydroepiandrosterone sulphate. The y-axis showed the SNP effect and SE on Alzheimer’s disease.
PM: particulate matter; SNP: single nucleotide polymorphism; SE: standard error.
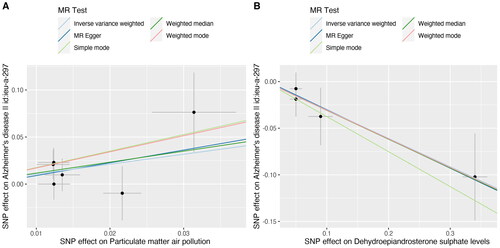
Figure 5. Leave-one-out sensitivity analysis for the effect of PM2.5 and dehydroepiandrosterone sulphate on Alzheimer’s disease: (A)PM 2.5, (B) dehydroepiandrosterone sulphate. The x-axis showed MR leave-one-out sensitivity analysis for PM2.5 or dehydroepiandrosterone sulphate on Alzheimer’s disease. The y-axis showed the analysis for the effect of leave-one-out of SNPs on Alzheimer’s disease.
MR: Mendelian randomisation; PM: particulate matter; SNP: single nucleotide polymorphism.
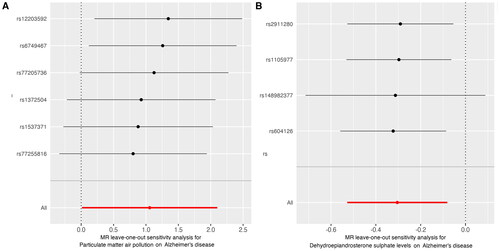
Discussion
This present MR study has provided new evidence for the causative influence of PM2.5 and DHEAS on AD, demonstrating that each additional SD of PM2.5 and DHEAS could potentially increase or decrease the incidence of AD by 188% and 26.2%, respectively. Moreover, we discovered that 18.3% of the effect of PM2.5 on AD was mediated through DHEAS. Furthermore, sensitivity analysis revealed no evidence of heterogeneity or horizontal pleiotropy, further affirming the reliability of our findings.
Pioneering in our approach, we were the first to utilise a two-sample multivariable MR analysis based on a large-scale GWAS dataset to explore the strong causal connection between PM2.5 concentration and the risk of AD. Our findings align with those from previous meta-analyses and cohort studies, highlighting PM2.5 exposure as a significant environmental risk factor for AD development (Dhiman et al. Citation2022; Fu and Yung Citation2020; Tsai et al. Citation2019). A recent meta-analysis involving 13 studies corroborated this, showing an increased risk of AD with PM2.5 exposure (the pooled hazard ratio was 1.47)(Cheng et al. Citation2022). Additionally, PM2.5 exposure has been associated with a higher risk of AD in diverse populations across Asia, Europe, and other regions, as evidenced by multiple cohort studies (Parra et al. Citation2022; Rhew et al. Citation2021; Yang et al. Citation2022), underscoring a universal link between PM2.5 exposure and AD.
Another pivotal discovery of this study was the identification of DHEAS as a protective factor against AD through MR analysis, alongside the quantification of DHEAS’s mediating role in the association between PM2.5 and AD. This study highlights a previous meta-analysis which observed lower DHEAS levels in AD patients compared to controls, suggesting that a decrease in DHEAS levels could be a significant marker for AD (Pan et al. Citation2019). A comprehensive review of the literature on DHEAS’s role across various preclinical studies, including in vitro and animal models, as well as in AD patients, has generally supported DHEAS’s involvement in AD pathophysiology. This suggests potential benefits of these neurosteroids in the prevention and treatment of AD (Strac et al. Citation2020). It is postulated that the presence of amyloid-beta (Aβ) and increased oxidative stress could elevate DHEAS levels, potentially serving as an adaptive or compensatory mechanism within the brains of AD patients (Strac et al. Citation2020). Moreover, further research has indicated that DHEAS possesses anti-amnestic and neuroprotective properties, making it a promising candidate for AD treatment (El Bitar et al. Citation2014; Ouanes et al. Citation2022). However, it is crucial to conduct large-scale human studies to fully elucidate DHEAS’s specific effects and mechanisms before considering its application in clinical settings.
The molecular mechanisms underlying the pathogenesis of AD related to PM2.5 and DHEAS are intricate. Firstly, the small particle size of PM2.5, compared to other atmospheric pollutants, facilitates its penetration through the blood-brain barrier, posing a unique threat to neurological integrity (Thangavel et al. Citation2022). Secondly, PM2.5 can traverse the gas-blood barrier through the “gut-microbial-brain” axis, inducing systemic oxidative stress and inflammation, or directly infiltrate brain tissue via the olfactory nerve, ultimately causing damage to cerebral blood vessels and nerves (Li et al. Citation2022). Thirdly, a significant link between intracranial atherosclerosis and AD has been established, with animal studies demonstrating that PM2.5 exacerbates cerebral atherosclerosis by promoting vascular endothelial dysfunction, systemic inflammation, and oxidative stress (Guan et al. Citation2019; Xie et al. Citation2021). Fourthly, preclinical research has shown a notable decline in spatial learning and memory abilities in mice following PM2.5 inhalation (Ku et al. Citation2017; Wang et al. Citation2020). Additionally, experimental findings suggest that PM2.5 exposure leads to increased tau phosphorylation and amyloid-beta levels, alongside other AD-related molecular and cellular alterations such as mitochondrial dysfunction, synaptic deficits, reduced neurite growth, neuronal cell death, glial cell activation, neuroinflammation, and neurovascular dysfunction (Li et al. Citation2022; Wang et al. Citation2020). In summary, PM2.5 can induce brain damage through multiple pathways, potentially culminating in AD, though the precise mechanisms remain to be fully elucidated.
The present study boasts several strengths that underscore its significance and impact. Firstly, it marks the inaugural MR study to delve into the genetic underpinnings of the causal connections between PM2.5, DHEAS, and AD. Secondly, it adeptly navigates the pitfalls of confounding bias and reverse causality that often beset observational studies, thanks to the robust MR methodology employed. Thirdly, leveraging the vast datasets and large sample sizes characteristic of GWAS, this research incorporates a variety of sensitivity analysis techniques to ensure the stability and reliability of its findings. However, cautious interpretation is warranted due to several limitations. Firstly, the exclusive reliance on GWAS data from European populations means the study’s conclusions may not be directly applicable to other ethnic groups. Secondly, the scarcity of individual-level data precludes detailed analysis of the dose-response relationship between PM2.5, DHEAS, and AD, as well as subgroup analyses. Thirdly, the absence of pleiotropy and heterogeneity notwithstanding, the failure of numerous analytical methods to achieve statistical significance suggests the need for further research to confirm these findings. Lastly, while various types of ambient particulate matter (PM) exist in the environment, this study focused solely on PM2.5, highlighting a specific area of concern but also a limitation in scope.
Conclusion
In conclusion, this study has elucidated a significant causal link between PM2.5 exposure and the risk of AD, underscoring that elevated concentrations of PM2.5 are associated with an increased risk of AD. Furthermore, our analysis revealed a protective effect of higher DHEAS levels against AD, highlighting the notable mediating role of DHEAS in the relationship between PM2.5 exposure and AD development. These insights underscore the public health significance of air quality in relation to AD risk, suggesting that improving air quality could play a crucial role in AD prevention and underscore the importance of air pollution control in mitigating AD development. Nonetheless, future experimental and mechanistic studies are essential to validate the findings of this study and further explore the underlying biological mechanisms.
Disclosure statement
No potential conflict of interest was reported by the author(s).
Availability of data and materials
The GWAS dataset were publicly available from the IEU open GWAS database (https://gwas.mrcieu.ac.uk/). The functions used in the TwoSampleMR package are available from the website https://mrcieu.github.io/TwoSampleMR/.
Additional information
Funding
References
- Burgess S, Daniel RM, Butterworth AS, Thompson SG, EPIC-InterAct Consortium. 2015. Network Mendelian randomization: using genetic variants as instrumental variables to investigate mediation in causal pathways. Int J Epidemiol. 44(2):1–10. doi: 10.1093/ije/dyu176.
- Carter AR, Sanderson E, Hammerton G, Richmond RC, Davey SG, Heron J, Taylor AE, Davies NM, Howe LD. 2021. Mendelian randomisation for mediation analysis: current methods and challenges for implementation. Eur J Epidemiol. 36(5):465–478. doi: 10.1007/s10654-021-00757-1.
- Cheng S, Jin Y, Dou Y, Zhao Y, Duan Y, Pei H, Lyu P. 2022. Long-term particulate matter 2.5 exposure and dementia: a systematic review and meta-analysis. Public Health. 212:33–41. doi: 10.1016/j.puhe.2022.08.006.
- Dhiman V, Trushna T, Raj D, Tiwari RR. 2022. Is air pollution associated with increased risk of dementia? A meta-analysis of epidemiological research. Neurol India. 70(3):1004–1019. doi: 10.4103/0028-3886.349654.
- El Bitar F, Meunier J, Villard V, Alméras M, Krishnan K, Covey DF, Maurice T, Akwa Y. 2014. Neuroprotection by the synthetic neurosteroid enantiomers ent-PREGS and ent-DHEAS against Abeta(2)(5)(-)(3)(5) peptide-induced toxicity in vitro and in vivo in mice. Psychopharmacology (Berl) 231(17):3293–3312. doi: 10.1007/s00213-014-3435-3.
- Fu P, Yung K. 2020. Air pollution and Alzheimer’s disease: a systematic review and meta-analysis. J Alzheimers Dis. 77(2):701–714. doi: 10.3233/JAD-200483.
- Guan L, Geng X, Stone C, Cosky E, Ji Y, Du H, Zhang K, Sun Q, Ding Y. 2019. PM(2.5) exposure induces systemic inflammation and oxidative stress in an intracranial atherosclerosis rat model. Environ Toxicol. 34(4):530–538. doi: 10.1002/tox.22707.
- Ku T, Li B, Gao R, Zhang Y, Yan W, Ji X, Li G, Sang N. 2017. NF-kappaB-regulated microRNA-574-5p underlies synaptic and cognitive impairment in response to atmospheric PM(2.5) aspiration. Part Fibre Toxicol. 14(1):34. doi: 10.1186/s12989-017-0215-3.
- Lawlor DA, Harbord RM, Sterne JA, Timpson N, Davey SG. 2008. Mendelian randomization: using genes as instruments for making causal inferences in epidemiology. Stat Med. 27(8):1133–1163. doi: 10.1002/sim.3034.
- Leowattana W. 2001. DHEA(S): the fountain of youth. J Med Assoc Thai. 84 Suppl 2:S605–S612.
- Li W, Lin G, Xiao Z, Zhang Y, Li B, Zhou Y, Ma Y, Chai E. 2022. A review of respirable fine particulate matter (PM(2.5))-induced brain damage. Front Mol Neurosci. 15:967174. doi: 10.3389/fnmol.2022.967174.
- Nørgaard M, Ehrenstein V, Vandenbroucke JP. 2017. Confounding in observational studies based on large health care databases: problems and potential solutions-a primer for the clinician. Clin Epidemiol. 9:185–193. doi: 10.2147/CLEP.S129879.
- Nounu A, Kar SP, Relton CL, Richmond RC. 2022. Sex steroid hormones and risk of breast cancer: a two-sample Mendelian randomization study. Breast Cancer Res. 24(1):66. doi: 10.1186/s13058-022-01553-9.
- Ouanes S, Rabl M, Clark C, Kirschbaum C, Popp J. 2022. Persisting neuropsychiatric symptoms, Alzheimer’s disease, and cerebrospinal fluid cortisol and dehydroepiandrosterone sulfate. Alzheimers Res Ther. 14(1):190.
- Pan X, Wu X, Kaminga AC, Wen SW, Liu A. 2019. Dehydroepiandrosterone and dehydroepiandrosterone sulfate in Alzheimer’s disease: a systematic review and meta-analysis. Front Aging Neurosci. 11:61. doi: 10.3389/fnagi.2019.00061.
- Parra KL, Alexander GE, Raichlen DA, Klimentidis YC, Furlong MA. 2022. Exposure to air pollution and risk of incident dementia in the UK Biobank. Environ Res. 209:112895. doi: 10.1016/j.envres.2022.112895.
- Prins BP, Kuchenbaecker KB, Bao Y, Smart M, Zabaneh D, Fatemifar G, Luan J, Wareham NJ, Scott RA, Perry JRB, et al. 2017. Genome-wide analysis of health-related biomarkers in the UK household longitudinal study reveals novel associations. Sci Rep. 7(1):11008. doi: 10.1038/s41598-017-10812-1.
- Rhew SH, Kravchenko J, Lyerly HK. 2021. Exposure to low-dose ambient fine particulate matter PM2.5 and Alzheimer’s disease, non-Alzheimer’s dementia, and Parkinson’s disease in North Carolina. Plos One. 16(7):e253253. doi: 10.1371/journal.pone.0253253.
- Rodriguez-Carrillo A, Remy S, D’Cruz SC, Salamanca-Fernandez E, Gil F, Olmedo P, Mustieles V, Vela-Soria F, Baken K, Olea N, et al. 2023. Kisspeptin as potential biomarker of environmental chemical mixture effect on reproductive hormone profile: a pilot study in adolescent males. Sci Total Environ. 868:161668. doi: 10.1016/j.scitotenv.2023.161668.
- Sattar N, Preiss D. 2017. Reverse causality in cardiovascular epidemiological research: more common than imagined? Circulation. 135(24):2369–2372. doi: 10.1161/CIRCULATIONAHA.117.028307.
- Skrivankova VW, Richmond RC, Woolf BAR, Yarmolinsky J, Davies NM, Swanson SA, VanderWeele TJ, Higgins JPT, Timpson NJ, Dimou N, et al. 2021. Strengthening the reporting of observational studies in epidemiology using Mendelian randomization: the STROBE-MR statement. JAMA. 326(16):1614–1621. doi: 10.1001/jama.2021.18236.
- Solerte SB, Fioravanti M, Schifino N, Cuzzoni G, Fontana I, Vignati G, Govoni S, Ferrari E. 1999. Dehydroepiandrosterone sulfate decreases the interleukin-2-mediated overactivity of the natural killer cell compartment in senile dementia of the Alzheimer type. Dement Geriatr Cogn Disord. 10(1):21–27. doi: 10.1159/000017093.
- Strac DS, Konjevod M, Perkovic MN, Tudor L, Erjavec GN, Pivac N. 2020. Dehydroepiandrosterone (DHEA) and its sulphate (DHEAS) in Alzheimer’s disease. Curr Alzheimer Res. 17(2):141–157. doi: 10.2174/1567205017666200317092310.
- Tainio M, Jovanovic Andersen Z, Nieuwenhuijsen MJ, Hu L, de Nazelle A, An R, Garcia LMT, Goenka S, Zapata-Diomedi B, Bull F, et al. 2021. Air pollution, physical activity and health: a mapping review of the evidence. Environ Int. 147:105954. doi: 10.1016/j.envint.2020.105954.
- Thangavel P, Park D, Lee YC. 2022. Recent insights into particulate matter (PM(2.5))-mediated toxicity in humans: An overview. Int J Environ Res Public Health. 19(12):7511.
- Tsai TL, Lin YT, Hwang BF, Nakayama SF, Tsai CH, Sun XL, Ma C, Jung CR. 2019. Fine particulate matter is a potential determinant of Alzheimer’s disease: A systemic review and meta-analysis. Environ Res. 177:108638. doi: 10.1016/j.envres.2019.108638.
- Underwood E. 2017. The polluted brain. Science. 355(6323):342–345. doi: 10.1126/science.355.6323.342.
- Wang L, Wei LY, Ding R, Feng Y, Li D, Li C, Malko P, Syed Mortadza SA, Wu W, Yin Y, et al. and others. 2020. Predisposition to Alzheimer’s and age-related brain pathologies by PM2.5 exposure: perspective on the roles of oxidative stress and TRPM2 channel. Front Physiol. 11:155. doi: 10.3389/fphys.2020.00155.
- Xie W, You J, Zhi C, Li L. 2021. The toxicity of ambient fine particulate matter (PM2.5) to vascular endothelial cells. J Appl Toxicol. 41(5):713–723. doi: 10.1002/jat.4138.
- Yang L, Wan W, Yu C, Xuan C, Zheng P, Yan J. 2022. Associations between PM(2.5) exposure and Alzheimer’s Disease prevalence Among elderly in eastern China. Environ Health. 21(1):119. doi: 10.1186/s12940-022-00937-w.
- Younan D, Petkus AJ, Widaman KF, Wang X, Casanova R, Espeland MA, Gatz M, Henderson VW, Manson JE, Rapp SR, et al. 2020. Particulate matter and episodic memory decline mediated by early neuroanatomic biomarkers of Alzheimer’s disease. Brain. 143(1):289–302. doi: 10.1093/brain/awz348.
- Zhao K, Li J, Du C, Zhang Q, Guo Y, Yang M. 2021. Ambient fine particulate matter of diameter </= 2.5 mum and risk of hemorrhagic stroke: a systemic review and meta-analysis of cohort studies. Environ Sci Pollut Res Int. 28(17):20970–20980. doi: 10.1007/s11356-021-13074-7.
- Zheng J, Baird D, Borges MC, Bowden J, Hemani G, Haycock P, Evans DM, Smith GD. 2017. Recent developments in Mendelian randomization studies. Curr Epidemiol Rep. 4(4):330–345. doi: 10.1007/s40471-017-0128-6.