Abstract
Introduction: This study investigated the health effects of two different architectural glass types: A two-layered low-iron high transmittance glass and a three-layered low energy glass with lower transmittance. The study investigated how these glass types affected daylight conditions in 72 residential apartments, as well as health and satisfaction of the residents.
Methods: The study installed high transmittance glass (light transmittance LT:0.82) in 36 apartments and low transmittance (LT:0.74) in 36 identical apartments. The study then analyzed the light transmittance of each glass type in the laboratory and analyzed the indoor environmental quality (IEQ) in eight representative apartments before and after renovation. Self-reported questionnaires were handed out and collected before and after renovation.
Results: The results showed that the glass types differed significantly in measured daylight transmittance. The two-layered high transmittance glass transmitted 15% more visual light (380–750 nm) and 20% more light in the spectral range (460–480 nm), stimulating ipRGCs and circadian rhythm, when compared to three-layered low energy glass. In addition, significant differences were observed in the UV-B spectrum (280–315 nm). While two-layered high transmittance glass transmitted UV-B, three-layered low transmittance glass did not. During the 12-month study period, residents in apartments with three-layered low energy glass reported more difficulties sleeping (p = 0.05), higher satisfaction with daylight (p = 0.03) and higher satisfaction with ventilation (p = 0.04). Residents in apartments with three-layered low energy glass experienced fewer days with too cold indoor temperatures (p = 0.02), compared to residents with two-layered low-iron glass. The results of energy consumption for heating showed that two-layered low-iron glass reduced the energy consumption by 11.0%, while three-layered low energy glass reduced the energy consumption by 9.4%, compared to the year prior to renovation.
Conclusion: The results contribute to a discussion about potential energy savings on one hand and potential non-energy benefits, such as daylight quality, overall health, and total economy/life cycle assessment of the built environment on the other hand. The results suggest further research performed in randomized large-scale studies.
1. Introduction
In recent years, research has provided novel insights into the importance of daylight in human health [Citation1–5]. Today, people spend most of their time indoors in built environments. This ‘indoor generation’ on average spends approximately 90% of the time in the indoor environment [Citation6] and is exposed to lower light intensity levels. This may impact. circadian rhythm, sleep quality and sleep regulation, comfort, mood as well as the ability to form vitamin D. Over the past decades, research has created an awareness of the importance of daylight in the general population. The window’s primary function, providing daylight and fresh air into the built environment, as well as a view out, in this context, is essential. This project focuses on light transmittance and daylight quality and looks at different architectural glass types and their effect on daylight quality and health.
In recent years, optimizing the energy performance of windows as a climate screen, has played a central role. Strict requirements on energy consumption, together with political demands on the independence of fossil fuels by 2050 [Citation7], have put renewed focus on the energy efficiency of the built environment. In Denmark, it is estimated that energy consumption for heating must be reduced by approximately 40% by 2050 for the green transition to take place most cost-effectively [Citation8]. In this respect, residential buildings built before 1985 have great potential. This is partly because these buildings account for approximately 67% of the total built area in Denmark and partly because they are built prior to most energy efficiency requirements. Since the first Danish energy requirements were introduced in 1961, the requirements have been tightened dramatically, from a demand equivalent of approximately 400 W/m2 per year to 41 W/m2 per year [Citation9]. This corresponded to a total energy reduction of approximately 90%. If the same high percentage of energy savings should be implemented again today, this would only account for an additional 9% of the initial savings already accounted for. This provides good reasons to focus on other drivers, such as daylight quality and health, when choosing the right window types, which is the focus of this study. Efforts to reduce future energy consumption are likely to be driven by other factors, such as broad-term sustainability, including indoor climate, which is within the framework of this study.
In the future, the largest part of the construction business will consist of renovations of existing buildings, including improved climate screens, facades, and windows. Therefore, this study is relevant, and its results may be useful in future renovations, which alone account for approximately 350,000 buildings in Denmark.
2. Method
Methodically, this project simultaneously examined and compared the effects before and after the renovation of two identical buildings. The intervention consisted of energy renovation of all existing windows in both buildings by replacing the existing 2-layered thermo-windows with the following window types:
3-layered low energy glass, Building A (Kløvervej 66 – 92, Haderslev, Denmark)
2-layered low-iron glass, Building B (Kløvervej 116 – 142, Haderslev, Denmark)
The study design was a block-randomized cohort study with two identical buildings ( and ). Sociodemographic and health effect data were collected before the renovation, September 2016–January 2017 (baseline measurement), and after the renovation, September 2017–January 2018 (effect measurement). Data on spectral light distribution, indoor environmental quality, self-reported general health, and satisfaction of residents, together with the measurement of energy consumption for heating, were collected for each of the two identical buildings, comparing the effect of the two different glass types with existing glass. All daylight measurements were performed at baseline (autumn equinox, 20 September 2016 before renovation), and endpoint (autumn equinox, 20 September 2017 after renovation).
Figure 1. Site plan and orientation of the two identical residential buildings. Building A, Kløvervej 66–92 with low transmittance glass (top) and building B, Kløvervej 116–142 with high transmittance glass (bottom). Inhabited, representative apartments (dark and light grey) for each floor were chosen for onsite daylight measurements between buildings at autumn equinox, before and after implementation of glass types, respectively.
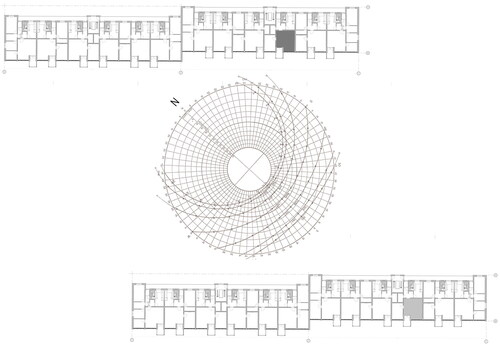
Figure 2. Elevation of SW-facing façade before (top) and after renovation (bottom), glass area marked with black.
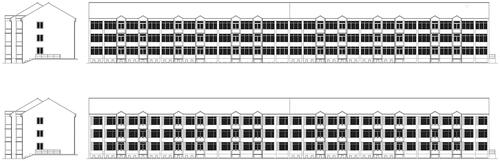
2.1. Experimental setup
The project took place in a social housing facility consisting of two identical 3-storeys residential buildings built in 1959, Kløvervej 66–92 and Kløvervej 116–142 in Haderslev, Denmark (Google map coordinates: 6FPW + 8M Haderslev, 6FMW + W9 Haderslev). This social housing facility was chosen because it represents a large number of properties built before 1987, before specific requirements on energy consumption were enforced. Hence, social housing represents a typical building expected to be renovated in Denmark in the coming years.
The study included 72 apartments, with a total gross area of 5,580 m2. All existing windows (originally from 1995) were replaced from June 2017 to August 2017, except for the front doors. The project implemented two different glass types in the two buildings. All apartments had the same window area before and after the replacement, except for the main windows in the SW-facing facades. Here the existing window area was reduced in both residential buildings so that the total glass/floor-area ratio changed from 25.5 to 23.8% in the largest rooms of all apartments, when compared to before renovation. This was performed to avoid potential overheating in both buildings.
The original partition of windows from 1959, with large, central windows and openable side windows, was re-introduced. However, all window sections were divided horizontally, with smaller window sections at the top, having manual openings for natural ventilation. One section of the main window in the SW-facing rooms was blinded, as shown in . This reduced the glass to floor area from 25.5 to 23.8%, corresponding to a total reduction of 0.38 m2, from A = 5.61 m2 to A = 5.23 m2 in the largest rooms (22 m2). This complied with the daylight requirements in Danish Building Class 2015 [Citation10], and reduction meant that the window sections, from being very large, became better balanced in relation to the size of the rooms and the external heat load from the sun. In the choice of frame construction, the choice of windows was based on two requirements: (1) cheapest supply and (2) delivery of both 2-layered and 3-layered window solutions from the same supplier. Under these terms and conditions, the end result was a window type with a glass-to-frame ratio (Ff) of 0.71. This applied for both buildings ().
Table 1. Specifications on types of window glass implemented.
2.2. Measurements of indoor environmental quality
Indoor environment conditions were evaluated before and after renovation by collection of data on the following parameters:
2.3.1 Daylight intensity measured on site in test apartments in terms of daylight factor measurements.
2.3.2 Spectral transmission of the various glass types was measured under laboratory conditions, for simulation of daylight transmission.
2.3.3 Indoor environmental quality, indoor climate factors measured on site in test apartments included CO2, temperature, and relative air humidity (RH%).
2.3.4 Residents’ self-reported sleep quality, mood, satisfaction, etc.
2.3.5 Annual energy consumption for heating, before and after renovation for each residential building.
2.3. Data collection
2.3.1. Daylight onsite measurements
Measurements of daylight conditions were performed on site in 8 representative test apartments, 4 apartments in building A and 4 apartments in building B. Daylight measurements were temporally synchronized onsite, using mobile phone contact. This measurement method allowed the precise simultaneous recording of daylight for each apartment. Comparing daylight in apartments with 2-layered low-iron windows with daylight in apartments with low energy windows. These measurements were performed before (baseline) and after (effect) the renovation. In this way, differences at the baseline and endpoint between apartments could be registered (). The daylight was measured horizontally at a height of 0.8 m and with measurement distances of 0, 1, 2, and 3 m from the window in the largest room (living room). The measurements were performed using two identical instruments (Metrue Inc). SIM-2 Plus spectral irradiance meter (Hayward, CA 94545, USA). All measurements were carried out simultaneously for each apartment in Buildings A and B under the same outdoor lighting conditions. Reference measurements were performed outside the area between the two buildings. Measurements in the test apartments included illuminance, CO2-levels, temperature, and relative humidity (RH%).
2.3.2. Laboratory measurements
On-site measurements were supplemented by laboratory measurements of the transmittance of three glass samples, including the two glass types used in the apartments (). The spectral light transmittance was measured under controlled conditions in a laboratory at DTU Electro using a Cary 50 spectrophotometer (Agilent Technologies), this device uses a full spectrum Xe pulse lamp and a Czerny-Turner spectral selector to create a monochrome tunable light source used to scan the wavelength range, measuring the direct transmission of light. The resulting daylight transmission was then simulated using a CIE D65 standard illuminant as a virtual light source (www.doi.org/10.25039/CIE.DS.hjfjmt59). The simulated spectral irradiance from D65 was normalized to approximately. illuminance value at 1 m from the window, corresponding to 2500 lx. The results concerning circadian stimuli were evaluated using the spectral weighting functions defined in the toolbox CIE S 026/E:2018 [Citation11].
2.3.3. Indoor climate measurements
The indoor climate was measured onsite using an IC-meter GSM version 4.2. IC meter units were placed vertically 1.2 m above the floor. IC meter units were mounted on the wall of the largest SW-facing room in the selected representative apartments, monitoring temperature, CO2 levels, and relative air humidity (RH%). Data collection took place online every five minutes for a consecutive period of three weeks, before and after the renovation. Data were subsequently extracted and analyzed before and after renovation. The data included average values of the registered indoor climate on weekdays [Citation12].
2.3.4. Questionnaires
The sociodemographic data, including residents’ health, and satisfaction with daylight quality and indoor environmental quality were registered using a questionnaire, with the option of responding digitally, using the SurveyXact (Rambøll Management Consulting) or in pen-and-paper. The questionnaires were distributed on paper to all apartments. Questionnaires were distributed before and after renovation [Citation12]. With non-responses to questionnaires, a telephone-based survey was conducted. Both baseline and effect measurements used the same questionnaire, which included data on self-reported sleep quality, mood, satisfaction with daylight, and indoor environmental quality. Questionnaires were distributed to all residents before and after renovation.
2.3.5. Energy consumption
Data for heating were collected by Haderslev Fjernvarme/Haderslev District Heating. Based on the data, the project compared the general energy consumption for heating in MWh per year before and after renovation for each of the two residential buildings. Data included energy consumption four years before renovation and one year after renovation (). Only the energy consumption for heating was included. Registrations did not include the power consumption for artificial lighting. Data on the energy consumption for heating were collected for each of the two separate buildings based on official tables of adjusted degree-days from Haderslev District Heating.
2.3.6. Ethical considerations
The researchers received permission to access the test apartments through the chairman of the resident association, approved by the main board of the Boligselskab HAB. Residents were informed about the project at the meetings and question cafes. The residents were told that the project would investigate daylight quality and the effect of daylight on their overall satisfaction and general health using two state-of-the-art glass types. Residents were informed about the process, the residents were not told what specific glass types they would receive in their building.
2.3.7. Regulatory approvals
The project was approved by the Danish Data Protection Agency.
2.3.8. Statistical analysis
All data from questionnaires from residents who had lived in residential buildings during the entire project period and who had answered questionnaires both before and after the renovation were used for analyzes. This included 34 residents, with 17 apartments with low energy windows and 17 apartments with high transmittance windows. We analyzed the data using SAS software. Sociodemographic variables were analyzed using an unpaired t-test or non-parametric test depending on whether the data were normally distributed. All comparisons between the residents in the two buildings were based on nominal logistic regression analysis with baseline control for the respective outcome. Fisher’s exact test was also used for table analysis. As this was a pilot study meant for generating hypotheses for larger scale studies, and with no primary outcome, we did not correct for multiple analyses.
3. Results
3.1. Daylight illuminance on-site measurements
Results of the measurements in the test apartments showed a significantly higher daylight illuminance (15%) in the apartments with high transmittance glass (10.600 lx), compared to the apartments with low energy glass (9.000 lx) after the renovation. In addition, when correcting for smaller differences between the two buildings at the baseline (see ).
Figure 4. On site measurements of horizontal illuminance measured at height 0.8 m. Before renovation (top) and after renovation (bottom), respectively. Data for representative apartments is shown for building A (low transmittance glass) (blue) and building B (high transmittance glass) (red). Measurements were carried out simultaneously at distances of 0, 1, 2 and 3 m from the windows the graphs show illuminance average (points), standard deviation (error bars) and fitted exponential decrease (lines).
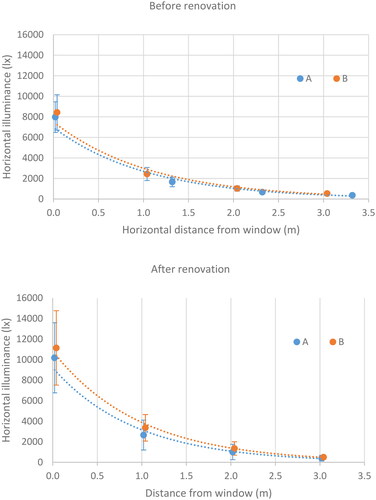
3.2. Daylight quality simulations as laboratory measurements
Results of the spectral light transmission measurements of the glazing used in the daylight quality simulations showed that 3-layer glass overall transmitted lees visual daylight and further reduced transmission in the UV and NIR ranges. The 2-layer glass showed the overall highest transmission in the visible and UV regions, whereas the existing glass showed a high transmission of visible daylight and transmission in the IR region, together with a low transmission in the UV region ().
Figure 5. Spectral transmittance of the glass used in the study, measured under laboratory conditions. Existing glass type (yellow), high transmittance glass/2-layered low-iron glass (blue) and low transmittance glass/3-layered low energy glass type (green)(left). Daylight spectrum CIE D65 (right).
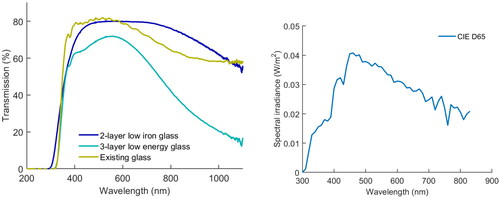
The short-wave spectral range, known for stimulating the circadian rhythm, was significantly reduced by the low energy window solution when compared to the existing window solution (before renovation) and 2-layered low-iron glass solution. The results showed that high transmittance glass transmitted approximately 20% more short-wave light in this spectral range than low energy glass. Overall, the 2-layered low-iron windows transmitted most of the full daylight spectrum, when compared to low energy glass and existing windows. Overall, the reductions occurred primarily in the short-waved and long-waved areas of the spectrum, with the least reduction in the visible spectrum from 380 to 750 nm.
shows the irradiance weighted according to the circadian stimulus described in CIE S 026, the resulting irradiances are based on the CIE D65 spectral illuminant, normalized to 2500 lx, and compared to direct transmission (with no glass). The high transmittance windows showed comparable results to the existing windows, while the 3-layer windows reduced the overall irradiances in the active α-optic spectrum responsible for the circadian stimulus.
Figure 6. Transmitted irradiance calculated using the CIE standard illuminant D65 as the source for each glass type and weighted by the five retinal photoreceptor responsivities. “no glass” represents the D65 with no filtering/without glass. NB. Peak sensitivity for each individual retinal photoreceptor, cyanopic S cone 419.0 nm. Melanopic melanopsin 480.0 nm. Rhodopic rod 496.3 nm. Chloropic M cone 530.8 nm. Erythropic L cone 558.4 nm.
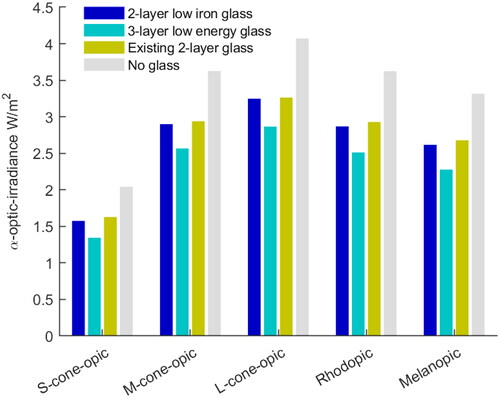
3.3. Spectral UV-B light distribution
In the laboratory, the glass transmittance was also analyzed in the short wavelength non-visual range, here we focused on the UV-B range (280–315 nm), which is the active spectral range in the formation of vitamin D. The laboratory measurements showed the following results ().
Figure 7. Transmittance of UV-B light spectrum (%) through the different glass type samples in the study. Existing glass/2-layered glass type (yellow), high transmittance glass/2-layered low-iron glass (blue) and low transmittance glass/3-layered low energy glass (green). Upper threshold for UV-B light is marked with a dotted line.
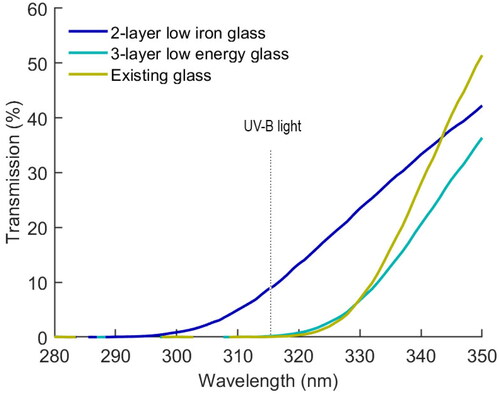
High transmittance glass transmitted approximately 4.5% UV-B light in the spectral range 280–315 nm, while low-iron glass transmitted 0% UV-B. Existing glass transmitted no measurable light (approximately 0%) UV-B light.
The results of laboratory measurements showed that 3-layered glass efficiently blocked UV-B light, acting as an effective barrier for the UV-A light spectrum (315–380 nm) and UV-B light spectrum (280–315 nm), transmitting less than 0.5% UV-B light. This result demonstrates how different glass types can create a barrier for ingress of UV-B in the built environment.
3.4. Indoor environmental quality
The results showed a better balanced average comfort temperature between 22 and 23 °C after the renovation, when compared to before. The results showed that the average CO2-levels went up from 650 ppm to 900 ppm after renovation in both apartments with low energy glass and 2-layered low-iron glass. The relative air humidity also increased from RH 50% to RH 58%, in both apartments with low energy glass and apartments with 2-layered low-iron glass, with CO2-levels and RH being highest during the nighttime.
3.5. Sociodemographic
The sociodemographic data showed a statistically significant difference between the buildings in terms of residents’ connections to the labor market. In apartments with low energy glass, only 5.9% of the residents were associated with the labor market, while this was the case for 47.1% of the residents in apartments with 2-layered low-iron glass (p = 0.02). The median age for residents in apartments with low energy glass was 70.3 (SD = 19.3) years, and 55.4 (SD = 22.2) years in apartments with 2-layered low-iron glass (X2=3.6) (p = 0.06). There were no other significant differences in any of the other sociodemographic data (Supplementary Table 2).
3.6. Questionnaire data
The results of the questionnaires showed statistically significant differences in the occupant’s general health. The residents in apartments with low energy windows reported more difficulties in some sleeping measures after the renovation, when compared to residents in apartments with 2-layered low-iron glass (trouble breathing p = 0.06; coughing/snoring p = 0.05) (2.29 Supplementary Table 3). The results also showed statistically significant differences between residents in terms of satisfaction with indoor environmental quality. Residents in apartments with low energy windows were more satisfied with the ventilation and air in their apartments after the renovation (3.1 g Supplementary Table 4), when compared to the residents in the apartments with 2-layered low-iron glass (p = 0.04). Residents in the apartments with low energy glass reported higher satisfaction with daylight (p = 0.03) (3.8 Supplementary Table 4) and furniture colors (p = 0.05) (3.2a Supplementary Table 4), when compared to residents in apartments with 2-layered low-iron glass. Residents in apartments with low transmittance glass also reported fewer days with too much cold in apartments when compared to residents in apartments with 2-layered low-iron glass (X2 = 5.7, p = 0.02). Finally, residents of apartments with 2-layered low-iron glass reported more work difficulties due to physical pain after renovation (p = 0.05).
3.7. Energy performance
The energy consumption for heating was reduced significantly in both buildings as a result of the new glass type. Overall, the energy consumption for heating was reduced by approximately 20 MWh per year in both buildings when compared to the last four years before the renovation. When comparing energy consumption for heating after the renovation, season 2017–18 with before the renovation, season 2016–17, results showed that energy consumption for heating in the building with low energy glass decreased by 9.4%, while energy consumption for heating in the building with 2-layered low-iron glass decreased by 11.0% ().
4. Discussion
4.1. Daylight quality
The results of on-site daylight measurements showed that the glass quality and number of glass layers had a significant effect on daylight intensity in apartments. The 2-layered low-iron glass transmitted approximately 15% more daylight than the 3-layered low energy glass did. These results were supported by the results from the laboratory, showing that this also applies to the active range stimulating the circadian rhythm (460–480 nm), which is reduced by approximately 20% through 3-layered low energy glass compared to 2-layered low-iron glass.
The laboratory results showed significant differences in the transmission of UV-A and UV-B light. Only the 2-layered low-iron glass transmitted significant amounts of UV-B light (280–315 nm). The 3rd layer of glass efficiently blocked the active spectral UV-B range for both the 3-layered low energy glass and the 3-layered low-iron glass; hence, the possibility of forming vitamin D in the indoor built environment is negligible behind 3-layered glass types. Several studies have pointed out that this is the case [Citation13], and that we are not able to form active vitamin D in the indoor environment. However, this study finds that 2-layered low-iron glass actually transmits UV-B light, breaking the barrier between UV-A and UV-B in the built environment.
Vitamin D is important for human health since Vitamin D plays a vital role in facilitating the absorption of calcium and phosphorus, which is crucial for optimal bone and teeth health. A deficiency in D vitamin can lead to osteoporosis, especially for elderly people. Sufficient D-vitamin levels are also important for our muscle function and our immune system. Detrimental effects of UV exposure are related to DNA damage and erythema. However, the estimated doses are much lower than the regulative doses and therefore no detrimental UV-B effects are expected to take place.
Sunlight is the primary and most important source of human vitamin D, and people in the Western World spend approximately 90% of their time indoors. Hence, they do not get enough sunlight to form vitamin D, according to this study most likely because virtually any glass type absorbs and reflects natural UV-B light, which is a prerequisite to convert 7-dihydro-cholesterol to active vitamin D, 1.25 (OH) 2D. The National Board of Health’s recommendation for vitamin D is 50 nmol/l blood, with an absolute minimum of 25 nmol/l. As a rule of thumb, we lose approximately 1 nmol/l per week during winter [Citation14]. If we start up having a concentration of 50 nmol/l in the blood in late summer, in September, we will typically have a deficit, corresponding to 19 nmol/l vitamin D approximately 31 weeks later, by the end of April,. However, if the amount of UV-B light indoors is boosted, during the summer and late autumn, we may instead start from 75 nmol/l, and instead terminate at 56 nmol/l. Based on these findings, a 2-layered low-iron glass type might make a difference. It is important to note that while the 2-layered low-iron glass actually can transmit UV-B radiation that activates the D vitamin in the human skin, this radiation decreases significantly as the distance from the window increases. As a result, the strongest possible effect is expected to be near the window. Furthermore, it is important that the residents are present at the window for a sufficiently long time to obtain any D-vitamin improvement effect.
Furthermore, laboratory showed that 2-layered low-iron glass transmits more germicidal UV-B light in the spectral range 295–315 nm, having a potential antiseptic effect on airborne bacteria [Citation15], bacterial colonies, and viruses such as COVID-19. Both UV-A and UV-B radiations are known to disinfect these microorganisms; however, UV-B is much more effective than UV-A for this purpose. The UV-doses required to inactivate specific species of bacteria and viruses were reported by Masjoudi et al. [Citation16]. When the results of the spectral light transmittance through the different glass types in this study were compared to the active spectral range for antiseptic light, it is found that the germicidal effect is optimum at 265 nm, but significant germicidal effects are present up to 315 nm (see ). This leads to the conclusion that most standard and low energy glass types exhibit limited germicidal effects, as they do not transmit light in this spectrum. Of the different glass types tested in this study, only 2-layered high transmission glass transmitted this active germicidal range of the spectrum; the other glass types did not.
Figure 9. The standard germicidal effect and range of UV-B light (adapted from CIE155-2003). Source: DIN 5031-10:1979-11. Strahlungsphysik im optischen bereich und lichttechnik. Deutsches institut für normung e.V. Berlin, 1979 and IESNA, 2000b. IESNA lighting handbook, 9th edition. ed. Rea M.S. Illuminating engineering society of North America, New York, 2000. This standard germicidal range of the UV-B spectrum is not transmitted through low transmittance glass/3-layered low energy glass types (dotted vertical line = lower transmittance threshold), while up to 0.4 of the relative germicidal efficiency is transmitted through high transmittance glass/2-layered low-iron glass type (full vertical line = lower transmittance limit).
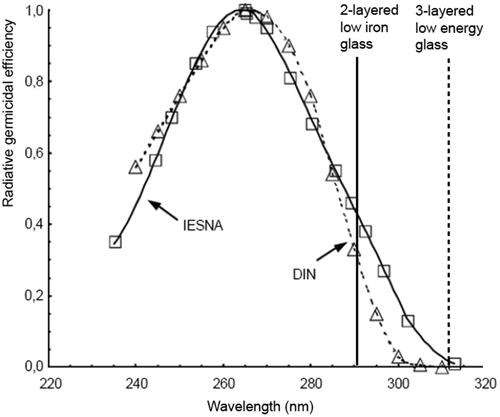
It should be noted that in this study, the implemented low transmittance glass/3-layered low energy glass had a relatively high total solar energy transmittance (g-value = 0.53) compared to standard low-energy glass types, often having lower g-values (g-value = 0.28 − 0.36); hence, this observed difference in transmittance of the glass types may be even greater. The g-value represents the transmission of the full spectrum light and is a central tenant for the short wave spectrum, affecting the circadian system, peaking in the short-waved range of 460–480 nm, as well as the UV-B range peaking in the range 280–315 nm, enabling formation of vitamin D [Citation17]. These spectra were not weighted in the light transmittance of glass (Lt), representing only the visual spectral range of 380–750 nm.
4.2. Indoor environmental quality
The results of measurements of the indoor environmental quality (IEQ) indicated that apartments in both buildings became more airtight after implementing the new window types. The levels of CO2 increased from an average of 650 ppm in the baseline measurements to an average of 900 ppm. The relative air humidity (RH) ranged from 50% to RH 58%. This was applied equally to both the residential buildings. Improved possibilities for airing-out through manual openings seemed to be insufficient, not compensating for a new generation of airtight window construction. While the existing windows (originally from 1995) established the natural infiltration of fresh air through leaks and joints, the new window types did not. Hence, more ventilation is needed to provide a sufficient supply of fresh air. The new air-tight windows in the buildings seem to call for more ventilation after renovation, the adequate air change per hour for disinfection of indoor air though, may be reduced in apartments with the high transmittance glass, since UV-B light acts as a germicidal agent. All though air changes per hour (ACH) is an important metric to ensure good air quality for disinfection, disinfection can be enhanced by supplementing the air with UV-B light. This so-called equivalent air change per hour (e-ACH) can help reduce viruses and bacteria in indoor air, even at lower air changes per hour. However, this does not solve the problem of increased levels of CO2 and supplementing with ventilation through facades is a prerequisite for a well-balanced indoor environment.
Regarding comfort temperatures, the results of onsite measurements showed more balanced temperatures in both residential buildings following renovation. Comfort temperatures ranged between 22 and 23 °C after renovation compared to 21–24 °C before renovation. This may be a result of a mandatory low-energy coating in both implemented glass types in the study; the low-energy coating reduces excessive heat loss through the window glass. Regarding overheating, the measurements showed no increased overheating after the renovation in apartments with high-transmittance glass, compared to apartments with low energy glass and existing glass. Regarding comfort, results showed that residents in apartments with 2-layered low-iron glass reported significantly more days with too much cold in the apartment (4.6 Supplementary Table 4), compared to residents in apartments with 3-layered glass (p = 0.01). However, this finding was not supported by onsite measurements of indoor temperatures in the study, revealing no difference between the buildings and showing balanced comfort temperatures (22–23 °C) in both buildings after the renovation when compared to before the renovation (21–24 °C). A possible explanation for this finding could be that 3-layered low transmittance glass types reduced the risk of cold falls, especially in tall window constructions, as implemented in this study. All large windows in the study were facing southwest, which is the prevailing wind direction in Denmark and potentially could increase the risk of cold fall and draught in apartments.
4.3. Health and satisfaction of the residents
The results showed statistically significant differences in the occupants’ self-reported sleep. The residents in apartments with low energy windows reported more difficulties in some sleeping measures after the renovation compared to residents in apartments with 2-layered low-iron glass. This is in line with daylight measurements, showing a reduction of 15% in transmitted light in the spectral range, stimulating the circadian rhythm.
Regarding daylight, the results showed a statistically significant difference in residents’ self-reported satisfaction. The residents in the building with low energy windows reported statistically significantly higher satisfaction regarding daylight characteristics, such as clarity and color of objects, compared to residents in the building with 2-layered low-iron glass. However, these results were not supported by on-site measurements and laboratory measurements, showing that low energy glass provided poorer light transmittance, less light intensity, and poorer color rendering. These findings, however, might in part be explained by age differences between the groups, with aged residents having different daylight preferences. Also this difference can be explained by the fact that residents in both buildings already lived in bright, daylit apartments, having a large glass to floor area ratio (23.8%). Finally, the age differences between the groups, with a mean age at baseline of 70.3 (SD = 19.3 years in apartments with low energy glass and 55.4 (SD = 22.2) years in apartments with 2-layered low-iron glass (p = 0.06), may explain the higher satisfaction with less daylight, since elderly people are more sensitive to glare and too bright light. Our results supported this, showing that, at endpoint, the elderly residents in the apartments with low energy glass were significantly more sensitive to light (57%) than younger residents in apartments with 2-layered low-iron glass (19%), (X2=4.7, p = 0.03), whereas there was no significant change from baseline to endpoint in light sensitivity in both groups (p = 0.13) (see 2.18 in Supplementary Table 2).
The results of general satisfaction with ventilation showed statistically significant differences between the groups. While residents’ satisfaction with apartments with low energy glass remained the same after renovation, it was reduced in apartments with 2-layered low-iron glass (3.1 g Supplementary Table 4) (p = 0.04). However, this result was not supported by on-site measurements, which showed that the air quality was comparable in both groups. A direct relationship between the difference in glass quality and ventilation seems difficult to explain, the latter resulting from new, more airtight window frame constructions and not variations in glass type. It should be mentioned that the reduction of window openings did not have any expected adverse effect on the overall satisfaction with daylight, and residents did not report more nuisance caused by passive solar heat, which might have been expected, implementing high-transmittance low-iron glass with a higher total solar factor.
The results of the questionnaires and sociodemographic data showed that several baseline measures were different between buildings, which may have induced unknown bias especially in the data only collected at endpoint. However, outcomes measured at baseline and endpoint were analyzed with control baseline, looking only at differences between endpoint values. In this case, the bias caused by baseline imbalance is likely little. As e.g. glare is more common among elderly residents, this may have been responsible for some of the results on satisfaction with daylight and color of furniture (question 3.2a) in the apartments renovated with low transmittance glass, where age was marginally higher (p = 0.06).
4.4. Energy performance
A comparison of the energy performances of the two glass types showed that the energy consumption for heating was reduced by approximately 20 MWh per year in both buildings. The exact reason for the overall energy saving is difficult to estimate, as part of the energy reduction can be explained by the increased airtightness of the building’s envelopes, which occurred at the expense of air quality (increased CO2 and RH%). However, the results indicate that 3-layered low energy windows do not provide increased energy savings (9.1%) when compared to the 2-layered low-iron windows (11%). Thus, the results suggest existing buildings do not achieve the calculated energy savings from 3-layered low energy windows. Even in the building types described in this case, having a relatively large glass area/floor area (23.8%).
5. Conclusion
This study implemented two different glass types; 2-layered low-iron high-transmittance glass and 3-layered low transmittance glass, in two identical residential buildings in Haderslev, Denmark. The study measured the on-site daylight intensity before and after renovation, as well as the spectral transmittance in the laboratory. Measurements showed that different glass types had different effects on both the daylight intensity and daylight quality. The study found significant differences in the transmittance of the visual spectral range (380–750 nm), the active spectral range of daylight stimulating the circadian system (460–480 nm), and the active spectral range of UV-B light stimulating the formation of vitamin D (280–315 nm). The latter having potential germicidal effects on bacteria and viruses. We found that low energy glass reduced light, stimulating the circadian system in the spectral range 460–480 nm by approximately 20% more than the 2-layered low-iron glass. The results showed that high transmittance glass transmitted approximately 4.5% of the outside natural UV-B light, while it was completely blocked by the 3-layered low energy glass.
The study found statistically significant differences in self-reported health. Residents in apartments with low energy glass reported more difficulties sleeping than residents in apartments with 2-layered low-iron glass. Residents in apartments with 2-layered low-iron glass reported more work difficulties due to physical pain than residents in apartments with low energy glass after renovation. This was in line with the sociodemographic data, showing a higher employment rate for residents in apartments with 2-layered low-iron glass, compared to residents of apartments with low energy glass, the latter having a higher average age, with many having reached retirement age.
The study found statistically significant differences in residents’ self-reported satisfaction with daylight. Data show higher scores from residents in apartments with low energy glass, compared to residents in apartments with high transmittance glass. This could be induced by age effects such a cataract of the eye as the mean age in thus building was marginally higher. Satisfaction with ventilation and comfort temperatures was higher in apartments with low energy glass than in compared with 2-layered low-iron glass. However, these findings were not supported by the physical onsite measurements, showing poorer daylight quality in apartments with low energy glass and no difference in indoor environmental quality (IEQ) after the renovation.
The results showed that energy consumption for heating was reduced by 11% in apartments with 2-layered low-iron glass and 9.1% in apartments with low energy glass. This was surprising, since 3-layered low energy glass should theoretically provide a lower energy consumption at the cost of less daylight. Using low transmittance glass/3-layered low energy glass types is only a rational choice if they, energy-wise, perform better than high transmittance glass/2-layered glass.
In terms of glass types, the results of the study confirmed that glass quality not only has an impact on daylight quality and energy consumption but also on the health and satisfaction of the residents. Therefore, the choice of glass should not only be based on energy considerations but also on other factors, such as the ability to transmit daylight. Based on the results of this study, ‘energy marking’ preferably may be supplemented with ‘daylight marking’, creating awareness and focus on the aspects of daylight quality, as a fix point in the choice of a high-performance window product.
Overall, the results imply that indoor daylight quality not only affects people confined to the indoor environment, such as hospitalized inpatients, it also affects healthy individuals living in residential buildings. These results imply that windows have the potential to create a good indoor environment for the ‘indoor generation’ spending 90% of their lives in the indoor environment. The built environment plays an essential role in the overall well-being and health of the population.
This is a new research field, which should be investigated further, in large-scale and long-term studies, as the results of the measurements of different glass types in this study indicate large differences in transmitted daylight intensity and daylight quality, whose health implications might be very important for circadian rhythm, vitamin D formation, and potential for reducing risks of infectious diseases in the built environment. However, these results need to be further investigated in future studies.
Supplemental Material
Download MS Word (59.7 KB)Acknowledgements
Thanks to other participants, Stefan Vestergaard, Rambøll A/S, Haderslev General Housing Company, HAB, and Haderslev Fjernvarme. Senior researchers Kjeld Johnsen and Jakob Markvart for supplementary measurements. Photos and illustrations: The authors.
Disclosure statement
No potential conflict of interest was reported by the author(s).
Additional information
Funding
References
- Berson D, Dunn FA, Motoharu T. Phototransduction by retinal ganglion cells that set the circadian clock. Science. 2002;295(5557):1–13. doi: 10.1126/science.1067262.
- Wallace‐Guy GM, Kripke DF, Jean‐Louis G, et al. Evening light exposure: implications for sleep and depression. J Am Geriat Soc. 2002;50(4):738–739. doi: 10.1046/j.1532-5415.2002.50171.x.
- Justice A, et al. ‘Natural’ light treatment of seasonal affective disorder. J Affect Disord. 1996;37(2–3):109–120.
- Boyce P, Hunter C, Howlet O. The benefits of daylight though windows; 2003. Rensselaer Polytechnic Institute, Troy.
- Gbyl K, Østergaard Madsen H, Dunker Svendsen S, et al. Depressed patients hospitalized in southeast-facing rooms are discharged earlier than patients in northwest-facing rooms. Neuropsychobiology. 2016;74(4):193–201.
- Volf C. Light, Architecture and Health [PhD-thesis]. Aarhus School of Architecture. 2013. Available from: https://www.psykiatri-regionh.dk/nid-group/Projects/built-environment/Documents/Carlo%20Volf_phd_uk_low_res.pdf
- According to Danish Energy Agency. Available from: https://ens.dk/en/our-responsibilities/energy-climate-politics/danish-climate-policies 2023
- Danish Energy Agency publication: “10 + 1 proposals for improved energy and climate policy in the EU”; 2019. Available from: https://www.danskenergi.dk/sites/danskenergi.dk/files/media/dokumenter/2019-07/10_plus_1_proposals_for_improved_energy_and_cliamte_policy_EU.pdf.
- According to Danish building regulations; 2018 (BR2018). Available from: https://bygningsreglementet.dk.
- According to Danish building regulations. Available from: https://historisk.bygningsreglementet.dk/english/0/40.
- CIE system for metrology of optical radiation for ipRGC-influenced responses to light CIE S 026/E; 2018; doi: 10.25039/S026.2018.
- Questionnaires are available from the webpage www.psykiatri-regionh.dk/nid-group.
- Cashman KD, Dowling KG, Škrabáková Z, et al. Vitamin D deficiency in Europe: pandemic? Am J Clin Nutr. 103(4):1033–1044. doi: 10.3945/ajcn.115.120873.
- Institute of Medicine, Food and Nutrition Board. Dietary reference intakes for calcium and vitamin D. Washington, DC: National Academy Press; 2010.
- International Commission on Illumination (CIE). CIE position statement on ultraviolet (UV) radiation to manage the risk of COVID-19 transmission. International Commission on Illumination (CIE); 2020. Available from: http://cie.co.at/publications/cie-position-statement-use-ultraviolet-uv-radiation-manage-risk-covid-19-transmission.
- Masjoudi M, Mohseni M, Bolton JR. Sensitivity of bacteria, protozoa, viruses, and other microorganisms to ultraviolet radiation. J Res Natl Inst Stan. 2021;126:126021. doi: 10.6028/jres.126.021.
- Barnkob LL, Argyraki A, Petersen PM, et al. Investigation of the effect of UV-LED exposure conditions on the production of vitamin D in pig skin. Food Chem. 2016;212:386–391. doi: 10.1016/j.foodchem.2016.05.155.