ABSTRACT
Inter-individual variability exists in recovery from jetlag following travel across time zones. Part of this variation may be due to genetic differences at the variable number tandem repeat (VNTR) polymorphism of the PERIOD3 (PER3) gene as this polymorphism has been associated with chronotype and sleep, as well as sensitivity to blue light on melatonin suppression. To test this hypothesis we conducted a laboratory-based study to compare re-entrainment in males genotyped as PER34/4 (n = 8) and PER35/5 (n = 8) following simulated eastward travel across six time zones. The recovery strategy included morning blue-enriched light exposure and appropriately-timed meals during the first 24 h after simulated travel. Dim light melatonin onset (DLMO), sleep characteristics, perceived sleepiness levels (Stanford Sleepiness Scale), and resting metabolic parameters were measured during constant routine periods before and after simulated travel. While DLMO time was similar between the two groups prior to simulated eastward travel (p = .223), it was earlier in the PER35/5 group (17h23 (17h15; 17h37)) than the PER34/4 group (18h05 (17h53; 18h12)) afterwards (p = .046). During resynchronisation, perceived sleepiness and metabolic parameters were similar to pre-travel in both groups but sleep was more disturbed in the PER35/5 group (total sleep time: p = .008, sleep efficiency: p = .008, wake after sleep onset: p = .023). The PER3 VNTR genotype may influence the efficacy of re-entrainment following trans-meridian travel when blue-enriched light exposure is incorporated into the recovery strategy on the first day following travel.
Introduction
The primary consequence of trans-meridian travel is a cluster of symptoms known as jetlag, caused by the desynchronization of the internal body clock from the environment (Bin et al. Citation2019; Weingarten and Collop Citation2013). Symptoms of jetlag, which are a transient inconvenience and usually evident following air travel across at least two time zones, may include daytime fatigue, decreased ability to perform mental and physical tasks, reduced alertness, headaches and disturbed sleep (Bin et al. Citation2020, Citation2019; Kölling et al. Citation2017). Jetlag symptom severity and an individual’s recovery time may vary based on the number of time zones crossed, the direction of travel, timing of flights, nutrition, age, sex, chronotype and genetics (Moline et al. Citation1992; Cingi et al. Citation2018; Janse Van Rensburg et al. Citation2020a; Zacharko et al. Citation2020). While the general rule is that for every time zone crossed, 24 hours of recovery time is required (Eastman et al. Citation2005; Waterhouse et al. Citation2007; Winget et al. Citation1984) it appears that this recovery time varies significantly between individuals (Kölling et al. Citation2017; Lee and Galvez Citation2012; Waterhouse et al. Citation2007).
Jetlag recovery strategies aim to realign the innate circadian system with the new environmental time as fast as possible. Current strategies include timed light exposure, manipulation of sleep schedules, meal times and the use of sedative or stimulant medication (Arendt Citation2009; Bin et al. Citation2020; Cingi et al. Citation2018; Janse Van Rensburg et al. Citation2020b). Humans are sensitive to sunlight, particularly the shorter wavelength (460 nm) or blue light. Carefully timed exposure to blue light has been shown to hasten phase shifting of the circadian clock (Chellappa et al. Citation2012; Eastman et al. Citation2005; Revell et al. Citation2005) purportedly by suppressing or stimulating the secretion of melatonin by the pineal gland, which in turn synchronises the principal circadian clock in the suprachiasmatic nuclei to the environment and the peripheral circadian clocks to each other. There is some evidence, however, to suggest that bright artificial light is as effective as blue light at phase shifting (Smith and Eastman Citation2009; Smith et al. Citation2009) and there is significant inter-individual variation in the phase shifting response to light (Gooley et al. Citation2011). Regardless, timed light exposure has been shown to be one of the most effective strategies to resynchronise internal circadian rhythms to the environment following trans-meridian travel (Eastman et al. Citation2016; Roach and Sargent Citation2019).
Individuals differ in the way they recover or re-entrain their circadian clocks following trans-meridian travel and variation in the human circadian clock genes may in part explain this inter-individual variation, given their associations with factors such as sleep timing (Chellappa et al. Citation2014; Dijk and Archer Citation2010; Hida et al. Citation2014; Viola et al. Citation2012) and melatonin secretion (Archer et al. Citation2008; Chellappa et al. Citation2012; Viola et al. Citation2012). For example, the PERIOD3 (PER3) variable number tandem repeat (VNTR) polymorphism, which is associated with chronotype (Archer et al. Citation2003; Kunorozva et al. Citation2012; Pereira et al. Citation2005), has also been associated with sensitivity to blue light. Specifically, individuals genotyped as PER35/5 for the VNTR polymorphism appear to be more sensitive to blue light from an entrainment perspective compared to PER34/4 individuals (Chellappa et al. Citation2012). In addition, the PER3 VNTR polymorphism has been associated with melatonin suppression and the subjective and objective alerting action of light (Chellappa et al. Citation2012; Lok et al. Citation2018), as well as with the cognitive brain responses to light (Vandewalle et al. Citation2011). The PER34/4 genotype is associated with eveningness (a behavioural preference for evening activity) and the PER35/5 genotype associated with morningness (Archer et al. Citation2010; Hida et al. Citation2014; Lázár et al. Citation2012; Nadkarni et al. Citation2005).
We speculate that the PER3 VNTR genotype may account for some of the inter-individual variability observed in the phase-shifting responses and subsequent re-entrainment following travel across time zones when morning blue light exposure is incorporated into the recovery strategy. Specifically, we hypothesise that individuals genotyped as PER35/5 will experience greater phase shifts during the first 48 hours of resynchronisation compared to those genotyped as PER34/4. Thus the aim of this study was to compare the re-entrainment response of individuals genotyped as PER34/4 and PER35/5 following simulated eastward travel across six time zones. The re-entrainment strategy included three hours of morning blue-enriched light exposure and appropriately-timed meals in the new time zone, and resynchronisation was based on changes in dim light melatonin onset (DLMO) circadian phase.
Methods
Participants
Sixteen males genotyped as either PER35/5 (n = 8) or PER34/4 (n = 8) were recruited for this study. Criteria for inclusion required that participants (i) be between 18–40 years of age, (ii) be free of sleep complaints, (iii) be in good self-reported physical and mental health, (iv) habitually sleep between 6 and 10 hours per night, (v) have low habitual levels of physical activity, and (vi) have either the PER34/4 or PER35/5 VNTR genotype. Exclusion criteria were (i) a diagnosed condition requiring chronic medication, (ii) any sleep disorder, (iii) use of any chronic medication known to affect sleep, melatonin and/or circadian rhythms in the previous six months, (iv) night or rotating shift work in the previous three months, (v) trans-meridian travel crossing ≥3 time zones within the previous two months, (vi) smoking, (vii) normal caffeine consumption greater than 300 mg∙d−1, (viii) red-green colour blind condition (may affect efficacy of blue light therapy (Smith et al. Citation2009)), (ix) engaged in any sport/physical activity which requires more than two training sessions per week, or (x) a body mass index ≥30 kg∙m−2. Relatively inactive participants were used as the protocol required long periods of sedentary behaviour.
Participants were recruited using flyers, posters and radio advertisements. Prior to formal entry into the study, participants were screened for eligibility (see below). The first eight eligible individuals in each of the two genotype categories (PER35/5 and PER34/4) were invited to take part in the study. The study was approved by the University of Cape Town’s Faculty of Health Sciences Human Research Ethics Committee (HREC/REF: 360/2014). All participants gave written informed consent. The study was performed in accordance with the principles of the Declaration of Helsinki (World Medical Association, Citation2013), the International Conference on Harmonisation and South African Good Clinical Practice guidelines.
Study overview
Following screening, a run-in period took place two weeks prior to the simulated jetlag protocol, to (i) ensure participants were not sleep deprived, (ii) verify supplement and medication use pre-trial, and (iii) confirm reported physical activity levels. The simulated jetlag protocol was an 82 h trial (), which took place in a sound- and light-proof space comprising two bedrooms, a lounge area, bathroom and kitchen. Participants reported to the laboratory at 11h30 on Day 1 (D1) of the study and were not allowed contact (physical or virtual) with the outside world until the end of the trial. All times given in this section refer to local time (as opposed to phase shifted environmental time). The simulated jetlag protocol began at 12h00 on D1 with the first 28 h constant routine (CR) period, designed to obtain baseline measures of participants’ innate circadian systems. Following this, participants were given an 8 h sleep opportunity. On waking they began a 24 h day in the new time zone (6 h ahead of home time zone). This day included 3 h of morning blue light therapy and concluded with an 8 h sleep opportunity. This was followed by a second 28 h CR period, designed to compare the rate at which the two groups had resynchronised to the new time zone. The trial ended at 22h00 on D4. A maximum of three participants were tested at one time, and at least one investigator remained with them at all times. During periods of wakefulness, participants interacted with one another in the lounge area. Participants retired to individual or partitioned bedrooms for sleep opportunities.
Detailed study procedures
Screening
A total of 117 participants were screened for eligibility for this study. Participants completed a questionnaire detailing demographics, medical history, medication and supplement use, work, travel, sleep, exercise and dietary history. The questionnaire also contained the Horne-Östberg morningness-eveningness personality questionnaire to determine chronotype (Horne and Ostberg Citation1976), the Sleep Timing Questionnaire (Monk et al. Citation2003) and the Pittsburgh Sleep Quality Index (PSQI) questionnaire to assess sleep quality (Buysse et al. Citation1989). The questionnaire was designed to determine eligibility and describe study participants. Participants then performed the online Ishihara colour blind test (Birch Citation1997) as colour blindness may negatively influence the efficacy of blue light therapy (Lavric and Pompe Citation2014; Rüger et al. Citation2013). Based on their responses to 24 different plates, the test indicated whether participants had (i) normal colour vision, (ii) red-green colour blindness or (iii) total colour blindness. None of the participants tested positive for red-green colour blindness or total colour blindness. The investigator measured the height (m) and weight (kg) of each participant to determine body mass index (kg∙m−2).
A buccal cell sample was obtained from 100 participants and a simple salting out protocol was used to extract human genomic DNA (Wen et al. Citation2000) with minor modifications as previously described (Kunorozva et al. Citation2012). The amplification of exon 18 and the adjacent introns on the PER3 gene was performed with the use of primers described in Archer et al. (Citation2003) and Ebisawa et al. (Citation2001) followed by restriction with NcoI (Promega, Madison, WI, USA) and resolving of the resultant fragments as previously described (Kunorozva et al. Citation2012). In total there were 37 individuals genotyped as PER34/4, 12 genotyped as PER35/5 and 51 genotyped as PER34/5. Those genotyped as PER34/4 and PER35/5 were invited to continue with the screening process for this study. Once eight eligible people in each group were identified, screening was stopped. The 16 eligible participants underwent a resting metabolic rate (RMR) test following an overnight fast (described in more detail below). This served as a familiarisation test, as well as to calculate participants’ RMR so that the caloric content of their meals during the simulated jetlag protocol could be optimised to meet their requirements.
Run-in period
During the two-week run-in period participants were asked to adhere to a regular sleep schedule (i.e., no more than 1 h variation between earliest and latest wake-up and bedtimes) and to aim for a “time-in-bed” length 1h longer than their habitual weekday sleep length to minimise sleep deprivation. They were also asked to keep a sleep diary to log their bedtimes and wake-up times, medications and supplement use, consumption of alcohol- and caffeine- containing products, habitual meal timing, and any exercise sessions. Habitual sleep was measured continuously in the second week of the run-in period using a wrist-worn actigraphy monitor (Actiwatch-2; Philips Respironics, Bend, OR, USA). Habitual bedtime, wake-up time, time-in-bed (TIB), total sleep time (TST), sleep onset latency (SOL), sleep efficiency (SE) and wake after sleep onset (WASO) were recorded. Participants were not permitted to consume alcohol and caffeine or to use non-steroidal anti-inflammatories 24 h prior to beginning the simulated jetlag trial.
Constant routine protocol
The CR protocol is a standard method used to unmask the endogenous circadian components of the physiological and behavioural rhythms by removing any rhythmic changes due to an individual’s lifestyle or environment (Qian and Fajl Citation2016; Wright et al. Citation2015). This was achieved by removing environmental, activity, meal timing and social zeitgebers. Participants were kept under constant temperature (25°C), humidity (49%) and dim indoor light conditions (≤10 lux from incandescent lamps measured at eye level using a handheld digital light meter, Maplin LD 140 Light Meter, model 631, UK). During each 28 h CR protocol participants were restricted to a regimen of semi-recumbent posture, low activity and continuous wakefulness (enforced by the investigators) and were given hourly isocaloric nutritional supplements. They were allowed to engage in activities such as reading, listening to music (no radio), watching TV (not live, only pre-recorded), computer work (no internet), and playing board games. Both the TV and computer were physically fitted with screen filters to block blue-light wavelength (LEE filters 767 Oklahoma Yellow, Camquip Trading CC, Johannesburg, South Africa). The clocks within the computers were deactivated so that participants could not obtain time cues from the devices. Subjective sleepiness was measured every three hours using the Stanford Sleepiness Scale (Hoddes et al. Citation1972). Saliva samples were obtained hourly from 18h00 – 24h00 on D1 and 13h00 – 22h00 on D4 to measure melatonin concentrations. RMR tests were performed at 06h00 on D2 and D4 and at 24h00 on D3 ().
Simulated 6 h phase shift and new time zone day
Following the 8 h recovery sleep that followed CR1, participants were woken up at 24h00 (local time) but told that it was 06h00 in their new “time zone,” thus simulating eastward travel crossing 6 time zones. Not knowing what true local time was, participants were not told the degree to which time had been shifted, but they were provided with a clock showing the time in the new time zone. Room light levels were increased to 80 lux (measured at eye level) delivered from incandescent bulbs, and participants ate breakfast, lunch, dinner and snacks at times appropriate for the new time zone. In the hour after waking participants underwent a RMR test, followed by 3 h of blue light therapy. During this day, participants were free to work, read, play games and socialise with each other. The day ended with an 8 h sleep opportunity ().
Resting metabolic rate test
The RMR tests were conducted using the indirect calorimetry ventilated hood technique (Quark RMR, Cosmed, Rome, Italy). RMR reflects the body’s energy expenditure when completely at rest. It is related to lean body mass but can be affected by a number of external factors such as lack of sleep, feeding/fasting status, and consumption of stimulants. Factors derived from the RMR test include (i) total energy expenditure (TEE), i.e. total calories burned by an individual in one day adjusted to level of physical activity (i.e., sedentary, moderate, strenuous); and (ii) the respiratory exchange ratio (RER), which is the ratio between the metabolic production of carbon dioxide and the uptake of oxygen and allows for the estimation of reliance on fat or carbohydrate for energy. Participants were asked to recline quietly while oxygen uptake and carbon dioxide production were measured at 10 s intervals for 20 min, or until a steady state was achieved (<5% change in respiratory exchange ratio, RER). Data were averaged over 60s intervals and RMR (kcal·day−1) was taken as the mean value over the last 10 min. To ensure accuracy, the gas analyser was calibrated before each trial was undertaken with a 3 L syringe and standard gas mixtures of oxygen (26% O2 with the balance nitrogen) and carbon dioxide (4% CO2, 16% O2 and the balance nitrogen) (BOC Special Gas, Afrox, Cape Town, South Africa). While the RMR test conducted during the screening session was used to calculate the caloric content of participants’ meals during the simulated jetlag protocol, those conducted during the protocol were used to observe resynchronisation of the energetic system following the simulated phase advance ().
Meals
All meals were provided for participants in the laboratory for the duration of the 82 h trial. For the CR periods, participants were given hourly isocaloric meal supplements to remove meal timing and composition cues and to ensure correct 24 h energy intake for each individual. The supplements were prepared using Ensure® Powder (Abbott Nutrition, Johannesburg, South Africa) mixed with water, which is a complete meal replacement supplement designed to deliver approximately 55% carbohydrate, 30% fat and 15% protein per serving. The exact dosage for each participant was based on his RMR, body weight and a metabolic equivalent of approximately 1.5 kcal·kg−1·h−1 for the 28 h period. In the new time zone, participants were given standardised breakfast, lunch and dinner meals scheduled six-hourly from 24h00 D2–24h00 D3 to coincide with appropriate meal times for the new time zone (). During this period, participants also had ad-libitum access to water and non-caffeinated tea (without sugar).
Blue-enriched light therapy
One hour after waking up in the new time zone, participants underwent 3 h of blue-enriched light therapy to promote synchronisation to the new time zone (). This time was chosen as it corresponds to 07h00 – 10h00 in the new time zone and we wanted to mimic morning light exposure following trans-meridian travel. Cool white light-emitting diode (LED) panels (5500–6000 K, model UP-PL3030-18 W, China) were mounted on adjustable wooden stands, which delivered blue-enriched light (wavelength 450–495 nm) at a brightness of 100 lux measured at eye level. Participants sat around a table for this period with the lights placed 50 cm from their eyes. During this time, they were allowed to engage with one another, play board or card games, eat breakfast and read novels, but were not permitted to nap.
Sleep opportunities
Participants were given two 8 h sleep opportunities during the trial. Both the first (SO1) and second (SO2) sleep opportunities started at 16h00-24h00 on D2 and D3 local time, but 22h00-06h00 in their new time zone (). Wrist-worn actigraphy was used to measure TST, SOL, SE and WASO. Participants were not allowed to use any electronic devices or talk to each other during the two sleep opportunities. They were required to remain in bed until further instructions by the investigator.
Dim light melatonin onset analysis
During the two CR periods, whole saliva was collected hourly from each participant by passive drool to avoid possible interference with the assay from swabs (Kozaki et al. Citation2011), starting ±4 h prior to and continuing to at least 4 h after habitual sleep onset (). Samples were immediately placed in a covered ice box to minimise light exposure. All samples were centrifuged for 10 min at 3000 x g at 4°C, and the supernatants removed and frozen at −20°C within 4 h of collection until subsequent analysis. Melatonin concentration at each time point was measured using an enzyme immunoassay kit (Salimetrics™ Salivary Melatonin kit 1–3402, Salimetrics Europe Ltd, Newmarket, Suffolk, UK), designed and validated for the quantitative measurement of salivary melatonin in humans. Samples collected from one trial were assayed in the same batch to minimise inter-assay variability, a standard curve was run for each plate and the high and low salivary melatonin controls supplied with the kit were included in each assay. Information supplied with the kit indicated that the functional sensitivity of the assay was 2.25 pg⋅mL−1 with a coefficient of variance below 20%, with good intra- and inter-assay precision. DLMO was determined using an absolute threshold of 4 pg⋅mL−1 and linear interpolation of the time at which the level was reached.
Data and statistical analyses
The Shapiro-Wilk test was performed to determine whether data were normally distributed. Data are reported as median with interquartile ranges (IQR) or 95% confidence intervals. Phase shifting for melatonin was determined by calculating the DLMO time for each individual during CR1 and subtracting that from the same individual’s DLMO time during CR2. A positive DLMO time difference meant participants had an advanced melatonin phase after the 6h phase shift. Between groups comparisons were made using one-way ANOVA or Mann-Whitney U tests. Categorical data were compared using Fisher’s Exact test. Within group comparisons were made using Wilcoxon matched pairs test. Mixed effects linear regression models were used to assess changes in sleepiness levels and metabolic parameters over time between groups. Data were analysed using STATISTICA version 12 (Tulsa Oklahoma, USA) and Stata version 15.1 (StataCorp, College Station, Texas,). Statistical significance was accepted for p < .05.
Results
Participant characteristics prior to the trial
Descriptive characteristics of the participants as measured during screening and the run-in period are shown in . There were no differences in any variables between the two groups prior to commencement of the trial. The PER34/4 and PER35/5 groups’ actigraphy-derived total sleep times were 6.20 h (interquartile range (IQR) 1.19 h) and 7.13 h (IQR 2.18 h), respectively, the night prior to the start of the trial (p = .435). This value was similar to habitual TIB for the PER35/5 group (p = .135) but shorter than habitual TIB for the PER34/4 group (p = .028).
Table 1. Participant characteristics measured prior to the trial.
Changes in sleep characteristics in response to the simulated jetlag protocol
Data for SO1 and SO2 are presented in . During SO1, the PER35/5 group slept longer (p = .026) and had a better SE (p = .026) than the PER34/4 group. During SO2, the PER35/5 group took less time to fall asleep (p = .046) than the PER34/4 group. The sleep characteristics of the PER34/4 group did not differ between SO1 and SO2. In contrast, the PER35/5 group slept for less time (p = .008), had lower SE (p = .008) and longer WASO (p = .023) during SO2 compared to SO1.
Table 2. Sleep characteristics of the PER34/4 and PER35/5 groups during the first (SO1) and second (SO2) sleep opportunities.
Changes in sleepiness
Subjective sleepiness data for both groups measured every 3 h throughout the trial are presented in . For CR1, there were no interaction (p = .427) or group (p = .787) effects, but there was a time effect (p < .001). Post-hoc analysis indicates that for both groups, sleepiness measured between 24h00 and 15h00 (i.e. time points 5–10) differed significantly to that measured at 12h00 (i.e. time point 1) (p < .001). The PER35/5 group reached their highest sleepiness levels 6 h earlier than the PER34/4 group during CR1. During the day time in the new time zone, there were no interaction (p = .248), group (p = .473) or time (p = .459) effects. Similarly, there were no interaction (p = .818), group (p = .713) or time (p = .135) effects during CR2.
Figure 2. Changes in perceived sleepiness levels during constant routine (CR) 1, first day in new time zone (TZ) and CR2, as measured by the Stanford Sleepiness Scale in the PER34/4 and PER35/5 groups. Data are presented as median with interquartile ranges. Significance was determined using mixed effects linear regression models.
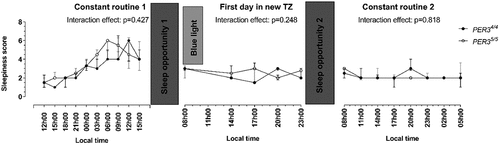
Changes in salivary melatonin concentration
Hourly salivary melatonin concentration and DLMO data for the two groups measured during CR1 and CR2 are presented in , and individual melatonin profiles are presented in Supplementary Fig. 1. There was a 1h50min phase advance in DLMO between CR1 (19h30 (19h17; 19h42)) and CR2 (17h40 (17h19; 18h10)), when data for both groups were pooled for analysis (, p < .001). DLMO was similar between the PER34/4 and PER35/5 groups (p = .223) during CR1 (i.e. prior to simulated travel). Both the PER34/4 () and PER35/5 () groups displayed significant phase advances in DLMO (p = .008 and p = .008, respectively). During CR2, however, DLMO occurred 42 min earlier in the PER35/5 (17h23 (17h15; 17h37)) compared to the PER34/4 (18h05 (17h53; 18h12)) group indicating a larger phase advance in the PER35/5 group (p = .046).
Figure 3. Changes in hourly salivary melatonin concentration and dim light melatonin onset (DLMO) during constant routines (CR) 1 and 2, respectively. A and B: All participants, C: PER34/4 group and D: PER35/5 group. Data are presented as median with interquartile ranges (A) or individual data points (B-D). The dotted lines indicates DLMO time for CR1 and CR2 at the DLMO threshold value of 4 pg⋅ml−1. Significance was determined using Wilcoxon matched pairs test.
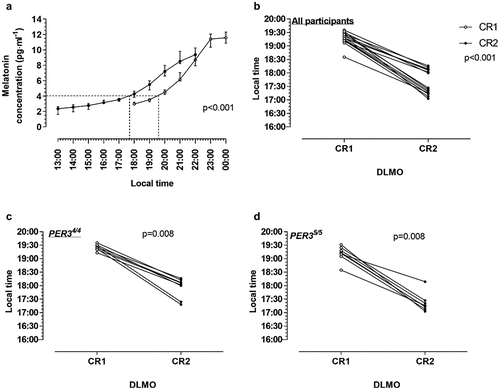
Changes in metabolic parameters
shows the changes in RMR (A) and RER (B) from pre-trial to 06h00 on day 1 in the new time zone (i.e. after waking from first recovery sleep after CR1) and to 06h00 on day 2 in the new time zone (i.e. after waking from sleep following the first day in the new time zone). For RMR there were no interaction (p = .938), group (p = .540) or time (p = .936) effects. For RER, there were no interaction (p = .385) or group (p = .694) effects, but there was a time effect (p < .001). Post-hoc analyses indicate that for the PER34/4 group, RER measured at 06h00 on day 1 in the new time zone was lower than that measured pre-trial (p = .022) and at 06h00 on day 2 in the new time zone (p < .001). For the PER35/5 group, RER measured at 06h00 on day 2 in the new time zone was higher than that measured pre-trial (p = .009) and at 06h00 on day 1 in the new time zone (p = .001).
Figure 4. Changes in resting metabolic rate (A) and respiratory exchange ratio (B) measured before the trial and at 08h00 on days 1 and 2 in the new time zone. TZ D1 and D2: time zones days 1 and 2 respectively. Data are presented as median with 95% confidence intervals. Significance was determined using mixed effects linear regression models.
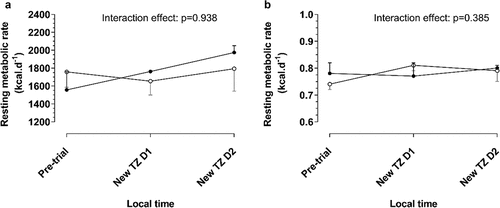
Discussion
The aim of this study was to compare the extent to which individuals genotyped as PER34/4 and PER35/5 re-entrain to a new time zone following simulated eastward travel across six time zones, when morning blue-enriched light exposure is incorporated into the resynchronisation strategy during the first day in the new time zone. The main finding was that while DLMO phase-advanced in both groups within 48 h following simulated eastward travel, and the magnitude of the shift was larger (42 min earlier) among the PER35/5 than the PER34/4 participants. Looking at the individual phase-advance responses, every single participant displayed earlier DLMO times during the second constant routine, but of the participants genotyped as PER34/4, only two phase-advanced to the same extent as that observed in the PER35/5 group. Of these two individuals, one habitually exercised in the morning and his HÖ-score was in the moderate morning-type range, while nothing stood out for the second individual. Collectively these data suggest a more rapid early re-entrainment response in young males genotyped as PER35/5 during resynchronisation to simulated jetlag compared to those with the PER34/4 genotype.
There was no difference between the genotype groups in their habitual bedtimes prior to the trial. Wake-up time, midpoint of sleep, and sleep onset, have been demonstrated to be correlated with DLMO (Burgess et al. Citation2003; Keijzer et al. Citation2011). We note that the difference between DLMO and bedtime for this cohort is at the higher end of the expected range of 2–4 h (Burgess et al. Citation2008; Flynn-Evans et al. Citation2017; Kennaway Citation2020; Micic et al. Citation2015; Sletten et al. Citation2010), and slightly earlier (12–13 h) relative to time after waking than has been previously measured (13–14 h) (Burgess et al. Citation2003). This cohort of young males was comprised mostly of students and it is thus very likely that although their wake-up times were imposed by study schedules, their habitual bedtimes were not biologically driven, but were indicative of a behavioural delay in sleep time driven by social schedules. This indicates that there was some level of circadian misalignment within the study cohort prior to the start of the experiment.
Chellappa et al. (Citation2012) previously reported that individuals with the PER35/5 genotype were more sensitive to the melatonin suppression and alerting effects of blue light. This may explain in part why the PER35/5 group in this study had an earlier DLMO in constant routine two compared to the PER34/4 group following morning blue-enriched light exposure. Blue light increased brain responses to a larger extent in individuals with PER35/5 genotype compared to PER34/4 only following sleep loss, in a study investigating the effects of light on cognitive brain function (Vandewalle et al. Citation2011). Alternatively, the differences in DLMO between the two PER3 VNTR groups under the same environmental conditions may have arisen due to differences in the phase angle of entrainment as a result of differences between the timing of the circadian clock and external environmental time cues (Archer et al. Citation2018; Dijk and Archer Citation2010; Lázár et al. Citation2012; Mongrain et al. Citation2006; Sletten et al. Citation2015; Viola et al. Citation2012; Wright et al. Citation2004). Indeed, the melatonin profile has been associated with the internal phase of the sleep-wake cycle (Arendt Citation2019; Drake et al. Citation2015; Rajaratnam et al. Citation2004; Wehr et al. Citation2001). This does not seem likely in the present study, however, since the two PER3 VNTR groups had similar DLMO times prior to the simulated eastward travel (17 min difference between groups) and their habitual sleep timing prior to the trial was not different, although we acknowledge the potential limitation of our small sample size. Rather we speculate that incorporation of morning blue light exposure as a part of the resynchronisation strategy may be more effective in promoting faster phase shifting in young males with the PER35/5 genotype. We propose that this phase shifting response relates to the entire circadian system rather than melatonin alone. We had collected saliva samples for cortisol assessment to complement the melatonin measures of circadian phase. We have not shown these data as our sampling strategy did not extend late enough to capture the peak for cortisol in the PER34/4 group in CR2, and as such we were unable to make any meaningful comparisons. The cortisol levels were significantly higher in the PER35/5 group compared to the PER34/4 group during CR1 and during CR2 which was also demonstrated by Wirth et al. (Citation2013).
While the only sleep-related difference between the two groups following the day of resynchronisation was that the PER35/5 group had a slightly shorter sleep onset latency, the changes in total sleep time, sleep efficiency and wake after sleep onset time measured in the PER35/5 group between the two sleep opportunities may reflect an earlier shift towards habitual sleep quality markers measured before the trial. This means that the PER34/4 individuals may experience impaired sleep duration and efficiency during the early part of their sleep opportunity due to attempting to sleep during their wake maintenance zone (Dijk and Czeisler Citation1994; Lavie Citation1986; Sletten et al. Citation2015), as a result of the misalignment between their sleep time and circadian clock. Measurement of sleep patterns over at least another five days, however, would provide better insight as to the extent which PER3 VNTR genotype might impact resynchronisation of sleep patterns. Furthermore, since the sleepiness scores of the two groups both during the first day of resynchronisation and during the second constant routine period were similar, it is possible that, as discussed below, the acute effects of sleep deprivation induced by the first constant routine (>28 h of continued wakefulness), may mask underlying circadian disruption-related effects on sleep characteristics or daytime sleepiness in the immediate period after simulated eastward travel.
From a metabolic perspective, the PER34/4 group had a lower RER (marker of greater reliance on fat as a fuel at rest) at 06h00 on day one in the new time zone compared to both pre-trial and on day two in the new time zone. In contrast, the PER35/5 group had a higher RER (i.e. greater reliance on carbohydrate as a fuel at rest) at 06h00 on day two in the new time zone compared to both pre-trial and at 06h00 on day one in the new time zone. This suggests that sleep deprivation, such as that induced by the first constant routine, together with desynchronisation induced by the simulated eastward travel may have affected lipid and carbohydrate metabolism differently in the PER34/4 and PER35/5 individuals. Specifically, sleep deprivation lowers serum leptin concentration (Spiegel et al. Citation2005), which may promote fatty acid oxidation in the mitochondria (Ruderman and Saha Citation2006). Other studies have reported a reduction in glucose uptake by peripheral tissues in favour of fat oxidation following sleep deprivation (Cedernaes et al. Citation2015; Knutson et al. Citation2007; Tasali et al. Citation2008). Both of these may explain the slight shift to elevated fat oxidation, especially in the PER34/4 group after the first recovery sleep. The subsequent shift to a greater reliance on carbohydrate for fuel at rest in both groups may reflect desynchronisation of the metabolic processes, which initially appears to have occurred to a similar extent in both groups. Future larger scale studies that investigate metabolic shifts following sleep deprivation and/or circadian desynchronisation may be useful not only for travellers in optimising their diets to minimise the effects of jetlag, but also for shift-workers. Unfortunately, we are unable to comment on any resynchronisation differences between the two groups beyond 24h post-simulated travel.
Another observation of this study was that the PER35/5 group reached their highest sleepiness levels earlier than the PER34/4 participants in the first constant routine period. This could be explained by the differential neurobehavioral sensitivity to sleep loss in the PER34/4 and PER35/5 genotypes as noted in previous sleep deprivation studies (Archer et al. Citation2010; Groeger et al. Citation2008; Lo et al. Citation2012; Maire et al. Citation2014a, Citation2014b; Taillard et al. Citation2003; Viola et al. Citation2007). Specifically, the PER35/5 genotype has been shown to have a faster homeostatic build-up of sleep pressure than the PER34/4 genotype (Cajochen et al. Citation1999; Dijk and Archer Citation2010; Goel et al. Citation2009; Groeger et al. Citation2008; Van Dongen et al. Citation2012; Viola et al. Citation2012), which may explain the earlier peak in sleepiness in this group. For example, Dijk and Archer (Citation2010), showed enhanced alpha activity (a marker of sleepiness) during rapid eye movement sleep and higher EEG theta activity during wakefulness in individuals with the PER35/5 genotype, indicative of higher homeostatic sleep pressure. This fits with our observation that the PER35/5 group had a longer total sleep time and higher efficiency in the first sleep opportunity following constant routine 1 compared to the PER34/4 group. Potentially, the PER35/5 group was more affected by the sleep deprivation during the first constant routine resulting in a higher homeostatic sleep drive than the PER34/4 group. This may still have been present ahead of the second sleep opportunity, in which the sleep onset latency was quicker in the PER35/5 than PER34/4 group, since a short time to fall asleep is a marker of sleep debt.
Most studies that have assessed the PER3 VNTR genotype distribution in various ethnic groups around the world have reported no significant differences in the distribution of this genotype (An et al. Citation2014; Bedini et al. Citation2016; Dagmura et al. Citation2021; Ozsoy et al. Citation2021; Voinescu and Coogan Citation2012) except in the Papua New Guinea population (Nadkarni et al. Citation2005). As such, males from all ethnicities were recruited for this study. Limitations for this study include a small sample size, and a lack of female participants. Ideally, a randomised crossover study in which participants repeated the trial with light of a longer wavelength (Blume et al. Citation2019; Wright et al. Citation2004) would have made a good control condition. The experiment only examined eastward travel with morning light exposure. A simulated westward travel with evening light exposure may have different findings (Phillips et al. Citation2019; Watson et al. Citation2018). These future studies would provide a full comparison of the effect of blue-enriched light in resynchronising the PER34/4 and PER35/5 genotypes to a new environment following simulated jetlag.
In conclusion, results from this study suggest that the two PER3 VNTR groups differed in the magnitude of the phase shift of DLMO when blue-enriched light therapy was used as part of the re-entrainment strategy after simulated eastward travel across 6 time zones. The earlier DLMO of the PER35/5 group compared to the PER34/4 group during the second constant routine suggests that they were in the process of re-entraining to the new time zone faster. One of the challenges facing individuals after eastward travel is being able to fall asleep early in the new time zone. Practically, an earlier DLMO in the PER35/5 group suggests that they may be able to sleep earlier since night-time sleepiness and sleep onset are related to DLMO timing.
Supplemental Material
Download PDF (223.3 KB)Acknowledgements
We are grateful to the participants for their time and commitment. The authors would like to thank former members of the Rhythms and Blooms Laboratory Dr Eric Banda, Dr Rob Henst, Dr Rageema Joseph, Chenjerai Muchapirei, Nyambura Shawa and the late Denford Banga, as well as Prof Vicki Lambert, Mrs Hendriena Victor and Dr Jacolene Kroff for their assistance with this study. We are grateful to Granny Goose Duvets, A1 Furnishers and Plumstead Electrical in Cape Town for their sponsorship of bedding, beds and lighting respectively.
Disclosure statement
No potential conflict of interest was reported by the author(s).
Supplementary material
Supplemental data for this article can be accessed online at https://doi.org/10.1080/07420528.2022.2139184
Additional information
Funding
References
- An H, Zhu Z, Zhou C, Geng P, Xu H, Wang H, Chen R, Qu X, Qian H, Gao Y, et al. 2014. Chronotype and a PERIOD3 variable number tandem repeat polymorphism in Han Chinese pilots. Int J Clin Exp Med. 7:3770–76.
- Archer SN, Carpen JD, Gibson M, Lim GH, Johnston JD, Skene DJ, Von Schantz M. 2010. Polymorphism in the PER3 promoter associates with diurnal preference and delayed sleep phase disorder. Sleep. 33:695–701. doi:10.1093/sleep/33.5.695
- Archer SN, Robilliard DL, Skene DJ, Smits M, Williams A, Arendt J, Von Schantz M. 2003. A length polymorphism in the circadian clock gene Per3 is linked to delayed sleep phase syndrome and extreme diurnal preference. Sleep. 26:413–15. doi:10.1093/sleep/26.4.413
- Archer SN, Schmidt C, Vandewalle G, Dijk DJ. 2018. Phenotyping of PER3 variants reveals widespread effects on circadian preference, sleep regulation, and health. Sleep Med Rev. 40:109–26. doi:10.1016/j.smrv.2017.10.008
- Archer SN, Viola AU, Kyriakopoulou V, Von Schantz M, Dijk DJ. 2008. Inter-individual differences in habitual sleep timing and entrained phase of endogenous circadian rhythms of BMAL1, PER2 and PER3 mRNA in human leukocytes. Sleep. 31:608–17. doi:10.1093/sleep/31.5.608
- Arendt J. 2009. Managing jet lag: Some of the problems and possible new solutions. Sleep Med Rev. 13:249–56. doi:10.1016/j.smrv.2008.07.011
- Arendt J. 2019. Melatonin: Countering Chaotic Time Cues. Front Endocrinol (Lausanne). 10:391. doi:10.3389/fendo.2019.00391
- Bedini G, Bersano A, Sebastiano DR, Sattin D, Ciaraffa F, Tosetti V, Brenna G, Franceschetti S, Ciusani E, Leonardi M, et al. 2016. Is period3 genotype associated with sleep and recovery in patients with disorders of consciousness? Neurorehabil Neural Repair. 30:461–69. doi:10.1177/1545968315604398
- Bin YS, Ledger S, Nour M, Postnova S, Stamatakis E, Cistulli PA, de Chazal P, Allman-Farinelli M, Caillaud C, Bauman A, et al. 2020. How do travelers manage jetlag and travel fatigue? A survey of passengers on long-haul flights. Chronobiol Int. 37:1621–28. doi:10.1080/07420528.2020.1787427
- Bin YS, Postnova S, Cistulli PA. 2019. What works for jetlag? A systematic review of non-pharmacological interventions. Sleep Med Rev. 43:47–59. doi:10.1016/j.smrv.2018.09.005
- Birch J. 1997. Efficiency of the Ishihara test for identifying red-green colour deficiency. Ophthalmic Physiol Opt. 17:403–08. doi:10.1016/S0275-5408(97)00022-7
- Blume C, Garbazza C, Spitschan M. 2019. Effects of light on human circadian rhythms, sleep and mood. Somnologie. 23:147–56. doi:10.1007/s11818-019-00215-x
- Burgess HJ, Fogg LF, Yamazaki S. 2008. Individual differences in the amount and timing of salivary melatonin secretion. PLoS One. 3:e3055. doi:10.1371/journal.pone.0003055
- Burgess HJ, Savic N, Sletten T, Roach G, Gilbert SS, Dawson D. 2003. The relationship between the dim light melatonin onset and sleep on a regular schedule in young healthy adults. Behavioral Sleep Medicine. 1:102–14. doi:10.1207/S15402010BSM0102_3
- Buysse DJ, Reynolds CF, Monk TH, Berman SR, Kupfer DJ. 1989. The Pittsburgh sleep quality index: A new instrument for psychiatric practice and research. Psychiatry Res. 28:193–213. doi:10.1016/0165-1781(89)90047-4
- Cajochen C, Khalsa SBS, Wyatt JK, Czeisler CA, Dijk DJ. 1999. EEG and ocular correlates of circadian melatonin phase and human performance decrements during sleep loss. Am J Physiol - Regul Integr Comp Physiol. 277:R640–9. doi:10.1152/ajpregu.1999.277.3.r640
- Cedernaes J, Schiöth HB, Benedict C. 2015. Determinants of shortened, disrupted, and mistimed sleep and associated metabolic health consequences in healthy humans. Diabetes. 64:1073–80. doi:10.2337/db14-1475
- Chellappa SL, Viola AU, Schmidt C, Bachmann V, Gabel V, Maire M, Reichert CF, Valomon A, Götz T, Landolt HP, et al. 2012. Human melatonin and alerting response to blue-enriched light depend on a polymorphism in the clock gene PER3. J Clin Endocrinol Metab. 97:E433–7. doi:10.1210/jc.2011-2391
- Chellappa SL, Viola AU, Schmidt C, Bachmann V, Gabel V, Maire M, Reichert CF, Valomon A, Landolt HP, Cajochen C. 2014. Light modulation of human sleep depends on a polymorphism in the clock gene Period3. Behav Brain Res. 271:23–29. doi:10.1016/j.bbr.2014.05.050
- Cingi C, Emre IE, Muluk NB. 2018. Jetlag related sleep problems and their management: A review. Travel Med Infect Dis. 24:59–64. doi:10.1016/j.tmaid.2018.05.008
- Dagmura H, Yiǧit S, Nursal AF, Duman E, Gumusay O. 2021. Possible association of per2/per3 variable number tandem repeat polymorphism variants with susceptibility and clinical characteristics in Pancreatic Cancer. Genet Test Mol Biomarkers. 25:124–30. doi:10.1089/gtmb.2020.0179
- Dijk DJ, Archer SN. 2010. PERIOD3, circadian phenotypes, and sleep homeostasis. Sleep Med Rev. 14:151–60. doi:10.1016/j.smrv.2009.07.002
- Dijk DJ, Czeisler CA. 1994. Paradoxical timing of the circadian rhythm of sleep propensity serves to consolidate sleep and wakefulness in humans. Neurosci Lett. 166:63–68. doi:10.1016/0304-3940(94)90841-9
- Drake CL, Belcher R, Howard R, Roth T, Levin AM, Gumenyuk V. 2015. Length polymorphism in the Period 3 gene is associated with sleepiness and maladaptive circadian phase in night-shift workers. J Sleep Res. 24:254–61. doi:10.1111/jsr.12264
- Eastman CI, Gazda CJ, Burgess HJ, Crowley SJ, Fogg LF. 2005. Advancing circadian rhythms before eastward flight: A strategy to prevent or reduce jet lag. Sleep. 28:33–44. doi:10.1093/sleep/28.1.33
- Eastman CI, Tomaka VA, Crowley SJ. (2016). Circadian rhythms of European and African-Americans after a large delay of sleep as in jet lag and night work. Sci Rep. 6:20–22. doi:10.1038/srep36716. Internet.
- Ebisawa T, Uchiyama M, Kajimura N, Mishima K, Kamei Y, Katoh M, Watanabe T, Sekimoto M, Shibui K, Kim K, et al. 2001. Association of structural polymorphisms in the human period3 gene with delayed sleep phase syndrome. EMBO Rep. 2:342–46. doi:10.1093/embo-reports/kve070
- Flynn-Evans EE, Shekleton JA, Miller B, Epstein LJ, Kirsch D, Brogna LA, Burke LM, Bremer E, Murray JM, Gehrman P, et al. 2017. Circadian phase and phase angle disorders in primary insomnia. Sleep. 40. doi:10.1093/sleep/zsx163
- Goel N, Banks S, Mignot E, Dinges DF, Bartell PA. 2009. PER3 polymorphism predicts cumulative sleep homeostatic but not neurobehavioral changes to chronic partial sleep deprivation. PLoS One. 4:e5874. doi:10.1371/journal.pone.0005874
- Gooley JJ, Chamberlain K, Smith KA, Khalsa SBS, Rajaratnam SMW, Van Reen E, Zeitzer JM, Czeisler CA, Lockley SW. 2011. Exposure to room light before bedtime suppresses melatonin onset and shortens melatonin duration in humans. J Clin Endocrinol Metab. 93:E463–E472. doi:10.1210/jc.2010-2098
- Groeger JA, Viola AU, Lo JCY, Von Schantz M, Archer SN, Dijk DJ. 2008. Early morning executive functioning during sleep deprivation is compromised by a PERIOD3 polymorphism. Sleep. 31:1159–67. doi:10.5665/sleep/31.8.1159
- Hida A, Kitamura S, Katayose Y, Kato M, Ono H, Kadotani H, Uchiyama M, Ebisawa T, Inoue Y, Kamei Y, et al. 2014. Screening of clock gene polymorphisms demonstrates association of a PER3 polymorphism with morningness-eveningness preference and circadian rhythm sleep disorder. Sci Rep. 4:1–6. doi:10.1038/srep06309
- Hoddes E, Dement W, Zarcone V. 1972. The development and use of the Stanford sleepiness scale (SSS). Psychophysiology. 369–70. https://doi.org/10.1007/978-1-4419-9893-4_91
- Horne JA, Ostberg O. 1976. A self assessment questionnaire to determine Morningness Eveningness in human circadian rhythms. Int J Chronobiol. 4:97–110.
- Janse Van Rensburg DC, Fowler P, Racinais S. 2020a. Practical tips to manage travel fatigue and jet lag in athletes. Br J Sports Med. 55:821–22. doi:10.1136/bjsports-2020-103163
- Janse Van Rensburg DC, Jansen Van Rensburg A, Fowler P, Fullagar H, Stevens D, Halson S, Bender A, Vincent G, Claassen-Smithers A, Dunican I, et al. 2020b. How to manage travel fatigue and jet lag in athletes? A systematic review of interventions. Br J Sports Med. 0:1–11. doi:10.1136/bjsports-2019-101635.
- Keijzer H, Smits MG, Peeters T, Looman CW, Endenburg SC, Gunnewiek JM. 2011. Evaluation of salivary melatonin measurements for Dim Light Melatonin Onset calculations in patients with possible sleep-wake rhythm disorders. Clin Chim Acta. 412:1616–20. doi:10.1016/j.cca.2011.05.014
- Kennaway DJ. 2020. Kennaway DJ measuring melatonin by immunoassay. J Pineal Res. 69:e12657. doi:10.1111/jpi.12657
- Knutson KL, Spiegel K, Penev P, Van Cauter E. 2007. The metabolic consequences of sleep deprivation. Sleep Med Rev. 11:163–78. doi:10.1016/j.smrv.2007.01.002
- Kölling S, Treff G, Winkert K, Ferrauti A, Meyer T, Pfeiffer M, Kellmann M. 2017. The effect of westward travel across five time zones on sleep and subjective jet-lag ratings in athletes before and during the 2015ʹs World Rowing Junior Championships. J Sports Sci. 35:2240–48. doi:10.1080/02640414.2016.1265141
- Kozaki T, Lee S, Nishimura T, Katsuura T, Yasukouchi A. 2011. Effects of saliva collection using cotton swabs on melatonin enzyme immunoassay. Journal of Circadian Rhythms. 9:1. doi:10.1186/1740-3391-9-1
- Kunorozva L, Stephenson KJ, Rae DE, Roden LC. 2012. Chronotype and PERIOD3 variable number tandem repeat polymorphism in individual sports athletes. Chronobiol Int. 29:1004–10. doi:10.3109/07420528.2012.719966
- Lavie P. 1986. Ultrashort sleep-waking schedule. III. “Gates” and “Forbidden zones” for sleep. Electroencephalogr Clin Neurophysiol. 63:414–25. doi:10.1016/0013-4694(86)90123-9
- Lavric A, Pompe MT. 2014. Do blue-light filtering intraocular lenses affect visual function? Optom Vis Sci. 91:1348–54. doi:10.1097/OPX.0000000000000390
- Lázár AS, Slak A, JCY L, Santhi N, Von Schantz M, Archer SN, Groeger JA, Dijk DJ. 2012. Sleep, diurnal preference, health, and psychological well-being: A prospective single-allelic-variation study. Chronobiol Int. 29:131–46. doi:10.3109/07420528.2011.641193
- Lee A, Galvez JC. 2012. Jet lag in athletes. Sports Health. 4:211–16. doi:10.1177/1941738112442340
- Lo JC, Groeger JA, Santhi N, Arbon EL, Lazar AS, Hasan S, von Schantz M, Archer SN, Dijk DJ, Yamazaki S. 2012. Effects of partial and acute total sleep deprivation on performance across cognitive domains. Individuals and Circadian Phase PLoS One. 7:e45987. doi:10.1371/journal.pone.0045987
- Lok R, Kchj S, Beersma DGM, de Kort Yaw, de Kort YAW. 2018. Light, alertness, and alerting effects of white light: A literature overview. J Biol Rhythms. 33:589–601. doi:10.1177/0748730418796443
- Maire M, Reichert CF, Gabel V, Viola AU, Krebs J, Strobel W, Landolt HP, Bachmann V, Cajochen C, Schmidt C. 2014a. Time-on-task decrement in vigilance is modulated by inter-individual vulnerability to homeostatic sleep pressure manipulation. Front Behav Neurosci. 8:1–10. doi:10.3389/fnbeh.2014.00059
- Maire M, Reichert CF, Gabel V, Viola AU, Strobel W, Krebs J, Landolt HP, Bachmann V, Cajochen C, Schmidt C. 2014b. Sleep ability mediates individual differences in the vulnerability to sleep loss: Evidence from a PER3 polymorphism. Cortex. 52:47–59. doi:10.1016/j.cortex.2013.11.008
- Micic G, Lovato N, Gradisar M, Burgess HJ, Ferguson SA, Kennaway DJ, Lack L. 2015. Nocturnal melatonin profiles in patients with delayed sleep-wake phase disorder and control sleepers. J Biol Rhythms. 30:437–48. doi:10.1177/0748730415591753
- Moline ML, Pollak CP, Monk TH, Lester LS, Wagner DR, Zendell SM, Graeber RC, Salter CA, Hirsch E. 1992. Age-related differences in recovery from simulated jet lag. Sleep. 15:28–40. doi:10.1093/sleep/15.1.28
- Mongrain V, Carrier J, Dumont M. 2006. Circadian and homeostatic sleep regulation in morningness-eveningness. J Sleep Res. 15:162–66. doi:10.1111/j.1365-2869.2006.00532.x
- Monk TH, Buysse DJ, Kennedy KS, Potts JM, DeGrazia JM, Miewald JM. 2003. Measuring sleep habits without using a diary: The sleep timing questionnaire. Sleep. 26:208–12. doi:10.1093/sleep/26.2.208
- Nadkarni NA, Weale ME, Von Schantz M, Thomas MG. 2005. Evolution of a length polymorphism in the human PER3 gene, a component of the circadian system. J Biol Rhythms. 20:490–99. doi:10.1177/0748730405281332
- Ozsoy F, Yigit S, Nursal AF, Kulu M, Nevin K. 2021. The impact of PER3 VNTR polymorphism on the development of schizophrenia in a Turkish population. Cytol Genet. 55:188–93. doi:10.1242/dev.157966
- Pereira DS, Tufik S, Louzada FM, Benedito-Silva AA, Lopez AR, Lemos NA, Korczak AL, D’Almeida V, Pedrazzoli M. 2005. Association of the length polymorphism in the human Per3 gene with the delayed sleep-phase syndrome: Does latitude have an influence upon it? Sleep. 28:29–32. doi:10.1093/sleep/28.1.29
- Phillips AJK, Vidafar P, Burns AC, McGlashan EM, Anderson C, Rajaratnam SMW, Lockley SW, Cain SW. 2019. High sensitivity and interindividual variability in the response of the human circadian system to evening light. Proc Natl Acad Sci U S A. 116:12019–24. doi:10.1073/pnas.1901824116
- Qian J, Fajl S. 2016. Circadian system and glucose metabolism: Implications for physiology and disease. Trends Endocrinol Metab. 27:282–93. doi:10.1016/j.tem.2016.03.005
- Rajaratnam SMW, Middleton B, Stone BM, Arendt J, Dijk DJ. 2004. Melatonin advances the circadian timing of EEG sleep and directly facilitates sleep without altering its duration in extended sleep opportunities in humans. J Physiol. 561:339–51. doi:10.1113/jphysiol.2004.073742
- Revell VL, Kim H, Tseng CY, Crowley SJ, Eastman CI. 2005. Circadian phase determined from melatonin profiles is reproducible after 1 wk in subjects who sleep later on weekends. J Pineal Res. 39:195–200. doi:10.1111/j.1600-079X.2005.00236.x
- Roach GD, Sargent C. 2019. Interventions to minimize jet lag after westward and eastward flight. Front Physiol. 10:1–11. doi:10.3389/fphys.2019.00927
- Ruderman NB, Saha AK. 2006. Metabolic syndrome: Adenosine monophosphate-activated protein kinase and malonyl coenzyme A. Obesity (Silver Spring). Suppl 14:25S–33S. doi:10.1038/oby.2006.279
- Rüger M, St Hilaire MA, Brainard GC, Khalsa SBS, Kronauer RE, Czeisler CA, Lockley SW. 2013. Human phase response curve to a single 6.5 h pulse of short-wavelength light. J Physiol. 591:353–63. doi:10.1113/jphysiol.2012.239046
- Sletten TL, Segal AY, Flynn-Evans EE, Lockley SW, Rajaratnam SMW. (2015). Inter-individual differences in neurobehavioural impairment following sleep restriction are associated with circadian rhythm phase. PLoS One [Internet]. 10:1–16. doi:10.1371/journal.pone.0128273
- Sletten TL, Vincenzi S, Redman JR, Lockley SW, Rajaratnam SM. 2010. Timing of sleep and its relationship with the endogenous melatonin rhythm. Front Neurol. 1:137. doi:10.3389/fneur.2010.00137
- Smith MR, Eastman CI. 2009. Phase delaying the human circadian clock with blue-enriched polychromatic light. Chronobiol Int. 26:709–25. doi:10.1080/07420520902927742
- Smith MR, Revell VL, Eastman CI. 2009. Phase advancing the human circadian clock with blue-enriched polychromatic light. Sleep Med. 10:287–94. doi:10.1016/j.sleep.2008.05.005
- Spiegel K, Knutson K, Leproult R, Tasali E, Van Cauter E. 2005. Sleep loss: A novel risk factor for insulin resistance and Type 2 diabetes. J Appl Physiol. 99:2008–19. doi:10.1152/japplphysiol.00660.2005
- Taillard J, Philip P, Coste O, Sagaspe P, Bioulac B. 2003. The circadian and homeostatic modulation of sleep pressure during wakefulness differs between morning and evening chronotypes. J Sleep Res. 12:275–82. doi:10.1046/j.0962-1105.2003.00369.x
- Tasali E, Leproult R, Ehrmann DA, Van Cauter E. 2008. Slow-wave sleep and the risk of type 2 diabetes in humans. Proc Natl Acad Sci U S A. 105:1044–49. doi:10.1073/pnas.0706446105
- Vandewalle G, Archer SN, Wuillaume C, Balteau E, Degueldre C, Luxen A, Dijk DJ, Maquet P. 2011. Effects of light on cognitive brain responses depend on circadian phase and sleep homeostasis. J Biol Rhythms. 26:249–59. doi:10.1177/0748730411401736
- Van Dongen HPA, Bender AM, Dinges DF. 2012. Systematic individual differences in sleep homeostatic and circadian rhythm contributions to neurobehavioral impairment during sleep deprivation. Accid Anal Prev. 45:11–16. doi:10.1016/j.aap.2011.09.018
- Viola AU, Archer SN, James LMM, Groeger JA, Lo JCY, Skene DJ, von Schantz M, Dijk DJ. 2007. PER3 polymorphism predicts sleep structure and waking performance. Curr Biol. 17:613–18. doi:10.1016/j.cub.2007.01.073
- Viola AU, Chellappa SL, Archer SN, Pugin F, Götz T, Dijk DJ, Cajochen C. 2012. Interindividual differences in circadian rhythmicity and sleep homeostasis in older people: Effect of a PER3 polymorphism. Neurobiol Aging. 33:1010.e17–27. doi:10.1016/j.neurobiolaging.2011.10.024
- Voinescu BI, Coogan AN. 2012. A variable-number tandem repeat polymorphism in PER3 is not associated with chronotype in a population with self-reported sleep problems. Sleep Biol Rhythms. 10:23–26. doi:10.1111/j.1479-8425.2011.00514.x
- Waterhouse J, Reilly T, Atkinson G, Edwards B. 2007. Jet lag: Trends and coping strategies. Lancet. 369:1117–29. doi:10.1016/S0140-6736(07)60529-7
- Watson LA, Phillips AJK, Hosken IT, McGlashan EM, Anderson C, Lack LC, Lockley SW, Rajaratnam SMW, Cain SW. 2018. Increased sensitivity of the circadian system to light in delayed sleep–wake phase disorder. J Physiol. 596:6249–61. doi:10.1113/JP275917
- Wehr TA, Aeschbach D, Duncan J. 2001. Evidence for a biological Dawn and dusk in the human circadian timing system. J Physiol. 535:937–51. doi:10.1111/j.1469-7793.2001.t01-1-00937.x
- Weingarten JA, Collop NA. 2013. Air travel: Effects of sleep deprivation and jet lag. Chest. 144:1394–401. doi:10.1378/chest.12-2963
- Wen B, He M, Song J, Zong B, Lu Y, Shu X. 2000. Study on DeNOx performance of Ce-Mg-Al mixed oxides. Chinese J Catal. 21:34.
- Winget CM, Deroshia CW, Markley CL, Holley DC. 1984. A review of human physiological and performance changes associated with desynchronosis of biological rhythms. Aviat Sp Environ Med. 55:1086–96.
- Wirth M, Burch J, Violanti J, Burchfiel C, Fekedulegn D, Andrew M, Zhang H, Miller DB, Youngstedt SD, Hébert JR, et al. 2013. Association of the period3 clock gene length polymorphism with salivary cortisol secretion among police officers. Neuro Endocrinol Lett. 34:27–37.
- World Medical Association 2013. World Medical Association Declaration of Helsinki: ethical principles for medical research involving human subjects. JAMA. 310:2191–2194. doi:10.1001/jama.2013.281053.
- Wright KP, Drake AL, Frey DJ, Fleshner M, Desouza CA, Gronfier C, Czeisler CA. 2015. Influence of sleep deprivation and circadian misalignment on cortisol, inflammatory markers, and cytokine balance. Brain Behav Immun. 47:24–34. doi:10.1016/j.bbi.2015.01.004
- Wright HR, Lack LC, Kennaway DJ. 2004. Differential effects of light wavelength in phase advancing the melatonin rhythm. J Pineal Res. 36:140–44. doi:10.1046/j.1600-079X.2003.00108.x
- Zacharko M, Konefał M, Ł R, Chmura P, Błażejczyk K, Chmura J, Andrzejewski M. 2020. Direction of travel of time zones crossed and results achieved by soccer players. The road from the 2018 FIFA world cup to UEFA EURO 2020. Res Sport Med. 30:145–55. doi:10.1080/15438627.2020.1853545