Abstract
Background. Respiratory distress syndrome (RDS) and bronchopulmonary dysplasia (BPD) have some common features with asthma.
Aim. To study whether G protein‐coupled receptor for asthma susceptibility (GPRA) contributes to RDS or BPD.
Methods. A haplotype association study was performed in a case‐control setting of 521 Finnish infants (including 176 preterm neonates with RDS and 37 with BPD). Immunoreactivity of GPRA isoforms A and B was determined in pulmonary samples of fetuses, term infants and preterm infants with RDS or BPD. GPRA mRNA expression was determined by quantitative real‐time polymerase chain reaction (PCR) in samples from nasal respiratory epithelium of adults, term infants and preterm infants.
Results. In infants with RDS born at 32–35 weeks of gestation, GPRA haplotype H1 was significantly underrepresented in RDS, whereas haplotype H4/H5 was associated with an increased risk. As in asthma, GPRA B isoform was induced in bronchial smooth muscle cells in RDS and BPD. In nasal respiratory epithelium, relative GPRA mRNA expression was strong in adults, weak in preterm and slightly higher in term samples.
Conclusions. The results suggest that near‐term RDS and asthma share the same susceptibility and protective GPRA haplotypes. Altered GPRA expression may play a role in the pathogenesis of RDS and BPD in preterm infants.
Introduction
We have recently identified a novel asthma susceptibility gene (GPRA, G protein‐coupled receptor for asthma susceptibility, alias GPR154, also known as neuropeptide S receptor) on chromosome 7p14‐15 by means of positional cloning Citation1. Three evolutionary closely related GPRA haplotypes were shown to associate with an increased risk of asthma‐related traits both among the Finnish and Canadian patients. The findings were recently replicated in large European cohorts in two independent studies Citation2,3.
GPRA has two full‐length variants, isoforms A and B, possessing alternatively spliced C termini with distinctive tissue distribution Citation1,Citation4. Both isoforms are expressed in multiple mucosal surfaces including epithelial cells throughout the bronchial tree. GPRA‐A has additional expression in smooth muscle cells. In human bronchial biopsies, GPRA‐B was upregulated in the smooth muscle cells of asthmatic airways in contrast to a lack of expression in the control samples. Thus, depending on the cytosolic signaling properties of the expressing cell types, the receptor activation may have variable biological effects.
We have also shown that the novel GPRA agonist, neuropeptide S (NPS) Citation5, co‐localizes with GPRA in the bronchial and colon epithelium Citation4. Although evidence concerning the functions of GPRA is only beginning to accumulate, the expression pattern of GPRA and NPS in the terminally differentiated epithelia may relate to a role involved in the first‐line defense of the innate immunity. The upregulated pulmonary mRNA levels of the murine ortholog Gpra after mold exposure in a murine model of severe ovalbumin‐induced inflammation support the hypothesis of GPRA as a responder gene against epithelial irritation Citation1.
In preterm infants, respiratory distress syndrome (RDS) is a major risk factor for bronchopulmonary dysplasia (BPD), and genetic polymorphisms of surfactant proteins (SPs) associate with the risk of RDS and BPD Citation6–8. Even though surfactant supplementation and other effective therapies have considerably decreased the incidence and severity of RDS, the disease cannot solely be attributed to surfactant deficiency. Several specific gene variants underlying the susceptibility or increasing the severity of RDS and BPD are yet to be identified. Although the biological relevance of the GPRA‐A and ‐B functions in the airways has not yet been resolved, the putative functions in the epithelial immunity and airway hyperresponsiveness prompted us to study whether GPRA haplotypes associate with RDS and BPD in near‐term or in very preterm infants. In addition, we used autopsy samples to determine whether the pulmonary expression of GPRA during fetal period and in infants with RDS or BPD differs from that of term subjects. Because suitable mRNA material from the pulmonary epithelium is virtually unavailable, we used scratch samples taken from the nasal respiratory epithelium of preterm infants to analyze whether GPRA mRNA expression differs from that of term and adult samples.
Key messages
Near‐term respiratory distress syndrome (RDS) and asthma share the same susceptibility and protective G protein‐coupled receptor for asthma susceptibility (GPRA) haplotypes.
Materials and methods
All the study protocols have been approved by the ethics committees of the participating centers, and an informed consent was signed by the parents of the subjects.
Diagnostics
The clinical diagnosis of RDS in the newborn infants was made by the presence of both clinical and X‐ray definition. They include requirement of supplemental oxygen and continuous distending airway pressures for at least 48 hours after birth unless treated with exogenous surfactant in established respiratory failure, diffuse reticulogranular pattern with air bronchograms and ground glass appearance of lung fields in the chest X‐rays. Infants receiving surfactant therapy prior to radiography were excluded. Neither pneumonia nor transient tachypnoea was diagnosed as RDS. BPD was defined as requirement of supplemental oxygen at postmenstrual age of 36 weeks for those born before 32 weeks of gestation Citation9.
DNA extraction, genotyping, haplotyping, and statistical analysis
The study population for association analysis of GPRA and RDS consisted of 521 Finnish infants born during the years 1996–2001 in the Oulu University Hospital. Two age‐matched case‐control comparisons were made (). The first comparison was performed in very preterm infants with gestational age (GA)<32 weeks with (n = 117) or without RDS (n = 57), and the second in infants born at 32⩽GA<35 weeks with (n = 59) or without RDS (n = 99). In the third analysis, very preterm infants with BPD (n = 37) were separately compared with GA‐matched controls with or without RDS. The group of term infants (n = 189) was used as a population control of haplotype frequencies.
Table I. Study subjects for genetic analyses. The number of excluded samples after quality assurance is indicated in brackets. RDS = respiratory distress syndrome; GA = gestation age.
DNA was extracted from a 3 mm disk punched from a blood spot dried on a S&S (Schleicher & Schuell) GB002 or S&S2992 filter paper using a method based on saponin and Chelex‐100 (Bio‐Rad) Citation10. Whole genome amplification was carried out with the extracted DNA using the improved primer preamplification (I‐PEP‐L) method and genotyped using the Sequenom platform as described Citation10,11.
Seven haplotype tagging intronic single nucleotide polymorphisms (SNPs) of the GPRA gene in the NCBI entry NT_007819 positions 34037316 (rs323917), 34044455 (rs323922), 34051648 (rs324377), 34068425 (not found in dbSNP), 34077700 (rs324384), 34085796 (rs324396) and 34107975 (rs740347) were initially genotyped (). Primers were designed by the SpectroDesigner software (Sequenom GmbH, USA), and genotyping was performed using matrix‐assisted laser desorption/ionization time‐of‐flight mass spectrometry (MALDI‐TOF) technology. The primer sequences and the technology have been previously described Citation2. All samples were analyzed in a sex‐specific assay (AMELXY, MWG‐Biotech AG) to validate the sample logistics. Three positive and three negative controls were included in each 96 well DNA plate, and all genotyping results were confirmed independently by two persons.
Table II. Haplotypes H1–H8 defined by the six tagging single nucleotide polymorphisms in a 70.7 kb interval of the GPRA gene intron 2 and haplotype frequencies among near‐term (32 weeks⩽gestation age<35 weeks) infants with respiratory distress syndrome (RDS) compared to the gestation‐age‐matched controls (Ctrl).
Hardy‐Weinberg equilibrium was evaluated for each SNP by chi‐square test with one degree of freedom. Haplotypes were analyzed using the SNPHAP program v.1.2.1 (http://www‐gene.cimr.cam.ac.uk/clayton/software/snphap.txt) for the cases and controls simultaneously. SNPHAP uses an expectation maximization (EM) algorithm to calculate maximum likelihood estimates of haplotype frequencies from population‐based unphased genotype data. SNPHAP lists the individual predicted chromosomewise haplotype assignments and their probabilities. The single‐marker data and pairwise linkage disequilibrium (LD) D´ values were calculated by Haploview v. 3.2 (available at http://www.broad.mit.edu/mpg/haploview/) Citation12. Haplotype comparisons were performed using a chi‐square test in a 2×2 contingency table (Arcus Quickstat Biomedical v. 1.0).
Immunohistochemistry
All the subjects for immunohistochemical analysis were treated in the Hospital for Children and Adolescents, Helsinki University Central Hospital, during the years 1992–2000. The paraffin‐embedded tissue samples were obtained from autopsies performed within 2 days of death (). Fetuses were aborted in mid‐pregnancy because of severe extrapulmonary anomalies. The term control group with macroscopically and microscopically normal lungs consisted of newborns who died of causes other than lung diseases at the age of <24 hours. The RDS group consisted of patients who died at the age of 3–16 days. The infants with severe BPD died at the age of 2.5–10 months.
Table III. Immunohistochemical analysis of paraffin‐embedded autopsy specimens with the GPRA‐A and ‐B isotype specific antibodies. The results are presented as the intensity of the expression (mean±SEM, score from 0 to 3) in the large bronchioles. GA = gestation age; SMC = smooth muscle cells; RDS = respiratory distress syndrome; BPD = bronchopulmonary dysplasia.
The specificities of the affinity‐purified antibodies against the two alternative carboxy termini of GPRA receptor as well as the staining protocol have been reported previously Citation1,Citation4. Briefly, the formalin‐fixed, paraffin‐embedded 5‐µm tissue sections were deparaffinized by xylene‐treatment followed by decreasing alcohol series. The slides were heated in a microwave oven in 10 mM citrate buffer, pH 6.0, for 5 min and stained using the enzymatic ABC (Avidin and Biotinylated horse‐radish peroxidase macromolecular Complex) method (Vectastain Elite ABC kit, Vector Laboratories, Burlingame, CA) with 3‐amino‐9‐ethylcarbazole (Vector Laboratories) as a substrate. Immunoreactivity was scored from 0–3 (0 = nonexistent, 1 = weak, 2 = moderate, 3 = strong) with two different observers in a blind‐review. Omission of the primary antibodies and staining with the nonimmunized sera was used as a negative control for parallel sections. Neither of these controls showed any immunoreactivity.
Quantitative real‐time reverse transcriptase PCR assay
The study subjects for mRNA analysis were treated in the Hospital for Children and Adolescents, Helsinki University Central Hospital, during the years 2004–2005. GPRA mRNA expression was studied in the nasal respiratory epithelium of 6 term (GA 39.3±1.2 weeks, mean±SD; birth weight 3480±200 g; male female ratio 3/3) and 7 preterm infants (GA 26.4±1.5 weeks; 980±180 g; 2/5), and 10 adults (41.4±8 years; 10/0) with no history of asthma or atopy. In the preterm infants the scratch test was performed twice, at the age of 1–4 h and 21–48 h. In the first sampling, the postnatal age of the subjects varied between 97±22 min in the term and 113±47 min in the preterm subjects.
The nasal samples were gathered under direct vision by scraping the nasal epithelium using a Rhino‐Probe (Arlington Scientific, Springville, UT), dispersed into lysis buffer and stored at −80°C as described Citation13. Subsequently, total RNA was extracted with RNeasy kit (Qiagen, Valencia, CA) and quantified using RiboGreen RNA Quantitation Kit according to the manufacturer's instructions (Molecular Probes, Eugene, OR). cDNA synthesis was performed with MultiScribe reverse transcriptase (Applied Biosystems, Foster City, CA) and oligodT primers for 60 min at 48°C after which the enzyme was inactivated at 95°C for 5 min. The quantitative real‐time reverse transcriptase PCR was performed by AbiPrism 7700 Sequence Detector System (Applied Biosystems, Foster City, CA) according to the manufacturer's instructions. The sequences of the GPRA primers and the probe designed by PrimerExpress version 2.0 software (ABI) were as follows: forward PCR primer 5'‐ CAAAGGCAAAAATCAAGGCTATC‐3', reverse PCR primer 5'‐AGGTTGAAATTGTCCAAAATGTCA‐3' and probe 6‐FAM‐5' CATCATCATTCTTGCCTTCATCTGCTGTTG‐3'‐TAMRA. 1 µl of the cDNA sample was amplified for GPRA detection in a final volume of 25 µl containing 12.5 µl of TaqMan Universal PCR Master Mix and AmplitaqGold polymerase (Applied Biosystems), 300 nM forward and reverse primers and 200 nM probe. After an initial incubation at 95°C for 10 min to activate the DNA polymerase, 40 cycles were performed for 15 s at 95°C and for 1 min at 60°C. Accumulation of PCR products was directly detected by continuous monitoring of the increase in fluorescence of the 6‐carboxyfluorescein (FAM) reporter dye using the Sequence Detection System software, version 1.7 (Applied Biosystems, Foster City, CA). The GPRA expression of each sample was normalized against that of cytokeratin 18 (CK18), which was used as an epithelial marker (GPRA:CK18, ng/fmol). Tissue excised from a healthy turbinate during rhinoplasty served as a standard. A dilution series of the standard was included with each reaction to improve the quantification information, which was calculated according to the amount of total RNA in the initial sample.
Results
Validation of the genotyping protocol
The genotyping success rate for the SNP at position 34068425 was 78%, while for the other six markers it varied between 84% and 97 %. The success rate for SNP 34068425 was low due to very low intensities in the mass spectrum, which made it virtually impossible to unambiguously distinguish between homozygotes for the minor allele and heterozygotes. Misclassification of the genotypes was therefore a potential problem, and this was supported by strong deviation from the Hardy‐Weinberg equilibrium. In order to avoid bias in the allele/genotype distributions, we excluded the haplotype H4 tagging SNP (34068425) and ended up analyzing the haplotypes H4 and H5 as one (H4/H5). Therefore, the final genetic analyses were performed using six haplotype tagging SNPs to generate the haplotypes H1–H8. The nomenclature of the SNPs is described in , where both the previously cited (NT_000380) and the database SNP reference contig (NT_007819) names of the genomic sequences are given.
We used dried blood spots as starting material because unamplified genomic DNA was not available from all the subjects. Construction of haplotypes resulted in an unexpectedly large number of haplotypes that did not fall into the categories of the previously identified haplotypes (H1–H8). Therefore, we selected 28 samples with unrecognized haplotypes (other than H1–H8) and regenotyped them in six replicates using genomic DNA as template. All replicates provided reproducible genotypes and increased the number of the H1–H8 haplotypes. This reanalysis showed that the original genotyping using whole genome amplified DNA template and only a single replicate produced a small fraction of false homozygote genotypes for some markers and resulted in an increased frequency of the predicted non‐H1–H8 haplotypes. In order to validate the whole genome amplification method itself, we randomly selected another set of 28 samples with recognized haplotypes (H1–H8) and re‐genotyped them using duplicates of both whole genome amplified and genomic DNA as template. According to the results, all of the samples provided reproducible haplotypes. However, among the original genotypes derived from a single replicate, there was a subset of samples having a biased haplotype distribution. These deviating results originated from the oldest sample cohort. Either the amount of blood on the filter paper was not enough to yield sufficient amounts of DNA or the quality of the paper was not compatible with the extraction method used. Since the DNA extraction method has successfully been used on similar S&S2992 filter papers of samples as old as 25 years Citation10, the problems were most likely originated from the amount of blood. Too low DNA concentration in the whole genome amplification reaction may have led to allele dropouts, and underestimation of the amount of heterozygotes. Therefore, this cohort (n = 63) and 23 additional samples with SNPHAP haplotype probability less than 0.5 were excluded from the final analysis as shown in .
GPRA polymorphisms are associated with RDS
The exclusion of the samples after quality assurance analysis decreased the study population from 521 subjects to a total of 435. The association analyses for RDS and BPD were carried out by comparing the haplotype frequencies in patients with GA‐matched healthy controls. RDS patients were divided into near‐term (32 wk⩽GA<35 wk) and very preterm (GA<32 wk) groups. All the BPD patients originated from the latter group. Among the near‐term infants H1 was underrepresented (OR 0.5; 95% CI 0.3–0.8; P = 0.01), whereas haplotype H4/H5 was overrepresented in the RDS group (OR 2.6; 95% CI 1.2–5.5; P = 0.01) (). Although the SNPs included in this study were specifically selected for tagging the previously identified haplotypes H1–H8 Citation1–3, we also analyzed allelic associations for each SNP and the outcomes mentioned above. The pairwise linkage disequilibrium values (D´) between the markers and the single‐marker allele frequencies are presented in . Corresponding associations were not found in the group of very preterm infants with RDS or BPD (data not shown).
Table IV. Pairwise linkage disequilibrium values (D´) and allele frequencies of the markers defining the haplotypes H1–H8 in the GPRA gene intron 2.
GPRA‐B is upregulated in the airways of premature infants with RDS and BPD
GPRA is abnormally expressed in bronchial biopsies of asthma patients when compared to healthy controls Citation1. Because the GPRA locus was identified here to associate with RDS, we examined whether the same abnormal expression pattern can be detected in premature infants with RDS and BPD. GPRA isoform‐specific expression in the lung was studied in the paraffin‐embedded autopsy samples of midterm fetuses, term infants and preterm infants with RDS and BPD (). Immunohistochemical analysis with the GPRA isoform‐specific antibodies demonstrated that neither GPRA‐A nor GPRA‐B was expressed in midterm fetal lung (). In full‐term infants who lived up to 24 hours and died of extrapulmonary causes, both GPRA‐A and ‐B were weakly expressed in the bronchial epithelium with an additional GPRA‐A expression in the bronchial smooth muscle cells (). In RDS and BPD, GPRA‐A immunoreactivity did not differ from the staining of the full‐term controls. GPRA‐A was weakly expressed in the bronchial epithelium, whereas bronchioles were mostly negative (, respectively). The epithelial staining of GPRA‐B in bronchi was also positive, slightly more intensive than in the full‐term controls. The most striking finding, however, was the upregulated GPRA‐B expression in the smooth muscle cells of the large bronchi in RDS and BPD (, respectively). These observations are identical to our previous findings in asthma Citation1,Citation4.
Figure 1. Immunohistochemical analysis of paraffin‐embedded specimens of fetuses (A, B), term infants without lung injury (C, D) and preterm infants with respiratory distress syndrome (RDS) (E, F) or bronchopulmonary dysplasia (BPD) (G, H) with the GPRA‐A (left panel) and ‐B isoform (right panel) specific antibodies. GPRA‐A and ‐B expression in the bronchial epithelium is absent in fetal samples and is induced in term samples. GPRA‐A is additionally expressed in the smooth muscle cells of the bronchioles. GPRA‐B expression is upregulated in the smooth muscle cells of the bronchioles in RDS and BPD.
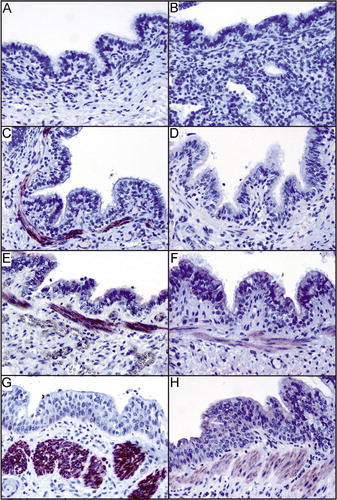
Atelectasis in the pulmonary autopsy samples hampered the detection of the alveolar staining pattern. Therefore, we studied adult human lung to demonstrate that the B isoform is expressed in the alveolar wall (). In addition, both GPRA isoforms are present in alveolar macrophages. These findings support the idea that GPRA has a role in the alveolus as well, and the GPRA pathway could be involved in the pathogenesis of RDS and BPD.
Expression of GPRA mRNA in the nasal respiratory epithelium
The nasal epithelium displays characteristics comparable to more distal airways and has been used as a representative of pulmonary epithelium Citation13–16. The results of the immunohistochemical stainings suggested that GPRA is upregulated after birth. In order to measure whether GPRA expression correlates with the maturity of the epithelial tissue, we used real‐time PCR to determine whether GPRA mRNA expression differs between the samples taken form the nasal epithelium of term and preterm infant as well as adult samples.
Strong signal (353±80.3, mean±SEM) was observed in all adult samples (n = 10), whereas at the age of 1–4 h, the relative GPRA mRNA expression was weak (6.3±1.5) in term infants (n = 6) and very weak or nonexistent (1.3±0.6) in preterm infants (n = 7) (). No significant differences were observed in the GPRA mRNA expression levels between the samples taken from the nasal epithelium at the age of 1–4 hours and 21–48 h of age (data not shown).
Discussion
The results of the present study suggest that the same susceptibility and protective GPRA haplotypes as previously described in the context of asthma may associate with the individual risk for RDS. As in asthma, the expression of the receptor B isoform was strong in the smooth muscle cell layer of the bronchi in severe RDS, and this expression persisted or even increased in infants developing fatal BPD. On the basis of the present evidence, we propose that GPRA plays a direct or indirect role in the pathogenesis of these diseases. However, the mechanism remains to be studied.
So far, significant associations between GPRA and asthma‐related phenotypes have been shown in four studies including several populations of European and Chinese origin Citation1–3, Citation17. Among the European cohorts, seven common and identical variants of the gene have been identified. The variants can be categorized into risk (H2, H4, H5, H6 and H7) and non‐risk/protective haplotypes (H1 and H3). These two groups represent different evolutionary branches of the phylogenetic tree of the haplotypes Citation2. The observed risk for a single haplotype varies between studies, but H1 and H3 are either neutral or protective for the disease risk in all cohorts. In the Chinese study cohort, the observed haplotype pattern was less polymorphic identifying only three common haplotypes Citation17. The strongest association was observed to a coding polymorphism in the first extracellular loop of the receptor that replaces the Asparagine 107 with Isoleucin Citation1. Based on sequencing results among the Finnish patients Citation1, Asn107Ile tags also the two groups of risk and non‐risk/protective haplotypes among the European study cohorts. Interestingly, a recent in vitro study showed that this coding polymorphism results in a gain‐of‐function characterized by an increase in agonist potency Citation18 in the Ile107 residue carrying receptor variant. Therefore the finding among the Chinese patients is not only further supporting the genetic hypothesis of the categorization of GPRA risk and non‐risk/protective haplotypes, but also suggesting direct involvement of this nonsynonymous polymorphism in the signal transduction capabilities of the receptor as a potential disease‐causing mechanism. In the present study, the genetic associations in the near‐term infants fall into the same categories: the carriers of H4/H5 show an increased risk and the carriers of H1 a decreased risk for RDS.
Asthma is characterized by obstruction of the airways, severe inflammatory response involving interleukin 1 (IL‐1), tumor necrosis factor α (TNF‐α), and other mediators, increased smooth muscle contraction and airway remodeling. The hypothesis that asthma and RDS/BPD may share some of the predisposing elements at the molecular level is based on indirect observations. First, epidemiological studies have repeatedly reported an increased risk of asthma or wheezing disease among preterm children with RDS or RDS and subsequent BPD Citation19–22. Second, the surfactant system prevents diffuse atelectasis, protects the airways from closure and may modulate atopic inflammation Citation23,24. Thus, besides the biophysical functions in alveoli and small airways, surfactant proteins modulate immune responses also in atopic conditions Citation24,25. Finally, the proinflammatory cytokines involved in asthma are known to be potent modulators of the expression of the surfactant components, including suppression by IL‐1 and TNF‐α in mature lung Citation26.
RDS in near‐term infants may have a more gradual onset after birth than RDS in very preterm infants, most of whom require ventilation at birth. Early description of type II respiratory distress syndrome among mostly near‐term infants revealed clinical characteristics of RDS shortly after birth (i.e. grunting, chest wall retraction, tachypnoea), yet the chest X‐ray showed diffuse overexpansion and lung edema Citation27. The infants with RDS in the present study all revealed low lung volumes and diffuse reticulogranularity or ground glass appearance of the chest X‐rays.
The association between RDS and GPRA haplotypes was observed in infants born at 32–35 weeks of gestation, whereas the same associations were not evident for RDS in very preterm infants born before 32 weeks of gestation. On the other hand, SP‐A haplotypes associated with RDS in the infants born very preterm but not in the near‐term infants Citation28. We propose that this genetic evidence is another reflection of the heterogeneity in the RDS phenotype. In the very preterm infants, immaturity of the surfactant system per se leads to RDS, whereas the significance of other etiological factors could become more dominant with more advanced lung maturity. RDS in very premature versus near‐term infants may in part be regarded as separate disease entities. Therefore, the gestation‐dependency in GPRA association in the present study is also in line with the previous observations Citation6.
In contrast to RDS, we could not detect any association between GPRA haplotypes and BPD, affecting many infants who were very preterm with RDS. In agreement with this, differences in allelic associations have previously been observed for these two overlapping diseases, since an intronic SP‐B variation has been shown to associate with BPD Citation7,Citation29, but not with GA‐matched RDS of the same ethnic background Citation6,Citation28. However, the small number of cases reduces the power to detect differences in the allelic frequencies leaving the question of potential association to be resolved in future studies with larger sample cohorts.
BPD is a complex multifactorial lung disease nearly exclusively affecting the infants born very preterm. Although the pathogenesis of BPD is not well understood, several studies have suggested that a preceding inflammation has a key role Citation30–33. Here, a clear upregulation of GPRA‐B protein expression was observed in the bronchial epithelia of the immature lung tissue in patients suffering from RDS and in infants with BPD (). Similar to our previous pathological finding in asthma, GPRA‐B was upregulated in the smooth muscle cells of the bronchi in RDS and remained highly expressed in BPD ( and ). Although this phenomenon may reflect increased work of breathing, it may as well be a consequence of inflammation. This result highlights the possibility that airways with a genetic predisposition for reactivity may become highly reactive in neonatal pulmonary disorders. Further studies are required to better understand the events observed both in neonatal lung diseases and asthma.
In RDS, the diffuse atelectasis shortly after the birth is due to insufficient pool size of surfactant and lack of critical surfactant components in the epithelial lining fluid, resulting in failure to lower the surface tension within the air spaces Citation34. However, the maintenance of alveolar stability, gas exchange and patency of the airways in acute lung injury throughout the course of RDS and in infants developing BPD becomes more dependent on complex interactions between the host defense systems, including the innate immunity. In the current study, immature lung tissue of fetal autopsies at GA<24 weeks expressed neither GPRA‐A nor ‐B. GPRA mRNA expression in the nasal respiratory epithelium of term and preterm infants (GA 26 weeks) up to 50 h after birth was very low, and only faint GPRA staining was observed in the bronchial epithelia of the lung tissue of term infants without lung injury. We have previously shown that GPRA is expressed in virtually all human epithelial tissues lining outer and internal body surfaces including skin, digestive tract and bronchial epithelium normally populated with commensal non‐dangerous microbes Citation4. GPRA mRNA was strongly expressed in the nasal respiratory epithelium of adult subjects. Thus, the results of the present study suggest that GPRA expression is developmentally regulated, with an additional environmental influence.
In conclusion, this is a pilot study demonstrating an association between GPRA and RDS, both at the gene and the expression levels. Infants suffering from RDS are also prone to wheezing during early childhood Citation19–22, and the present finding serves as additional support for the importance of airway reactivity in the pathogenesis. GPRA might participate in the development of RDS and, possibly BPD, in preterm infants, raising the possibility that molecules targeted to inhibit the GPRA pathway may play a role in moderation of severe respiratory diseases.
Acknowledgements
We would like to thank Marjatta Vallas for her skilful laboratory work.
This study has been supported by Finnish National Technology Agency Tekes, Academy of Finland, Sigrid Juselius Foundation, Päivikki and Sakari Sohlberg Foundation, Finska Läkaresällskapet, The Foundation for Pediatric Research, The Pulmonary Association Heli, The Maud Kuistila Memorial Foundation, Jalmari and Rauha Ahokas Foundation, The Research and Science Foundation of Farmos and The Swedish Research council.
References
- Laitinen T., Polvi A., Rydman P., Vendelin J., Pulkkinen V., Salmikangas P., et al. Characterization of a common susceptibility locus for asthma‐related traits. Science 2004; 304: 300–4
- Melén E., Bruce S., Doekes G., Kabesch M., Laitinen T., Lauener R., et al. Haplotypes of G‐protein‐coupled receptor 154 are associated with childhood allergy and asthma. Am J Respir Crit Care Med 2005; 171: 1089–95
- Kormann M., Carr D., Klopp N., Illig T., Leupold W., Fritzsch C., et al. G‐protein coupled receptor polymorphisms are associated with asthma in a large German population. Am J Respir Crit Care Med 2005; 171: 1358–62
- Vendelin J., Pulkkinen V., Rehn M., Pirskanen A., Räisänen‐Sokolowski A., Laitinen A., et al. Characterization of GPRA, a novel G protein‐coupled receptor related to asthma. Am J Respir Cell Mol Biol 2005; 33: 262–70
- Gupte J., Cutler G., Chen J. L., Tian H. Elucidation of signaling properties of vasopressin receptor‐related receptor 1 by using the chimeric receptor approach. Proc Natl Acad Sci U S A 2004; 101: 1508–13
- Haataja R., Rämet M., Marttila R., Hallman M. Surfactant proteins A and B as interactive genetic determinants of neonatal respiratory distress syndrome. Hum Mol Genet 2000; 9: 2751–60
- Rova M., Haataja R., Marttila R., Ollikainen V., Tammela O., Hallman M. Data mining and multiparameter analysis of lung surfactant protein genes in bronchopulmonary dysplasia. Hum Mol Genet 2004; 13: 1095–104
- Lahti M., Marttila R., Hallman M. Surfactant protein C gene variation in the Finnish population—association with perinatal respiratory disease. Eur J Hum Genet 2004; 12: 312–20
- Jobe A. H., Bancalari E. Bronchopulmonary dysplasia. Am J Respir Crit Care Med 2001; 163: 1723–29
- Hannelius U., Lindgren C. M., Melén E., Malmberg A., von Dobeln U., Kere J. Phenylketonuria screening registry as a resource for population genetic studies. J Med Genet 2005; 42: e60
- Sun G., Kaushal R., Pal P., Wolujewicz M., Smelser D., Cheng H., et al. Whole‐genome amplification: relative efficiencies of the current methods. Leg Med (Tokyo) 2005; 7: 279–86
- Barrett J. C., Fry B., Maller J., Daly M. J. Haploview: analysis and visualization of LD and haplotype maps. Bioinformatics 2005; 21: 263–5
- Helve O., Pitkänen O. M., Andersson S., O'Brodovich H., Kirjavainen T., Otulakowski G. Low expression of human epithelial sodium channel in airway epithelium of preterm infants with respiratory distress. Pediatrics 2004; 113: 1267–72
- Knowles M., Gatzy J., Boucher R. Increased bioelectric potential difference across respiratory epithelia in cystic fibrosis. N Engl J Med 1981; 305: 1489–95
- Jorissen M., Bertrand B., Eloy P. Ciliary dyskinesia in the nose and paranasal sinuses. Acta Otorhinolaryngol Belg 1997; 51: 353–66
- Knowles M. R., Carson J. L., Collier A. M., Gatzy J. T., Boucher R. C. Measurements of nasal transepithelial electric potential differences in normal human subjects in vivo. Am Rev Respir Dis 1981; 124: 484–90
- Feng Y., Hong X., Wang L., Jiang S., Chen C., Wang B., et al. G protein‐coupled receptor 154 gene polymorphism is associated with airway hyperresponsiveness to methacholine in a Chinese population. J Allergy Clin Immunol 2006; 117: 612–7
- Reinscheid R. K., Xu Y. L., Okamura N., Zeng J., Chung S., Pai R., et al. Pharmacological characterization of human and murine neuropeptide s receptor variants. J Pharmacol Exp Ther 2005; 315: 1338–45
- Schaubel D., Johansen H., Dutta M., Desmeules M., Becker A., Mao Y. Neonatal characteristics as risk factors for preschool asthma. J Asthma 1996; 33: 255–64
- Evans M., Palta M., Sadek M., Weinstein M. R., Peters M. E. Associations between family history of asthma, bronchopulmonary dysplasia, and childhood asthma in very low birth weight children. Am J Epidemiol 1998; 148: 460–6
- Smith J. An update on bronchopulmonary dysplasia: is there a relationship to the development of childhood asthma?. Med Hypotheses 2003; 61: 495–502
- Koivisto M., Marttila R., Saarela T., Pokela M. L., Valkama A. M., Hallman M. Wheezing illness and re‐hospitalization in the first two years of life after neonatal respiratory distress syndrome. J Pediatr 2005; 147: 486–92
- Hohlfeld J. M. The role of surfactant in asthma. Respir Res 2002; 3: 4
- Haczku A., Atochina E. N., Tomer Y., Cao Y., Campbell C., Scanlon S. T., et al. The late asthmatic response is linked with increased surface tension and reduced surfactant protein B in mice. Am J Physiol Lung Cell Mol Physiol 2002; 283: L755–65
- Hohlfeld J. M., Erpenbeck V. J., Krug N. Surfactant proteins SP‐A and SP‐D as modulators of the allergic inflammation in asthma. Pathobiology 2002–2003; 70: 287–92
- Väyrynen O., Glumoff V., Hallman M. Regulation of surfactant proteins by LPS and proinflammatory cytokines in fetal and newborn lung. Am J Physiol Lung Cell Mol Physiol 2002; 282: L803–10
- Sundell H., Garrott J., Blankenship W. J., Shepard F. M., Stahlman M. T. Studies on infants with type II respiratory distress syndrome. J Pediatr 1971; 78: 754–64
- Rämet M., Haataja R., Marttila R., Floros J., Hallman M. Association between the surfactant protein A (SP‐A) gene locus and respiratory‐distress syndrome in the Finnish population. Am J Hum Genet 2000; 66: 1569–79
- Makri V., Hospes B., Stoll‐Becker S., Borkhardt A., Gortner L. Polymorphisms of surfactant protein B encoding gene: modifiers of the course of neonatal respiratory distress syndrome?. Eur J Pediatr 2002; 161: 604–8
- Yoon B. H., Romero R., Kim K. S., Park J. S., Ki S. H., Kim B. I., et al. A systemic fetal inflammatory response and the development of bronchopulmonary dysplasia. Am J Obstet Gynecol 1999; 181: 773–9
- Martin R. J., Walsh‐Sukys M. C. Bronchopulmonary dysplasia—no simple solution. N Engl J Med 1999; 340: 1005–10
- Bancalari E. Changes in pathogenesis and prevention of chronic lung disease of prematurity. Am J Perinatol 2001; 18: 1–9
- Speer C. P. New insights into the pathogenesis of pulmonary inflammation in preterm infants. Biol Neonate 2001; 79: 205–9
- Hallman M., Merritt T. A., Akino T., Bry K. Surfactant protein A, phosphatidylcholine, and surfactant inhibitors in epithelial lining fluid. Correlation with surface activity, severity of respiratory distress syndrome, and outcome in small premature infants. Am Rev Respir Dis 1991; 144: 1376–84