Abstract
Background. We recently found that direct homotypic cell‐cell contacts between human dermal fibroblasts induce a novel form of cell activation leading to non‐apoptotic programmed cell death. As the major features of this process we identified massive induction of cyclo‐oxygenase‐2 and production of inflammatory prostaglandins. On the surface of the decomposing spheroids, activation of the major extracellular proteolytic cascade, plasminogen activation, associated with surface exposure of α‐enolase, took place.
Aim. To further characterize pericellular proteolysis by cell‐cell contact‐activated fibroblasts we studied the role of the other major extracellular proteolytic system, matrix metalloproteinases (MMPs).
Methods. MMP expression in fibroblast clusters and monolayers was compared using mRNA microarrays and immunoblot analyses. The activities of MMPs were confirmed using MMP inhibitors and caseinolysis.
Results. In microarrays MMP‐1, ‐10, and ‐14 (MT1‐MMP) were induced 5.8‐, 106‐, and 5.6‐fold, respectively. These findings were confirmed by immunoblotting. Radial caseinolysis showed low level of proteolytic activity in spheroid‐conditioned media; ilomastat, a general inhibitor of MMPs, suppressed 50% of the proteolytic activity thus confirming it to be at least in part due to MMPs. A cocktail of tetracycline‐derived MMP inhibitors suppressed lactate dehydrogenase (LDH) release only 11%, and if combined with aprotinin 28%.
Conclusions. Cell‐cell contact activation of fibroblasts induced MMP‐1, ‐10, and MT1‐MMP expression, suggesting similar signaling to that in inflammation and cancer.
Introduction
Regulation of mesenchymal cell responses is an integral part of normal organ function and development, and also plays a significant role in disease processes such as inflammation and cancer. Mesenchymal cells, ubiquitous sentinels, can be activated by different stimuli to evoke various responses within their environment. We recently made the inaugural observation that direct fibroblast cell‐cell contacts induced inflammatory activation leading to non‐apoptotic cell death with characteristic necrotic morphology Citation1. Interestingly, in apoptosis, an extensively studied form of programmed cell death (PCD), no inflammation is provoked and the cells die in immunological anonymity. In contrast, cell death by necrosis is associated with a massive inflammatory reaction Citation2. We showed that an endogenous non‐apoptotic death program can be triggered within mesenchymal cells by homotypic cell‐cell interactions, and that this type of PCD is associated with massive induction of cyclo‐oxygenase‐2 (COX‐2) and production of inflammation‐associated prostaglandins Citation1. This novel type of PCD presented typical features of necrosis, and no activation of molecules related to apoptosis was seen. We found that pericellular proteolysis was associated with increased exposure of α‐enolase on the cell surface. The proteolytic activity was identified as plasminogen activation Citation1.
Matrix metalloproteinases (MMPs) are considered major pericellular proteolytic executors. The genetically distinct but structurally related MMP family members have wide substrate specificities, and whilst dedicated to degradation of the extracellular matrix (ECM) for purposes of development and normal physiology, their activation has been closely associated with tissue destruction in various inflammatory and malignant disease processes as well Citation3–6. Besides ECM components, MMPs are capable of cleaving and processing serpins, complement factors, various receptors and adhesion molecules on cell surfaces, cytokines and growth factors, such as interleukin‐1 and transforming growth factor‐β or their pro‐forms, modifying immune responses Citation6. Certain MMPs can even have anti‐inflammatory effects Citation7,8.
In addition to different levels of transcriptional and translational regulation, the MMPs are inhibited by endogenous inhibitors, tissue inhibitors of MMPs (TIMPs). There are at least 23 MMPs and 4 TIMPs in humans Citation9–11. Collagenases, gelatinases, stromelysins and matrilysins are soluble, secreted MMPs, whereas membrane‐type matrix metalloproteinases (MT‐MMPs) are anchored to the cell membrane either with a transmembrane domain or a glycosylphosphatidylinositol anchor Citation12. TIMP‐2, ‐3, and ‐4 are able to inhibit most MMPs in vitro, while TIMP‐1, the readily inducible member of the family, is a rather poor inhibitor of the membrane‐bound MMPs Citation13,14.
As sentinel cells, fibroblasts are the initial translators of signals to recruit immunocompetent cells to sites of distress Citation15. They play an important regulatory role in developmental processes, wound healing as well as in tumor progression and inflammation Citation15,16. Fibroblasts are known to make direct cell‐cell contacts and even gap junctions in vivo in wound healing Citation17. We recently showed that cultured fibroblasts can be activated and ultimately sacrificed solely by the biological stimulus of direct cell‐cell contacts Citation1. This phenomenon may thus represent a powerful and ubiquitous biological mechanism of mesenchymal cell activation. The present study shows that increased expression of a specific set of MMPs, namely MMP‐1, MMP‐10 and MT1‐MMP, is tightly associated with the process of mesenchymal cell activation by cell‐cell contacts.
Key messages
Direct contacts between fibroblasts activate the cells to a massive proinflammatory, growth factor and proteolytic response and non‐apoptotic programmed cell death.
The proteolytic response, previously shown to involve plasminogen activation, is accompanied by upregulation of MMP‐1 (interstitial collagenase), MMP‐10 (stromelysin‐2) and MMP‐14 (MT1‐MMP, membrane‐type).
These events may be involved in inflammation, wound healing and tumor progression.
Materials and methods
Cell cultures
Monolayers of human dermal fibroblasts from neonatal foreskin were cultured at +37°C in 5% CO2 atmosphere in DMEM/F‐12 (1:1) medium (Gibco, Paisley, Scotland) supplemented with 5% fetal bovine serum (Gibco), 100 µg/mL streptomycin, and 100 U/mL penicillin and were used at passages 5–15. Spheroid formation was initiated as previously described Citation1. In brief, 200‐µL aliquots per well of single cell suspensions (4×104 cells/mL) were plated on agarose‐pretreated U‐bottom 96‐well plates (Costar, Cambridge, MA). The corresponding monolayer cultures were seeded at similar density, in the same medium, on tissue culture‐treated flat bottom 96‐well plates (Costar).
RNA isolation and microarray analysis
Total RNA was extracted from corresponding monolayer and spheroid cultures at 36 hours after seeding by standard methods with Trizol Reagent (Invitrogen, Carlsbad, CA) followed by purification using RNeasy kit according to manufacturer's instructions (QIAGEN, Hilden, Germany). Quality of the RNA was assessed on the Agilent Bioanalyser 2100 (Agilent Technologies, Palo Alto, CA).
Biotinylated cRNAs were prepared from 5 µg of total RNA for hybridization according to the manufacturer's protocol (Affymetrix, Santa Clara, CA). Briefly, RNA was converted to first‐strand cDNA using a T7‐(dT)24 primer (Invitrogen), followed by second‐strand synthesis (Invitrogen). This double‐stranded cDNA was then used as a template for labeled in vitro transcription using biotinylated ribonucleotides (Enzo, Farmingdale, NY). Fifteen µg of each labeled cRNA were chemically fragmented, and were hybridized to Affymetrix HG‐U133A GeneChips (Affymetrix) using standard conditions in an Affymetrix fluidics station. To eliminate false‐positive results, duplicate samples and chips were performed for both monolayers and spheroids.
Data analysis
The arrays were scanned according to the manufacturer's instructions. Obvious artefacts and their outliners on the scanned images were excluded from analysis by Affymetrix® Microarray Suite 5.0 software. The raw expression intensity data were scaled and the mean signal of probe sets on the chip was adjusted to a user‐specified signal value of 500 for each array. Statistical significance of microarray results was tested using Student's t‐test and Bonferroni post testing to exclude false‐positive results using the GeneSpring™ software version 7.2 (Silicon Genetics, Redwood, CA). The Gross‐Gene Error model was active.
Immunoblotting
Equal amounts of cell culture medium (MMP‐1, MMP‐10 and MT1‐MMP) or numbers of spheroids (MT1‐MMP) were collected at indicated time points, washed with Dulbecco's phosphate‐buffered saline (PBS), and the samples were boiled in Laemmli's sample buffer without reducing agents; 200 KIU/mL of aprotinin (Bayer AG) was used to study the effect of plasmin on MMP expression. The incubated samples were separated according to size on an SDS‐polyacrylamide (12%) gel (SDS‐PAGE) and transferred onto polyvinylidene fluoride (PVDF) microporous membrane (Immobilon P, Transfer membrane, Millipore, Billerica, MA). After transfer, non‐specific binding was blocked by incubation with Tris‐buffered saline, pH 7.4 (TBS) supplemented with 5% non‐fat dry milk for 60 min. After washing with TBS‐Tween‐20 (0.05%), the filters were incubated with polyclonal or monoclonal antibodies to MMP‐13 (1 µg/mL) Citation18, to MMP‐1 (1 µg/mL; Oncogene Research Products, Boston, MA), to TIMP‐1 (1 µg/mL; Chemicon International, Temecula, CA), to MMP‐10 (1 µg/mL; R&D Systems, Minneapolis, MN), to MT1‐MMP (1 µg/mL; Biogenesis Ltd, Poole, England, UK), or to MMP‐9 (1 µg/mL, Calbiochem, Merck Biosciences, Darmstadt, Germany) overnight at room temperature and washed with TBS‐Tween‐20. The filters were then incubated with biotinylated anti‐rabbit or anti‐mouse secondary antibodies (1:100, DAKO A/S, Glostrup, Denmark) for 1 h at room temperature. After washing, the filters were incubated with avidin‐peroxidase complex (DAKO A/S) for 45 min. An ECL Western blotting detection kit (Amersham Pharmacia Biotech, Buckinghamshire, UK) was used as described in the product protocol. The chemiluminescence reaction produced was detected by autoradiography. The immunoblots were quantified with an image processing and analysis program (ScionImage PC, Scion Corporation, Frederick, MD) as described previously Citation19. The gradually decreasing content of actin served as a representative control marker of spheroid degradation Citation1.
Spheroid decomposition assay
Lactate dehydrogenase (LDH) release into the culture medium by spheroids was assayed, as described Citation1, at 24, 48, 72 and 96 h time points. Spheroids were incubated with 200 KIU/mL of aprotinin and/or MMP inhibitors, chemically modified tetracyclines (CollaGenex Pharmaceuticals, Inc., Newtown, PA; CMT‐3, CMT‐5 and CMT‐308, 5 μM each, pooled together) and compared to controls.
Immunocapture assay for plasminogen activator activities in cell culture medium
The activities of urokinase‐type (uPA) and tissue‐type plasminogen activators (tPA) were measured in cell culture supernatants using immunocapture by solid‐phase antibodies in microtiter wells, as described in detail earlier Citation20. tPA was assayed by a slightly modified procedure using poly‐D‐lysine in the assay buffer. Polystyrene immunoplates were coated overnight at +37°C with 50 µL of a solution of rabbit IgG antibodies to human uPA (10 µg/mL) or goat IgG antibodies to human tPA (2.5 µg/mL) (cat. # 398 and 387, respectively, American Diagnostica, Greenwich, CT). After rinsing the wells, the samples were absorbed for 2 h at room temperature. To measure the bound activity, 40 µL of purified human plasminogen (100 µg/mL in uPA or tPA assay buffer) was incubated in treated wells and the amount of plasmin generated was determined colorimetrically.
Radial caseinolysis
The proteolytic activity of the conditioned medium of the spheroids was determined by the radial caseinolysis procedure Citation21. Parts of the spheroids were treated with the MMP inhibitor Ilomastat (5 μM; Chemicon International), which was added at the 0 h time point, and incubated in equal conditions as control spheroids. For radial caseinolysis, 10 μL of the unconcentrated conditioned media were added in the wells of opaque agarose gel containing 1% casein and allowed to diffuse. The gel was incubated at +37°C for 72 h in a humidified atmosphere. Lytic zones which developed around the wells indicated proteinase activity. The diameters of the lytic zones were measured.
In situ hybridization (ISH) and immunohistochemistry (IHC)
For the uPA and tPA mRNA in situ hybridization (ISH) experiments, riboprobes were used. cDNA fragments (a 2.3 kb PstI fragment of human tPA and a 1.5 kb of the pHUK‐8 uPA clone) were obtained from American Type Culture Collection, Rockville, MD. The probe sizes were 243 bases for tPA, 240 bases for uPA and were labeled with digoxigenin‐uridine triphosphate by in vitro transcription with SP6 and T7 RNA polymerases according to the manufacturer's instructions (Roche Applied Science, Espoo, Finland). In negative control hybridizations, probes in sense orientation were used. ISH and immunohistochemistry (IHC) were performed using the Ventana Discovery in situ hybridization/immunohistochemistry Slide Stainer (Ventana Medical Systems, Inc., Tucson, AZ). Monoclonal biotinylated anti‐digoxin (Jackson ImmunoResearch Laboratories, Inc., West Grove, PA) was incubated with the sections for 28 min. The probe was detected with Ventana Blue Map kit.
For IHC, tissue sections were incubated with primary antibodies for 32 min. Monoclonal antibodies against tPA and uPA, (cat. # 373 and 3689, respectively; American Diagnostica) were used at 20 μg/mL. A surface antigen on monocytes/macrophages was used as a negative control and stained with a monoclonal antibody against CD 68 (DAKO A/S, Glostrup, Denmark) and was used in 1:50 dilution. The staining was performed with the Ventana 3,3'‐diaminobenzidine tetrahydrochloride (DAB) biotin avidin detection kit.
Results
Microarray data
Ten species of MMPs out of 23 in total in humans were found to be expressed on mRNA level at 36 h after spheroid formation (). As analyzed from duplicate samples and chips, we found a statistically significant and enhanced induction of MMP‐1, MMP‐10, and MT1‐MMP mRNAs in the spheroids compared to corresponding monolayer cultures. MMP‐10 showed the most dramatic increase of 106‐fold on mRNA level. At 36 h no induction or downregulation of TIMP‐1, ‐2, and ‐3 were evident. TIMP‐4 mRNA was absent in all chips. Complete analysis of microarray data will be published elsewhere.
Table I. Expression profile of matrix metalloproteinases (MMPs) and their inhibitors (TIMPs) as analyzed by microarrays.
Immunoreactive MMPs
While there was increased MMP‐1 mRNA expression in spheroids, a steady level of secreted 55‐kDa proMMP‐1 immunoreactivity could be observed in spheroid medium ().
Figure 1. Immunoblots showing expression of(A) MMP‐1; (B) MMP‐10; (C) MT1‐MMP; and (D) TIMP‐1 in cell culture media of cell‐cell activated fibroblasts at the indicated time points. (E) Expression of MT1‐MMP in spheroid lysates at the indicated time points.
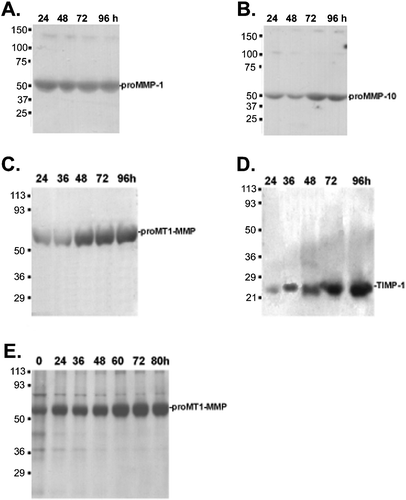
The increased expression of MMP‐10, seen on mRNA level at 36 h, was also seen on protein level as an induction at 72–96 h ().
Constitutive and slightly increasing expression of 70‐kDa proMMP‐14 (proMT1‐MMP) immunoreactivity could be found during 0–80 h experimentation (). The expression of soluble form of MT1‐MMP peaked at 48 h and maintained a steady state after that ().
The 3.16‐fold increased expression of MMP‐9 mRNA was seen as a weak immunoreaction mainly at 92 kDa. Also lower molecular fragments (40–70 kDa) could be observed (results not shown).
Protein expression of TIMP‐1 was cumulatively increasing in cell culture medium throughout the experiment ().
In order to confirm also a negative result on microarrays, we utilized the lack of MMP‐13 mRNA in the 36‐h chips. MMP‐13 protein (45–110 kDa) could be observed only at the beginning (0 h) of experimentation, and no MMP‐13 immunoreactivity could be observed at later time points (results not shown).
Inhibition of plasmin by aprotinin did not affect MMP or TIMP‐1 induction. After 48 h confluent fibroblast monolayers and spheroids secreted/released equal amounts of MMPs, while TIMP‐1 expression was clearly increased in monolayer cultures (results not shown).
Spheroid decomposition
The presence MMP inhibitors (CMT‐3, CMT‐5 and CMT‐308, pooled together) in the cultures of fibroblast spheroids decreased LDH release into the medium only slightly, at the 96‐h time point by 11% and if combined with aprotinin by 28% at the same time point.
Proteolytic activities
Lytic zones in casein‐agarose gel (radial caseinolysis) indicated low level of proteolytic activity in spheroid‐conditioned medium collected at 72 h. The diameter of the lytic zone of spheroid‐conditioned medium without Ilomastat treatment was 0.4 cm and with Ilomastat 0.2 cm (results not shown), which corresponds to at least a 2‐fold decrease in activity.
Plasminogen activator activities were measured using an immunocapture assay. The activity of uPA in spheroid‐conditioned medium was below the lowest standard level and that of tPA at 48‐ and 72‐h time points was at the level of the lowest standard (0.007 IU/mL).
ISH and IHC of plasminogen activators
Positive tPA and uPA mRNA signals were seen at the 24‐h time point, and the signal intensity and the amount of positive cells were somewhat higher at 48‐ and 72‐h time points (). Immunostaining showed pericellular uPA and tPA staining at all time points ().
Figure 2. In situ hybridizations (ISH) of (A) uPA mRNA; (B) tPA mRNA; and (F) a sense, negative control for uPA mRNA, are made with Blue Map staining. (C) uPA immunostaining (IHC); (D) tPA IHC; and (E) CD‐68 IHC (negative control) are made with brown DAB staining. Time point was 72 h. Arrows show some positive signals of ISH. 40× objective was used in microscopy.
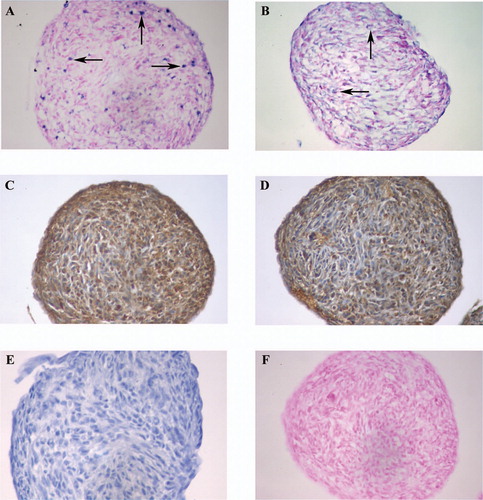
Discussion
We report here the activation of extracellular proteolysis by induction of a specific set of matrix metalloproteinases (MMPs) following homotypic cell‐cell contact activation of cultured human dermal fibroblasts. We previously reported that also induction of the other major extracellular proteolytic pathway, plasminogen activation, largely by cell surface exposure of α‐enolase, takes place in this novel phenomenon characterized by proinflammatory activation and non‐apoptotic cell death Citation1. In the case of fibroblast clusters, we considered the cell activation, enhanced pericellular proteolysis, and production of proinflammatory mediators to reflect their sentinel nature. The role of fibroblasts in alerting and priming the immune system against threatening stimuli is crucial for normal pathophysiology, but a profuse activation of these sentinel cells may also promote tissue destruction and development of disease, such as chronic inflammation and cancer.
As stated above, as one of the characteristic features of cell‐cell contact activation we initially identified production of proinflammatory prostaglandins and activation of proteolysis evidenced by pronounced activation of plasminogen Citation1. In order to clarify the pericellular proteolytic activation, we studied MMPs, the other major extracellular proteolytic enzymes, in addition to plasmin, as identified in microarray analyses. In this study we have identified the members of the MMP family of which expression is activated during this process, which we have designated nemosis Citation22—according to Nemesis, Greek Goddess of Inevitable Consequence—to distinguish it from apoptosis and necrosis.
By using microarray mRNA analyses and comparing the results to those of immunoblotting, we found a characteristic pattern of cell‐cell contact‐induced MMPs, namely MMP‐1, MMP‐10, and MT1‐MMP (MMP‐14), to be upregulated in nemosis. This pattern of MMPs suggests involvement in immune activation, cancer progression, and inflammation Citation23–25. Interestingly, clustered fibroblasts also secrete massive amounts of hepatocyte growth factor/scatter factor which could directly promote tumor invasion Citation22.
Most MMPs are secreted as proteolytically inactive proenzymes and are activated extracellularly by the uPA/plasmin system or by other activated MMPs. The activities of plasminogen activators were marginal in the conditioned medium, but positive mRNA signal and pericellular immunostaining of uPA and tPA in the clustered fibroblasts suggest local proteolytic activation. Inhibition of plasmin with or without MMP inhibitors only delayed the cell decomposition process but did not halt it, indicating these processes as a cascade to be only part of the decomposition mechanisms of the spheroids. Release of LDH after 48 h into the spheroid culture media and a uniformly decreasing actin level from 0 to 96 h Citation1 indicated decomposition of the clustered fibroblasts. Since the levels of MMP expression in immunoblotting were similar in spheroids and in monolayer cultures (data not shown) but fibroblast monolayer had 1.4‐fold more cells at 48 h and more viable cells, the expression/release of MMPs was increased in spheroids.
We determined the activity of MMPs in the conditioned media of spheroids using caseinolysis in the assay. From unconcentrated spheroid‐conditioned media, caseinolytic activity was detectable only after ten days. Although casein is a common substrate for MMPs, MMP‐1 is not optimally detected by caseinolysis, suggesting that all MMP activity was not detectable by this method. Ilomastat is a potent broad‐spectrum inhibitor of MMPs, but it inhibited only 50% of the caseinolytic activity, indicating also other proteinases, such as plasmin Citation1, to be present in the spheroid‐conditioned media.
MMP‐1 or interstitial collagenase, which is important in cleaving ECM proteins, was induced on mRNA level and also protein level according to immunoblotting. Intracellular MMP‐1 was present at the early stages of the process. MMP‐1 expression has been shown to be induced by cytokines, such as interleukin‐1 or tumor necrosis factor‐α Citation5, and to be suppressed by COX‐2‐derived prostaglandins Citation25. In our system it may be that the signals inducing MMP‐1 expression are suppressed by the extensive prostaglandin production at later stages of nemosis.
The role of MMP‐10 (stromelysin‐2) in pathological processes is largely unknown, but it is expressed in cutaneous and intestinal wound healing Citation26. It has been suggested that MMP‐10 is induced mainly by growth factors and cytokines, such as tumor necrosis factor‐α, transforming growth factor‐β, and epidemal growth factor, but not by cell contacts Citation26. However, our results now show that MMP‐10 is intensively upregulated by homotypic cell‐cell interactions, which may represent the ultimate sentinel phenotype of fibroblasts and thus mimic conditions during wound healing, inflammation and tumor progression. Interestingly, of the four stromelysins (MMP‐3, MMP‐10, MMP‐11 and MMP‐19), only MMP‐10 (stromelysin‐2) was induced in nemosis. These enzymes have a broad substrate specificity including proteoglycans, types III, IV, and V collagens, casein, and fibronectin Citation9. They have a highly homologous primary structure and substrate specificity, and are critical for the activation of MMP‐1, MMP‐8, and MMP‐13 Citation12. Expression of the stromelysins has been shown to be differently regulated Citation4, yet our current data now show that mesenchymal cell‐cell contacts elicit signaling can upregulate especially MMP‐10.
The membrane type MT1‐MMP has a wide range of substrates. It is an activator of other metalloproteinases, such as proMMP‐2, proMMP‐13, proMMP‐8, can cleave various ECM components, and its activity is associated with tumor invasiveness Citation27,28. Since MT1‐MMP is membrane‐bound and has an intracellular domain, it may participate in activation of intracellular signaling pathways Citation28, and in cleavage and/or activation of membrane‐associated receptors, cytokines, or growth factors. It has been shown that fibroblasts can produce a soluble form of MT1‐MMP Citation29,30, and its soluble levels can be upregulated during inflammatory conditions Citation31. The activity of MT1‐MMP is associated with tumor invasiveness.
An integral part of the regulation of MMP activity is the production of their endogenous inhibitors, TIMPs. While secreted TIMP‐1 accumulated in the medium, no upregulation of TIMP mRNAs was seen inactivated fibroblasts.
The involvement of MMPs in cell‐cell contact‐activated fibroblasts warrants further study as their expression and activity are interconnected with other known processes in activated fibroblasts, such as production of inflammatory prostaglandins by COX‐2 and plasminogen activation. Inflammation, proteolytic activation and excess degradation of the ECM are associated with various disease processes, such as myocardial infarction, atherosclerosis, thrombosis, leg ulcers and periodontal diseases Citation32–35. MMPs are closely associated with angiogenesis and tumor invasiveness Citation5,Citation11,Citation36. The role of stromal fibroblasts as ubiquitous sentinel cells, which can trigger or amplify different stimuli, makes them active participants in these events.
Acknowledgements
We thank Irina Suomalainen for expert technical assistance. This work was supported by grants from the Biomedicum Helsinki Foundation, Finnish Cancer Societies, Finnish Medical Foundation, Academy of Finland, Helsinki University Central Hospital EVO Grants (TYH 4258, TYH 5306, TI020Y0002), Helsinki University Research Funds, Slovak Grant Agency (VEGA 2/3022), and the Wilhelm and Else Stockmann Foundation.
References
- Bizik J., Kankuri E., Ristimäki A., Taieb A., Vapaatalo H., Lubitz W., et al. Cell‐cell contacts trigger programmed necrosis and induce cyclooxygenase‐2 expression. Cell Death Differ 2004; 11: 183–95
- Arends M. J., Wyllie A. H. Apoptosis: mechanisms and roles in pathology. Int Rev Exp Pathol 1991; 32: 223–54
- Vu T. H., Werb Z. Matrix metalloproteinases: effectors of development and normal physiology. Genes Dev 2000; 14: 2123–33
- Daniels J. T., Geerling G., Alexander R. A., Murphy G., Khaw P. T., Saarialho‐Kere U. Temporal and spatial expression of matrix metalloproteinases during wound healing of human corneal tissue. Exp Eye Res 2003; 77: 653–64
- Chang C., Werb Z. The many faces of metalloproteases: cell growth, invasion, angiogenesis and metastasis. Trends Cell Biol 2001; 11: S37–43
- McCawley L. J., Matrisian L. M. Matrix metalloproteinases: they're not just for matrix anymore!. Curr Opin Cell Biol 2001; 13: 534–40
- Owen C. A., Hu Z., Lopez‐Otin C., Shapiro S. D. Membrane‐bound matrix metalloproteinase‐8 on activated polymorphonuclear cells is a potent, tissue inhibitor of metalloproteinase‐resistant collagenase and serpinase. J Immunol 2004; 172: 7791–803
- Gueders M. M., Balbin M., Rocks N., Foidart J. M., Gosset P., Louis R., et al. Matrix metalloproteinase‐8 deficiency promotes granulocytic allergen‐induced airway inflammation. J Immunol 2005; 175: 2589–97
- Nagase H., Woessner J. F., Jr. Matrix metalloproteinases. J Biol Chem 1999; 274: 21491–4
- Sternlicht M. D., Werb Z. How matrix metalloproteinases regulate cell behavior. Annu Rev Cell Dev Biol 2001; 17: 463–516
- Egeblad M., Werb Z. New functions for the matrix metalloproteinases in cancer progression. Nat Rev Cancer 2002; 2: 161–74
- Visse R., Nagase H. Matrix metalloproteinases and tissue inhibitors of metalloproteinases: structure, function, and biochemistry. Circ Res 2003; 92: 827–39
- Baker A. H., Edwards D. R., Murphy G. Metalloproteinase inhibitors: biological actions and therapeutic opportunities. J Cell Sci 2002; 115: 3719–27
- Will H., Atkinson S. J., Butler G. S., Smith B., Murphy G. The soluble catalytic domain of membrane type 1 matrix metalloproteinase cleaves the propeptide of progelatinase A and initiates autoproteolytic activation. Regulation by TIMP‐2 and TIMP‐3. J Biol Chem 1996; 271: 17119–23
- Smith R. S., Smith T. J., Blieden T. M., Phipps R. P. Fibroblasts as sentinel cells. Synthesis of chemokines and regulation of inflammation. Am J Pathol 1997; 151: 317–22
- Silzle T., Randolph G. J., Kreutz M., Kunz‐Schughart L. A. The fibroblast: sentinel cell and local immune modulator in tumor tissue. Int J Cancer 2004; 108: 173–80
- Gabbiani G., Chaponnier C., Huttner I. Cytoplasmic filaments and gap junctions in epithelial cells and myofibroblasts during wound healing. J Cell Biol 1978; 76: 561–8
- Lindy O., Konttinen Y. T., Sorsa T., Ding Y., Santavirta S., Ceponia A., et al. Matrix metalloproteinase 13 (collagenase 3) in human rheumatoid synovium. Arthritis Rheum 1997; 40: 1391–9
- Prikk K., Maisi P., Pirilä E., Reintam M. A., Salo T., Sorsa T., et al. Airway obstruction correlates with collagenase‐2 (MMP‐8) expression and activation in bronchial asthma. Lab Invest 2002; 82: 1535–45
- Stephens R. W., Leung K. ‐. C., Pöllänen J., Salonen E. ‐. M., Vaheri A. Microplate immunoassay for plasminogen activators and their specific inhibitors. J Immunol Methods 1987; 105: 245–51
- Saksela O. Radial caseinolysis in agarose: A simple method for detection of plasminogen activator in the presence of inhibitory substances and serum. Anal Biochem 1981; 111: 276–82
- Kankuri E., Cholujova D., Comajova M., Vaheri A., Bizik J. Induction of hepatocyte growth factor/scatter factor by fibroblast clustering directly promotes tumor cell invasiveness. Cancer Res 2005; 65: 9914–22
- Knäuper V., Murphy G., Tschesche H. Activation of human neutrophil procollagenase by stromelysin 2. Eur J Biochem 1996; 235: 187–91
- Seiki M. Membrane‐type 1 matrix metalloproteinase: a key enzyme for tumor invasion. Cancer Lett 2003; 194: 1–11
- Pillinger M. H., Rosenthal P. B., Tolani S. N., Apsel P., Dinsell V., Greenberg J., et al. Cyclooxygenase‐2‐derived E prostaglandins down‐regulate matrix metalloproteinase‐1 expression in fibroblast‐like synoviocytes via inhibition of extracellular signal‐regulated kinase activation. J Immunol 2003; 171: 6080–9
- Rechardt O., Elomaa O., Vaalamo M., Paakkonen K., Jahkola T., Hook‐Nikanne J., et al. Stromelysin‐2 is upregulated during normal wound repair and is induced by cytokines. J Invest Dermatol 2000; 115: 778–87
- Holopainen J. M., Moilanen J. A., Sorsa T., Kivelä‐Rajamäki M., Tervahartiala T., Vesaluoma M. H., et al. Activation of matrix metalloproteinase‐8 by membrane type 1‐MMP and their expression in human tears after photorefractive keratectomy. Invest Ophthalmol Vis Sci 2003; 44: 2550–6
- Gingras D., Bousquet‐Gagnon N., Langlois S., Lachambre M. P., Annabi B., Beliveau R. Activation of the extracellular signal‐regulated protein kinase (ERK) cascade by membrane‐type‐1 matrix metalloproteinase (MT1‐MMP). FEBS Lett 2001; 507: 231–6
- Li H., Bauzon D. E., Xu X., Tschesche H., Cao J., Sang Q. A. Immunological characterization of cell‐surface and soluble forms of membrane type 1 matrix metalloproteinase in human breast cancer cells and in fibroblasts. Mol Carcinogen 1998; 56: 84–94
- Tervahartiala T., Pirilä E., Ceponis A., Maisi P., Salo T., Tuter G., et al. The in vivo expression of the collagenolytic matrix metalloproteinases (MMP‐2, ‐8, ‐13, and ‐14) and matrilysin (MMP‐7) in adult and localized periodontitis. J Dent Res 2000; 79: 1969–77
- Maisi P., Prikk K., Sepper R., Pirilä E., Salo T., Hietanen J., et al. Soluble membrane‐type 1 matrix metalloproteinase (MT1‐MMP) and gelatinase A (MMP‐2) in induced sputum and bronchoalveolar lavage fluid of human bronchial asthma and bronchiectasis. APMIS 2002; 110: 771–82
- Lindsey M. L. MMP induction and inhibition in myocardial infarction. Heart Fail Rev 2004; 9: 7–19
- Palolahti M., Lauharanta J., Stephens R. W., Kuusela P., Vaheri A. Proteolytic activity in leg ulcer exudates. Exp Dermatol 1993; 2: 29–37
- Shah P. K. Role of inflammation and metalloproteinases in plaque disruption and thrombosis. Vasc Med 1998; 3: 199–206
- Sirén V., Kauhanen P., Carpén O., Luther M., Lepäntalo M., Vaheri A., et al. Urokinase, tissue‐type plasminogen activator and plasminogen activator inhibitor‐1 expression in severely stenosed and occluded vein grafts with thrombosis. Blood Coagul Fibrin 2003; 14: 369–77
- Pagano M., Benmaamar R. When protein destruction runs amok, malignancy is on the loose. Cancer Cell 2003; 4: 251–6