Abstract
BACKGROUND. Alterations in the growth hormone (GH)/insulin‐like growth factor I (IGF‐I) axis are associated with increased cardiovascular morbidity and mortality, but previous studies have yielded conflicting results. In addition, the T1169A polymorphism in the GH1 gene has been associated with IGF‐I levels.
AIMS. To investigate whether IGF‐I concentrations and the T1169A polymorphism of the GH1 gene are associated with cardiovascular risk factors and the intima media thickness (IMT) of the carotid artery.
METHODS. Fasting plasma IGF‐I concentrations (n = 1008) were measured in a large population‐based OPERA (Oulu Project Elucidating Risk of Atherosclerosis) cohort. Genotype variants were determined by the restriction fragment length polymorphism method.
RESULTS. Low IGF‐I concentrations associated with several cardiovascular risk factors including age, adiposity, and high triglyceride, fasting insulin and C‐reactive protein concentrations in the analysis of all subjects. In the multivariate models, however, IGF‐I concentrations were positively associated with the mean IMT of women (ß = 0.127, P = 0.009) whereas the association in men was weaker and negative (ß = −0.088, P = 0.034). The 1169A allele was associated with low low‐density lipoprotein cholesterol in both sexes and with low systolic blood pressure levels in women.
CONCLUSIONS. IGF‐I concentrations were associated with several traditional cardiovascular risk factors. The observed gender difference in the association between IGF‐I concentrations and carotid artery atherosclerosis warrants further study. The GH1 1169A allele may be associated with a favourable metabolic profile.
Introduction
Insulin‐like growth factor I (IGF‐I) is a ubiquitous peptide that has both growth hormone‐like and insulin‐like effects. The principal source of circulating IGF‐I is the liver, where its expression is regulated mainly by growth hormone (GH). Measurement of circulating IGF‐I is therefore considered a convenient indicator of GH secretion. IGF‐I, in turn, regulates the secretion of GH from the pituitary by means of negative feedback control Citation1. IGF‐binding proteins (IGFBP) regulate the bioavailability and activity of IGF‐I Citation2. Of the six characterized mammalian IGFBPs, only IGFBP‐1 is acutely regulated by metabolic status, and it can rapidly and dynamically modulate the metabolic and mitogenic effects of free IGF‐I Citation3.
Alterations in the GH/IGF‐I axis are associated with cardiovascular disease (CVD). GH hypersecretion as well as GH deficiency are characterized by an increased prevalence of CVD Citation4. It is therefore not surprising that the studies concerning the role of IGF‐I in the development of CVD have also yielded conflicting results. Low IGF‐I levels have been associated with an increased incidence of ischaemic heart disease (IHD) Citation5 and IHD mortality Citation6, but IGF‐I levels have also been shown to correlate positively with coronary artery disease progression Citation7. Obviously, further studies are needed to elucidate the role of IGF‐I in the development of CVD.
The aim of the present study was to investigate whether plasma IGF‐I concentrations are associated with carotid artery atherosclerosis and the risk factors of CVD in a large population‐based cohort. We also genotyped the GH1 T1169A polymorphism which has been shown to be associated with GH secretion and IGF‐I levels Citation8, Citation9, to explore whether this genetic variant is associated with cardiovascular risk factors.
Key messages
Insulin‐like growth factor I (IGF‐I) concentrations were associated with several traditional cardiovascular disease risk factors in our population‐based cohort.
According to our results, the effect of IGF‐I on intima‐media thickness appears to be significantly different in men and women.
Subjects and methods
Study population
Oulu Project Elucidating Risk of Atherosclerosis (OPERA) is a population‐based, epidemiological study addressing the risk factors and disease end‐points of atherosclerotic cardiovascular disease. The study population consists of middle‐aged (40–59 years) drug‐treated hypertensive subjects selected randomly by age stratification from the Finnish Social Insurance Institute's register, and age‐ and sex‐matched control subjects drawn from the above‐mentioned register and representing a random sample of the population of Oulu. The study group and the measurements and laboratory analyses performed have been described in detail previously Citation10. In the present study, a total of 1008 participants were screened and 1003 were successfully genotyped. All the subjects volunteered into this study, which was approved by the Ethical Committee of the University of Oulu. The study was conducted according to the principles of the Declaration of Helsinki.
Laboratory assays
Blood glucose concentrations were determined with the glucose dehydrogenase method (Diagnostica, Merck, Darmstadt, Germany) and plasma insulin concentration with the double radioimmunoassay method (AIA‐PACK IRI Tosoh Corp., Tokyo, Japan). Insulin sensitivity was assessed by the quick index (1 / [ log(fasting insulin) + log(fasting glucose)]) Citation11. Very low density lipoprotein (VLDL), high density lipoprotein (HDL), low density lipoprotein (LDL) and total cholesterol and triglyceride (TG) concentrations were determined from plasma by ultracentrifugation and enzymatic colorimetric methods using commercial kits (Boehringer Diagnostica, Mannheim GmbH, Germany). Fasting plasma total IGF‐I concentration was determined using a commercial kit which uses a modified version of the standard acid‐ethanol extraction procedure (DSL‐10‐2800 ACTIVE Non‐Extraction IGF‐I ELISA, Diagnostic Systems Laboratories, Webster, Texas, U S A) with intra‐ and interassay coefficients of variation (CV) of 4.5%–8.6 % and 3.3%–6.8 %, respectively. IGFBP‐1 was measured using a commercial kit (IGFBP‐1 IEMA test, Oy Medix Biochemica, Kauniainen, Finland) with intra‐ and interassay CVs of 2.4%–3.4 % and 4.9%–7.4 %, respectively. C‐reactive protein (CRP) was determined using a commercially available enzyme‐linked immunoassay kit (Diagnostic Systems Laboratories, Webster, Texas, U S A).
Carotid ultrasonography
Carotid ultrasonography was performed by a single trained radiologist. The intima‐media thickness (IMT) and the size and number of atheromatous plaques were measured using a duplex ultrasound system with 7.5 MHz scanning frequency in B‐mode, pulsed Doppler mode and colour mode (Toshiba SSA‐270A, Toshiba Corp., Tokyo, Japan). The ultrasonography methods have been described in detail previously Citation12.
Genotyping
Genomic DNA had been extracted from leucocytes. The GH1 T1169A polymorphism was detected using the restriction fragment length polymorphism method. The methods for genotyping were modified based on a previous article Citation8. Since the results obtained were not always totally unambiguous, they were confirmed by sequencing using the DYEnamicTM ET Terminator Cycle Sequencing Kit (Amersham Biosciences, Buckingshamshire, UK) on an ABI Prism 377 sequencer (Pel‐Freez Clinical Systems, LLC®). The following primers were used in sequencing: (forward) 5'‐AGGAGTGTCTTCGCCAACAG‐3', (reverse) 5'‐GCGTCATCGTTGTGTGAGTT‐3'. The sequences were analysed using the Chromas 2.23 program (Technelysium Pty Ltd).
Statistical methods
All the statistical analyses were done using SPSS version 11.0 (© SPSS Inc.). The associations were tested by analysis of variance (ANOVA) and by analysis of covariance (ANCOVA) with age, sex and body mass index (BMI) as covariates. If any statistically significant interaction was observed between the IGF‐I tertile and sex, the IGF‐I tertile and study group, or the IGF‐I tertile and menopausal status, the analyses were performed separately in the subgroups. The association between IGF‐I concentrations and blood pressure was tested only in the control group. The correlations between cardiovascular risk factors and IGF‐I were explored by partial correlation analysis controlled for study group. To find out the independent determinants of mean IMT, linear regression analyses with a stepwise selection method were performed. The following variables were included in the model: age, sex, BMI, smoking, systolic blood pressure, LDL cholesterol, IGF‐I, triglycerides, CRP, IGFBP‐1 and type 2 diabetes.
The associations between the GH1 genotype and cardiovascular risk factors were analysed by the chi‐square test and logistic regression (categorical variables) and ANCOVA (continuous variables) using age, sex and BMI as covariates. If any interaction between the genotype and sex, or the genotype and study group was observed, the subgroups were analysed separately. The association between the genotype and blood pressure values was tested only in the control group.
Results
The basic characteristics in the hypertensive and control cohorts are shown in .
Table I. Basic characteristics in the hypertensive and control cohorts.
IGF‐I and cardiovascular risk factors
The associations between cardiovascular risk factors and IGF‐I concentrations were analysed by correlation analysis (), ANOVA and ANCOVA (). In the ANOVA of all subjects, the IGF‐I concentrations were negatively associated with age (P<0.001), BMI (P<0.001), waist‐to‐hip ratio (WHR) (P<0.001), fasting insulin (P = 0.001), IGFBP‐1 (P<0.001), triglycerides (P<0.001), CRP (P<0.001) and mean IMT (P = 0.009). In ANCOVA, adjusted for age, sex and BMI when relevant, the associations remained significant between IGF‐I concentrations and age (P<0.001), BMI (P<0.001), fasting insulin (P = 0.003), IGFBP‐1 (P<0.001), triglycerides (P = 0.019) and CRP (P<0.001). The association became significant between the IGF‐I tertile and HDL cholesterol (P = 0.003) when adjusted for age, sex and BMI, but the relationship between the IGF‐I tertile and the mean HDL cholesterol concentration was not linear.
Table II. Associations between IGF‐I tertile and CVD risk factors.
Figure 1 Correlations between IGF‐I concentrations and cardiovascular risk factors in men and women. The values were obtained by partial correlation analysis controlled for study group.
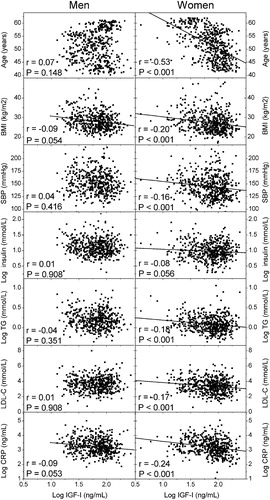
Since there were statistically significant interactions between sex and IGF‐I as a predictor of some of the cardiovascular risk factors (age, total cholesterol, LDL cholesterol, HDL cholesterol, WHR), the analyses were further stratified by sex. The statistically significant correlations between IGF‐I concentrations and cardiovascular risk factors in men and women are shown in . The values in were obtained by partial correlation analysis controlled for study group. A negative correlation between IGF‐I concentrations and several cardiovascular risk factors was observed in women, but the correlations were not statistically significant in men. After further adjustment for the effect of age, a negative correlation was observed between IGF‐I concentration and BMI (r = −0.09, P = 0.042 and r = −0.17, P<0.001, for men and women, respectively) and CRP (r = −0.10, P = 0.036 and r = −0.15, P = 0.001, for men and women, respectively). The analyses in women were performed also after exclusion of the three subjects with exceptionally low IGF‐I values (>3 SD below the mean), but this did not alter the results significantly (data not shown).
IGF‐I and carotid IMT
The relationship between IGF‐I concentrations and carotid IMT was assessed using ANCOVA and linear regression analyses. An interaction was observed between sex and the IGF‐I tertile as a predictor of mean IMT (P<0.05), and the analyses were therefore performed separately for both sexes. The relationships between IGF‐I concentrations and mean IMT in men and women are shown in . In ANCOVA adjusted for age, IGF‐I concentrations were positively associated with mean IMT in women (P = 0.010), but the association was not statistically significant in men (P = 0.144). After further adjustments for the commonly recognized risk factors of atherosclerosis (age, BMI, systolic blood pressure, LDL cholesterol and pack‐years), the positive association between mean IMT and IGF‐I concentrations in women persisted (P = 0.031) whereas no statistically significant association was observed in men ().
Figure 2 The relationships between IGF‐I concentrations and mean IMT in men and women. a) Age‐adjusted IMT values in sex‐specific IGF‐I tertiles. P‐value for IGF‐I obtained by ANCOVA adjusted for age, b) IMT values after further adjustments. P‐value for IGF‐I obtained by ANCOVA adjusted for age, BMI, systolic blood pressure, LDL cholesterol and pack‐years.
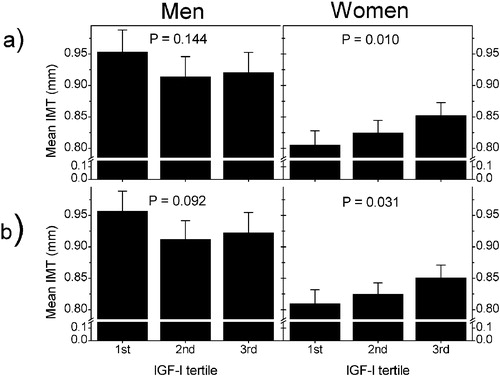
The following factors were included in the linear regression analysis: age, sex, BMI, pack‐years, systolic blood pressure, LDL cholesterol, IGF‐I, IGFBP‐1, triglycerides, CRP, fasting insulin and type 2 diabetes. The statistically significant determinants of mean IMT in the whole cohort were sex, age, BMI, LDL cholesterol, systolic blood pressure and pack‐years (). When men and women were analysed separately, IGF‐I was positively associated with mean IMT in women (P = 0.009), whereas the association was negative in men (P = 0.034).
Table III. Linear regression models explaining the variation in mean carotid IMT.
In order to explore whether menopausal status affects the observed association between IGF‐I concentrations and carotid IMT, the women were classified as pre‐ or postmenopausal. Women with at least one year of amenorrhea or surgical removal of both ovaries were classified as postmenopausal. Of the women, 44% were premenopausal and 51% were postmenopausal. Menopausal status could not be defined for 5% of the women. No interaction was observed between the IGF‐I tertile and menopause as a predictor of carotid IMT (ANCOVA, P>0.05). When menopause was included in the multivariate linear regression analysis, IGF‐I remained a statistically significant determinant of mean carotid IMT (β = 0.127, P = 0.009) along with age (β = 0.363, P = 0.000), systolic blood pressure (β = 0.216, P = 0.000), BMI (β = −0.097, P = 0.024) and pack‐years (β = 0.084, P = 0.039). Furthermore, hormone replacement therapy (estrogen and/or progestin) had no statistically significant influence on the association between IGF‐I and carotid IMT in either pre‐ or postmenopausal women (multivariate ANCOVA, P>0.05 for interaction in both groups).
Association analysis of the GH1 T1169A polymorphism
The genotype frequencies were in Hardy‐Weinberg equilibrium and did not differ statistically significantly between the study groups or genders. Altogether 159 (15.9 %) individuals had the A/A genotype, while 446 (44.5 %) the A/T genotype and 398 (39.6%) the T/T genotype. The association between the polymorphism and cardiovascular risk factors was analysed by ANCOVA adjusted for age, sex and BMI. The results are shown in . The GH1 genotype was associated with systolic blood pressure in women (P = 0.003), with the A/A genotype having the lowest and the T/T genotype the highest mean values. An association was also observed between the genotype and LDL cholesterol (P = 0.005) in the whole cohort with the A/A genotype having the lowest mean value and no statistical difference in the mean values between the A/T and T/T genotypes. The 1169A allele generally seemed to be associated with a more beneficial spectrum in cardiovascular risk factors, although only the above‐mentioned associations were statistically significant. We found no statistically significant association between the T1169A polymorphism and carotid IMT, even though the mean IMT values tended to be lowest in the subjects homozygous for the 1169A allele.
Table IV. Basic characteristics and risk factors of CVD with statistical significance by the GH1 T1169A genotypes.
Discussion
The aim of our study was to shed light on the association between IGF‐I concentrations, cardiovascular risk factors and atherosclerotic alterations measured as the carotid IMT. Our results showed that the effect of IGF‐I on IMT appears to be significantly different in men and women, possibly due to hormonal modulation. Our results confirmed the findings of several previous studies showing that IGF‐I affects many of the traditional CVD risk factors.
This study supports the view put forward by many previous studies that IGF‐I may have pro‐atherogenic effects. On the other hand, IGF‐I concentrations were inversely associated with several cardiovascular risk factors, as has also been shown earlier, suggesting that low IGF‐I concentrations may be a risk marker for the development of cardiovascular disease. These results indicate that the role of IGF‐I in the development of atherosclerosis and other cardiovascular disease is more or less unclear and needs further study. It could well be that IGF‐I has both pro‐ and anti‐atherogenic effects, depending on other risk factors, the modifying effect of other growth factors and hormones and on the stage of disease development.
IGF‐I levels were positively and independently associated with mean IMT in women in all of our statistical analyses. On the contrary, men showed a negative, even though relatively weak association between IGF‐I concentrations and mean IMT. Obviously, these gender differences may be one of the factors that could explain the discrepancies between the effects of IGF‐I on IMT in various populations. To our knowledge, however, there are no studies concerning the sex‐specific effects of IGF‐I on atherosclerotic processes, and this issue should therefore be studied further. In our study, IGF‐I concentrations were positively associated with mean IMT in women despite the general trend towards a negative association between IGF‐I concentrations and the traditional cardiovascular risk factors, which indicates that IGF‐I may indeed have sex‐specific effects on atherosclerotic processes independent of the traditional CVD risk factors. However, our analyses suggest that estrogen and/or progestin may not be the principal hormones modulating the effects of IGF‐I, since a positive association between IGF‐I and carotid IMT was observed in the postmenopausal group not using exogenous hormones. One explanation for the observed sex discrepancy in the present study could be that in women the level of atherosclerosis and its risk factors are generally smaller compared to men. Weaker risk factors, such as IGF‐I levels, may be apparent more easily when the overall risk of the disease is smaller.
There are several potential mechanisms by which IGF‐I could modify atherosclerotic processes either locally or in a systemic manner Citation13. The non‐beneficial pro‐atherogenic effects of IGF‐I have mainly been described in relation to the function of vascular smooth muscle cells (VSMCs). IGF‐I is able to stimulate VSMC proliferation and migration in vitroCitation14–16. Ruotolo et al. showed that serum IGF‐I levels were positively associated with the progression of premature coronary artery disease and suggested that this might, at least partly, be caused by the effects of IGF‐I on the proliferation and migration of VSMCs Citation7.
A positive association between carotid IMT and high IGF‐I levels has also been observed in acromegalic patients Citation17. It has not yet been clarified how the excess of GH/IGF‐I induces IMT thickening, but the process could partly be attributed to the direct effects of IGF‐I on VSMCs Citation18. In addition, acromegalic patients show both impaired endothelium‐dependent vasodilatation and an exaggerated vasoconstrictor response mediated by the sympathetic nervous system Citation19. It is known that elevated blood pressure and hypertension may induce increased IMT Citation20, and this could be one reason for the increased IMT in acromegaly.
The majority of studies have reported low IGF‐I concentrations to have pro‐atherogenic effects Citation21, Citation22. GH deficiency and associated IGF‐I deficiency may lead to increased cardiovascular morbidity and mortality Citation23–25 as well as increased IMT Citation26, Citation27. This could be related to the direct effects of GH and IGF‐I on vascular cells, or to metabolic disturbances associated with GH/IGF‐I deficiency, such as obesity, hyperlipidaemia, and insulin resistance Citation4. IGF‐I levels were negatively associated with BMI and WHR in the present study. GH secretion is impaired in obese patients, probably mostly due to their elevated fatty acid levels, which have an inhibitory effect on somatotroph secretion Citation28. Since GH is the main regulator of IGF‐I secretion from the liver, it seems reasonable that IGF‐I levels would also be reduced in obesity. Because of the complex interplay between different endocrine and metabolic factors on the GH‐IGF‐I axis, however, the outcome is not so straightforward, since total IGF‐I levels have been reported to be decreased, normal or even increased in obesity Citation29–31. However, it has also been shown that low IGF‐I concentrations are associated with abdominal obesity Citation32, which is in accordance with our results. Triglycerides, which are often associated with abdominal obesity, were also negatively associated with IGF‐I levels in our study. Administration of recombinant IGF‐I to humans has been reported to cause a decrease in triglyceride levels Citation33.
Insulin resistance in type 2 diabetes has been shown to correlate with IMT Citation34, Citation35. The highest fasting insulin concentrations were associated with the lowest IGF‐I tertile in our study. IGF‐I suppresses insulin secretion in humans and has insulin‐like, hypoglycaemic effects Citation36. IGF‐I administration to humans can improve insulin sensitivity Citation37 and hepatic IGF‐I deletion has led to insulin resistance in mice Citation38. By improving insulin sensitivity, IGF‐I may reduce the risk of cardiovascular disease Citation39.
Inflammation plays a central role in the pathogenesis of atherosclerosis. CRP is an important marker of inflammation and is considered a risk marker for cardiovascular disease Citation40. In our study, IGF‐I concentration was inversely correlated with CRP, which has also been reported earlier Citation41, Citation42. It is likely that the GH/IGF‐I axis acts both directly and indirectly on the immune system. GH and IGF‐I receptors are expressed on immune cells and these hormones have various effects on the immune system Citation43. The GH/IGF‐I axis may also influence the immune system indirectly, through effects on body composition. Compared with BMI‐matched controls, GH‐deficient patients have increased fat mass Citation44. Adipose tissue is a major source of interleukin‐6 Citation45, which, in turn, controls the hepatic synthesis and secretion of CRP Citation40.
In previous studies, the GH1 T1169A polymorphism has been associated with GH secretion and IGF‐I concentration. It has been shown that the A/A genotype had significantly lower GH peak and IGF‐I levels and higher IGFBP‐1 values compared to the T/T genotype Citation8, Citation9. In this study, we did not observe any association between the T1169A genotype and the IGF‐I concentration. An association between the GH1 T1169A genotype and systolic blood pressure was observed in women. Significantly higher systolic blood pressure but no difference in diastolic blood pressure in a GH‐deficient group compared to a control group has been reported Citation46. In severe GH deficiency, endothelial dysfunction has been observed Citation47, and endothelium‐independent vasodilatation was also shown to be reduced in non‐treated GH‐deficient patients Citation26. Thus, endothelial dysfunction and the possible reduced output of GH might partly explain the association observed in the present study, although GH levels were not measured. The polymorphism might also be in linkage disequilibrium with another gene affecting blood pressure. For example, a linkage between SLC4A1 and GH1 has been demonstrated in mammals and birds Citation48, and SLC4A1 has been presented as a potential candidate gene for pseudohypoaldosteronism II (OMIM #145260), a disease characterized by hypertension Citation49.
GH deficiency is associated with lipid abnormalities, including low HDL cholesterol and high LDL cholesterol and triglyceride concentrations Citation46, Citation50, Citation51. We observed an association between the T1169A genotype and LDL cholesterol. It has been shown that GH has a major role in hepatic cholesterol metabolism. GH administration increases the clearance of LDL, probably by up‐regulating hepatic LDL receptors Citation52. The effect of GH on LDL receptors is not likely to be mediated by IGF‐I Citation53.
The study population in the present study is not a random population sample, since the OPERA cohort is comprised of middle‐aged, drug‐treated hypertensive subjects and age‐ and sex‐matched control subjects. The control cohort does not either represent the normotensive part of the population, mostly due to the reimbursement criteria of the Finnish Social Insurance Institution, but the main characteristics of the control cohort are well comparable to the general Finnish population. These facts were taken into account in the statistical analyses, and the principal findings of this study remained substantially unchanged if only the control group was taken into account.
It should also be noted that this study is a cross‐sectional one, and thus no conclusions about the possible causes and outcomes can be made based on these results. However, results from cross‐sectional studies can generate new hypotheses that can be further tested in prospective studies, and ultimately, true causality can only be demonstrated by extensive clinical studies.
In conclusion, IGF‐I concentrations were negatively associated with several traditional cardiovascular risk factors in the present study. Furthermore, IGF‐I levels were strongly and positively associated with IMT in women but not in men. The GH 1169A allele was associated with a favourable cardiovascular risk profile, but there was no association between the polymorphism and IGF‐I levels. Our data emphasize the role of gender when studying the interaction between IGF‐I and IMT.
Acknowledgements
The authors thank Ms Heidi Häikiö, Ms Helena Kalliokoski, Ms Saija Kortetjärvi and Ms Riitta Vanhanen for skilful technical assistance. This study was supported by the Medical Council of the Academy of Finland and the Finnish Foundation for Cardiovascular Research.
References
- Berelowitz M., Szabo M., Frohman L. A., Firestone S., Chu L., Hintz R. L. Somatomedin‐C mediates growth hormone negative feedback by effects on both the hypothalamus and the pituitary. Science 1981; 212: 1279–81
- Hwa V., Oh Y., Rosenfeld R. G. The insulin‐like growth factor‐binding protein (IGFBP) superfamily. Endocr Rev 1999; 20: 761–87
- Lee P. D., Conover C. A., Powell D. R. Regulation and function of insulin‐like growth factor‐binding protein‐1. Proc Soc Exp Biol Med 1993; 204: 4–29
- Lombardi G., Colao A., Marzullo P., Ferone D., Longobardi S., Esposito V., et al. Is growth hormone bad for your heart? Cardiovascular impact of GH deficiency and of acromegaly. J Endocrinol 1997; 155(Suppl 1)33–7
- Juul A., Scheike T., Davidsen M., Gyllenborg J., Jorgensen T. Low serum insulin‐like growth factor I is associated with increased risk of ischemic heart disease: a population‐based case‐control study. Circulation 2002; 939–44
- Laughlin G. A., Barrett‐Connor E., Criqui M. H., Kritz‐Silverstein D. The prospective association of serum insulin‐like growth factor I (IGF‐I) and IGF‐binding protein‐1 levels with all cause and cardiovascular disease mortality in older adults: the Rancho Bernardo Study. J Clin Endocrinol Metab 2004; 89: 114–20
- Ruotolo G., Bavenholm P., Brismar K., Efendic S., Ericsson C. G., de Faire U., et al. Serum insulin‐like growth factor‐I level is independently associated with coronary artery disease progression in young male survivors of myocardial infarction: beneficial effects of bezafibrate treatment. J Am Coll Cardiol 2000; 35: 647–54
- Le Marchand L., Donlon T., Seifried A., Kaaks R., Rinaldi S., Wilkens L. R. Association of a common polymorphism in the human GH1 gene with colorectal neoplasia. J Natl Cancer Inst 2002; 94: 454–60
- Hasegawa Y., Fujii K., Yamada M., Igarashi Y., Tachibana K., Tanaka T., et al. Identification of novel human GH‐1 gene polymorphisms that are associated with growth hormone secretion and height. J Clin Endocrinol Metab 2000; 85: 1290–5
- Rantala A. O., Kauma H., Lilja M., Savolainen M. J., Reunanen A., Kesaniemi Y. A. Prevalence of the metabolic syndrome in drug‐treated hypertensive patients and control subjects. J Intern Med 1999; 245: 163–74
- Katz A., Nambi S. S., Mather K., Baron A. D., Follmann D. A., Sullivan G., et al. Quantitative insulin sensitivity check index: a simple, accurate method for assessing insulin sensitivity in humans. J Clin Endocrinol Metab 2000; 85: 2402–10
- Paivansalo M., Rantala A., Kauma H., Lilja M., Reunanen A., Savolainen M., et al. Prevalence of carotid atherosclerosis in middle‐aged hypertensive and control subjects. A cross‐sectional systematic study with duplex ultrasound. J Hypertens 1996; 14: 1433–9
- Bayes‐Genis A., Conover C. A., Schwartz R. S. The insulin‐like growth factor axis: A review of atherosclerosis and restenosis. Circ Res 2000; 86: 125–30
- Gonzalez B., Lamas S., Melian E. M. Cooperation between low density lipoproteins and IGF‐I in the promotion of mitogenesis in vascular smooth muscle cells. Endocrinology 2001; 142: 4852–60
- Yamamoto M., Yamamoto K. Growth regulation in primary culture of rabbit arterial smooth muscle cells by platelet‐derived growth factor, insulin‐like growth factor‐I, and epidermal growth factor. Exp Cell Res 1994; 212: 62–8
- Delafontaine P., Lou H. Angiotensin II regulates insulin‐like growth factor I gene expression in vascular smooth muscle cells. J Biol Chem 1993; 268: 16866–70
- Colao A., Spiezia S., Cerbone G., Pivonello R., Marzullo P., Ferone D., et al. Increased arterial intima‐media thickness by B‐M mode echodoppler ultrasonography in acromegaly. Clin Endocrinol (Oxf) 2001; 54: 515–24
- Brevetti G., Marzullo P., Silvestro A., Pivonello R., Oliva G., di Somma C., et al. Early vascular alterations in acromegaly. J Clin Endocrinol Metab 2002; 87: 3174–9
- Maison P., Demolis P., Young J., Schaison G., Giudicelli J. F., Chanson P. Vascular reactivity in acromegalic patients: preliminary evidence for regional endothelial dysfunction and increased sympathetic vasoconstriction. Clin Endocrinol (Oxf) 2000; 53: 445–51
- Hughes A. D., Sinclair A. M., Geroulakos G., Mayet J., Mackay J., Shahi M., et al. Structural changes in the heart and carotid arteries associated with hypertension in humans. J Hum Hypertens 1993; 7: 395–7
- Spallarossa P., Brunelli C., Minuto F., Caruso D., Battistini M., Caponnetto S., et al. Insulin‐like growth factor‐I and angiographically documented coronary artery disease. Am J Cardiol 1996; 77: 200–2
- Watanabe T., Yamamoto H., Idei T., Iguchi T., Katagiri T. Influence of insulin‐like growth factor‐1 and hepatocyte growth factor on carotid atherosclerosis and cognitive function in the elderly. Dement Geriatr Cogn Disord 2004; 18: 67–74
- Rosen T., Bengtsson B. A. Premature mortality due to cardiovascular disease in hypopituitarism. Lancet 1990; 336: 285–8
- Bulow B., Hagmar L., Eskilsson J., Erfurth E. M. Hypopituitary females have a high incidence of cardiovascular morbidity and an increased prevalence of cardiovascular risk factors. J Clin Endocrinol Metab 2000; 85: 574–84
- Svensson J., Bengtsson B. A., Rosen T., Oden A., Johannsson G. Malignant disease and cardiovascular morbidity in hypopituitary adults with or without growth hormone replacement therapy. J Clin Endocrinol Metab 2004; 89: 3306–12
- Capaldo B., Guardasole V., Pardo F., Matarazzo M., Di Rella F., Numis F., et al. Abnormal vascular reactivity in growth hormone deficiency. Circulation 2001; 103: 520–4
- Borson‐Chazot F., Serusclat A., Kalfallah Y., Ducottet X., Sassolas G., Bernard S., et al. Decrease in carotid intima‐media thickness after one year growth hormone (GH) treatment in adults with GH deficiency. J Clin Endocrinol Metab 1999; 84: 1329–33
- Maccario M., Grottoli S., Procopio M., Oleandri S. E., Rossetto R., Gauna C., et al. The GH/IGF‐I axis in obesity: influence of neuro‐endocrine and metabolic factors. Int J Obes Relat Metab Disord 2000; 24(Suppl 2)96–9
- Maccario M., Gauna C., Procopio M., Di Vito L., Rossetto R., Oleandri S. E., et al. Assessment of GH/IGF‐I axis in obesity by evaluation of IGF‐I levels and the GH response to GHRH+arginine test. J Endocrinol Invest 1999; 22: 424–9
- Frystyk J., Skjaerbaek C., Vestbo E., Fisker S., Orskov H. Circulating levels of free insulin‐like growth factors in obese subjects: the impact of type 2 diabetes. Diabetes Metab Res Rev 1999; 15: 314–22
- Wabitsch M., Blum W. F., Muche R., Heinze E., Haug C., Mayer H., et al. Insulin‐like growth factors and their binding proteins before and after weight loss and their associations with hormonal and metabolic parameters in obese adolescent girls. Int J Obes Relat Metab Disord 1996; 20: 1073–80
- Marin P., Kvist H., Lindstedt G., Sjostrom L., Bjorntorp P. Low concentrations of insulin‐like growth factor‐I in abdominal obesity. Int J Obes Relat Metab Disord 1993; 17: 83–9
- Froesch E. R., Hussain M. Therapeutic potential of rhIGF‐I in diabetes and conditions of insulin resistance. J Intern Med 1993; 234: 561–70
- Bonora E., Tessari R., Micciolo R., Zenere M., Targher G., Padovani R., et al. Intimal‐medial thickness of the carotid artery in nondiabetic and NIDDM patients. Relationship with insulin resistance. Diabetes Care 1997; 20: 627–31
- Watarai T., Yamasaki Y., Ikeda M., Kubota M., Kodama M., Tsujino T., et al. Insulin resistance contributes to carotid arterial wall thickness in patients with non‐insulin‐dependent‐diabetes mellitus. Endocr J 1999; 46: 629–38
- Hussain M. A., Schmitz O., Mengel A., Glatz Y., Christiansen J. S., Zapf J., et al. Comparison of the effects of growth hormone and insulin‐like growth factor I on substrate oxidation and on insulin sensitivity in growth hormone‐deficient humans. J Clin Invest 1994; 94: 1126–33
- Hussain M. A., Schmitz O., Mengel A., Keller A., Christiansen J. S., Zapf J., et al. Insulin‐like growth factor I stimulates lipid oxidation, reduces protein oxidation, and enhances insulin sensitivity in humans. J Clin Invest 1993; 92: 2249–56
- Yakar S., Liu J. L., Fernandez A. M., Wu Y., Schally A. V., Frystyk J., et al. Liver‐specific igf‐1 gene deletion leads to muscle insulin insensitivity. Diabetes 2001; 50: 1110–8
- Frystyk J., Ledet T., Moller N., Flyvbjerg A., Orskov H. Cardiovascular disease and insulin‐like growth factor I. Circulation 2002; 106: 893–5
- Jialal I., Devaraj S., Venugopal S. K. C‐reactive protein: risk marker or mediator in atherothrombosis?. Hypertension 2004; 44: 6–11
- Heald A. H., Anderson S. G., Ivison F., Laing I., Gibson J. M., Cruickshank K. C‐reactive protein and the insulin‐like growth factor (IGF)‐system in relation to risk of cardiovascular disease in different ethnic groups. Atherosclerosis 2003; 170: 79–86
- Sesmilo G., Miller K. K., Hayden D., Klibanski A. Inflammatory cardiovascular risk markers in women with hypopituitarism. J Clin Endocrinol Metab 2001; 86: 5774–81
- Auernhammer C. J., Strasburger C. J. Effects of growth hormone and insulin‐like growth factor I on the immune system. Eur J Endocrinol 1995; 133: 635–45
- Cuneo R. C., Salomon F., McGauley G. A., Sonksen P. H. The growth hormone deficiency syndrome in adults. Clin Endocrinol (Oxf) 1992; 37: 387–97
- Yudkin J. S., Kumari M., Humphries S. E., Mohamed‐Ali V. Inflammation, obesity, stress and coronary heart disease: is interleukin‐6 the link?. Atherosclerosis 2000; 148: 209–14
- Barreto‐Filho J. A., Alcantara M. R., Salvatori R., Barreto M. A., Sousa A. C., Bastos V., et al. Familial isolated growth hormone deficiency is associated with increased systolic blood pressure, central obesity, and dyslipidemia. J Clin Endocrinol Metab 2002; 87: 2018–23
- Elhadd T. A., Abdu T. A., Oxtoby J., Kennedy G., McLaren M., Neary R., et al. Biochemical and biophysical markers of endothelial dysfunction in adults with hypopituitarism and severe GH deficiency. J Clin Endocrinol Metab 2001; 86: 4223–32
- Toye A. A., Van Hest B. J., Sheldon B. L., Moran C. Linkage between growth hormone (GH1) and solute carrier family 4 anion exchanger member 1 (SLC4A1) is conserved between birds and mammals. Anim Genet 1997; 28: 457–8
- Mansfield T. A., Simon D. B., Farfel Z., Bia M., Tucci J. R., Lebel M., et al. Multilocus linkage of familial hyperkalaemia and hypertension, pseudohypoaldosteronism type II, to chromosomes 1q31‐42 and 17p11‐q21. Nat Genet 1997; 16: 202–5
- Murray R. D., Wieringa G. E., Lissett C. A., Darzy K. H., Smethurst L. E., Shalet S. M. Low‐dose GH replacement improves the adverse lipid profile associated with the adult GH deficiency syndrome. Clin Endocrinol (Oxf) 2002; 56: 525–32
- Colao A., Marzullo P., Lombardi G. Effect of a six‐month treatment with lanreotide on cardiovascular risk factors and arterial intima‐media thickness in patients with acromegaly. Eur J Endocrinol 2002; 146: 303–9
- Angelin B., Rudling M. Growth hormone and hepatic lipoprotein metabolism. Curr Opin Lipidol 1994; 5: 160–5
- Rudling M., Olivecrona H., Eggertsen G., Angelin B. Regulation of rat hepatic low density lipoprotein receptors. In vivo stimulation by growth hormone is not mediated by insulin‐like growth factor I. J Clin Invest 1996; 97: 292–9