Abstract
To study the pathogenesis of a H7N1 highly pathogenic avian influenza virus strain, specific pathogen free chickens were inoculated with decreasing concentrations of virus: 105.5 median embryo lethal dose (ELD50) (G1), 103.5 ELD50 (G2) and 101.5 ELD50 (G3). Disease progression was monitored over a period of 16 days and sequential necropsies and tissue samples were collected for histological and immunohistochemical examination. Viral RNA loads were also quantified in different tissues, blood, oropharyngeal swabs, and cloacal swabs using quantitative real-time reverse transcriptase-polymerase chain reaction (RT-qPCR). Clinical signs of depression, apathy, listlessness, huddling and ruffled feathers were recorded in G1 and a few G2 birds, whilst neurological signs were only observed in chickens inoculated with the highest dose. Gross lesions of haemorrhages were observed in the unfeathered skin of the comb and legs, and skeletal muscle, lung, pancreas and kidneys of birds inoculated with 105.5 ELD50 and 103.5 ELD50 doses. Microscopic lesions and viral antigen were demonstrated in cells of the nasal cavity, lung, heart, skeletal muscle, brain, spinal cord, gastrointestinal tract, pancreas, liver, bone marrow, thymus, bursa of Fabricius, spleen, kidney, adrenal gland and skin. Viral RNA was detected by RT-qPCR in kidney, lung, intestine, and brain samples of G1 and G2 birds. However, in birds infected with the lowest dose, viral RNA was detected only in brain and lung samples in low amounts at 5 and 7 days post infection. Interestingly, viral shedding was observed in oropharyngeal and cloacal swabs with proportionate decrease with the inoculation dose. We conclude that although an adequate infectious dose is critical in reproducing the clinical infection, chickens exposed to lower doses can be infected and shed virus representing a risk for the dissemination of the viral agent.
Introduction
Avian influenza viruses (AIVs) in poultry have been categorized into two groups according to their ability to produce clinical signs and the severity of disease. The low-pathogenicity AIVs do not cause clinical signs or produce a mild localized infection limited to the respiratory or gastrointestinal tracts. In contrast, the highly pathogenic avian influenza viruses (HPAIVs) have been associated with an acute fatal disease as a consequence of systemic replication (Swayne & Halvorson, Citation2008).
Based on the antigenic diversity of the two surface glycoproteins of the AIV, 16 different haemagglutinin (HA) subtypes and nine different neuraminidase subtypes have been identified (Alexander, Citation2007). Some viruses of H5 and H7 subtypes are associated with high pathogenicity in chickens, although the majority of the H5 and H7 subtypes are of low pathogenicity (Panigrahy et al., 2002 ; Swayne & Halvorson, Citation2008). The difference in the pathogenicity is mainly determined by the HA gene and the presence of additional basic amino acids at the proteolytic cleavage site. HA of low-pathogenicity AIVs is cleaved by trypsin-like proteases that are associated with respiratory and intestinal epithelial cells, confining the infection to these sites. In contrast, HA from HPAIVs can be cleaved by the aforementioned proteases and also by ubiquitous furin proteases, leading to a generalized infection (Swayne, Citation2007).
Infections by some HPAIVs produce serious outbreaks in poultry with mortality rates that approach 100% in the affected flocks (Alexander, Citation2000). HPAIVs have been shown to transmit from birds to people, representing a significant threat to human health (Joseph et al., Citation2007). This is the case for the H5N1 HPAIV that emerged in Hong Kong in 1997 affecting chicken farms and also causing infection and death in people (Shortridge et al., Citation1998; Subbarao et al., Citation1998). H7 subtype viruses have also been implicated in the direct transmission to humans: H7N7 and H7N3 viruses caused infection in people related with the poultry industry in the Netherlands in 2003 (Fouchier et al., Citation2004) and in British Columbia, Canada in 2007, respectively (Tweed et al., Citation2004; Joseph et al., Citation2007).
HPAIVs are able to produce clinical disease and death in poultry by a variety of mechanisms including dysfunction of the nervous, cardiovascular and endocrine systems (Swayne, Citation1997). Although the mechanisms of pathogenicity and tissue tropism of many HPAIV strains show similar clinical presentations (Kobayashi et al., Citation1996b; Nakamura et al, 2008), a unique conclusion about the dynamics of the disease could not be generalized for all HPAIV strains (Feldman et al., 2001). The aim of the present study was to assess the pathogenicity of the A/chicken/Italy/5093/99 H7N1 strain, which was associated with a serious epidemic in Italy (Capua et al., Citation2000). To reproduce a natural infection, birds were inoculated by the intranasal route with one of three different doses (105.5 median embryo lethal dose [ELD50], 103.5 ELD50 and 101.5 ELD50). Dynamics of infection, distribution of lesions and viral antigen, viraemic status and viral shedding were evaluated by means of immunohistochemical and quantitative real-time reverse transcriptase-polymerase chain reaction (RT-qPCR) techniques.
Materials and Methods
Virus
The AIV A/chicken/Italy/5093/99 H7N1 of the fifth egg passage was kindly provided by Dr Ana Moreno from the Istituto Zooprofilattico Sperimentale della Lombardia e dell’ Emilia Romagna in Brescia, Italy. After arrival at the Centre de Recerca en Sanitat Animal (CReSA), the virus was passaged once and propagated in 9-day-old to 11-day-old specific pathogen free (SPF) embryonated fowls eggs. The ELD50 was calculated as described previously (Reed & Muench, Citation1938). The amino acid sequence at the HA0 cleavage site for the isolate used in the present experimental infection was PEIPKGSRVRR*GLF, which was identical to the cleavage site sequence of H7N1 HPAIV strains isolated in Italy during the epidemic of 1999 and 2000 (Campitelli et al., Citation2008). The intravenous pathogenicity index was performed as described previously (OIE, Citation2008); a score of 2.8 was obtained, thereby confirming the highly pathogenic phenotype of this isolate.
Experimental design
Birds
Eighty SPF chickens (SPAFAS, Charles River), were hatched and subsequently placed in negative-pressure isolators under biosafety level 3 containment conditions at the CReSA for the duration of the experiment. Bird care and all procedures were performed in accordance with the regulations required by the Ethics Committee of Animal and Human Experimentation of the Universitat Autònoma de Barcelona.
Infection
Fifteen-day-old SPF chickens were divided into four groups and placed in separate negative-pressure isolators. Each group was infected with different doses of virus through the intranasal route. The first group (G1) was inoculated with 105.5 ELD50 H7N1 HPAI virus in a final volume of 0.05 ml. The second group (G2) was inoculated with 103.5 ELD50, and the third group (G3) received 101.5 ELD50 virus. The remaining chickens were inoculated with phosphate-buffered saline and served as the sham-inoculated control group (G4). Clinical signs and mortality were monitored daily throughout the 16 days of the experiment. On days 1, 3, 5, 7, 10, and 16 post inoculation (d.p.i.), three birds of the infected and control groups were selected for necropsy (birds were randomly selected at 1 d.p.i. and later biased toward sick or apparently unhealthy birds). Birds found dead as well as those killed humanely for ethical reasons were included in the study.
Histology and immunohistochemistry
Tissue samples from all necropsied birds in the four groups were collected and fixed by immersion in 10% buffered formalin for 24 h. Samples selected included: nasal turbinates, trachea, oesophagus, gizzard, proventriculus, thymus, heart, lung, spleen, liver, small and large intestines (duodenum, jejunum, ileum, caecum/caecal tonsil, rectum), pancreas, adrenal gland, bursa of Fabricius, bone marrow, skin, central nervous system (CNS) (which included the brain and the thoracic spinal cord), myocardium, skeletal muscle (face, breast, and muscle of the lumbar areas), sciatic and vagus nerves. All tissues were embedded in paraffin and duplicate 4 µm sections were cut for haematoxylin and eosin staining and immunohistochemistry for the detection of the type A influenza virus nucleoprotein. Procedures for the immunohistochemistry followed those described (Kreijtz et al., Citation2007). Briefly, an antigen retrieval step was performed using protease XIV (Sigma-Aldrich, USA) for 10 min at 37°C. Samples were then incubated with the primary antibody at a dilution of 1:250 at 4°C overnight. The primary antibody used was a commercially available mouse-derived monoclonal antibody (ATCC, HB-65, H16L-10-4R5), followed by 1-h incubation with biotinylated goat antimouse IgG secondary antibody (Dako Immunoglobulins AS, Denmark). Finally, an avidin–biotin-peroxidase system (Thermo Fisher Scientific, Rockford, Illinois, USA) was used and antigen-antibody reaction was visualized with diaminobenzidine as chromogen. Sections were counterstained with Mayer's haematoxylin. Contribution of nonspecific staining of the primary antibody was evaluated by substitution of the primary antibody with phosphate-buffered saline and by performing the standard method with tissues of non-infected SPF chicken embryos. Positive controls consisted of formalin-fixed paraffin-embedded tissues of chicken embryos inoculated with the H7N1 virus and that were positive by RT-qPCR.
Viral RNA quantification in tissue samples, oropharyngeal and cloacal swabs and blood
Blood samples were collected from G1 chickens at 1, 3 and 5 d.p.i. with 1 ml Alsever's solution (Sigma-Aldrich). Oropharyngeal and cloacal swabs, as well as brain, kidney, intestine and lung samples, were collected from all birds and each placed in 500 µl “RNA later” (Qiagen, Hilden, Germany). All samples were kept at 4°C for 16 h and then stored at –80°C. Viral RNA was extracted from tissue samples and oropharyngeal and cloacal swabs using QIAamp viral mini kit (Qiagen) and following the manufacturer's instructions. Viral RNA was extracted from 150 mg tissue samples or from 140 µl swab, mixed with RNA later, and RNA was eluted in 40 µl. The resulting viral RNA extracts were tested by one-step RT-qPCR for the detection of a highly conserved region of the matrix (M) gene influenza A viruses using the primers described by Spackman et al. (Citation2002) with the amplification conditions described by Busquets et al. (Citation2010a ). This procedure uses an internal positive control to avoid false negative results due to RT-qPCR inhibitors.
Viral RNA quantification was carried out as described by Busquets et al. (Citation2010b ). Briefly, standard curves were obtained by prior amplification of 99 bp of the M gene using the H7N1 strain RNA as a template. The amplified M-gene fragment was cloned into the pGEM-T vector (Promega, USA) and transformed by heat shock into Escherichia coli competent cells (Invitrogen, USA). The recombinant plasmid was purified using a QIAprep Spin kit (Qiagen) followed by digestion with SacI restriction enzyme (New England Biolabs, UK) to obtain overhanging ends, and then converted to blunt ends using DNA polymerase I large (Klenow) fragment (Promega). In-vitro-transcribed RNA was generated from the T7 promoter (RiboMax kit; Promega). The residual template plasmid was removed by several RNase-free DNAse I (Roche, Switzerland) treatments. The RNA transcript obtained was quantified spectrophotometrically at 260 nm (Qubit; Invitrogen). RNA copy numbers were calculated as described (Fronhoffs et al., Citation2002). Ten-fold RNA transcript dilutions, ranging from 6 to 6 x 107 molecules, were used to obtain standard curves. The limit of virus detection for the one-step RT-qPCR assay used in this study was six viral RNA copies per reaction, which is equivalent to 1 ELD50 per reaction. The limit of virus detection obtained on this assay was equivalent to 1.46 log10 viral RNA copies/ml for swab and blood samples, and 2.28 log10 viral RNA copies/g tissue.
Results
Clinical signs, mortality and gross lesions
Clinical signs were limited to G1 and G2, and mortality was only seen in G1 group. Non-specific clinical signs consisting of moderate to severe depression, apathy, listlessness, huddling and ruffled feathers were first noted in 80% of birds from G1 at 2 d.p.i. More severe clinical signs of torticollis, lack of coordination and bilateral paralysis were observed at 6 d.p.i. Mortality was recorded at 3 d.p.i. (one bird), 4 d.p.i. (one bird), 5 d.p.i. (two birds) and 6 d.p.i. (two birds) in this group, but two birds survived until day 10. Gross lesions were observed from 3 d.p.i. and consisted of multifocal to diffuse haemorrhages and cyanosis of the comb and beak, mild diffuse oedema, multifocal haemorrhages in the unfeathered skin of the legs and slight mucous nasal discharge. At necropsy, petechial haemorrhages on leg skeletal muscles and serosal surfaces of the proventriculum were also detected and lasted until 6 d.p.i. From 5 to 7 d.p.i., paleness in the liver, kidney swelling with a prominent lobular pattern, splenomegaly and random multifocal areas of necrosis and haemorrhages in the pancreas were observed. In G2, one bird showed clinical signs and gross lesions similar to the G1 birds at 3 d.p.i. while a second chicken showed necrosis in the comb, pulmonary congestion, kidney swelling and multifocal haemorrhages in the proventricular serosa at 5 d.p.i. The remaining chickens in the G2 group showed listlessness and ruffled feathers, from 3 to 6 d.p.i., and there was no mortality. In G3 and the sham infected group, no mortality, clinical signs or macroscopic lesions were observed.
Histology and immunohistochemistry
Histological lesions associated with the presence of viral antigen were observed in chickens from G1 and G2. In G1 chickens, lesions and specific immunostaining were observed from 3 d.p.i. and persisted until 7 d.p.i. in nearly all of the tissues (). No lesions or immunostaining were detected in birds at 1 d.p.i. or in those birds that survived until 10 d.p.i. in the G1 group. In G2, lesions and specific immunostaining were only detected in one bird on day 3 and another on day 5. In the first case, histological lesions and viral immunostaining were limited to the heart, pancreas and brain, whereas samples collected from the bird at 5 d.p.i. showed lesions and distribution similar to those observed in G1 chickens at 5 d.p.i. No histological lesions or antigen-positive cells were observed in tissues from birds of the sham-infected and G3 groups.
Table 1. Distribution of AIV nucleoprotein antigen determined by immunohistochemistry in tissue samples from G1 chickens inoculated with H7N1 HPAIV.
Histological and immunohistochemical examination of G1 birds revealed severe morphological changes particularly in the respiratory tract, cardiovascular system, pancreas, kidney, adrenal glands and central nervous system. There was a strong correlation between the manifestation of viral antigen and the identification of histological lesions. Presence of viral antigen was not limited to the sites where the histopathologic alterations were visible by haematoxylin and eosin but was also distributed in the surrounding tissue. The brown antigenic staining was generally intense and compact in the nucleus, and in some cells it was also cytoplasmic in distribution, although it was less intense and speckled.
In the respiratory tract, slight to moderate lymphoplasmacytic inflammation in the lamina propria of the nasal cavity, and multifocal areas of loss of cilia, vacuolar degeneration and necrosis of the respiratory epithelium were observed from 3 to 7 d.p.i. Single-cell necrosis was also observed in the olfactory epithelium of the posterior turbinate from 3 to 6 d.p.i. AIV antigen was demonstrated in individual cells in the respiratory and olfactory epithelium as well as in the Bowman's gland cells of the posterior turbinate (). Endothelial cells and intravascular inflammatory cells in the nasal cavity were also positive from 3 to 6 d.p.i. Necrosis and positive immunostaining was also observed in the lateral nasal glands located in the nasal cavity and, sporadically, in salivary glands of the roof of the oral cavity. Interestingly, in one chicken killed at 7 d.p.i., a focal area of nerve fibre degeneration and necrosis, associated with the presence of viral antigen, was observed in the ophthalmic medial branches of the trigeminal nerve located dorsally in the nasal cavity. Lung lesions consisting of an increase of cellularity in the air capillaries of the parabronchi were evident from 3 to 7 d.p.i., mainly due to the presence of heterophils and most probably macrophages inside the capillaries or within the air capillaries’ interstitium. Microthrombosis of capillaries and scattered single-cell necrosis were also seen on these days. The presence of viral antigen was only detected in scattered endothelial cells and macrophages in the air capillaries. No lesions or immunostaining was observed in the trachea of any of the birds evaluated.
Figure 1. Immunohistochemical detection of influenza A viral nucleoprotein in the nasal cavity of SPF chickens inoculated intranasally with 105.5 ELD50 of H7N1 A/Chicken/Italy/5093/99 at 7 d.p.i. Epithelial necrosis in the transition between respiratory and olfactory epithelium of the nasal cavity, associated with the presence of viral antigen (brown staining) in the olfactory (arrow), and respiratory epithelial cells, Bowman glands (a), mucous glands (b) and inflammatory cells in lamina propria from a G1 chicken at 7 d.p.i.
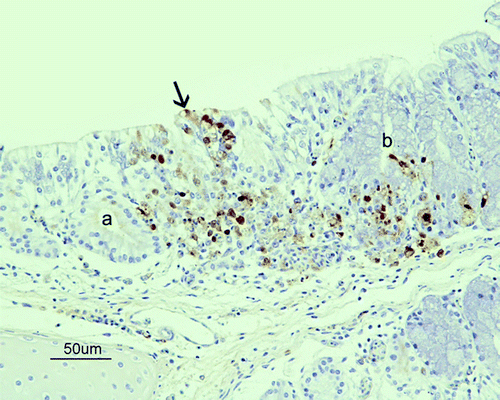
Randomly distributed multifocal to coalescing areas of myocardial degeneration and necrosis, with fibre hyalinization and fragmentation associated with a slight infiltration of macrophages and heterophils, were observed in birds from 3 d.p.i. AIV antigen was observed in myocytes in the necrotic areas as well as in the adjacent inflammatory cells and endothelial cells. Skeletal muscle lesions were slight and were only observed in sections taken from the face and lumbar area. They consisted of scattered areas of fibre degeneration and necrosis surrounded by infiltrated macrophages. Muscle cells and macrophages were positive for viral antigen. Breast muscle lesions were rare and slight.
Histological lesions in the alimentary tract (oesophagus, gizzard, proventriculus, duodenum, colon, caecum, caecal tonsil and cloacae) of lymphocyte depletion and infiltration with macrophages were confined to the submucosa and lymphoid-associated areas in the proventriculus and the caecal tonsils, where AIV antigen was detected in endothelial cells and infiltrating macrophages and heterophils in the submucosa of the proventriculus, caecal tonsil and in the gizzard. Similarly, only mild lesions were observed in the liver and consisted of slight periportal lymphoplasmacytic infiltration and marked Kupffer cell hypertrophy. Erythrophagocytosis in Kupffer cells and occasional fibrinous thrombi in the hepatic sinusoids were also observed from 3 to 5 d.p.i. Both endothelial and Kupffer cells were positive for viral antigen, in addition to inflammatory cells in the portal vessels and periportal areas (). No positivity was observed on hepatocytes or biliary duct epithelial cells. More severe lesions were observed on pancreatic parenchyma, which showed focally extensive to diffuse areas of degeneration and necrosis and stained strongly for the presence of associated viral antigen. Occasionally, there was inflammatory infiltrate associated with necrotic areas, which was also positive for viral antigen from 3 to 7 d.p.i.
Figure 2. Immunohistochemical detection of influenza A viral nucleoprotein in the liver of SPF chickens inoculated intranasally with 105.5 ELD50 (G1) of H7N1 A/Chicken/Italy/5093/99 at 5 d.p.i. Influenza A viral antigen detected in Kupffer cells, endothelial cells and mononuclear intravascular cells in the liver.
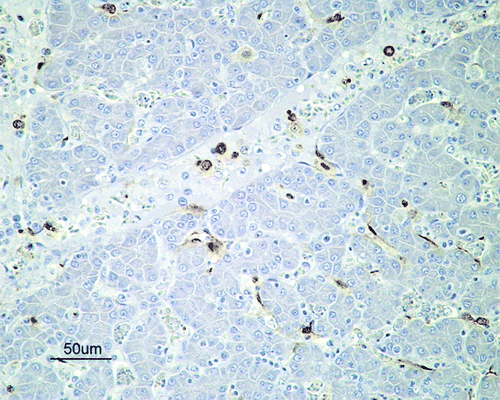
Moderate lesions were observed in the kidney, in which proximal convoluted tubular epithelial cells showed multiple areas of tubular necrosis in association with the presence of viral antigen. AIV was also demonstrated on endothelial cells in the renal interstitium. Inflammatory infiltrate was rarely observed associated with necrotic areas. Severe lesions were detected in adrenal corticotrophic cells, and less frequently chromaffin cells, which showed disseminated vacuolar degeneration and necrosis associated with the presence of AIV antigen. Necrotic areas were infiltrated by macrophages and heterophils that were also positive.
In lymphohaematopoietic organs, moderate erythroid and myeloid cellular depletion were noted in the bone marrow from 5 to 7 d.p.i. The spleen, thymus and bursa of Fabricius had slight to moderate lymphoid depletion of all histological compartments with apoptosis and/or necrosis of lymphocytes from 3 to 7 d.p.i. On these organs the antigen was restricted to endothelial cells and macrophages.
Skin lesions were confined to the face and comb samples, and consisted mainly in focal epidermal necrosis and diffuse oedema, hyperaemia and perivascular mixed inflammatory infiltrate (macrophages and heterophils) that was positive for viral antigen in the underlying dermis. There was microthrombosis and vasculitis affecting dermal vessels that caused focal areas of oedema, haemorrhage and necrosis. Positive staining was also observed in epidermal degenerated keratinocytes, dermal endothelial cells, follicular epithelial cells and endothelial and inflammatory cells in the follicular pulp.
Nervous system lesions were detected in the grey matter and, less frequently, in the white matter, and consisted of multifocal areas of malacia that were evident in all regions of the CNS (spinal cord, cerebellum, brain stem, cerebral hemispheres, and olfactory bulb). These areas of malacia were characterized by neuropil vacuolation, neuronal central chromatolysis and neuronophagia, astrocytes and microgliosis, and were associated with intranuclear and cytoplasmic immunostaining on neurons and glial cells. Perivascular oedema was observed in capillaries near the affected areas, and endothelial cells as well as astrocytes of the glial limiting membrane were positive for AIV antigen. Progressive coalescence of the areas of malacia was observed from 3 to 7 d.p.i. Ependymocytes showed degenerative changes and necrosis and were also positive for AIV antigen from 3 to 7 d.p.i. Slight inflammatory cell infiltration of the choroid plexus that consisted basically of macrophages was detected at 5 and 6 d.p.i. Choroid plexus epithelial cells were also positive for viral antigen.
Thoracic sections of the spinal cord showed necrosis of ependymal cells in the central canal and neurons in the ventral or dorsal grey matter columns in chickens from 3 to 6 d.p.i. (). The peripheral nervous system did not show changes or the presence of viral antigen in any bird.
Figure 3. Immunohistochemical detection of influenza A viral nucleoprotein in the spinal cord of SPF chickens inoculated intranasally with 105.5 ELD50 (G1) of H7N1 A/Chicken/Italy/5093/99 at 3 d.p.i. Viral antigen was detected on ependymal cells surrounding the central canal and in the nearest glial cells and neurons in the grey matter from a G1 chicken at 3 d.p.i.
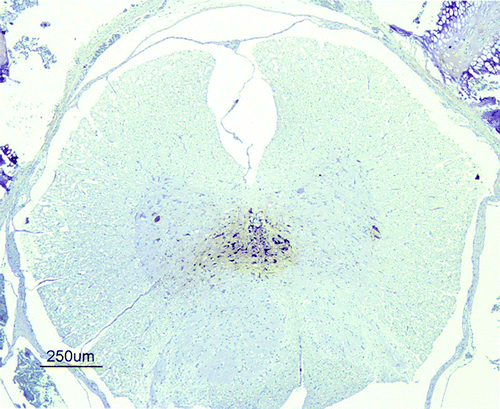
Viral RNA quantification in blood and tissue samples by RT-qPCR
In G1, viral RNA was detected in blood samples at 1 d.p.i. (5.03 log10 viral RNA copies/ml), 3 d.p.i. (9.21 log10 viral RNA copies/ml) and 5 d.p.i. (7.70 log10 viral RNA copies/ml). Regarding the viral load in all of the sampled tissues (CNS, lung, kidney and intestine), viral RNA copies could be detected in G1 birds, inoculated with the highest ELD50, as early as 1 d.p.i. in the brain and kidney (a). In all of the organs sampled, viral RNA copies were found in birds killed humanely or found dead from 3 to 7 d.p.i. In birds from G1 sacrificed at 10 d.p.i., no viral RNA load was detected. The central nervous system and kidney were the tissues that showed the highest viral load during the whole experiment. In G2, viral RNA was detected from 3 d.p.i. and lasted until 7 d.p.i. in all tissues. At 10 d.p.i., viral RNA was detected in the kidney (4.16 log10 viral RNA copies/g) (b). High variability of the viral load was observed among individuals in this group. In G3, inoculated with the lowest ELD50, low amounts of viral genome in the CNS and lung at 5 and 7 d.p.i. were detected (c). Viral RNA copies were not detected in blood and tissue samples of control birds.
Figure 4. Viral RNA detected by RT-qPCR expressed as log10 viral RNA copies per gram of tissue in samples of the brain, lung, intestine, and kidney collected from day 1 to day 10 in G1 (4a) birds and from day 1 to day 16 in group G2 (4b) and G3 (4c). Rates above the bars indicate the relation between positive birds and the total of birds examined. Limit of detection indicated by the dashed line (2.28 log10 viral RNA copies/g tissue).
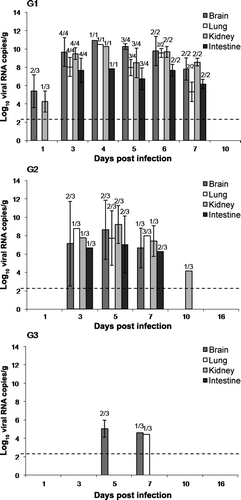
Viral RNA quantification in oropharyngeal and cloacal swabs by RT-qPCR
Viral RNA levels detected in oropharyngeal and cloacal swab samples were proportional to the inoculated ELD50 dose, being highest in G1, intermediate in G2 and low in G3. In G1, one bird showed cloacal shedding at 1 d.p.i., but the viral load levels in oropharyngeal and cloacal swabs were high and similar between 3 and 7 d.p.i. (a). In G2, viral RNA copies were first detected on 3 d.p.i. and gradually increased to peak levels for both samples (oropharyngeal and cloacal) on 5 d.p.i. Detection of viral RNA copies decreased on 7 d.p.i. and reached the lowest level on 10 d.p.i., when oropharyngeal swabs were negative (b). In G3, viral RNA copies were detected in cloacal and oropharyngeal swabs on 5 d.p.i. and low levels could still be detected in cloacal swab samples on 7 d.p.i. (c). The viral genome was not detected in oropharyngeal and cloacal swabs of control birds.
Figure 5. Viral RNA levels detected by RT-qPCR in 1 ml oropharyngeal and cloacal swabs obtained from day 1 to day 10 in G1 (5a) birds and from day 1 to day 16 in group G2 (5b) and G3 (5c). Dotted line indicates the limit of the detection. Rates above the bars indicate the relation between positive birds and the total of birds examined. Limit of detection indicated by the dashed line (1.46 log10 viral RNA copies/ml).
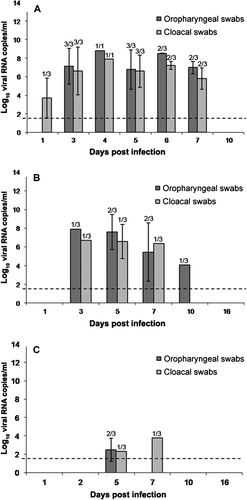
Discussion
The H7N1 HPAIV used in the present study caused an important HPAIV outbreak affecting different bird species in the late 1990s in northern Italy (Capua et al., Citation2000). A complete description of the clinical and pathological findings observed during the epidemic has already been published (Capua et al., Citation2000; Mutinelli et al., Citation2003). However, the pathogenic mechanisms and the dynamics of infection in chickens have not been studied in depth. Here, a time course evaluation of the clinical presentation, lesions and infection/disease outcome and histopathological lesions, as well as virus distribution, detection of viraemia and viral shedding, was assessed in SPF chickens using three different doses of this HPAIV. Remarkable findings were observed that differed from the natural disease. Firstly, fibrinous peritonitis was a usual macroscopic lesion described during the H7N1 HPAIV natural outbreak (Capua et al., Citation2000; Mutinelli et al., Citation2003), but was not observed in the present experimental infection or in other HPAIV experimental infections (Swayne, Citation1997, Citation2007; Perkins & Swayne, Citation2001; Jones & Swayne, Citation2004; Swayne & Beck, Citation2005). Fibrinous peritonitis is a very common lesion in commercial birds, associated with secondary bacterial infections (Barnes et al., Citation2008) and its description in a natural outbreak can reflect a simultaneous viral–bacterial infection (Zanella, Citation2003; Swayne & Halvorson, Citation2008). In the present study, evaluation of birds under experimental conditions allowed the identification of lesions caused only by H7N1 HPAIV. During the outbreak only the pancreas, heart, and nervous tissues were positive for viral antigen by immunohistochemistry on samples of chickens and turkeys (Capua et al., Citation2000; Mutinelli et al., Citation2003). In contrast, in the present study viral antigen was widely distributed in many tissues in chickens infected with the highest and middle doses. These differences in the extension of lesions and viral antigen distribution observed during the natural outbreak compared with the experimental infection can be attributed to the selection of a limited set of tissues (pancreas, kidney, spleen, brain and heart) during the natural outbreak for a rapid diagnosis of the disease. In addition, this extensive distribution of the virus is comparable with other reports of natural and experimental infections caused by H7 and H5 subtype HPAIVs (Swayne, Citation1997; Perkins & Swayne, Citation2001, Citation2002, Citation2003a; Jones & Swayne, Citation2004; Pálmai et al., Citation2007; Swayne, Citation2007; Nakamura et al., Citation2008; Van Riel et al., 2009). Finally, it cannot be ruled out that the detection of a wider distribution of viral antigen can be attributed to differences in the immunohistochemistry methodology used.
Most of the experimental infections with HPAIV strains are carried out using high concentrations of virus to reproduce the early onset and rapid progress observed during the natural infection (Alexander et al., Citation1986; Perkins & Swayne, Citation2002; Swayne & Beck, Citation2005). In the present study, we also evaluated the infectivity of this H7N1 HPAIV strain using two lower doses (103.5 ELD50 and 101.5 ELD50). Our results revealed low morbidity and no mortality with the 103.5 ELD50 dose and a subclinical infection with viral replication and shedding with the 101.5 ELD50 dose. This strongly suggests that although an adequate, and usually high, infectious dose is critical in reproducing the clinical infection, chickens exposed to lower doses can be infected and shed virus representing a risk for the dissemination of the viral agent.
Viraemia has been observed at 1 d.p.i. in chickens infected with H5 HPAIV strains, such as the H5N3 (A/tern/South Africa/61) and H5N2 (A/chicken/Pennsylvania/1379/83), and also in Pekin ducks infected with H5N1 (A/duck/Vietnam/12/05) at 3 d.p.i. (Kishida et al., Citation2004; Swayne & Beck, Citation2005; Beato et al., Citation2007). Moreover, Toffan et al. (Citation2008) have detected viraemia from 1 to 4 d.p.i. in turkeys infected with H7N1 HPAIV. The capacity of those HPAIVs to produce viraemia may be attributed to the ability to infect endothelial cells, as demonstrated by Feldman et al. (2000)Feldman et al. (2001) using chicken embryos infected with H7N1 HPAIV A/Rostock/FVP/34, or by Capua et al. (Citation2002) using embryos of different avian species (chicken, turkey, Muscovy duck) infected with H7N1 HPAIV A/ty/Italy/4580/99. HPAIVs have been frequently reported in endothelial cells of poultry species naturally or experimentally infected (Brown et al., Citation1992; Kobayashi et al., Citation1996b; Mo et al., Citation1996; Swayne, Citation1997, Citation2007; Hooper & Selleck, Citation1998; Suarez et al., Citation1998; Subbarao et al., Citation1998; Capua et al., Citation1999, Citation2000; Feldman et al., 2001; Perkins & Swayne, Citation2001, Citation2003a; Park et al., Citation2002; Jones & Swayne, Citation2004; Muramoto et al., Citation2006; Nakamura et al., Citation2008; Van Riel et al., 2009; Pasick et al., Citation2010). A clear link between the degree of antigenic staining on endothelial cells and the severity of the disease in chickens has been reported (Perkins & Swayne, Citation2001). In addition, HPAIV antigen has also been demonstrated in leucocytes (Feldman et al., 2001; Perkins & Swayne, Citation2003a), heterophils (Capua et al., Citation1999; Jones & Swayne, Citation2004), and monocytes and macrophages (Jones & Swayne, Citation2004; Muramoto et al., Citation2006). In our study, the presence of viral antigen in endothelial cells in venules, arterioles, and in capillary endothelial cells of many organs, in circulating mononuclear cells in association with the detection of viral RNA in blood samples from 1 d.p.i., provides evidence to indicate that this H7 subtype HPAIV strain is able to produce viraemia early in the infection of chickens. This could explain the rapid dissemination of the virus to some tissues; that is, the brain and kidney did not show histological lesions or presence of AIV antigen at 1 d.p.i., but virus was detected by RT-qPCR on this day. Similarly, high levels of viral RNA copies were detected in the intestine and lung, although lesions were almost absent in these organs and few cells were positive for viral antigen. Therefore, the detection of virus in these samples could be attributed to the presence of viral RNA copies in the bloodstream.
As observed in this study and in agreement with previous studies in chickens, ducks, and herring gulls infected with HPAIVs (Swayne & Beck, Citation2005; Brown et al., Citation2008; Bingham et al., Citation2009), oropharyngeal shedding was the principal route of excretion in birds from all groups. This could be explained by the detection of viral antigen in the respiratory and olfactory epithelial cells, as well as salivary and lateral nasal glands in birds inoculated with the higher dose and in some inoculated with the middle dose. With respect to the source for viral genetic material found in birds infected with HPAIV in cloacal swab samples, previous studies have suggested the intestinal contents, urine, bile and pancreatic excretions as sources of virus (Van Riel et al., Citation2009), as all these tracts empty into the cloaca. However, the immunohistochemical results obtained in the present study indicate that the most likely source of virus in the cloacal samples was the kidney, because no viral antigen was found in intestinal epithelial cells, hepatocytes or bile canaliculus. Viral detection in the pancreas was evident, but the volume of pancreatic secretions that empty into the intestinal tract is very low in comparison with the urine (Denbow et al., Citation1988).
The detection of high quantities of viral RNA in the CNS of all inoculated groups, even in the birds inoculated with the lower doses, was also an interesting finding. These results corroborate the strong neurotropism of this particular HPAIV strain as reported during the Italian outbreak in different poultry species, and also demonstrated experimentally in mice (Capua et al., Citation2000; Rigoni et al., Citation2007).
Involvement of the CNS in HPAIV infections has been widely described (Kawaoka & Webster, Citation1988; Brown et al., Citation1992; Kobayashi et al., Citation1996a , Citationb; Swayne, Citation1997; Perkins & Swayne, Citation2002, Citation2003b; Rowe et al., Citation2003; Ellis et al., Citation2004; Kwon et al., Citation2005; Klopfleisch et al., Citation2006; Pálmai et al., Citation2007; Teifke et al., Citation2007; Yamamoto et al., Citation2007; Nakamura et al., Citation2008; Brown et al., Citation2009; Pantin-Jackwood & Swayne, Citation2009; Tang et al., Citation2009; Goletic et al., Citation2010). However, our study shows that, not only the encephalus, but also the spinal cord is consistently affected early in the infection with this H7 subtype HPAIV, which has been previously reported in chickens and mice infected with different HPAIV subtypes (Kobayashi et al., Citation1996a; Lipatov et al., Citation2003; Park et al., 2000 ; Pasick et al., Citation2010). Viral antigen detection was also described in ependymal cells lining the spinal canal of mute swan (Cygnus olor) and whooper swan (Cygnus cygnus) naturally infected with H5N1 HPAIV (Teifke et al., Citation2007). Viral antigen has been also demonstrated in peripheral autonomic ganglia, plexi of the enteric tract and nerve in the skeletal muscle of turkeys, Japanese quail, Bobwhite quail, pheasants and partridges infected with HPAIV (Perkins & Swayne, Citation2001). Moreover, it has been detected in peripheral ganglia that provide innervation to the heart, adrenal gland and gastrointestinal tract of house sparrows (Brown et al., Citation2009), in the myenteric plexus of Pekin ducks (Vascellari et al., Citation2007; Yamamoto et al., Citation2007; Löndt et al., Citation2008) and peripheral nerves and ganglia of domestic ducks (Kwon et al., Citation2005). However, in the present study we did not detect virus in nerves or ganglia of the peripheral nervous system.
The presence and distribution of the viral antigen in the CNS of chickens infected with H7N1 HPAIV is of particular interest and different viral pathways have been hypothesized: the virus can reach the CNS through the olfactory nerves (Majde et al., Citation2007), through the peripheral nervous system (Tanaka et al., Citation2003, Matsuda et al., Citation2004) or through the bloodstream (Mori et al., Citation1995). In our study, viral antigen was detected in the olfactory epithelium of birds inoculated with a higher dose during the first days post infection, but location of the viral antigen in the CNS at 3 d.p.i. was widespread and did not correspond with an entry by the olfactory route; further studies aimed to determine whether the cells that support viral replication in the olfactory mucosa are neurons or supporting stromal cells would clarify this point. The viral entrance to the brain could be via the peripheral nervous system, especially the vagus nerve as demonstrated in mice infected with H5N3 HPAI (Matsuda et al., Citation2004), but this also seems unlikely since the presence of virus in the vagus nerve was not detected. Therefore, based on our results, we suggest that virus dissemination to different organs and tissues and its entry to brain in chicken is most probably through the bloodstream. This assumption is further supported by the finding that astrocytes of the glial limiting membrane were conspicuously positive to viral antigen detection by immunohistochemistry. Migration of the virus across the blood–brain barrier may occur directly from the blood through opening of endothelial cell junctional complexes (para-cellular route) (Lossinsky & Shivers, Citation2004) or using vesiculo-tubular structures (trans-cellular route) (Liu et al., Citation2002; Lossinsky & Shivers, 2004a). Another possibility is the “Trojan horse mechanism” where viral particles are transported through the blood–brain barrier using leukocytes and/or mononuclear cells (Verma et al., Citation2009). Whether influenza viruses use any of these routes has not been investigated; therefore, more studies are needed to discern the underlying mechanism of blood–brain barrier disruption that leads to the severe necrotizing encephalopathy and death of the birds.
Acknowledgements
The present work was partially supported by the European Project SP5B-CT-2007-044098 from the Sixth Framework Programme. The authors thank Dr. Ana Moreno for kindly providing the AIV A/chicken/Italy/5093/9 H7N1 strain. Aida J. Chaves is supported by the Programme Alβan, the European Union Programme of High Level Scholarships for Latin America, Scholarship No. E07D400404CR.
References
- Alexander , D.J. 2000 . A review of avian influenza in different bird species . Veterinary Microbiology , 74 , 3 13 .
- Alexander , D.J. 2007 . An overview of the epidemiology of avian influenza . Vaccine , 25 , 5637 5644 .
- Alexander , D.J. , Parsons , G. & Manvell , R.J. 1986 . Experimental assessment of the pathogenicity of eight avian influenza A viruses of H5 subtype for chickens, turkeys, ducks and quail . Avian Pathology , 15 , 647 662 .
- Barnes , H.J. , Nolan , L.K. & Vaillancourt , J.P. 2008 . Collibacillosis . In Y.M. Saif , A.M. Fadly , J.R. Glisson , L.R. McDougald , L.K. Nolan & D.E. Swayne Diseases of Poultry , 11th edn 691 712 . Ames, IA : Iowa State Press
- Beato , M.S. , Toffan , A. , De Nardi , R. , Cristalli , A. , Terregino , C. , Cattoli , G. and Capua , I. 2007 . A conventional, inactivated oil emulsion vaccine suppresses shedding and prevents viral meat colonisation in commercial (Pekin) ducks challenged with HPAI H5N1 . Vaccine , 25 : 4064 – 4072 .
- Bingham , J. , Green , D.J. , Lowther , S. , Klippel , J. , Burggraaf , S. , Anderson , D.E. , et al. . 2009 . Infection studies with two highly pathogenic avian influenza strains (Vietnamese and Indonesian) in Pekin ducks (Anas platyrhynchos), with particular reference to clinical disease, tissue tropism and viral shedding . Avian Pathology , 38 , 267 278 .
- Brown , C.C. , Olander , H.J. & Senne , D.A. 1992 . A pathogenesis study of highly pathogenic avian influenza virus H5N2 in chickens, using immunohistochemistry . Journal of Comparative Pathology , 107 , 341 348 .
- Brown , J.D. , Stallknecht , D.E. & Swayne , D.E. 2008 . Experimental infections of herring gulls (Larus argentatus) with H5N1 highly pathogenic avian influenza viruses by intranasal inoculation of virus and ingestion of virus-infected chicken meat . Avian Pathology , 37 , 393 397 .
- Brown , J.D. , Stalknecht , D.E. , Berghaus , R.D. and Swayne , D.E. 2009 . Infectious and lethal doses of H5N1 highly pathogenic avian influenza virus for house sparrows (Passer domesticus) and rock pigeons (Columbia livia) . Journal of Veterinary Diagnostic Investigation , 21 : 437 – 455 .
- Busquets , N. , Alba , A. , Napp , S. , Sánchez , A. , Serrano , E. , Rivas , R. , et al. . 2010a . Influenza A virus subtypes in wild birds in North-Eastern Spain (Catalonia) . Virus Research , 149 , 10 18 .
- Busquets , N. , Abad , F.X. , Alba , A. , Dolz , R. , Allepuz , A. , Rivas , R. , et al. . 2010b . Persistence of highly pathogenic avian influenza virus (H7N1) in infected chickens: feather as a suitable sample for diagnosis . Journal of General Virology 91 2307 2313
- Campitelli , L. , Di Martino , A. , Spagnolo , D. , Smith , G.J. , Di Trani , L. , Facchini , M. , et al. . 2008 . Molecular analysis of avian H7 influenza viruses circulating in Eurasia in 1999–2005: detection of multiple reassortant virus genotypes . Journal of General Virology , 89 , 48 59 .
- Capua , I. , Marangon , S. , Selli , L. , Alexander , D.J. , Swayne , D.E. , Pozza , M.D. & Cancellotti , F.M. 1999 . Outbreaks of highly pathogenic avian influenza (H5N2) in Italy during October 1997 to January 1998 . Avian Pathology , 28 , 455 460
- Capua , I. , Mutinelli , F. , Marangon , S. & Alexander , D.J. 2000 . H7N1 Avian influenza in Italy (1999–2000) in intensively reared chickens and turkeys . Avian Pathology , 29 , 537 543 .
- Capua , I. , Mutinelli , F. & Hablovarid , M.H. 2002 . Avian embryo susceptibility to Italian H7N1 avian influenza viruses belonging to different genetic lineages . Archives of Virology 147 , 1611 1621 .
- Denbow , D.M. , Duke , G.E. & Chaplin , S.B. 1988 . Food intake, gastric secretion, and motility as affected by avian pancreatic polypeptide administered centrally in chickens . Peptides , 9 3 , 449 454 .
- Ellis , T.M. , Bousfield , R.B. , Bissett , L.A. , Dyrting , K.C. , Luk , G.S.M. , Tsim , S.T. , et al. . 2004 . Investigation of outbreaks of highly pathogenic H5N1 avian influenza in waterfowl and wild birds in Hong Kong in late 2002 . Avian Pathology , 33 , 492 505 .
- Feldmann , A. , Schafer , M.K.-H. , Garten , W. and Klenk , H-D. 2000 . Targeted infection of endothelial cells by avian influenza virus A/FPV/Rostock/34 (H7N1) in chicken embryos . Journal of Virology , 74 : 8018 – 8027 .
- Feldmann , A. , Looser , N. , Wagner , R. & Klenk , H.-D. 2001 . Targeted influenza virus infection of endothelial cells and leucocytes . International Congress Series , 1219 , 557 571 .
- Fouchier , R.A.M. , Schneeberger , P.M. , Rozendaal , F.W. , Broekman , J.M. , Kemink , S.A.G. , Munster , V.J. , et al. . 2004 . Avian influenza A virus (H7N7) associated with human conjunctivitis and a fatal case of acute respiratory distress syndrome . Proceedings of the National Academy of Sciences of the United States of America 101 , 1356 1361 .
- Fronhoffs , S. , Totzke , G. , Stier , S. , Wernert , N. , Rothe , M. , Bruning , T. , et al. . 2002 . A method for the rapid construction of cRNA standard curves in quantitative real-time reverse transcription polymerase chain reaction . Molecular and Cellular Probes 16 , 99 110 .
- Goletic , T. , Gagic , A. , Residbegovic , E. , Kustura , A. and Kavazovic , A. 2010 . Highly pathogenic avian influenza virus subtype H5N1 in mute swans (Cygnus olor) in central Bosnia . Avian Diseases , 54 : 496 – 501 .
- Hooper , P. & Selleck , P. 1998 . Pathology of low and high virulent influenza virus infections . In D.E. Swayne & R.D. Slemons . Proceedings of the Fourth International Symposium on Avian Influenza , Athens, GA 134 141 . Athens, GA : US Animal Health Association, The University of Georgia .
- Jones , Y.J. & Swayne , D.E. 2004 . Comparative pathobiology of low and high pathogenicity H7N3 Chilean avian influenza viruses in chickens . Avian Diseases , 48 , 119 128 .
- Joseph , T. , McAuliffe , J. , Lu , B. , Jin , H. , Kemble , G. & Subbarao , K. 2007 . Evaluation of replication and pathogenicity of avian influenza A H7 subtype 1 viruses in a mouse model . Journal of Virology , 81 , 10558 10566 .
- Kawaoka , Y. and Webster , R.G. 1988 . Molecular mechanisms of acquisition of virulence in influenza virus in nature . Microbial Pathogenesis , 5 : 311 – 318 .
- Kishida , N. , Sakoda , Y. , Eto , M. , Sunaga , Y. & Kida , H. 2004 . Co-infection of Staphylococcus aureus or Haemophilus paragallinarum exacerbates H9N2 influenza A virus infection in chickens . Archives of Virology , 149 , 2095 2104 .
- Klopfleisch , R. , Werner , O. , Mundt , E. , Harder , T. & Teifke , J.P. 2006 Neurotropism of highly pathogenic avian influenza virus A/chicken/Indonesia/2003 (H5N1) in experimentally infected pigeons (Columbia livia f. domestica) . Veterinary Pathology , 43 463 470 .
- Kobayashi , Y. , Horimoto , Y. , Kawaoka , Y. , Alexander , D.J. & Itakura , C. 1996a . Neuropathological studies of chickens infected with highly pathogenic avian influenza viruses . Journal of Comparative Pathology , 114 , 131 147 .
- Kobayashi , Y. , Horimoto , T. , Kawaoka , Y. , Alexander , D.J. & Itakura , C. 1996b . Pathological studies of chickens experimentally infected with two highly pathogenic avian influenza viruses . Avian Pathology , 25 , 285 304 .
- Kreijtz , J.H.C.M. , Bodewes , R. , van Amerongen , G. , Kuiken , T. , Fouchier , R.A.M. Osterhaus , A.D.M.E. & Rimmelzwaan , G.F. 2007 . Primary influenza A virus infection induces cross-protective immunity against a lethal infection with a heterosubtypic virus strain in mice . Vaccine , 25 , 612 620 .
- Kwon , Y.-K. , Joh , S.-J. , Kim , M.-C. , Sung , H.-W. , Lee , Y.-J. , Choi , J.-G. , et al. . 2005 . Highly pathogenic avian influenza (H5N1) in the commercial domestic ducks of South Korea . Avian Pathology , 34 , 367 370 .
- Lipatov , A.S. , Krauss , S. , Guan , Y. , Peiris , M. , Rehg , J.E. , Perez , D.R. & Webster , R.G. 2003 . Neurovirulence in mice of H5N1 influenza virus genotypes isolated from Hong Kong poultry in 2001 . Journal of Virology , 77 , 3816 3823 .
- Liu , N.Q. , Lossinsky , A.S. , Popik , W. , Li , X. , Gujuluva , C. , Kriederman , B. , et al. . 2002 . Human immunodeficiency virus type 1 enters brain microvascular endothelia by macropinocytosis dependent on lipid rafts and the mitogen-activated protein kinase signaling pathway . Journal of Virology , 7 , 6689 6700 .
- Löndt , B.Z. , Nunez , A. , Banks , J. Nili Hassan , J. , Johnson L. K & Alexander , D.J. 2008 . Pathogenesis of highly pathogenic avian influenza A/turkey/Turkey/1/2005 H5N1 in Pekin ducks (Anas platyrhynchos) infected experimentally . Avian Pathology , 37 , 619 627 .
- Lossinsky , A.S. and Shivers , R.R. 2004 . Structural pathways for macromolecular and cellular transport across the blood–brain barrier during inflammatory conditions. Review . Histology and Histopathology , 19 : 535 – 564 .
- Majde , J.A. , Bohnet , S.G. , Ellis , G.A. , Churchill , L. , Leyva-Grado , V. , Wu , M. , et al. . 2007 . Detection of mouse-adapted human influenza virus in the olfactory bulbs of mice within hours after intranasal infection . Journal of Neurovirology , 13 , 399 409 .
- Matsuda , K. , Park , C.H. , Sunden , Y. , Kimura , T. , Ochiai , K. , Kida , H. & Umemura , T. 2004 . The vagus nerve is one route of transneural invasion for intranasally inoculated influenza A virus in mice . Veterinary Pathology , 41 , 101 107 .
- Mo , I.P. , Brugh , O.J. , Fletcher , G.N. , Rowland , M. and Swayne , D.E. 1996 . Comparative pathology of chickens experimentally inoculated with avian influenza viruses of low and high pathogenicity . Avian Diseases , 41 : 125 – 136 .
- Mori , I. , Komatsu , T. , Takeuchi , K. , Nakakuki , K. , Sudo , M. & Kimura , Y. 1995 . Viremia induced by influenza . Microbiology and Pathogenesis , 19 , 237 244 .
- Muramoto , Y. , Ozaki , H. , Takada , A. , Park , C-H. , Sunden , Y. Umemura , T. 2006 . Highly pathogenic H5N1 influenza virus causes coagulopathy in chickens . Microbiology and Immunology , 50 : 73 – 81 .
- Mutinelli , F. , Capua , I. , Terregino , C. & Cattoli , G. 2003 . Clinical, gross, and microscopic findings in different avian species naturally infected during the H7N1 low- and high-pathogenicity avian influenza low- and high-pathogenicity avian influenza epidemics in Italy during 1999 and 2000 . Avian Diseases , 47 , 844 848 .
- Nakamura , K. , Imada , T. , Imai , K. , Yamamoto , Y. , Tanimura , N. , Yamada , M. , et al. . 2008 . Pathology of specific-pathogen-free chickens inoculated with H5N1 avian influenza viruses isolated in Japan in 2004 . Avian Diseases , 52 , 8 13 .
- OIE . 2008 . Avian influenza . In Manual of Diagnostic Tests and Vaccines for Terrestrial Animals 2008 , 6th edn Office International des Epizooties (OIE). Available online at http://www.oie.int/eng/normes/mmanual/2008/pdf/2.03.04_AI.pdf .
- Pálmai , N. , Erdélyi , K. , Bálint , Á. , Márton , L. , Dán , Á. , Deim , Z. , et al. . 2007 . Pathobiology of highly pathogenic avian influenza virus (H5N1) infection in mute swans (Cygnus olor) , Avian Pathology , 36 , 245 249 .
- Panigraphy , B. , Senne , D. A. and Pedersen , J. C. 2002 . Avian influenza virus subtypes inside and outside the live bird markets, 1993–2000: a spatial and temporal realationship . Avian Diseases , 46 : 298 – 307 .
- Pantin-Jackwood , M.J. and Swayne , D.E. 2009 . Pathogenesis and pathobiology of avian influenza virus infection in birds . Revue Scientifique et Technique , 28 : 113 – 136 .
- Park , C.H. , Ishinaka , M. , Takada , A. , Kida , H. , Kimura , T. , Ochiai , K. & Umemura , T.T. 2002 . The invasion routes of neurovirulent A/Hong Kong/483/97 (H5N1) influenza virus into the central nervous system after respiratory infection in mice . Archives of Virology , 147 , 1425 1436 .
- Pasick , Y. , Berhane , Y. , Hisanaga , T. , Kehler , H. , Hooper-McGrevy , K. , Handel , K. , et al. . 2010 . Diagnostic test results and pathology associated with the 2007 Canadian H7N3 highly pathogenic avian influenza outbreak . Avian Diseases , 54 , 213 219 .
- Perkins , L.E.L. & Swayne , D.E. 2001 . Pathobiology of A/chicken/Hong Kong/220/97 (H5N1) avian influenza virus in seven gallinaceous species . Veterinary Pathology , 38 , 149 164 .
- Perkins , L.E.L. and Swayne , D.E. 2002 . Pathogenicity of a Hong Kong-origin H5N1 highly pathogenic avian influenza virus for emus, geese, ducks, and pigeons . Avian Diseases , 46 : 53 – 63 .
- Perkins , L.E.L. and Swayne , D.E. 2003a . Comparative susceptibility of selected avian and mammalian species to a Hong Kong-Origin H5N1 high-pathogenicity avian influenza virus . Avian Diseases , 47 : 956 – 967 .
- Perkins , L.E.L. & Swayne , D.E. 2003b . Varied pathogenicity of a Hong Kong-origin H5N1 avian influenza virus in four passerine species and budgerigars . Veterinary Pathology , 40 , 14 24 .
- Reed , L.J. and Muench , H. 1938 . A simple method of estimating fifty percent endpoints . The American Journal of Hygiene , 27 : 493 – 497 .
- Rigoni , M. , Shinya , K. , Toffan , A. , Milani , A. , Bettini , F. , Kawaoka , Y. , et al. . 2007 . Pneumo- and neurotropism of avian origin italian highly pathogenic avian influenza H7N1 isolates in experimentally infected mice . Virology , 364 , 28 35 .
- Rowe , T. , Cho , D.S. , Bright , R.A. , Zitzow , L.A. and Katz , J.M. 2003 . Neurological manifestations of avian influenza viruses in mammals . Avian Diseases , 47 : 1122 – 1126 .
- Shortridge , K.F. , Zhou , N.N. , Guan , Y. , Gao , P. , Ito , T. , Kawaoka , Y. , et al. . 1998 . Characterization of avian H5N1 influenza viruses from poultry in Hong Kong . Virology , 252 , 331 342
- Spackman , E. , Senne , D.A. , Myers , T.J. , Bulaga , L.L. , Garber , L.P. Perdue , M.L. 2002 . Development of a real-time reverse transcriptase PCR assay for type A influenza virus and the avian H5 and H7 hemaglutinin subtypes . Journal of Clinical Microbiology , 40 : 3256 – 3260 .
- Suarez , D.L. , Perdue , M.L. , Cox , N. , Rowe , T. , Bender , C. , Huang , J. and Swayne , D.E. 1998 . Comparison of highly virulent H5N1 influenza A viruses isolated from humans and chickens from Hong Kong . Journal of Virology , 72 : 6678 – 6688 .
- Subbarao , K. , Klimov , A. , Katz , J. , Regnery , H. , Lim , W. , Hall , H. , et al. . 1998 . Characterization of an avian influenza A H5N1 virus isolated from a child with a fatal respiratory illness . Science , 16 , 393 396 .
- Swayne , D.E. 1997 . Pathobiology of H5N2 Mexican avian influenza virus infections in chickens . Veterinary Pathology , 31 , 557 567 .
- Swayne , D.E. 2007 . Understanding the complex pathology of high pathogenicity avian influenza viruses in birds . Avian Diseases , 51 , 242 249 .
- Swayne , D.E. and Beck , J.R. 2005 . Experimental study to determine if low-pathogenicity and high-pathogenicity avian influenza viruses can be present in chicken breast and thigh meat following intranasal virus inoculation . Avian Diseases , 49 : 81 – 85 .
- Swayne , D.E. and Halvorson , D.A. 2008 . “ Influenza ” . In Diseases of Poultry , 11th edn , Edited by: Saif , Y.M. , Fadly , A.M. , Glisson , J.R. , McDougald , L.R. , Nolan , L.K. and Swayne , D.E. 153 – 185 . Ames, IA : Iowa State Press .
- Tanaka , H. , Park , C. , Ninomiya , A. , Ozaki , H. , Takada , A. , Umemura , T. & Kida , H. 2003 . Neurotropism of the 1997 Hong Kong H5N1 influenza virus in mice . Veterinary Microbiology , 95 , 1 13 .
- Tang , Y. , Wu , P. , Peng , D. , Wang , X. , Wan , H. , Zhang , P. , et al. . 2009 . Characterization of duck H5N1 influenza viruses with differing pathogenicity in mallard (Anas platyrhynchos) ducks . Avian Pathology , 38 , 457 467 .
- Teifke , J.P. , Klopfleisch , R. , Globig , A. , Starick , E. , Hoffmann , B. , Wolf , P.U. , et al. . 2007 . Pathology of natural infections by H5N1 highly pathogenic avian influenza virus in mute (Cygnus olor) and whooper (Cygnus cygnus) swans . Veterinary Pathology , 44 , 137 143 .
- Toffan , A. , Serena Beato , M. , De Nardi , R. , Bertoli E. , Salviato , A. , Cattoli , G. , et al. . 2008 . Conventional inactivated bivalent H5/H7 vaccine prevents viral localization in muscles of turkeys infected experimentally with low pathogenic avian influenza and highly pathogenic avian influenza H7N1 isolates . Avian Pathology , 37 , 407 412 .
- Tweed , S.A. , Skowronski , D.M. , David , S.T. , Larder , A. , Petric , M. Lees , W. 2004 . Human illness from avian influenza H7N3, British Columbia . Emerging Infectious Diseases , 10 : 2196 – 2199 .
- Van Riel , D. , van den Brand , J.M.A. , Munster , V.J. , Besteboer , T.M. , Fouchier , R.A.M. , Osterhaus , A.D.M.E. & Kuiken , T. 2009 . Pathology and virus distribution in chickens naturally infected with highly pathogenic avian influenza A virus (H7N7) during the 2003 outbreak in The Netherlands . Veterinary Pathology , 46 , 971 976 .
- Vascellari , M. , Granato , A. , Trevisan , L. , Basilicata , L. , Toffan , A. , Milani , A. & Mutinelli , F. 2007 . Pathologic findings of highly pathogenic avian influenza virus A/Duck/Vietnam/12/05 (H5N1) in experimentally infected Pekin ducks, based on immunohistochemistry and in situ hybridization . Veterinary Pathology , 44 , 635 642 .
- Verma , S. , Lo , Y. , Chapagain , M. , Lum , S. , Kumar , M. , Gurjav , U. , et al. . 2009 . West Nile virus infection modulates human brain microvascular endothelial cells tight junction proteins and cell adhesion molecules: transmigration across the in vitro blood–brain barrier . Virology , 382 , 425 433 .
- Yamamoto , Y. , Nakamura , K. , Kitagawa , K. , Ikenaga , N. , Yamada , M. , Mase , M. & Narita , M. 2007 . Severe nonpurulent encephalitis with mortality and feather lesions in call ducks (Anas platyrhyncha var. domestica) inoculated intravenously with H5N1 highly pathogenic avian influenza virus . Avian Diseases , 51 , 52 57 .
- Zanella , A. 2003 . Avian influenza attributable to serovar H7N1 in light layers in Italy . Avian Diseases , 47 , 1177 1180 .