Abstract
Synthetic pigments from petrochemicals have been extensively used in a wide range of food products. However, these pigments have adverse effects on human health that has rendered it obligatory to the scientific community in order to explore for much safer, natural, and eco-friendly pigments. In this regard, exploiting the potential of agri-food wastes presumes importance, extracted mainly by employing green processing and extraction technologies. Of late, pigments market size is growing rapidly owing to their extensive uses. Hence, there is a need for sustainable production of pigments from renewable bioresources. Valorization of vegetal wastes (fruits and vegetables) and their by-products (e.g. peels, seeds or pomace) can meet the demands of natural pigment production at the industrial levels for potential food, pharmaceuticals, and cosmeceuticals applications. These wastes/by-products are a rich source of natural pigments such as: anthocyanins, betalains, carotenoids, and chlorophylls. It is envisaged that these natural pigments can contribute significantly to the development of functional foods as well as impart rich biotherapeutic potential. With a sustainability approach, we have critically reviewed vital research information and developments made on natural pigments from vegetal wastes, greener extraction and processing technologies, encapsulation techniques and potential bioactivities. Designed with an eco-friendly approach, it is expected that this review will benefit not only the concerned industries but also be of use to health-conscious consumers.
GRAPHICAL ABSTRACT
Schematic representation of vegetal waste utilization for the exploitation of the pigments and their functional properties.
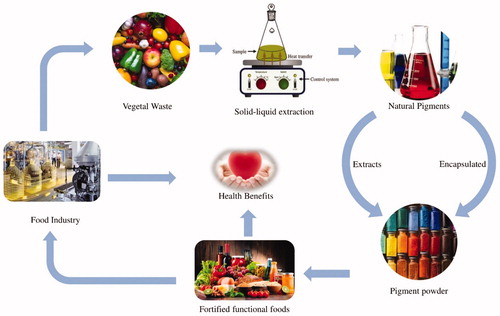
Valorization of vegetal wastes and by-products to produce natural pigments
Recent developments in green-extraction techniques for the isolation of natural pigments
Encapsulation of pigments from vegetal wastes
Natural pigments impart rich health benefits.
Highlights
Introduction
Historically, natural pigments or colorants used in food applications were obtained from renewable resources such as from plants or from microbes (e.g. bacteria, algae, fungi, and yeasts) and insects. Extracts of: saffron, paprika, turmeric, and various flowers are some examples from which natural pigments were traditionally derived [Citation1]. With wide profound applications, synthetic pigments gained popularity as one of the main food coloring (pigments) compounds. These synthetic compounds have been related with high stability, low production costs, and high tinctorial strength as well as ease of application in the food system. Edible pigments are ubiquitously present in plant origin foods, especially in colored fruits and vegetables (). Nature provides a wide array of colors to fruits and vegetables, and thus is a pigment enriched food resource. These pigments occur mainly as secondary plant metabolites in fruits and vegetables and are usually coined as natural pigments. Nowadays, an increasing trend has been witnessed wherein consumers are more concerned about the diet pattern they consume and their health impact.
Table 1. Natural pigments extracted from vegetal wastes.
Owing to consumers demand, considerable innovative research has been undertaken to explore for natural and safe food ingredients such as natural pigments with potential health benefits () [Citation2–53]. This has rendered it a necessity to find alternatives for chemical-based pigments [Citation54]. Researchers are continually looking for natural food coloring alternatives to meet the market challenges and demands, and those which can fulfill and meet regulatory restrictions for food and biotherapeutics applications.
The food processing industry produces enormous amount of waste and by-products, and these form the second-largest generator of wastes after household sewage wastes [Citation55]. Processing waste generation is continuously increasing with increased industrialization and urbanization. Fruit and vegetable wastes and/or by-products obtained from the processing industries mainly contains the skin portion or the peel, seeds, and pomace. These are excellent resources for ingredients such as protein, peptides, polysaccharides, dietary fibers, and others along with bioactive-functional ingredients such as polyphenols, antioxidants and antimicrobial compounds including natural pigments [Citation56]. Among all of these, pigments are considered to be natural, safe, and possess potential antioxidant activities, as well as can be a potential coloring material source in food applications [Citation57]. These natural pigments, with coloring and pharmacological properties, have wide applications in the food industry and can be utilized in product development (functional and nutraceutical foods) because of their health-related beneficial effects [Citation58]. On the other hand, due to the shortage of pilot testing of the innovative technologies, the exploitation of the vegetal wastes for pigment extraction has been highly limited [Citation55]. Various innovative techniques, such as pulsed-electric field, pulse-light, high pressure processing, ionizing radiation have been explored for the extraction of new potential food colorants from vegetal wastes [Citation59]. The wastes contain significant amounts of phyto-pigments which can help in overcoming cardiovascular, cerebrovascular, and certain types of cancers [Citation60]. These natural pigments can be isolated from wastes and by-products and can possess potential bioactivities such as antimicrobial, antioxidant, antiproliferative, anti-inflammatory properties, etc. [Citation60]. Due to significant nutritional and beneficial health properties, as well as phyto-pigment-antioxidant nature, these plants secondary metabolites can be considered as ‘functional food ingredients’.
In this review, the focus is on the major types of natural pigments in vegetal wastes, effective utilization of vegetal wastes for extraction of natural pigments, and encapsulation technologies to enhance the stability of natural pigments, as well as their applications during the development of novel functional foods. From a biotechnological viewpoint, recent developments on the utilization of vegetal wastes for the exploitation of natural pigments as bioactives in a sustainable manner is also highlighted.
Designed with an eco-friendly environmental concern based approach and keeping in mind circular economy concepts, it is expected that this review will benefit not only the dependent industries but also be of use to health-conscious consumers.
Natural pigments – types and sources
Pigments are classified as either natural or synthetic, water and/or fat-soluble, organic or inorganic types. Further, they are also classified based on their structural affinities, solubility and natural occurrence. The classification of natural pigments primarily extracted from vegetal wastes are specified in the . Most often, these are mainly divided into 04 major groups: anthocyanins, betalains, carotenoids and chlorophyll (see ) [Citation61].
Anthocyanins
Anthocyanins are natural, water-soluble, nontoxic, vacuolar and the largest group of polyphenolic pigments. These are, known to be nearly 700 distinctive structures of anthocyanins [Citation62]. Among vegetables- red cabbage, black carrot, red radish, purple sweet potatos and amongst fruits- blackcurrants, cherries, and berries (e.g. strawberries, elderberries, blackberries, chokeberries, black raspberries, blueberries, black goji berries, concord grapes etc.) have been identified as rich sources of anthocyanins. Anthocyanins belong to the class of flavonoids with the major plant-based anthocyanins being: cyanidin, pelargonidin, delphinidin, peonidin, petunidin and malvidin. The color difference in anthocyanins is due to the structural differences in hydroxyl groups, their number and the position of sugar moieties [Citation9].
Wastes and by-products from food processing industries, like wine or the juice industry, are considered to be additional enriched sources for anthocyanin pigments, which can be exploited as natural colorants for various food applications [Citation55]. Juice processing industries inevitably produce waste and by-products. Blackberry residues are reported to be important sources of natural colorants and nutraceuticals due to the presence of high anthocyanin contents (4.31 mg Cy3GlE/g) [Citation6]. Apple peel can also be a good source of this valuable pigment. The anthocyanin content of Rome beauty apple peels was reported to be 169.7 mg cyanindin 3-glucoside equivalent/100g of dried peel powder [Citation2]. Grape pomace is a well-known source of polyphenolic compounds including: anthocyanins, pro-anthocyanidins, trans-resveratrol and quercetin [Citation63]. Barros et al. [Citation18], extracted anthocyanins (3.4 mg/g raw material) from freeze-dried jaboticaba peel powder by using an hydroalcoholic mixture (50 v/v), of three different acids (formic acid- 88%, acetic acid- 99.7%, and ortho-phosphoric acid- 85%) at three pH ranges (1.0, 2.0 and 3.0), followed by an ultrasonic bath (frequency- 40 kHz and power- 150 W).
Betalains
Betalains are next to anthocyanins in terms of their naturally occurring pigments and are mainly divided as betacyanins and betaxanthins. These pigments impart red-purple and yellow-orange colors to fruits and vegetables, respectively [Citation64]. These compounds are commonly found as a pigment in Beta vulgaris (beetroot), in some fruits like Opuntia (prickly pears), Hylocereus and Mamillaria species [Citation65]. Betalains are immonium conjugates of betalamic acid with cyclo-dopa and amino groups (amino acids, amines or their derivatives) [Citation61]. The betalamic acid moieties conjugated with amino acids or amines, are termed betaxanthins. Condensation reactions between the betalamic acid and cyclo-dopa producing betanidin is a precursor of the betacyanins. Due to the presence of glycosylation or acylation, betanidins exhibit 29 different structures in nature. Betanins have also undergone several chemical reactions (isomerization, deglycosylation, hydrolysis, decarboxylation, and dehydrogenation) [Citation66], but no reported study specifies the number of isomers or degradation products of betanins.
Several studies have been carried out on the extraction of betalains from vegetal wastes [Citation25–33]. Kushwaha et al. [Citation67] optimized an ecofriendly method for the extraction of betalains from beetroot pomace by changing different experimental variables such as the solid to liquid ratio, time, temperature and pH. Results showed the yield of betacyanin and betaxanthin to range from 1.75–62 and 1.79–61.62 mg/L, respectively. Similarly, the water extract of red dragon fruit (Hylocereus polyrhizus) peels were observed to have a betalain content of 30.18 mg/100 g of dry peel [Citation26]. Further, microwave assisted extraction of betalains (9 mg/L of betalain content) from dried peel powder of dragon fruits has also been optimized [Citation68]. Melgar et al. [Citation69] extracted betalains from peels of Opuntia engelmannii using ultrasound and microwave-assisted extraction and reported betalain content to be 201.6 and 132.9 mg/g, with and without methanol, respectively.
Carotenoids
Among natural pigments, carotenoids are the most vital phytochemicals which are lipophilic in nature accounting for the red, yellow or orange color range in a variety of fruits and vegetables. Carotenoids widely occur as all-trans and cis-isomers forms [Citation70]. Carotenoids are mainly categorized into two: carotenes (α-carotene, β-carotene and lycopene); and xanthophylls (lutein, zeaxanthin, and β-cryptoxanthin). Both carotenes and xanthophylls contain chains of hydrogen and carbon, but the presence of hydroxyl groups which represent oxygenated carotenoids in structure, to differentiate them [Citation71]. Paprika is one of the rich sources of red carotenoids [Citation50]. The capsanthin pigment is found in the fruits of Capsicum annuum (paprika) or Capsicum frutescens (piri piri). As per EU food legislation, capsanthin is listed as a natural food colorant (E160c), however, in USA legislation, oleoresin of paprika is permitted as a food colorant [Citation72]. Commercially available carotenoids used as food coloring compounds are usually synthesized chemically [Citation73]. However, currently, to boost green consumerism, these pigments can be produced from wastes generated by the fruit and vegetable processing industry. For example, paprika waste, (lutein- 232.60 μg/g) [Citation50], tomato peel (carotenoids-253.5 μg/g) [Citation47], carrot peel [Citation42] and their by-products (carotenoids- 82.66 μg/g) [Citation74]. Further, in spinach by-products, lutein recovery is reported to be 70% and chlorophyll recovery 96% [Citation48]). Vegetal wastes are rich sources of natural carotenes exhibiting provitamin-A activity. In addition, seeds of Bixa Orellana (achiote), a source of annatto (natural pigment), has been used to color dairy formulations. However, there are no reports available on the natural pigments obtained from wastes or by-products of this plant, which should be explored in the near future.
Several studies have been reported on the extraction of carotenoids from vegetal wastes [Citation34–39]. Recently, Tiwari et al. [Citation74] extracted carotenoids from carrot pomace using a green extraction approach (flaxseed oil as green solvent) in combination with innovative extractions (ultrasonication, high shear dispersion) techniques. They concluded that the biorefinery approach is helpful to improve the extraction of carotenoids (82.66 μg/g) and β-carotene (78.37 μg/g) from carrot pomace. Goula et al. [Citation39] adopted green extraction methods (sunflower oil and soy oil as green solvents) in combination with ultrasound for the extraction of carotenoids from pomegranate peels (0.6134 and 0.6715 mg carotenoids/100 g of dry peels in sunflower oil and soy oil, respectively). Kang et al. [Citation50] optimized the method for lutein extraction by accelerated solvent extraction using response surface methodology from paprika leaves waste. These researchers reported the lutein content of paprika leaves extracted in ethanol to be 232.60 μg/g of the leaves. Kehili et al. [Citation45] extracted lycopene and β-carotene from tomato by-products using supercritical CO2 extraction and reported the recovery range to be 32 to 61% for lycopene and 28.38 to 58.8% for β-carotene. Similarly, Wang [Citation46] extracted β-carotene (18.50 mg/100 g sample) from pumpkin seeds using supercritical CO2 extraction. On the other note, de Andrade et al. [Citation47] considered on carotenoids recovery (by supercritical fluid extraction) from various vegetal wastes. They reported on waste from the peel of the sweet potato, tomato, apricot, pumpkin and peach peels and pepper wastes (165.1, 253.5, 285.1, 142.0, 59.5, and 109.2 μg/g dry weight basis, respectively). Recently, ionic liquids in combination with ultrasonic-assisted extraction have been introduced for the extraction of carotenoids from the orange peel [Citation75].
Chlorophyll
Chlorophylls is an oil-soluble, amphiphilic green pigment which is extensively dispersed in plants [Citation76]. Chlorophyll molecules constitute a hydrophilic (porphyrin) group head and a lipophilic hydrocarbon tail (phytol group). Similar to lutein, due to its lipophilic hydrocarbon chains as phytol tail, it is generally considered insoluble in polar solvents [Citation77]. Chlorophyll, found in plant foods are of two types: chlorophyll a and chlorophyll b. At position 7-carbon, methyl group (-CH3) it is present in chlorophyll a whereas an aldehyde group (-CHO) is present in chlorophyll b. The difference in the structure leads to different colors of chlorophylls: blue-green (chlorophyll a) or yellow-green (chlorophyll b) [Citation78]. Chlorophyll is well recognized, natural, green permitted food colorant (EU standard number is E140) [Citation79]. According to the US legislation for natural food colorants published in Title 21 CFR 73, only copper chlorophyllin (water soluble) is authorized as a natural green food-colorant (CFR Section 73.125) [Citation80]. Demand for chlorophyll is continuously increasing, corresponding to enhanced awareness on the use of natural colorants as well as health benefits imparted by them.
Chlorophylls are used for coloring food formulations and can be extracted from many types of vegetal wastes. However, there are no reports on the utilization of vegetal wastes for the extraction of chlorophylls and their further application as a colorant in food formulations. Limited available studies have explained the coloring attributes of chlorophyll. Considering the extraction of chlorophyll as an antioxidant, Zeyada et al. [Citation43] have analyzed methanolic extracts of cucumber and watermelon peels for their chlorophyll content using HPLC and have recorded the content to be 3.46 and 5.28 mg/g (d.w.) respectively. They reported that except for the tomato peel, cucumber and watermelon peels exhibit the highest antioxidant activity among all the tested vegetable varieties, which was attributed to a high chlorophyll content. According to the study of Wang et al. [Citation81] results obtained from eight varieties of avocado (Persea americana) peel and indicated them to be a good source of green pigment (Chlorophyll) (range of 25 to 66 μg/g) . Hence, avocado, being a rich source of green chlorophyll pigment, can be a platform to fortify or use as a coloring agent in various food formulations. Derrien et al. [Citation48,Citation49] optimized the extraction methods for lutein and chlorophyll from spinach (Spinacia oleracea) by-products with supercritical CO2 [48] and green solvent extraction [Citation49] using response surface methodology. Under optimum conditions, they reported the extraction yield for lutein and chlorophyll to be 72% lutein and 50% of chlorophyll [Citation48], whereas, 70% for lutein and 96% for chlorophyll were recorded using green solvent extraction [Citation49].
According to these authors’ knowledge, there are no studies available which is practically based on the valorization of vegetal wastes for chlorophyll extraction. Hence, this review discusses avenues for future exploitation of the vegetal wastes in order to obtain natural green pigments. The addition of these green pigments valorized from vegetal wastes, in food formulations, synergizes the coloring attributes with an antioxidant potential which mainly favors the green consumerism and limits the application of synthetic coloring compounds in food.
Extraction techniques
Extraction of pigments involves the production of natural pigments for food applications. For the extraction of natural colorants, the first step is to obtain the crude pigment from the plant resources. Usually, conventional methods such as Soxhlet extraction techniques using organic/inorganic solvents, maceration or hydro-distillation have been widely used for natural pigment extraction [Citation82]. For conventional extraction, generally water or diluted alcohol are employed for water-soluble pigments, while non-polar solvents are used for the extraction of lipophilic pigments [Citation83]. The non-polar solvents used for the extraction of pigments are mainly of petrochemical origin e.g. hexane (carotenoids from Gac fruit peel [Citation37]), acetone (lycopene from tomato pulp waste [Citation84]), methanol (anthocyanins from eggplant peel [Citation5]), trifluroacetic acid (betalains from pitaya fruits peel [Citation85]). Most of them are mainly toxic in nature, though owing to their volatile nature, they can efficiently dissolve target pigments to facilitate convenient removal. Rather than being technically advantageous, traces of these solvents are unsafe for human consumption and will lead to carcinogenic risks in humans and can also contribute to environmental pollution [Citation82].
Concurrently, traditional or conventional extraction methods have been escorted with assisted extractions techniques impregnated with other physical methods. Moreover, conventional extraction techniques that have shown a low extraction proficiency, are complex, and require a longer extraction time and huge capital investments. With these drawbacks, environmentally friendly innovative techniques are needed to allow safe extraction by using green solvents obtained from natural resources like plant based edible oils, wood etc. The use of greener extraction techniques is now considered to be an emerging, re-discovered and innovative method gaining much importance aimed toward avoiding the adverse effects of synthetic solvents. Nevertheless, these green extraction modes can also improve the extraction efficiency of natural pigments from vegetal sources.
Green extraction techniques
Many studies have been undertaken on the extraction of natural pigments by using synthetic solvents, but in this section, the focus is mainly on green technologies. summarizes the greener extraction techniques that assist with various innovative techniques such as: ultra-sound-assisted extraction (UAE), microwave-assisted extraction (MAE), enzyme-assisted extraction (EAE), supercritical fluid extraction (SFE), pressurized liquid extraction (PLE), and pulse-electric field-assisted extraction (PEF) employed for the extraction of natural pigments from vegetal wastes and by-products [Citation86–96]. Greener extraction techniques involve solvents such as: ionic liquids (ILs), water, ethanol, esters of fatty acid or oils of fruits and vegetables (soybean, rapeseed oil, cocoa oils etc.), glycerol etc. which are all gaining importance for the extraction methods for natural pigments. Many researchers are employing green solvents and other organic methods for the extraction of natural pigments [Citation97]. Non-polar solvents used for the green extractions of lipophilic pigments having GRAS status incuding cyclopentyl methyl ether (CPME), 2‐methyl tetrahydrofuran (MeTHF), dimethyl carbonate (DMC), ethyl lactate, ethyl acetate, α-pinene, D-limonene and plant based essential oils [Citation98]. One more opportunity can be the use of a multi-stage extraction techniques which can be timely assisted by different physico-chemical approaches which may selectively target the desired pigments.
Table 2. Various green extraction techniques to extract pigments from vegetal waste.
In some of the innovative extraction techniques/strategies viz., UAE, MAE, SFE, PLE, PEF, and ILs which are proposed as substitutes to the conventional methods is depicted. These techniques are in demand due to their several advantages like the requirement for minimal solvents, are fast, convenient, can increase the extraction yield, protect pigments from degradation, enhance the quality of natural colorants, and are eco-friendly than conventional extraction techniques [Citation99].
Figure 2. Different extraction methods recommended/commonly employed for extraction of natural pigments from vegetal wastes.

The toxicity of solvents used during extraction has encouraged the advancement of greener techniques and materials to extract bioactive compounds from vegetal wastes and by-products. Moreover, termed as cold extraction methodologies, these methods maintain the natural composition and stability of extracted compounds while requiring lower energy, less number of process steps, a reduction in setup size, better mass and heat transfer and higher yield [Citation100–101].
Yamashita et al. [Citation10] developed an easy and cost-effective method by using less toxic extractants for the extraction of anthocyanins from blackberry by-products. Frozen blackberry pulp by-products were extracted with water (1:3 ratio, respectively) for anthocyanins, and concentrated in a rotatory evaporator at 60 °C. The aqueous and concentrated extracts had a higher anthocyanin content (718 and 389 mg cyanindin3-glucoside/100g of d.w.). Derrien et al. [Citation49], utilized green extraction technique assisted with supercritical CO2 (SC-CO2) extraction using 93% ethanol for the extraction of chlorophyll and lutein from spinach by-products/wastes and confirmed higher recovery of phytopigments (70% for lutein and 96% for chlorophylls) when compared with conventional extraction using acetone.
Parra-Campos & Ordóñez-Santos [Citation12], extracted anthocyanins from coffee exocarp and observed the highest content of anthocyanins (0.145 mg cyd 3-glucoside/g) obtained with 60% ethanol used as an extracting medium and with a 25% solid solvent ratio. Monrad et al. [Citation11] contemplated the extraction of anthocyanins from red grape pomace by utilizing expeller techniques. These authors have recorded an increase in the yield of anthocyanins (68 and 41% for crude and dried sample, respectively). In a study by Drosou et al. [Citation24] three different extraction methods (Soxhlet, MAE and UAE) along with solvent extraction (ethanol, water and their mixture-1:1), were employed to extract anthocyanins from the pomace of ‘Agiorgitico’ red grapes. Results of this study indicated highest procyanidins (43469 ppm) and anthocyanin contents (34188 ppm) in UAE- assisted techniques with solvents in combination (water: ethanol) compared to all treatments. Vulić et al. [Citation31] extracted betalains from the peel and pomace of beetroots by using a mixture of water and ethanol (1:1) acidified with acetic acid (0.5%) and assisted with ultrasonic extraction (50–60 Hz, 22 °C, 125 W, 30 min). They reported three major betalains from beetroot peel waste: betanin (3.8 to 7.5 mg/g), isobetanin (1.2 to 3.1 mg/g) and vulgaxanthin (1.4 to 4.3 mg/g). Beetroot pomace extract showed significant amounts of betanin (37.22 mg/100g) (all on dry weight basis). Recently, Goula et al. [Citation39] have developed a new process for the extraction of carotenoids from pomegranate peel waste by utilizing UAE assisted extraction with vegetable oils (sunflower and soybean oils) as a green solvent. They reported pomegranate peels to be an enriched source of carotenoids (0.6134 and 0.6715 mg carotenoids/100 g of dry peels with sunflower oil and soy oil, respectively). Working with tomato wastes, Strati et al. [Citation102] utilized pressurized liquid extraction (PLE) assisted with ethanol at a pressure about 700 MPa/10 min, and reported a higher content of total carotenoids (9.30 mg/kg d.w.) and lycopene (7.04 mg/kg d.w.) as compared to conventional extraction (total carotenoids- 3.63 mg/kg dw, lycopene- 2.47 mg/kg dw). Corrales et al. [Citation17] compared a pulsed electric field (PEF) assisted extraction with two other extraction techniques (ultrasonics and high hydrostatic pressure, HHP) for anthocyanins extraction from grape by-products. They observed an improvement in extraction efficiency (10% and 17%) in PEF technique compared to HHP and conventional solvent extraction. Results showed increased antioxidant activity; about four-fold in PEF, three-fold in HHP and two-fold in with ultrasonic treated samples when compared to conventional solvent extraction techniques (70 °C for 1 h). In another study, Kehili et al. [Citation45] explored SC-CO2 techniques assisted with solvent extraction techniques and using three solvents (hexane, ethanol and ethyl acetate), for the extraction of lycopene and β-carotene from tomato peel. Extraction with SC-CO2 yielded lycopene about 728.98 mg/kg dry basis under suitable processing conditions (pressure- 400 bar, CO2− 4 g/min at 80 °C). In contrast, extraction with solvents (hexane, ethanol and ethyl acetate) were recorded to have a lower lycopene content (608, 284 and 320 mg/kg dry basis, respectively). Enzyme-assisted extraction (cellulase and pectinase), assisted with ethyl lactate, resulted in an increase in the yield of carotenoids (six-fold) and lycopene (ten-fold) was reported for tomato waste when compared with non-enzyme treated samples [Citation102]. This study suggested that the use of commercially cheaper food-grade enzymes have huge potential to be explored on a pilot scale for the extraction phytopigments from agri-food wastes and by-products.
Recently, ionic liquids (ILs) have been used on various raw materials to obtain bioactive compounds, carotenoids being one such example [Citation103]. Martins and Rosso [Citation86], reported the use of IL [BMIM][PF6] (1-butyl-3-methylimidazolium hexafluorophosphate) and [BMIM][Cl] (1-butyl-3-methylimidazolium chloride) to extract lycopene from tomato waste and reported higher amounts (5.56 μg/g) of lycopene as compared to acetone (3.65 μg/g). Murador et al. [Citation75], demonstrated a novel method to extract carotenoid from orange peel, using four different ILs ([BMIM][PF6], [BMIM][Cl], 1-hexyl-3-methylimidazolium chloride ([HMIM][Cl]) and 1-n-butyl-3-methylimidazolium tetrafluoroborate ([BMIM][BF4])), assisted by ultrasound. [BMIM][Cl] was determined to be the most effective at extracting the carotenoid (32.08 μg/g), while acetone could only extract 7.88 μg/g of dry matter.
Some of the available innovative techniques along with organic solvents again cause an ill-impact on health even though in minimal quantity. Studies are ongoing with continuous efforts made to search new economical methods suitable for the extraction of all the pigments with improved quality following extraction. More research activities need to be undertaken aimed toward avoiding the use of synthetic colorants in foods to reduce the impact of synthetic colorants on the human body.
Considerable research works is lacking in the area of green technologies assisted with innovative technologies for the extraction of natural pigments on an industrial scale. There is a need to analyze the extraction costs for different phytopigments extracted from various green extraction methods and to identify the most feasible technique which can be best suitable for all the applications on an industrial scale.
Encapsulation of natural pigments
Stability is one of the vital aspects to be considered for the utilization of natural pigments as colorants and antioxidants in food formulations. The strength and stability of natural pigments are affected by several factors during processing and storage. Natural pigments extracted from plant resources or agri-food wastes are highly unstable and are susceptible to degradation by external (processing conditions such as temperature and pH) as well as internal factors (e.g. concentration of pigment). The naturally occurring pigments are inclined to degrade easily in aqueous and lipophilic solutions [Citation104]. The stability of these pigments can be increased by various concentrations in the system; low pH and temperature; presence of stabilizers (e.g. chelating agents, antioxidants); absence of light and the presence of acylation or glycosylation in the structure. In this way, micro or nanoencapsulation represents a promising concept and a best-suited technique for the entrapment of natural pigments in a coating enclosure to enhance their shelf-life [Citation105]. This is a technique which entraps the natural pigments using biopolymers to protect them from various processing and environmental hazards such as: moisture, oxygen, temperature, light, etc. This further improves their stability and ensures easier handling by changing them from liquid to powder forms [Citation106]. The potential of encapsulation techniques aimed to preserve the natural pigments extracted from vegetal wastes is are summarized in [Citation107–115]. Several studies have reported on the use of micro/nanoencapsulation techniques such as spray drying [Citation108–114], freeze-drying [Citation6,Citation10,Citation14,Citation19,Citation21,Citation23,Citation108,Citation109,Citation112,Citation113], electrospinning-nanofibers [Citation34] or complex coacervation- microsphere [Citation52] for the encapsulation of natural pigments. Spray drying is the best technique for the encapsulation of natural colorants because of it is a minimal exertion, rapid, and a reliable modern technique. During spray drying, the free-flowing ability, quality, powder efficiency, and bio-activity of natural pigments after drying treatment depends on the processing conditions such as temperature, atomization speed, feed rate, and carrier to feed ratio [Citation116]. Microencapsulation represents a promising application to the modern-day food industry by encapsulating unstable natural pigments which can help in enhancing the stability while maintaining their color during processing conditions. Microencapsulated powders obtained from natural pigments extracted from wastes or by-products can be utilized in the formulation of several types of functional foods and beverages such as: confectionery, chocolates, jellies, sauces, ice-creams, candies, juices and instant drink powders to color the food commodities and enrich the health-promoting potential.
Table 3. Various techniques recommended to encapsulate natural pigments from vegetal wastes.
Bioactivities and health benefits
Food industries are using pigments as color intensifiers, additives as well as antioxidants. There is a need to explore various vegetal waste resources to obtain food-grade pigments with bioactive potentials. This is mainly due to consumers’ awareness on health and numerous benefits delivered by these compounds (see , ). These pigments possess various biological roles in the protection of human health, like anti-inflammatory (utilizing cytokines signaling) [Citation117], antioxidant (utilizing free radical scavenging pathway) [Citation118], anti-cancer properties, antimicrobial, cardioprotective and antithrombotic (utilizing mitogen-activated protein kinase pathway), improving ocular and neural health and the prevention of non-communicable diseases (utilizing cyclo-oxygenase pathway) [Citation25,Citation119].
Antioxidant activity
The majority of the wastes and by-products have been reported to be a rich source of bioactive antioxidant compounds. These antioxidant rich compounds have been mainly isolated from berries (e.g. ribes, chokeberries, blueberries, blackberries and raspberries). Nutritional properties of these waste materials have turned them into promising sources of functional compounds. Usually, the waste discarded from fruits and vegetables contains high amounts of antioxidant compounds [Citation120]. Anthocyanins, flavonoids, vitamin E, C, phenolic compounds, fibers, carotenoids, and other antioxidants are the key bioactive compounds present in these waste materials, thus making them valuable sources of these compounds. These compounds can thus be extracted to be reused as functional food ingredients in other food products and supplements to provide much required antioxidants and to provide health benefits.
Seed pomace waste of both raspberry and blackberry can be a good source antioxidants. Besides containing omega‐3 (α‐linolenic) and omega‐6 (linoleic) (ratio 1: 2–4), these oils can be a potential source of bioactive compounds such as: phenols, tocopherols, carotenoids and sterols, which are recognized antioxidant agents. The presence of these antioxidants in the oil extracted from raspberry and blackberry seed pomace have elevated the usage of these seemingly waste products [Citation121]. Many health-promoting compounds can also be extracted from leaves as well. Cranberry leaves and pomace have been shown to contain more polyphenols resulting in the elevated antioxidant properties when compared with fruits, thus making them an alternative source to extract these beneficial compounds [Citation122]. Orange peel has a lower antioxidant potential than flesh wastes. Though both extracts have been shown to be effective in protecting against hydrogen peroxide free radicals, induced DNA damage on human leukocytes [Citation123]. Further, in a recent study on pomegranate extract, the presence of ellagic acid is reported to show antioxidant properties [Citation124]. It has also been reported that the presence of natural antioxidant additives in the wastes of tomato and potato renders them to be an excellent protective agent aimed to preserve vegetable oils (by reducing the oxidation of oils) [Citation125].
Anti-cancer activity
Natural plant based pigments have been well established to be a potential anti-cancer agent. Núñez Selles et al. [Citation126] have explored the application of mangiferin – a naturally occurring bioactive xanthonoid extracted from the mango tree for cancer treatment. Besides being a good antioxidant and anti-inflammatory agent, Mangiferin, when used either individually or in conjunction with other anti-cancer chemicals, is potentially beneficial in treating lung, brain, cervix, prostate and breast cancers, and leukemia. On the other note, berry extracts have been proven to exhibit anti-tumour properties due to the presence of polyphenols, ellagitannins and anthocyanins [Citation127]. Black raspberry seed wastes can cause apoptosis in colon cancer cells (HT-29) resulting in suppressed cellular spread [Citation128]. In freeze dried apple wastes, the inhibitory effects of non-extractable antioxidants was more pronounced than the extractable antioxidants on human cancer cells (viz. HepG2, HeLa, and HT-29) [Citation129]. It has been proved that ellagitannins extracted from pomegranate are useful in prostate LNCaP and breast MCF-7 cancer cell growth [Citation130]. Production waste of tomato juice exhibited anti-cancer properties via superoxide and hydroxyl anion radicals, which inhibited tumorous cell growth of MCF7, HeLa, and MRC-5 [Citation131]. Betalains extracted from beetroot pomace have been shown to be responsible for their potent antioxidative and anticancer properties, which exhibited significant proliferative effects in human cell lines- MCF7 and MRC-5 [Citation132].
Anti-inflammatory
Various phytochemicals including those of natural pigments from fruits and vegetables have gained the attention as therapeutic agents, which has been proved to reduce inflammation. Besides, they are recommended to be used as analgesics and anti-inflammatory agents in food and pharmaceutical preparations. Pectinolytic preparations were used to extract polyphenolic compounds and anthocyanins to study the potential anti-inflammatory activities of enzymatically treated raspberry pomace [Citation133]. These researchers found that anthocyanins thus obtained were able to inhibit lipoxygenase and cyclooxygenase 2 activities, resulting in the anti-inflammatory properties. Multiple studies have conclusively found that citrus pectin can regulate inflammatory response by affecting immune cells. In-vitro and in-vivo studies have found citrus pectin to induce endotoxin, causing alleviation of inflammatory sensations [Citation134]. Another phytochemical, limonin (a triterpenoid) has been extracted from citrus waste [Citation134]. Limonin can act as an anti-inflammatory, anti-bacterial, antioxidant and anti-cancer agent [Citation135]. Huynh et al. [Citation136] found cauliflower by-products, when subjected to solid state fermentation (using filamentous fungi) to produce significant amounts of phenolic compounds which increased the extractability of kaempferol glucosidase Kaempferol is a natural flavonoid that exhibits anti-carcinogenic and anti-inflammatory effects [Citation137]. A key usage of peel extracts is its role as an inhibitor toward nitric oxide (an inflammation mediator) and cytokines TNF-α, which could be explained by a decrease in free radicals during inflammation [Citation138].
Anti-microbial activity
Antimicrobial activity of fruit extracts (mainly of peel) is likely to be caused by the presence of colored pigments (anthocyanins, carotenoids, etc) which exhibits multiple antimicrobial mechanisms and synergies inside a microbial cell. Avocado peel extracts exhibited elevated antimicrobial potential than nisin, a naturally occurring dipeptide with antimicrobial properties. Additionally, avocado peel extracts also exhibited antimicrobial properties against Listeria innocua (L. innocua), Escherichia coli (E. coli), Leuconostoc mesenteroides, Weissella viridescens and Lactobacillus sakei. When it is combined with 39% nisin peel extracts it displayed the highest antimicrobial impact on L. innocua [Citation139]. In general, the antibacterial impact of peel extract was not only significant against Gram-positive bacteria, but also on E. coli [Citation140]. Kosińska et al. [Citation141] described that the polyphenol and tannin content of avocado seed extract had an antimicrobial effect on Staphylococcus epidermidis, Listeria monocytogenes and Mycobacterium avium, thus concluding that urinary tract infections could be prevented by the tannins present in avocado seed extracts [Citation141]. Kapadia et al. [Citation142] brought forward the antimicrobial effects of banana peels extracts on Aggregatibacter actinomycetemcomitans and Porphyromonas gingivalis. By-products from grape processing have been used to extract products that can serve as antimicrobial agents and natural antioxidants in fruit juices and meat products [Citation143]. For instance, grape seed oil has been proven to have antimicrobial properties, as demonstrated by Garavaglia et al. [Citation144], along with other health benefits. Beetroot pomace has been used in many functional supplements and foods due to its antimicrobial impact [Citation67]. By-products of cauliflower were evaluated for their antimicrobial impact on L. monocytogenes. The results of this study demonstrated its antimicrobial effects rendering it to be an important source to create biobased preservatives for ready-to-eat refrigerated foods [Citation145].
Anti-obesity activity
The natural phytopigments (mainly anthocyanins and carotenoids) are envisaged to play a significant role in lessening obesity contributing toward a decrease in adipose tissue (fat deposition). Various components and extracts from mango wastes have exhibited anti-oxidative and anti-inflammatory properties and at the same time have shown promising results at reducing obesity and diabetes in humans and animals. They have also proven to be good at: neuroprotection, managing intestinal health, prevention of skin cancers and cardiovascular diseases (constructive impact on the microbiota of gut) [Citation146]. Alam et al. [Citation147] demonstrated the anti-obesity effects of hydroxycinnamic acid derived from the Mavolanate-Shikimate biosynthesis pathways in plants. Betanine extracted from beetroot waste can serve as an excellent alternative route to prevent cancer and other disorders such as: Alzheimer’s disease, diabetes, and obesity [Citation148]. Gao et al. [Citation149] observed the impact of betanine intake on a large general population, and found direct correlation between betaine consumption and better body composition. Devalaraja et al. [Citation150] reported persimmon (Diospyros kaki L.) fruit peel extract to contain bioactive proanthocyanidins which exhibited anti-diabetic and anti-obesity effects. Apple pomace was also found to be rich in Phlorizin and Phloretein which suppressed obesity [Citation151].
Neuroprotective activity
The extracts rich in natural pigments have been proved to exhibit higher neuroprotective activity than polyphenols. The bioactivity of banana peel extract has been found to deliver strong neuroprotective effects, among other advantages, such as: antioxidant, antibacterial, antifungal and others [Citation152]. D-Psicose is another product extracted from fruit and vegetable wastes that have been found to exhibit multiple physiological advantages such as lower glycemic levels, suppress blood glucose, neuroprotective impact and an anti-dyslipidemic [Citation153]. Angeloni et al. [Citation154] studied the bioactivity of phenols derived from olive oil, finding its advantageous effects in recovery from: ischemic brain injury, Alzheimer’s, spinal cord injury and Parkinson’s diseases.
Based on the above reported bioactivities, it is hereby opined that pigments obtained from vegetal wastes and by-products can exhibit rich bioactivities. Future research is warranted to explore the biological potential of these pigments to be utilized in food, pharmaceutical and cosmetic industrial applications.
Future prospects and conclusion
Vegetal wastes and/or by-products obtained from the food bioprocessing industry remains as a major left-over leading to environmental pollution. However, these wastes and by-products can be a valuable and promising source of natural pigments. New advances, innovations and challenges in the extraction and application of these natural pigments to harvest their potential in functional bioactive are the core focus of this review. This review offers certain safe, potential, biodegradable, pigment alternatives to the currently used artificial coloration paradigm, which are usually extracted from renewable waste resources and by-products from fruits and vegetable industry. Recent investigations on the extraction of natural pigments embrace the quest for more innovative and financially feasible resources, suitable extraction strategies, developments in bioprocessing technologies, related innovations in pigment stabilization, suitability of a method for a wide range of pigments, to obtain a better quality of colors, probability of developing pigment-enriched functional foods and bioactives. To meet the current demands, there is an urgent need for food industry to take steps to minimize the vegetal waste to exploit the natural pigments in a sustainable manner that are natural, cost-effective, biodegradable and those which facilitates the minimal production of harmful intermediates when they enter the ecosystem and/or the food chain.
There is still ample scope for research activities that can be undertaken to obtain a suitable, reliable alternative which is considered to be safe, highly efficient, non-solvent based, environmentally friendly model techniques for pigment extraction which can contribute for higher extraction yields. The extraction of natural pigments from vegetal wastes followed by the incorporation of food products not only improves the color of the food commodities but also enriches the antioxidant capacity of food, aiding the health benefits by chelating free radicals from the body when introduced in diets, improving an esthetic appeal to consumers, raising the monetary aspects for manufactures and the ultimate benefits to the ecological atmosphere by reducing the waste in the fruitful arena. Also, there is minimal information available on the utilization of pigment-encapsulated functional foods. Encapsulation of the natural pigments can enhance the stability of these compounds within the processing environment of a model food system. Efforts need to be made to develop greener technologies to extract natural pigments from vegetal wastes, micro/nanoencapsulation techniques to enrich the bioavailability and stability that can simultaneously provide health benefits. Thus, the selection of greener technologies, suitable encapsulating material, nature of the encapsulant, encapsulation methods, nature of the core (natural pigment) material, processing conditions suitable for natural pigments still remains a challengeable constraint.
Finally, it is concluded that exploitation of natural and health benefiting pigments from food wastes and by-products not only helps to minimize the environmental stress and supports the circular economy concepts, but can also helps the economic gains to the dependent industry.
Disclosure statement
No potential conflict of interest was reported by the author(s).
Additional information
Funding
References
- Burrows JDA. Palette of our palates: a brief history of food coloring and its regulation. Compr Rev Food Sci Food Saf. 2009;8(4):394–408.
- Wolfe KL, Liu RH. Apple peels as a value-added food ingredient. J Agric Food Chem. 2003;51(6):1676–1683.
- González-Montelongo R, Gloria LM, González M. Antioxidant activity in banana peel extracts: Testing extraction conditions and related bioactive compounds. Food Chem. 2010;119(3):1030–1039.
- Mathew NS, Negi PS. Traditional uses, phytochemistry and pharmacology of wild banana (Musa acuminata Colla): a review. J Ethnopharmacol. 2017;196:124–140.
- Boulekbache-Makhlouf L, Medouni L, Medouni-Adrar S, et al. Effect of solvents extraction on phenolic content and antioxidant activity of the byproduct of eggplant. Ind Crops Prod. 2013;49:668–674.
- APdF M, Rezende CA, Rodrigues RA, et al. Encapsulation of anthocyanin-rich extract from blackberry residues by spray-drying, freeze-drying and supercritical antisolvent. Powder Technol. 2018;340:553–562.
- Espada-Bellido E, Ferreiro-González M, Carrera C, et al. Extraction of Antioxidants from Blackberry (Rubus ulmifolius L.): comparison between Ultrasound- and Microwave-Assisted Extraction Techniques. Agronomy. 2019;9(11):745.
- Gordillo B, Sigurdson GT, Lao F, et al. Assessment of the color modulation and stability of naturally copigmented anthocyanin-grape colorants with different levels of purification. Food Res Int. 2018;106:791–799.
- Farooque S, Rose PM, Benohoud M, et al. Enhancing the potential exploitation of food waste: extraction, purification, and characterization of renewable specialty chemicals from blackcurrants (Ribes nigrum L.). J Agric Food Chem. 2018;66(46):12265–12273.
- Yamashita C, Chung MM, dos Santos S, et al. Microencapsulation of an anthocyanin-rich blackberry (Rubus spp.) by-product extract by freeze-drying. LWT. 2017;84:256–262.
- Monrad JK, Suárez M, Motilva MJ, et al. Extraction of anthocyanins and flavan-3-ols from red grape pomace continuously by coupling hot water extraction with a modified expeller. Food Res Int. 2014;65:77–87.
- Parra-Campos A, Ordóñez-Santos LE. Natural pigment extraction optimization from coffee exocarp and its use as a natural dye in French meringue. Food Chem. 2019;285(1):59–66. 2019
- Saraband K, Jafari SM, Mahoonak AS, et al. Application of gum Arabic and maltodextrin for encapsulation of eggplant peel extract as a natural antioxidant and color source. Int J Biol Macromol. 2019;140:59–68.
- Šaponjac VT, Ćetković G, Čanadanović-Brunet J, et al. Sour cherry pomace extract encapsulated in whey and soy proteins: Incorporation in cookies. Food Chem. 2016;207:27–33.
- Bleve M, Ciurlia L, Erroi E, et al. An innovative method for the purification of anthocyanins from grape skin extracts by using liquid and sub-critical carbon dioxide. Sep Purif Technol. 2008;64(2):192–197.
- Ghafoor K, Park J, Choi YH. Optimization of supercritical fluid extraction of bioactive compounds from grape (Vitis labrusca B.) peel by using response surface methodology. Innov Food Sci Emerg Technol. 2010;11(3):485–490.
- Corrales M, Toepfl S, Butz P, et al. Extraction of anthocyanins from grape by-products assisted by ultrasonics, high hydrostatic pressure or pulsed electric fields: A comparison. Innov Food Sci Emerg Technol. 2008;9(1):85–91.
- Barros HDFQ, Baseggio AM, Angolini CFF, et al. Influence of different types of acids and pH in the recovery of bioactive compounds in Jabuticaba peel (Plinia cauliflora). Food Res Int. 2019;124:16–26. 2019
- Souza ACP, Gurak PD, Marczak LDF. Maltodextrin, pectin and soy protein isolate as carrier agents in the encapsulation of anthocyanins-rich extract from jaboticaba pomace. Food Biopdts P. 2017;102:186–194.
- Santos DT, Albarelli JQ, Beppu MM, et al. Stabilization of anthocyanin extract from jabuticaba skins by encapsulation using supercritical CO2 as solvent. Food Res Int. 2013;50(2):617–624.
- Rezende RRS, Nogueira JP, Narain N. Microencapsulation of extracts of bioactive compounds obtained from acerola (Malpighia emarginata DC) pulp and residue by spray and freeze drying: Chemical, morphological and chemometric characterization. Food Chem. 2018;254:281–291.
- Albishi T, John JA, Al-Khalifa AS, et al. Phenolic content and antioxidant activities of selected potato varieties and their processing by-products. J Func Foods. 2013;5(2):590–600.
- Milea AȘ, Vasile AM, Cîrciumaru A, et al. Valorizations of sweet cherries skins phytochemicals by extraction, microencapsulation and development of value-added food products. Foods. 2019;8(6):188.
- Drosou C, Kyriakopoulou K, Bimpilas A, et al. A comparative study on different extraction techniques to recover red grape pomace polyphenols from vinification byproducts. Ind Crops Prod. 2015;75:141–149.
- Wu L, Hsu H-W, Chen Y-C, et al. Antioxidant and antiproliferative activities of red pitaya. Food Chem. 2006;95(2):319–327.
- Rodriguez EB, Vidallon MLP, Mendoza DJR, et al. Health-promoting bioactivities of betalains from red dragon fruit (Hylocereus polyrhizus (Weber) Britton and Rose) peels as affected by carbohydrate encapsulation. J Sci Food Agric. 2016;96(14):4679–4689.
- Osorio-Esquivel O, Álvarez VB, Dorantes-Álvarez L, et al. Phenolics, betacyanins and antioxidant activity in Opuntia joconostle fruits. Food Res Int. 2011;44(7):2160–2168.
- Castro-Enríquez DD, Montaño-Leyva B, Del Toro-Sánchez CL, et al. Effect of Ultrafiltration of pitaya extract (Stenocereus thurberi) on its phytochemical content, antioxidant capacity, and UPLC-DAD-MS profile. Molecules. 2020;25(2):281.
- Cejudo-Bastante MJ, Hurtado N, Mosquera N, et al. Potential use of new Colombian sources of betalains. Color stability of ulluco (Ullucus tuberosus) extracts under different pH and thermal conditions. Food Res Int. 2014;64:465–471.
- Varelis P, Melton L, Shahidi F. Encyclopedia of Food chemistry. Amsterdam, Netherlands: Elsevier; 2019.
- Vulić JJ, Ćebović TN, Čanadanović-Brunet JM, et al. In vivo and in vitro antioxidant effects of beetroot pomace extracts. J Funct Foods. 2014;6:168–175.
- Mello FR, de Bernardo C, Dias CO, et al. Antioxidant properties, quantification and stability of betalains from pitaya (Hylocereus undatus) peel. Cienc Rural. 2014;45(2):323–328.
- Cunha LCM, Monteiro MLG, Costa-Lima BRC, et al. Effect of microencapsulated extract of pitaya (Hylocereus costaricensis) peel on color, texture and oxidative stability of refrigerated ground pork patties submitted to high pressure processing. Innov Food Sci Emerg Technol. 2018;49:136–145.
- Horuz TI, Belibağlı KB. Nanoencapsulation by electrospinning to improve stability and water solubility of carotenoids extracted from tomato peels. Food Chem. 2018;268:86–93.
- Strati IF, Oreopoulou V. Process optimisation for recovery of carotenoids from tomato waste. Food Chem. 2011;129(3):747–752.
- Rizk EM, El-Kady AT, El-Bialy AR. Characterization of carotenoids (lyco-red) extracted from tomato peels and its uses as natural colorants and antioxidants of ice cream. Ann Agric Sci. 2014;59(1):53–61.
- Chuyen HV, Roach PD, Golding JB, et al. Optimisation of extraction conditions for recovering carotenoids and antioxidant capacity from Gac peel using response surface methodology. Int J Food Sci Technol. 2017;52(4):972–980.
- De Abreu FP, Dornier M, Dionisio AP, et al. Cashew apple (Anacardium occidentale L.) extract from by-product of juice processing: A focus on carotenoids. Food Chem. 2013;138(1):25–31.
- Goula AM, Ververi M, Adamopoulou A, et al. Green ultrasound-assisted extraction of carotenoids from pomegranate wastes using vegetable oils. Ultrason Sonochem. 2017;34:821–830.
- Ghosh S, Chatterjee JK, Chakraborty B, et al. Estimation of beta carotene from fruit peel wastes by high performance thin layer chromatography. J Pharmacog Phytochem. 2019;8(1):2598–2600.
- Ghosh S, Chatterjee JK, Chakraborty B, et al. Total phenolic, flavonoid, β- carotene and in-vitro anti-oxidant activity of vegetable wastes collected from hotels and food processing centre. FSRJ. 2018;9(1):169–174.
- Chantaro P, Devahastin S, Chiewchan N. Production of antioxidant high dietary fiber powder from carrot peels. LWT. 2008;41(10):1987–1994.
- Zeyada NN, Zeitoum M, Barbary O. Utilization of some vegetables and fruit waste as natural antioxidants. Alex J Food Sci Technol. 2008;5:1–11.
- Palozza P, Colangelo M, Simone R, et al. Lycopene induces cell growth inhibition by altering mevalonate pathway and Ras signaling in cancer cell lines. Carcinogenesis. 2010;31(10):1813–1821.
- Kehili M, Kammlott M, Choura S, et al. Supercritical CO2 extraction and antioxidant activity of lycopene and β-carotene-enriched oleoresin from tomato (Lycopersicum esculentum L.) peels by-product of a Tunisian industry. Food Biopdts P. 2017;102:340–349.
- Wang X, Wang C, Zha X, et al. Supercritical carbon dioxide extraction of β-carotene and α-tocopherol from pumpkin: a Box–Behnken design for extraction variables. Anal Methods. 2017;9(2):294–303.
- de Andrade Lima M, Kestekoglou I, Charalampopoulos D, et al. Supercritical Fluid Extraction of Carotenoids from Vegetable Waste Matrices. Molecules. 2019;24(3):466.
- Derrien M, Aghabararnejad M, Gosselin A, et al. Optimization of supercritical carbon dioxide extraction of lutein and chlorophyll from spinach by-products using response surface methodology. LWT. 2018;93:79–87.
- Derrien M, Badr A, Gosselin A, et al. Optimization of a green process for the extraction of lutein and chlorophyll from spinach by-products using response surface methodology (RSM). LWT. 2017;79:170–177.
- Kang J-H, Kim S, Moon B. Optimization by response surface methodology of lutein recovery from paprika leaves using accelerated solvent extraction. Food Chem. 2016;205:140–145.
- Baysal T, Ersus S, Starmans DAJ. Supercritical CO(2) extraction of beta-carotene and lycopene from tomato paste waste. J Agric Food Chem. 2000;48(11):5507–5511.
- Martínez-Hernández GB, Castillejo N, Artés-Hernández F. Effect of fresh–cut apples fortification with lycopene microspheres, revalorized from tomato by-products, during shelf life. Postharvest Biol Technol. 2019;156:110925.
- Lavecchia R, Zuorro A. Improved lycopene extraction from tomato peels using cell-wall degrading enzymes. Eur Food Res Technol. 2008;228(1):153–158.
- Sigurdson GT, Tang P, Giusti MM. Natural Colorants: Food Colorants from Natural Sources. Annu Rev Food Sci Technol. 2017;8(1):261–280.
- Joshi VK, Kumar A, Kumar V. Antimicrobial, antioxidant and phyto-chemicals from fruit and vegetable wastes: A review. Int J Food Fermt Technol. 2012;2(2):123–136.
- Ayala-Zavala JF, Rosas-Domínguez C, Vega-Vega V, et al. Antioxidant enrichment and antimicrobial protection of fresh-cut fruits using their own byproducts: looking for integral exploitation. J Food Sci. 2010;75(8):R175–R181.
- Sagar NA, Pareek S, Sharma S, et al. Fruit and vegetable waste: bioactive compounds, their extraction, and possible utilization. Compr Rev Food Sci Food Saf. 2018;17(3):512–531.
- Fernández-López JA, Fernández-Lledó V, Angosto JM. New insights into red plant pigments: more than just natural colorants. RSC Adv. 2020;10(41):24669–24682.
- Faustino M, Veiga M, Sousa P, et al. Agro-food byproducts as a new source of natural food additives. Molecules. 2019;24(6):1056.
- Parashar S, Sharma H, Garg M. Antimicrobial and antioxidant activities of fruits and vegetable peels: A review. J of Pharmacognosy Phytochem. 2014;3(1):160–164.
- Rodriguez-Amaya DB. Natural food pigments and colorants. In: Mérillon JM, Ramawat K, editors. Bioactive molecules in food. Reference Series in Phytochemistry. Switzerland: Springer International Publishing; 2019, p. 1–35.
- Andersen ØM, Jordheim M. Basic anthocyanin chemistry and dietary sources. In: Wallace TC, Giusti MM, editors. Anthocyanins in health and disease. Boca Raton, FL: CRC Press; 2014, p. 13–90.
- Brazinha C, Cadima M, Crespo JG. Optimization of extraction of bioactive compounds from different types of grape pomace produced at wineries and distilleries. J Food Sci. 2014;79(6):E1142–E1149.
- Stintzing FC, Carle R. Betalains – emerging prospects for food scientists. Trends Food Sci Technol. 2007;18(10):514–525.
- Ochoa-Velasco CE, Guerrero-Beltrán JÁ. The effects of modified atmospheres on prickly pear (Opuntia albicarpa) stored at different temperatures. Postharvest Biol Technol. 2016;111:314–321.
- Herbach KM, Stintzing FC, Carle R. Betalain stability and degradation? structural and chromatic aspects. J Food Science. 2006;71(4):R41–R50.
- Kushwaha R, Kumar V, Vyas G, et al. Optimization of different variable for eco-friendly extraction of betalains and phytochemicals from beetroot pomace. Waste Biomass Valor. 2018;9(9):1485–1494.
- Thirugnanasambandham K, Sivakumar V. Microwave assisted extraction process of betalain from dragon fruit and its antioxidant activities. J Saudi Soc Agri Sc. 2017;16(1):41–48.
- Melgar B, Dias MI, Barros L, et al. Ultrasound and microwave assisted extraction of Opuntia fruit peels biocompounds: optimization and comparison using RSM-CCD. Molecules. 2019;24(19):3618.
- Rivera SM, Canela-Garayoa R. Analytical tools for the analysis of carotenoids in diverse materials. J Chromatogr A. 2012;1224:1–10.
- Bian Q, Gao S, Zhou J, et al. Lutein and zeaxanthin supplementation reduces photooxidative damage and modulates the expression of inflammation-related genes in retinal pigment epithelial cells. Free Radic Biol Med. 2012;53(6):1298–1307.
- British Pharmacopoeia Commission, Great Britain. Department of Health. British pharmacopoeia 2013 London: The Stationery Office; 2013. Available from: https://www.worldcat.org/title/british-pharmacopoeia-2013/oclc/992371858?referer=di&ht=edition
- Rodriguez-Amaya DB. Food carotenoids: chemistry, biology and technology. Oxford: IFT Press-Wiley; 2015.
- Tiwari S, Upadhyay N, Singh AK, et al. Organic solvent-free extraction of carotenoids from carrot bio-waste and its physico-chemical properties. J Food Sci Technol. 2019;56(10):4678–4687.
- Murador DC, Braga ARC, Martins PLG, et al. Ionic liquid associated with ultrasonic-assisted extraction: A new approach to obtain carotenoids from orange peel. Food Res Int. 2019;126:108653.
- Cubas C, Gloria-Lobo M, González M. Optimization of the extraction of chlorophylls in green beans (Phaseolus vulgaris L.) by N,N-dimethylformamide using response surface methodology. J Food Comp Anlys. 2008;21(2):125–133.
- Humphrey AM. Chlorophyll as a color and functional ingredient. J Food Sci. 2006;69(5):C422–C425.
- Steet JA, Tong CH. Degradation kinetics of green color and chlorophylls in peas by colorimetry and HPLC. J Food Science. 1996;61(5):924–928.
- Ferruzzi MG, Blakeslee J. Digestion, absorption, and cancer preventative activity of dietary chlorophyll derivatives. Nutr Res. 2007;27(1):1–12.
- Viera I, Pérez-Gálvez A, Roca M. Green natural colorants. Molecules. 2019;24(1):154.
- Wang W, Bostic TR, Gu L. Antioxidant capacities, procyanidins and pigments in avocados of different strains and cultivars. Food Chem. 2010;122(4):1193–1198.
- Ngamwonglumlert L, Devahastin S, Chiewchan N. Natural colorants: pigment stability and extraction yield enhancement via utilization of appropriate pretreatment and extraction methods. Crit Rev Food Sci Nutr. 2017;57(15):3243–3259.
- Aberoumand A. A review article on edible pigments properties and sources as natural biocolorants in foodstuff and food industry. World J Dairy Food Sci. 2011;6(1):71–78.
- Poojary MM, Passamonti P. Optimization of extraction of high purity all-trans-lycopene from tomato pulp waste . Food Chem. 2015;188:84–91.
- García-Cruz L, Dueñas M, Santos-Buelgas C, et al. Betalains and phenolic compounds profiling and antioxidant capacity of pitaya (Stenocereus spp.) fruit from two species (S. pruinosus and S. stellatus). Food Chem. 2017;234:111–118.
- Martins PL, de Rosso VV. Carotenoids achieving from tomatoes discarded using ionic liquids as extracting for application in food industry, presented at XIV Safety, health and environment world Congress conference, Cubatäo, Brazil, July 20–23, 2014.
- Da Silva RPFF, Rocha-Santos TAP, Duarte AC. Supercritical fluid extraction of bioactive compounds. TrAC. 2016;76:40–51.
- Machado APDF, Pasquel-Reátegui JL, Barbero GF, et al. Pressurized liquid extraction of bioactive compounds from blackberry (Rubus fruticosus L.) residues: a comparison with conventional methods. Food Res Int. 2015;77:675–683.
- Eh AL, Teoh SG. Novel modified ultrasonication technique for the extraction of lycopene from tomatoes. Ultrason Sonochem. 2012;19(1):151–159.
- Rajha HN, Boussetta N, Louka N, et al. Effect of alternative physical pretreatments (pulsed electric field, high voltage electrical discharges and ultrasound) on the dead-end ultrafiltration of vine-shoot extracts. Sep Purif Technol. 2015;146:243–251.
- Pap N, Beszédes S, Pongrácz E, et al. Microwave-assisted extraction of anthocyanins from black currant marc. Food Bioprocess Technol. 2013;6(10):2666–2674.
- Li Y, Fabiano-Tixier AS, Vian MA, et al. Solvent-free microwave extraction of bioactive compounds provides a tool for green analytical chemistry. TrAC. 2013;47:1–11.
- Brianceau S, Turk M, Vitrac X, et al. High voltage electric discharges assisted extraction of phenolic compounds from grape stems: effect of processing parameters on flavan-3-ols, flavonols and stilbenes recovery. Innov Food Sci Emerg Technol. 2016;35:67–74.
- Barba FJ, Zhu Z, Koubaa M, et al. Green alternative methods for the extraction of antioxidant bioactive compounds from winery wastes and by-products: a review. Trends Food Sci Technol. 2016;49:96–109.
- López N, Puértolas E, Condón S, et al. Enhancement of the extraction of betanine from red beetroot by pulsed electric fields. J Food Eng. 2009;90(1):60–66.
- Briones-Labarca V, Plaza-Morales M, Giovagnoli-Vicuña C, et al. High hydrostatic pressure and ultrasound extractions of antioxidant compounds, sulforaphane and fatty acids from Chilean papaya (Vasconcellea pubescens) seeds: effects of extraction conditions and methods. LWT. 2015;60(1):525–534.
- Mohapatra D, Kate A. Extraction techniques of color pigments from fruits and vegetables. In: Khan KA, Goyal MR, Kalne AA, editors. Processing of fruits and vegetables from farm to fork. United Kingdom: Apple Academic Press, CRC press, Taylor and Francis; 2019, p. 175–200.
- Chemat F, Abert VM, Ravi HK, et al. Review of alternative solvents for green extraction of food and natural products: panorama, principles, applications and prospects. Molecules. 2019;24(16):3007.
- Azmir J, Zaidul ISM, Rahman MM, et al. Techniques for extraction of bioactive compounds from plant materials: a review. J Food Eng. 2013;117(4):426–436.
- Tiwari BK. Ultrasound: a clean, green extraction technology. TrAC. 2015;71:100–109.
- Jacotet-Navarro M, Rombaut N, Deslis S, et al. Towards a “dry” bio-refinery without solvents or added water using microwaves and ultrasound for total valorization of fruit and vegetable by-products. Green Chem. 2016;18(10):3106–3115.
- Strati IF, Gogou E, Oreopoulou V. Enzyme and high pressure assisted extraction of carotenoids from tomato waste. Food Biopdts Proc. 2015;94:668–674.
- Usmani Z, Sharma M, Gupta P, et al. Ionic liquid based pretreatment of lignocellulosic biomass for enhanced bioconversion. Bioresour Technol. 2020;304:123003.
- Cai Y, Sun M, Schliemann W, et al. Chemical stability and colorant properties of betaxanthin pigments from Celosia argentea. J Agric Food Chem. 2001;49(9):4429–4435.
- Ersus S, Yurdagel U. Microencapsulation of anthocyanin pigments of black carrot (Daucus carota L.) by spray drier. J Food Eng. 2007;80(3):805–812.
- Robert P, Gorena T, Romero N, et al. Encapsulation of polyphenols and anthocyanins from pomegranate (Punica granatum) by spray drying. Int J Food Sci Technol. 2010;45(7):1386–1394.
- Flores FP, Singh RK, Kong F. Physical and storage properties of spray-dried blueberry pomace extract with whey protein isolate as wall material. J Food Eng. 2014;137:1–6.
- Correia R, Grace MH, Esposito D, et al. Wild blueberry polyphenol-protein food ingredients produced by three drying methods: comparative physico-chemical properties, phytochemical content, and stability during storage. Food Chem. 2017;235:76–85. 2017
- Kuck LS, Noreña CPZ. Microencapsulation of grape (Vitis labrusca var. Bordo) skin phenolic extract using gum Arabic, polydextrose, and partially hydrolyzed guar gum as encapsulating agents. Food Chem. 2016;194:569–576.
- Baldin JC, Michelin EC, Polizer YJ, et al. Microencapsulated jabuticaba (Myrciaria cauliflora) extract added to fresh sausage as natural dye with antioxidant and antimicrobial activity. Meat Sci. 2016;118:15–21.
- Chuyen HV, Roach PD, Golding JB, et al. Encapsulation of carotenoid-rich oil from Gac peel: Optimisation of the encapsulating process using a spray drier and the storage stability of encapsulated powder. Powder Technol. 2019;344:373–379.
- Vulić J, Šeregelj V, Kalušević A, et al. Bioavailability and bioactivity of encapsulated phenolics and carotenoids isolated from red pepper waste. Molecules. 2019;24(15):2837.
- Šeregelj V, Šaponjac V, Lević S, et al. Application of encapsulated natural bioactive compounds from red pepper waste in yogurt. J Microencapsul. 2019;36(8):704–714.
- Ordoñez-Santos LE, Martínez-Girón J, Villamizar-Vargas RH. Encapsulation of β-carotene extracted from peach palm residues: a stability study using two spray-dried processes. DYNA. 2018;85(206):128–134.
- Amr AS, Hussein DS. Tomato pomace pigment: extraction and use as food colorant. JJAS. 2013;9(1):72–85.
- Tonon RV, Brabet C, Hubinger MD. Influence of process conditions on the physicochemical properties of açai (Euterpe oleraceae Mart.) powder produced by spray drying. J Food Eng. 2008;88(3):411–418.
- Vidal PJ, López-Nicolás JM, Gandía-Herrero F, et al. Inactivation of lipoxygenase and cyclooxygenase by natural betalains and semi-synthetic analogues. Food Chem. 2014;154:246–254.
- Gengatharan A, Dykes GA, Choo WS. Betalains: natural plant pigments with potential application in functional foods. LWT. 2015;64(2):645–649.
- Balasundram N, Sundram K, Samman S. Phenolic compounds in plants and agri-industrial by-products: antioxidant activity, occurrence, and potential uses. Food Chem. 2006;99(1):191–203.
- Gomez-Estaca J, Calvo MM, Sanchez-Faure A, et al. Development, properties, and stability of antioxidant shrimp muscle protein films incorporating carotenoid-containing extracts from food by-products. LWT. 2015;64(1):189–196.
- Radočaj O, Vujasinović V, Dimić E, et al. Blackberry (Rubus fruticosus L.) and raspberry (Rubus idaeus L.) seed oils extracted from dried press pomace after longterm frozen storage of berries can be used as functional food ingredients. Eur J Lipid Sci Technol. 2014;116(8):1015–1024.
- Oszmiański J, Wojdyło A, Lachowicz S, et al. Comparison of bioactive potential of cranberry fruit and fruit-based products versus leaves. J Func Foods. 2016;22:232–242.
- Jae-Hee P, Minhee L. Eunju P. Antioxidant activity of orange flesh and peel extracted with various solvents. Prev Nutr Food Sci. 2014;19:291–298.
- Masci A, Coccia A, Lendaro E, et al. Evaluation of different extraction methods from pomegranate whole fruit or peels and the antioxidant and antiproliferative activity of the polyphenolic fraction. Food Chem. 2016;202:59–69.
- del Carmen Robles-Ramírez M, Monterrubio-López R, Mora-Escobedo R, et al. Evaluation of extracts from potato and tomato wastes as natural antioxidant additives. Arch Latinoam Nutr. 2016;66:66–73.
- Núñez Selles AJ, Daglia M, Rastrelli L. The potential role of mangiferin in cancer treatment through its immunomodulatory, anti-angiogenic, apoptopic, and gene regulatory effects. Biofactors. 2016;42(5):475–491.
- Rugină D, Diaconeasa Z, Coman C, et al. Chokeberry anthocyanin extract as pancreatic beta-cell protectors in two models of induced oxidative stress. Oxid Med Cell Longev. 2015;2015:429075–429010.
- Cho H, Jung H, Lee H, et al. Chemopreventive activity of ellagitannins and their derivatives from black raspberry seeds on HT-29 colon cancer cells. Food Funct. 2015;6(5):1675–1683.
- Tow WW, Premier R, Jing H, et al. Antioxidant and antiproliferation effects of extractable and nonextractable polyphenols isolated from apple waste using different extraction methods. J Food Sci. 2011;76(7):T163–T172.
- Orgil O, Schwartz E, Baruch L, et al. The antioxidative and anti-proliferative potential of non-edible organs of the pomegranate fruit and tree. LWT. 2014;58(2):571–577.
- Ćetković G, Savatović S, Čanadanović-Brunet J, et al. Valorisation of phenolic composition, antioxidant and cell growth activities of tomato waste. Food Chem. 2012;133(3):938–945.
- Vulić J, Čanadanović-Brunet J, Ćetković G, et al. Antioxidant and cell growth activities of beet root pomace extracts. J Func Foods. 2012;4(3):670–678.
- Szymanowska U, Baraniak B. Antioxidant and potentially anti-inflammatory activity of anthocyanin fractions from pomace obtained from enzymatically treated raspberries. Antioxidants. 2019;8(8):299.
- Ishisono K, Yabe T, Kitaguchi K. Citrus pectin attenuates endotoxin shock via suppression of Toll-like receptor signaling in Peyer’s patch myeloid cells. J Nutr Biochem. 2017;50:38–45.
- Liu S, Zhang S, Lv X, et al. Limonin ameliorates ulcerative colitis by regulating STAT3/miR-214 signaling pathway. Int Immunopharmacol. 2019;75:105768.
- Huynh NT, Smagghe G, Gonzales GB, et al. Extraction and bioconversion of kaempferol metabolites from cauliflower outer leaves through fungal fermentation. Biochem Eng J. 2016;116:27–33.
- Kashyap D, Sharma A, Tuli HS, et al. Kaempferol - A dietary anticancer molecule with multiple mechanisms of action: Recent trends and advancements. J Funct Foods. 2017;30:203–219.
- Tremocoldi MA, Rosalen PL, Franchin M, et al. Exploration of avocado by-products as natural sources of bioactive compounds. PLoS One. 2018;13(2):e0192577.
- Calderon-Oliver M, Escalona-Buendıa HB, Medina-Campos ON, et al. Optimization of the antioxidant and antimicrobial response of the combined effect of nisin and avocado byproducts. LWT. 2016;65:46–52.
- Rodrıguez-Carpena JG, Morcuende D, Andrade MJ, et al. Avocado (Persea americana Mill.) phenolics, in vitro antioxidant and antimicrobial activities, and inhibition of lipid and protein oxidation in porcine patties. J Agric Food Chem. 2011;59(10):5625–5635.
- Kosińska A, Karamać M, Estrella I, et al. Phenolic compound profiles and antioxidant capacity of persea americana mill. peels and seeds of two varieties. J Agric Food Chem. 2012;60(18):4613–4619.
- Kapadia SP, Pudakalkatti PS, Shivanaikar S. Detection of antimicrobial activity of banana peel (Musa paradisiaca L.) on Porphyromonas gingivalis and Aggregatibacter actinomycetemcomitans: an in vitro study. Contemp Clin Dent. 2015;6(4):496–499.
- Mattos GN, Tonon RV, Furtado AA, et al. Grape by-product extracts against microbial proliferation and lipid oxidation: a review. J Sci Food Agric. 2017;97(4):1055–1064.
- Garavaglia J, Markoski MM, Oliveira A, et al. Grape seed oil compounds: biological and chemical actions for health. Nutr Metab Insights. 2016;9:59–64.
- Sanz-Puig M, Pina-Perez MC, Rodrigo D, et al. Antimicrobial activity of cauliflower (Brassica oleracea var. Botrytis) by-product against Listeria monocytogenes. Food Cntrl. 2015;50:435–440.
- Burton-Freeman BM, Sandhu AK, Edirisinghe I. Mangos and their bioactive components: adding variety to the fruit plate for health. Food Funct. 2017;8(9):3010–3032.
- Alam MA, Subhan N, Hossain H, et al. Hydroxycinnamic acid derivatives: a potential class of natural compounds for the management of lipid metabolism and obesity. Nutr Metab. 2016;13(1):27.
- Zhao G, He F, Wu C, et al. Betaine in inflammation: mechanistic aspects and applications. Front Immunol. 2018;9:1070.
- Gao X, Wang Y, Randell E, et al. Higher dietary choline and betaine intakes are associated with better body composition in the adult population of Newfoundland. PLoS One. 2016;11(5):e0155403.
- Devalaraja S, Jain S, Yadav H. Exotic fruits as therapeutic complements for diabetes, obesity and metabolic syndrome. Food Res Int. 2011;44(7):1856–1865.
- Shin SK, Cho SJ, Jung UJ, et al. Phlorizin supplementation attenuates obesity, inflammation, and hyperglycemia in diet-induced obese mice fed a high-fat diet. Nutrients. 2016;8(2):92.
- Vu HT, Scarlett CJ, Vuong QV. Phenolic compounds within banana peel and their potential uses: a review. J Funct. Foods. 2018;40:238–248.
- Patel SN, Sharma M, Lata K, et al. Improved operational stability of D-psicose 3-epimerase by a novel protein engineering strategy, and D-psicose production from fruit and vegetable residues. Bioresour Technol. 2016;216:121–127.
- Angeloni C, Malaguti M, Barbalace M, et al. Bioactivity of olive oil phenols in neuroprotection. IJMS. 2017;18(11):2230.