Abstract
Inflammatory diseases are on the rise in westernized countries, but also in urbanized areas of developing countries. A number of studies have now demonstrated a negative association between helminth infections and inflammatory diseases, such as allergy, suggesting a potential role for helminth‐induced immune responses. However, this is not the case for all studies. In this review both supporting and opposing literature on the role of helminth infections, particularly in allergy, are discussed. Furthermore, the concept is put forward that chronic helminth infections, but not acute infections, may be associated with the expression of regulatory networks necessary for downmodulating allergic immune responses to harmless antigens. Lastly, different components of helminth‐induced regulatory networks are detailed, such as the role of regulatory T and B cells, modulation of dendritic cells, the presence of suppressory alternatively activated macrophages, and their individual contributions to protection against allergic diseases. Advantage should be taken from this knowledge to identify and select individual helminth‐derived molecules that may harbor therapeutic potential against inflammatory diseases.
Introduction
During the course of evolution man has constantly been exposed to a wide variety of microorganisms, ranging from harmless to life‐threatening ones. Our immune system has evolved to fiercely battle dangerous infectious agents but tolerate or ignore the innocent ones. In parallel with economic development, we are confronted with unexpected changes in disease patterns: an alarming rise in diverse chronic inflammatory disorders is observed. Countries with highly developed economies have more allergic disorders, more autoimmune diseases (e.g. type 1 diabetes or multiple sclerosis (MS)), and more inflammatory bowel disease (IBD) (ulcerative colitis and Crohn's disease) Citation1. It is proposed that education of the immune system by certain microbes and parasites can prevent the development of inflammatory diseases Citation2, Citation3. Reduced infections due to improved health care and personal hygiene, as well as decreased exposure to microorganisms and their products in our immediate environment as a result of urbanization, may lead to insufficient stimulation of the immune system. This would result in an altered programming of the immune system, allowing uncontrolled expression of inflammatory molecules, and therefore explaining the rise of inflammatory diseases in westernized countries. Indeed, a large body of epidemiological data indicates that some infectious agents tend to control inflammatory diseases, in which parasitic worms form an important group. In particular, the interplay between helminth infections and allergic disorders has been studied in great detail. In the current review we will focus on this interaction and highlight the importance of the infection dynamics and the regulatory network. Helminth‐induced mechanisms not only regulate host immunity, resulting in a mutually beneficial environment for survival of both the parasite and the host, but also control the development of allergic diseases.
Do helminths protect against allergy: evidence from epidemiological studies
One approach to determine the relationship between helminths and allergy is to study children who are living in endemic areas and are naturally infected with helminths, and analyze their allergic status and immune responses to parasite antigens and allergens. In most of these population studies general parameters for allergic reactions, such as allergen skin prick provocation tests and questionnaires (clinician‐assessed or self‐reported) were analyzed. A recent meta‐analysis on published data for studies involving intestinal helminth infections (Ascaris, Trichuris, and hookworms) did not show a consensus on the role of nematode infections in preventing allergic asthma, although hookworm infections did show some beneficial effects Citation4. In this report all published studies were included that reported parasite infection in at least 1% of the available study population. This led to an enormous variation in helminth intensities, in species of parasitic helminths involved, and in the populations studied in terms of genetics and environmental exposures. It is known that in some endemic areas close to 100% of the inhabitants can be infected, representing intense and chronic helminth infections, while in others helminth infections can occur occasionally and in a few percent of the population with intensities that are often mild. In areas with at least moderate endemicity for different species of helminth parasites, a considerable number of studies have demonstrated an inverse association between helminth infections and allergic disorders. For example, chronic infection with intestinal helminths (Ascaris, Trichuris, and hookworms) in Venezuela Citation5, Citation6, Gambia Citation7, Ethiopia Citation8, Taiwan Citation9, and Ecuador Citation10 was reported to protect from allergic reactivity. But also in areas endemic for other helminths, such as for schistosomes or filaria, the presence of infections appear to be associated with lower prevalences of allergies, as shown in studies in Gabon Citation11, Brazil Citation12, Citation13, Ghana (Hartgers et al., unpublished) or Indonesia (Djuardi et al., unpublished). However, population studies in areas where helminth infection intensities are low, occur only sporadically Citation14, Citation15, or are more transient in nature (e.g. when humans are not the definitive host and therefore chronic infection is not established) Citation16, Citation17, then helminth infections appear to potentiate atopic disorders. In addition, a proportion of travelers to endemic areas who become infected with schistosomes during a brief encounter develop acute schistosomiasis and can suffer from fever, lung eosinophilia, and pulmonary symptoms like cough and shortness of breath Citation18–20.
Short‐term application of anti‐helminth drugs (12 months) in Ecuador Citation21 did not change the prevalence or atopy, nor clinical signs of allergy (wheeze) in comparison to the untreated group. However, long‐term anti‐helminth treatment in Venezuelan (>22 months) or Gabonese children (>30 months) resulted in increased skin prick test reactivity to house dust mite Citation22, Citation23, supporting a direct link between chronic and intense helminth exposure and protection from allergy.
Therefore, it is likely that the different outcomes of helminth infection on allergic diseases are due to differences in acute versus chronic stages of infection or due to differences in intensity of infection (). To dissect the mechanisms that lead to protection against inflammatory diseases, including allergies, immune responses during helminth infections need to be evaluated in detail.
Key messages
Chronic, but not acute, helminth infection protects against allergic disease.
During chronic helminth infections regulatory mechanisms are induced that dampen immune responses to bystander antigens, such as allergens.
Different cell types are involved in immunoregulation during chronic helminth infection, i.e. regulatory T and B cells, alternatively activated macrophages, and possibly more.
Immunity during helminth infections
In developing countries, over one billion people are infected by different species of intestinal helminths (Ascaris, Trichuris, and hookworm) and/or tissue helminths (such as schistosomes, filarial worms, and tapeworms). Males show a higher susceptibility to helminth infections Citation24, Citation25. Direct mortality is low, as the majority of individuals chronically infected with helminths appears relatively asymptomatic (90%–95%), and a minority suffers from severe immunopathological disorders (5%–10%), often paralleled by enhanced cellular reactivity Citation26–28. Most of these helminths are long‐lived multicellular parasites that are masters in establishing chronic infections. For example, adult schistosome worms do not multiply but can lay millions of eggs that are deposited in mucosal tissues of the gastrointestinal tract or urinary bladder and result in granuloma formation and local pathological reactions. In the host, helminths induce polarized immune responses with complex characteristics.
The overall immune response in helminth‐infected individuals is characterized by polarized Th2 cells with increased production of interleukin (IL‐) 4, IL‐5, and IL‐13, eosinophilia and increased total serum immunoglobulin (Ig) E levels Citation29. Yet, Th2 responses are accompanied by a regulatory component, which seems to lead to immune hyperresponsiveness against parasite antigens and increased IL‐10 and transforming growth factor (TGF‐) β levels, often found in helminth‐infected individuals Citation11, Citation30. Immune suppression by regulatory networks is likely to play a major role in parasite survival strategies but can simultaneously benefit the host by minimizing pathologic responses in tissues involved. It has been argued that the observed immunosuppression may at least in part be accounted for by helminth‐induced regulatory T (Treg) cells. Indeed, in patients suffering from onchocerciasis, such antigen‐specific Treg cells could be cloned. These cells were characterized by the secretion of high levels of IL‐10 and/or TGF‐β and the inhibition of the proliferation of other T cells Citation31, Citation32. Likewise in patients infected with Brugia malayi increased expression of FoxP3 (specific transcription factor of natural Treg cells) was demonstrated, and the involvement of the regulatory molecules such as TGF‐β and cytotoxic T lymphocyte antigen 4 (CTLA‐4) in suppressory activity was demonstrated Citation33. Several observations indicate that this suppression is not strictly antigen‐specific and can extend to third party antigens, other pathogens, or vaccine antigens. Early studies indicated that Schistosoma mansoni infections resulted in reduced cytokine production and T cell proliferation to non‐related sperm whale myoglobin in an IL‐10‐dependent manner Citation34. With respect to influencing responses to coinfections, several studies have described an interaction between helminths and malarial parasites. Although the findings on malaria‐parasite loads are controversial, data on malaria pathology seem more uniform, showing that helminth infection protects from renal failure and cerebral malaria Citation35, Citation36. This protective effect has been described for AscarisCitation37, Citation38, S. haematobiumCitation39, Citation40, and for Brugia pahangiCitation41 infections. In addition, in S. mansoni‐infected mice the clearance of vaccinia virus infections was impaired Citation42. The effect of helminth infections on responses to vaccines has been explored, and in several studies impaired Th1 responses were reported to tetanus toxoid (TT) immunization in Schistosoma‐ and Onchocerca‐infected patients Citation43, Citation44. Likewise, experimental schistosome infections reduced the protective efficacy of bacille Calmette Guerin (BCG) vaccination against Mycobacterium tuberculosisCitation45.
It is hypothesized that bystander immunoregulation by helminth infection can also control allergen‐specific inflammatory responses and thereby lead to lower prevalence of allergies in helminth‐infected subjects (). Yet control of bystander T cell responses may depend on particular life cycle stages of an infection. Therefore, to understand how helminths can affect inflammatory diseases, we must first analyze how distinct life stages of helminths affect the host immune system.
Changes in the immune response during helminth infection: lessons from the mouse models
To appreciate the effect of distinct stages of helminth infections on the immune system, experimental models are needed as humans living in endemic areas are exposed early in life and continuously become reinfected and therefore simultaneously would carry different developmental stages of the parasite. In this respect, schistosome infections in mice have been studied in some detail, showing a higher susceptibility to infection in females Citation46. The subsequent usage of gene‐deficient mice, cell transfer experiments, and assessment of immune reactivity in different tissues or different lymphoid organs permit the dissection of immune events that may be extrapolated to the human situation.
In the first 4–5 weeks following skin penetration of cercariae, the prepatent period, immune responses to larval worm antigens are primarily Th1 in nature. A dramatic transition in the progression of schistosome life cycle occurs at week 5–6 of infection, when female parasites living in the portal vasculature mature, pair with males, and begin to produce eggs Citation47. At this time the immune response takes on an entirely different character, becoming strongly Th2‐polarized by week 8 post‐infection. This Th2 response is dependent on egg production, and its development is accompanied by the loss of worm antigen‐specific Th1 response Citation48, Citation49. Coincident with the development of Th2 responses, increases in serum IgE and circulating eosinophil numbers are found, reflecting the production of signature Th2 cytokines, IL‐4 and IL‐5, that help B cells in IgE class switching and act as growth and survival factor for eosinophils Citation50. After about 12–16 weeks of infection, Th2 and T cell proliferative responses decrease gradually Citation48. This state of comparative hyporesponsiveness persists for the remainder of the infection and is one of the hallmarks of the chronic stage of infection.
In parallel to humans, mice chronically infected with schistosomes exhibit dichotomy in symptoms Citation51: an asymptomatic intestinal form and a severe hepatosplenic form. Importantly, the proportion of mice and time by which the animals develop severe hepatosplenic disease and die, varies greatly between different mouse strains. For example, Balb/C mice develop very strong Th2 granulomas around the eggs compared to C57/Bl6 or CBA/J mice Citation52. Furthermore, portal hypertension and organomegaly (characteristics of severe hepatosplenic syndrome) during progression into chronic stage of infection are frequently observed in Balb/C and CBA/J mice, but less so in C57/Bl6 mice Citation53. Indeed, we have unpublished observations that schistosome‐infected Balb/C mice developed severe hepatosplenic disease already at low infection intensities compared to C57/Bl6 mice and thereafter died rapidly, resulting in a complete loss of a colony before week 16 (chronic stage). These findings have great implications for inflammatory disease models in which the interaction with chronic helminth infection in mice is studied. Since C57/Bl6 mice rarely display severe hepatosplenic disease at high infection intensities and readily develop asymptomatic chronic infections, this mouse strain would seem to be highly suitable for studying chronic asymptomatic schistosomiasis and its interaction with other diseases in an experimental model.
Murine models for allergy and helminth infections
To study the causal relationship between helminth infections and the development of allergic diseases, several groups have developed combined mouse models of asthma and infection. For example, studies with rodent nematodes, such as Heligmosomoides polygyrus, have demonstrated that infection leads to strongly reduced ovalbumin (OVA)‐driven eosinophilic airway inflammation Citation54, Citation55. In another study, immune responses to food allergens (IgE and IL‐13 production) were downmodulated by infection with H. polygyrusCitation56. This effect was reversed when animals were treated with anti‐IL‐10. A similar inhibition of allergic eye disease Citation57 or lung inflammation and airway hyperresponsiveness Citation58, Citation59 was demonstrated by either Ascaris suum eggs, A. suum extract implants, and by infection with Nippostrongylus brasiliensis. Importantly, infection with N. brasiliensis in IL‐10‐deficient mice strongly increased allergen‐induced airway eosinophilia and the other inflammatory parameters in comparison to control mice, supporting the view that immunosuppressive activities during helminth infections can inhibit allergic disorders Citation58.
However, there are also a number of studies showing no effect or a partial effect on a few immunological markers. For example, during infection with Strongyloides venezuelensis or S. stercoralis T cell cytokine responses or allergen‐specific IgE production, but not eosinophilic airway inflammation, are suppressed Citation60, Citation61. Some studies even find an exacerbation of allergic disease. For example, initiating infections with helminths like A. suum potentiated airway inflammation Citation62, and infection with Trichinella spiralis increased anaphylaxis in mice Citation63. That different parasitic helminths induce opposing effects in mouse models may suggest variations in the immune modulating capacity of distinct species. However, this paradox could also very well reflect the difference between acute and chronic stages of infection.
Infection of C57/Bl6 mice with S. mansoni is a well defined model in which distinct phases of acute and chronic infection have been detailed Citation47, Citation64. Indeed, by exploring acute and chronic infections with S. mansoni, we have observed a clear dose‐dependent reduction in ovalbumin (OVA)‐specific eosinophilic airway inflammation and airway hyperresponsiveness during chronic, but not during acute infection (Smits HH, et al, unpublished observations).
It has been reported in the literature that both worms and eggs of different helminths can modulate immune responses by affecting different types of innate and adaptive immune cells, thereby suppressing the host immune system to guarantee their own survival. Next to polarized Th2 responses, the development of regulatory T and B cells, possibly via immune‐modulated dendritic cells, as well as the induction of the so‐called suppressory, alternatively activated macrophages appears to be important elements of a chronic helminth infection.
Immune modulation by helminths and their effects on allergic disorders
Allergy includes a spectrum of different atopic diseases and symptoms like bronchial constriction, itchy eyes, runny nose, or eczema and is triggered by mediators released by mast cells and eosinophils after IgE is cross‐linked by allergens. These systemic inflammatory reactions are initiated by aberrant type 2 cytokines in response to allergens Citation65.
It is clear from a number of studies that regulatory networks, including cytokines, IL‐10, TGF‐β and regulatory T cells (both natural CD4+CD25+Foxp3+ and adaptive CD4+IL10+ Tr1 cells) protect against allergic disease Citation66 and that successful allergen‐specific immunotherapy in humans, leading to reduction in allergic symptoms, is associated with the emergence of IL‐10‐producing regulatory T cells Citation67–69. Indeed evidence is accumulating now that helminth‐induced regulatory T cells may also confer protection against allergic disease ().
Figure 2 Overview of immune modulation by helminths. 1) Immature dendritic cells (iDC) become activated by the exposure to either (compounds from) eggs (brown rod‐shaped symbols) or worms and will migrate through the draining lymph nodes (LN) while acquiring a mature phenotype. In the LN they will drive the development of polarized Th2 and regulatory T (Treg) cells, expressing IL‐4 and IL‐13 or IL‐10, respectively. These Treg cells, once they have migrated to the local site of inflammation, can inhibit the proliferation and effector function of other T cells. 2) Upon parasitic exposure together with harmless antigens, such as allergens, mature germinal centers (GC) are formed in the lymph nodes. In the GC B cells (B) are selected that recognize specific antigens, such as allergenic antigens, and will receive signals to develop into mature B cells. Some of these B cells have acquired the capacity to produce IL‐10 and will act as regulatory B cells at the peripheral site and will inhibit the effector function of other T cells. 3) Both the cytokines of Th2 cells and Treg cells, such as IL‐4, IL‐13 and IL‐10, as well as compounds from eggs or worms, can change the activation state of macrophages (MΦ), resulting in alternatively activated MΦ (aaMΦ). aaMΦ produce high levels of IL‐10 and TGF‐β instrumental in inhibiting the proliferation of other T cells, such as Th2 cells.
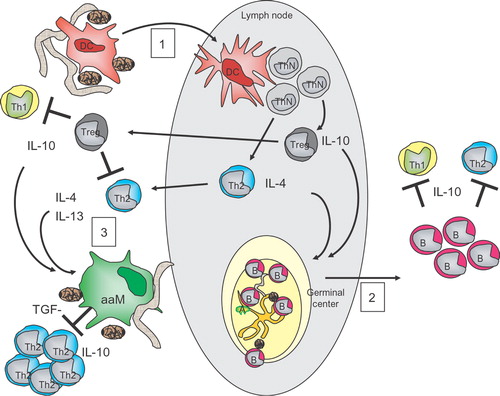
Helminth‐induced regulatory T and B cells
Several, but not all, studies have identified a role for either adaptive or naturally occurring regulatory T (Treg) cells in immune modulation during chronic helminth infections. For example, increased numbers of FoxP3‐expressing cells (FoxP3 being a natural Treg‐specific transcription factor) have been described in chronic nematode infections Citation54, Citation70, Citation71, while in chronic schistosome infections the ratio of natural Treg to effector Th cells remains unaltered Citation72. Interestingly, the frequency of CD103‐expressing cells in the natural Treg compartment increased during disease progression, indicating that the phenotype of the Treg cells changes during the course of schistosome infection Citation72. Since CD103 is described as an activation marker for natural Treg cells, it was suggested that these cells become more active during chronic schistosomiasis Citation73.
Elevated IL‐10 has been reported in many human and murine studies in the context of helminth infections, but the source and role of IL‐10 appears to vary in response to different helminth species Citation11, Citation31, Citation32. In some experimental models, the high IL‐10 was attributed to adaptive Treg cells Citation54, Citation55, Citation70, Citation71, while in others IL‐10 has been linked to non‐Treg cell populations Citation72, Citation74. By using IL‐10‐deficient mice and by adoptive transfer of different cell subsets, it was shown that both T‐cell‐ and non‐T‐cell‐derived IL‐10 play an essential part in helminth‐induced immune modulation Citation70, Citation74, Citation75. Importantly, during experimental infections with H. polygyrus allergic airway inflammation was suppressed by helminth‐induced Treg cells Citation54, Citation55, although this appeared to be mediated by IL‐10 in only one of the studies Citation55. This is in agreement with the capacity of Schistosoma‐derived molecules, such as lyso‐PS, to prime human dendritic cells in vitro, which in turn can induce naïve T cells to become IL‐10‐producing Treg cells Citation76. There is as yet no agreement on the role of IL‐10. Several studies have pointed out that although IL‐10 was increased (either produced by T cells or by other cell types), this may have no role in immune hyporesponsiveness, as immunosuppression in some helminth infections was still seen even in IL‐10‐deficient mice Citation54, Citation71, Citation77. More studies are needed in uniform experimental models where the helminth species, background of the mouse strains, kinetics, and intensity of infections is harmonized.
B cells possess a variety of immune functions, including production of antibodies, presentation of Ags and production of cytokines Citation78. A few studies have indicated that B cells may also participate in the induction of immune modulation by helminths. For example, µMT mice (lack mature B cells) rapidly die during the course of a S. mansoni infection Citation29. In this study granulomatous pathology in chronic schistosome‐infected mice was downregulated in a B cell‐dependent and Fc receptor signaling‐dependent manner Citation29. B cells have also been shown to contribute to immune tolerance and suppression of diseases, such as experimental encephalomyelitis and collagen‐induced arthritis; such B cells are named regulatory B cells Citation79. Several observations point to the presence of IL‐10‐producing regulatory B cells and the possible modulation of allergic disease by these cells (). For example, IL‐10‐producing B cells protect against the development of allergic anaphylaxis Citation80 or allergic airway inflammation Citation81 in single‐sex schistosome‐infected mice. In addition, also in H. polygyrus‐infected allergen‐sensitized mice, B cells can transfer protection against allergic airway inflammation (Wilson M, personal communication). Detailed studies have demonstrated that particularly peritoneal B‐1 cells (CD5+B220+) can produce large amounts of IL‐10 during murine schistosomiasis Citation82. Interestingly, schistosome‐infected B‐1 cell‐deficient xid mice were equally resistant to allergic anaphylaxis as infected wild‐type (WT) mice, suggesting that also a helminth‐modulated B‐2 cell population may protect against allergic symptoms, possibly via IL‐10 Citation80. Future studies need to dissect the role of different (regulatory) B cell subsets in immune suppression and protection against allergic disease.
Role of myeloid cells in driving suppression
Dendritic cells (DC), residing in the mucosal lining of various peripheral tissues, are central to the generation and polarization of adaptive immune responses. The main function of DC is to patrol the environment for possible danger signals, immediately activate local innate immune cells and subsequently initiate appropriate adaptive immune responses. Dendritic cells, but also other innate immune or resident tissue cells, are ultimately equipped to recognize a great variety of different pathogen‐associated molecular patterns (PAMP) by means of various families of pattern recognition receptors (PRR). Among PRR, the families of the Toll‐like receptors (TLR), which often recognize lipid‐conjugated moieties, and the C‐type lectins, which can bind different carbohydrate structures, are presently the best studied ones. As a consequence of ligation of TLR on their own or in combination with other receptors, intracellular signaling via MyD88‐ or Toll‐IL‐1R domain‐containing adaptor‐inducing IFN‐beta (TRIF)‐dependent pathways leads to downstream activation of activator protein‐1 (AP‐1), nuclear factor‐κB (NF‐κB), and interferon regulatory factor‐3 (IRF‐3) transcription factors Citation83. DC will integrate these signals and translate them by inducing different sets of Th1, Th2, or Treg‐polarizing molecules Citation84.
Several studies have implied that DC function is modulated by helminth infection and exposure to helminth or helminth egg antigens (). As such it was demonstrated that adoptive transfer of spleen or mesenteric lymph node DC from H. polygyrus‐infected mice could modulate the immune response to a Citrobacter rodentium infection in recipient mice, resulting in enhanced colitis. This effect was IL‐10‐dependent as helminth‐primed IL‐10‐deficient DC failed to induce enhanced C. rodentium‐mediated colitis Citation85.
Microarray analysis of schistosome egg‐stimulated mouse myeloid DC revealed the activation of a large set of inflammatory cytokines and chemokines in contrast to larval schistosomula‐stimulated DC Citation86. Interestingly, schistosome eggs also caused myeloid DC to produce interferon (IFN‐) β and the induced interferon‐stimulated gene (ISG) expression Citation86. While exploring the signaling pathways involved, it appeared that in schistosome‐infected MyD88‐deficent mice, egg granulomas in the liver were smaller and contained less eosinophils but showed stronger fibrosis. In these mice spleen cells produced less IFN‐γ, but more IL‐13 and IL‐10 compared to infected wild‐type mice, implying a role for MyD88 in the Th1/Th2 balance Citation87. Furthermore, in vitro inflammatory cytokine expression and NF‐κB activation was impaired in egg‐stimulated DC from MyD88‐deficient mice Citation88. However, signal transducer of activated T cells (STAT)‐1 phosphorylation and ISG expression was still intact. Indeed, it was noted that schistosome‐derived double‐stranded RNA was capable of triggering TLR3 activation (MyD88‐independent) in mouse myeloid DC in vitro, resulting in the production of IFN‐β Citation88. The in vivo consequences of TLR3 ligation on innate/acquired immune responses during schistosomiasis are still elusive.
So far a few signature molecules of various helminths have been identified that can modulate DC to drive strong Th2 responses. An important molecule in this respect is excretory secretory product (ES‐) 62, a secreted phosphorylcholine‐containing glycoprotein of the filarial nematode Acanthocheilonema viteae. Also soluble egg antigens (SEA) of S. mansoni contain several carbohydrate structures, such as α3‐fucose‐ and β2‐xylose‐containing N‐glycans, which appear to play an important role in Th2 cell polarization Citation89. Moreover, the molecule lacto‐N‐fucopentaose III (LNFPIII), terminating in the Lewis X trisaccharide (Lex), can confer Th2‐inducing properties to non‐schistosome molecules, such as to human serum albumin Citation90. Although LNFPIII specifically binds to the C‐type lectin, DC‐specific intercellular adhesion molecule (ICAM)‐3 grabbing non‐integrin (DC‐SIGN), via LexCitation91, its Th2‐promoting activity appears to be dependent on the simultaneous ligation of a different PRR, the TLR4 Citation92. Importantly, stimulation of TLR4 by LNFPIII leads only to activation of the mitogen‐activated protein (MAP)‐kinase intracellular signaling pathway, extracellular signal‐regulated kinase (ERK), while TLR4 activation by lipopolysaccharide (LPS) leads to the activation of three MAP‐kinase pathways: ERK, p38 and c‐Jun N‐terminal kinase (JNK). This suggests that the findings are not easily explained by LPS contamination of the SEA‐preparations. Recent findings indicate that ligation of multiple receptors, such as macrophage galactose‐type lectin (MGL), DC‐SIGN, and mannose receptor by SEA, inhibited the TLR‐mediated maturation of iDCs in response to poly I:C or LPS Citation93, suggesting cross‐regulation of different PRR pathways. Also Nippostrongylus brasiliensis excretory‐secretory (NES) glycoproteins have Th2‐promoting activity on DC Citation94 and a Th2‐inducing adjuvant function on bystander antigens Citation95. The exact nature of molecules involved in NES are unknown; however, the activity is heat‐labile and protease‐sensitive Citation95. Similarly, an IL‐4‐inducing factor in human basophils, the glycoprotein IPSE/alpha‐1 Citation96, has been identified in ES from S. mansoni eggs, which also contains several Lex structures Citation97. However, its effector function on dendritic cells still needs to be established. The Th2‐driving capacity of adult Brugia extracts may also depend on the presence of intact glycans, as this activity is abolished by periodate treatment, although it cannot be excluded that other structures are also destroyed by periodate Citation98. Taken together, helminth glycans are becoming characterized in much detail and are providing us with a large array of structures that can contribute to the induction of Th2 development.
Although there is much evidence for helminth‐mediated hyporesponsiveness in which the activity of Treg cells may play a crucial role, little is known about particular helminth molecules that can induce Treg cells. Nevertheless, it has been reported that a mixture of high molecular weight components (PI) from A. suum can inhibit the expression of major histocompatibility complex (MHC) II, CD80, CD86, and CD40 molecules on mouse CD11c+ DC, resulting in cells that do not support a strong T cell‐proliferative response in vitro. The inhibitory effect of PI was abolished in IL‐10‐deficient mice Citation99. Furthermore, schistosomal lyso‐phosphatidylserine (lyso‐PS), containing acyl chains that differ in length and possibly in the position of the double bond from mammalian lyso‐PS, can modulate DC to drive IL‐10‐producing Treg responses in vitroCitation76. Interestingly, this modulating activity was TLR2‐dependent Citation76. Recent reports claim a clear link between Treg cells and TLR2, as TLR2‐deficient mice showed a reduced number of CD4CD25 Treg subset. In addition, Treg cells responded directly to TLR2‐ligands, e.g. Pam3Cys, but not to other TLR ligands, to augment Treg cell proliferation Citation100, Citation101. It would be interesting to evaluate the effects of schistosome‐derived TLR2 ligands on Treg cell function in vivo.
Macrophages count among the most pleiotropic cells of the immune system, exhibiting a whole range of biological functions, including phagocytosis, killing and pro‐ and anti‐inflammatory activities. It is well recognized that microbial products together with the cytokines produced by effector T cells influence the activation state of macrophages. Well known are the classically activated macrophages (caMΦ), induced in a Th1 cytokine environment, which release inflammatory products, nitric oxide, and undergo oxidative burst, essential in protection against intracellular bacteria. Recently, it has been shown that a different macrophage type arises in a Th2 environment. Since these cells exhibit a different activation program, these are termed alternatively activated macrophages (aaMΦ) to distinguish them from the deactivated macrophages found in an exclusive IL‐10 environment Citation102. In the mouse, the aaMΦ cells can easily be recognized, because they upregulate Fizz1, Ym‐1, Arg‐1 and the macrophage galactose‐type lectin (MGL) Citation103, Citation104. Alternatively activated MΦ express high levels of PRR, fail to generate nitric oxide, but produce high amounts of IL‐10 and TGF‐β instead. Accordingly, aaMΦ exert selective immunosuppressive functions and inhibit T cell proliferation. They are found in healthy individuals in the placenta, lungs, and immune‐privileged sites, but also during chronic inflammatory diseases.
The presence of these aaMΦ was demonstrated in several parasitic infections, both in humans, in case of filariasis Citation105 and schistosomiasis Citation106, and in animal infection models of Brugia malayiCitation107, chronic Taenia crassicepsCitation108, Nippostrongylus brasiliensisCitation109 and Litomosoides sigmodontis infections Citation110. In helminth infections, aaMΦ are involved in fibrogenesis and protection against organ injury and therefore are necessary for the survival of the host. A cell‐specific knockout showed an extreme susceptibility to quickly die after S. mansoni infection, which was associated with increased Th1 cytokines, hepatic and intestinal histopathology, increased nitrite oxide synthase (NOS)‐2 activity, impaired egg expulsion, and sepsis Citation111. Furthermore, aaMΦ were shown to be involved in a cell contact‐dependent suppression of T cell proliferative responses Citation110. Recent studies suggested that negative regulation of T cell proliferation may occur via CD45 ligation by aaMΦ‐expressed macrophage galactose type C‐type lectin (MGL) Citation112. Studies on S. mansoni egg‐derived glycoconjugates, such as LNFPIII and lacto‐N‐neotetraose (LNnT), showed that parasite‐derived molecules can directly induce aaMΦ‐like cells Citation113. Intraperitoneal injection of LNFPIII or LNnT coupled to dextran induced the expression of a GR1+CD11b+F4/80+CD11c− macrophage population that did not secrete nitric oxide (NO) or prostaglandinE2 (PGE2) but instead produced high levels of IL‐10 and TGF‐β. The LNFPIII‐ and LNnT‐primed macrophages also suppressed the proliferation of anti‐CD3/CD28‐stimulated T cells in vitroCitation113. In contrast, the CD11b+GR‐1+ aaMΦ that were found in experimental T. crassiceps infections developed suppressive activity during the course of infection that relied on arginase activity and the production of reactive oxygen species Citation114. These data indicate that during chronic helminth infection aaMΦ cells are generated and, because of their tolerizing function at immune‐privileged sites, contribute to the general immune hyporesponsiveness observed in helminth‐infected individuals ().
Concluding remarks
There is evidence that chronic, but not acute, helminth infection is driving responses that protect against allergic disorders. It is hypothesized that in particular during chronic disease immunoregulatory processes are switched on, for example the development of regulatory T and B cells, possibly via their priming by dendritic cells and alternatively activated macrophages that have been in contact with certain signature immunomodulatory molecules. Interestingly, these molecules may affect not only the antigen‐presenting cells via PRR, but may also act on regulatory T cells directly to influence their effector function. The question still remains why tolerating mechanisms are only found in particular during chronic disease, but not during acute infection, as some of the immunomodulatory helminth‐derived molecules are already present early during infection. Is it just a matter of kinetics? Does it take more time and exposure to have all the individual regulatory processes in full action to form a robust regulatory network necessary for general hyporesponsiveness? The fact that higher infection intensity results in faster and stronger immune hyporesponsiveness is in favor of this possibility. In this respect, it is also interesting that individual mouse strains are either much more susceptible to helminth infection and die, or develop chronic disease instead and survive longer. The differences are most likely dependent on their genetic predisposition to develop Th1, Th2, or Treg cell responses with different kinetics. In search of novel therapies for inflammatory diseases, such as allergy, it would be ideal to exploit the ability of chronic helminth infections to modulate the immune system. Although promising results have been obtained by treating colitis patients with Trichuris suisCitation115, a whipworm that naturally infects pigs, it would be more practical to focus on individual helminth‐derived immunomodulatory molecules to selectively induce regulatory immune responses and avoid any possible side effects of natural worm infections. In view of the above conclusions, it would be sensible to focus on molecules that are expressed during the chronic phase of infection. A few molecules have been now identified (detailed above) that would make suitable candidates for therapy. It is important that efforts are made to bring these molecules to the clinic, preferably coupled to allergens to target allergen‐specific changes and allow low concentrations to be effective.
Acknowledgements
The work was supported by a VENI grant of the Dutch Foundation for Sciences (to HS) and by a ZonMW program grant (to HS and MY)
References
- Bach J. F. The effect of infections on susceptibility to autoimmune and allergic diseases. N Engl J Med 2002; 347: 911–20
- Schaub B., Lauener R., von Mutius E. The many faces of the hygiene hypothesis. J Allergy Clin Immunol 2006; 117: 969–77
- Kamradt T., Goggel R., Erb K. J. Induction, exacerbation and inhibition of allergic and autoimmune diseases by infection. Trends Immunol 2005; 26: 260–7
- Leonardi‐Bee J., Pritchard D., Britton J. Asthma and current intestinal parasite infection: systematic review and meta‐analysis. Am J Respir Crit Care Med 2006; 174: 514–23
- Lynch N. R., Lopez R., Isturiz G., Tenias‐Salazar E. Allergic reactivity and helminthic infection in Amerindians of the Amazon Basin. Int Arch Allergy Appl Immunol 1983; 72: 369–72
- Hagel I., Lynch N. R., Perez M., Di Prisco M. C., Lopez R., Rojas E. Modulation of the allergic reactivity of slum children by helminthic infection. Parasite Immunol 1993; 15: 311–5
- Nyan O. A., Walraven G. E., Banya W. A., Milligan P., Van Der S. M., Ceesay S. M., et al. Atopy, intestinal helminth infection and total serum IgE in rural and urban adult Gambian communities. Clin Exp Allergy 2001; 31: 1672–8
- Scrivener S., Yemaneberhan H., Zebenigus M., Tilahun D., Girma S., Ali S., et al. Independent effects of intestinal parasite infection and domestic allergen exposure on risk of wheeze in Ethiopia: a nested case‐control study. Lancet 2001; 358: 1493–9
- Huang S. L., Tsai P. F., Yeh Y. F. Negative association of Enterobius infestation with asthma and rhinitis in primary school children in Taipei. Clin Exp Allergy 2002; 32: 1029–32
- Cooper P. J., Chico M. E., Rodrigues L. C., Ordonez M., Strachan D., Griffin G. E., et al. Reduced risk of atopy among school‐age children infected with geohelminth parasites in a rural area of the tropics. J Allergy Clin Immunol 2003; 111: 995–1000
- van den Biggelaar A. H., van Ree R., Rodrigues L. C., Lell B., Deelder A. M., Kremsner P. G., et al. Decreased atopy in children infected with Schistosoma haematobium: a role for parasite‐induced interleukin‐10. Lancet 2000; 356: 1723–7
- Medeiros M Jr., Figueiredo J. P., Almeida M. C., Matos M. A., Araujo M. I., Cruz A. A., et al. Schistosoma mansoni infection is associated with a reduced course of asthma. J Allergy Clin Immunol 2003; 111: 947–51
- Araujo M. I., Lopes A. A., Medeiros M., Cruz A. A., Sousa‐Atta L., Sole D., et al. Inverse association between skin response to aeroallergens and Schistosoma mansoni infection. Int Arch Allergy Immunol 2000; 123: 145–8
- Palmer L. J., Celedon J. C., Weiss S. T., Wang B., Fang Z., Xu X. Ascaris lumbricoides infection is associated with increased risk of childhood asthma and atopy in rural China. Am J Respir Crit Care Med 2002; 165: 1489–93
- Obihara C. C., Beyers N., Gie R. P., Hoekstra M. O., Fincham J. E., Marais B. J., et al. Respiratory atopic disease, Ascaris‐immunoglobulin E and tuberculin testing in urban South African children. Clin Exp Allergy 2006; 36: 640–8
- Buijs J., Borsboom G., Renting M., Hilgersom W. J., van Wieringen J. C., Jansen G., et al. Relationship between allergic manifestations and Toxocara seropositivity: a cross‐sectional study among elementary school children. Eur Respir J 1997; 10: 1467–75
- Gonzalez‐Quintela A., Gude F., Campos J., Garea M. T., Romero P. A., Rey J., et al. Toxocara infection seroprevalence and its relationship with atopic features in a general adult population. Int Arch Allergy Immunol 2006; 139: 317–24
- Schwartz E., Kozarsky P., Wilson M., Cetron M. Schistosome infection among river rafters on Omo River, Ethiopia. J Travel Med 2005; 12: 3–8
- Schwartz E., Rozenman J., Perelman M. Pulmonary manifestations of early schistosome infection among nonimmune travelers. Am J Med 2000; 109: 718–22
- Meltzer E., Artom G., Marva E., Assous M. V., Rahav G., Schwartzt E. Schistosomiasis among travelers: new aspects of an old disease. Emerg Infect Dis 2006; 12: 1696–700
- Cooper P. J., Chico M. E., Vaca M. G., Moncayo A. L., Bland J. M., Mafla E., et al. Effect of albendazole treatments on the prevalence of atopy in children living in communities endemic for geohelminth parasites: a cluster‐randomised trial. Lancet 2006; 367: 1598–603
- Lynch N. R., Hagel I., Perez M., Di Prisco M. C., Lopez R., Alvarez N. Effect of anthelmintic treatment on the allergic reactivity of children in a tropical slum. J Allergy Clin Immunol 1993; 92: 404–11
- van den Biggelaar A. H., Rodrigues L. C., van Ree R., Van der Zee J. S., Hoeksma‐Kruize Y. C., Souverijn J. H., et al. Long‐term treatment of intestinal helminths increases mite skin‐test reactivity in Gabonese schoolchildren. J Infect Dis 2004; 189: 892–900
- Braga C., Dourado I., Ximenes R., Miranda J., Alexander N. Bancroftian filariasis in an endemic area of Brazil: differences between genders during puberty. Rev Soc Bras Med Trop 2005; 38: 224–8
- Mohamed‐Ali Q., Elwali N. E., Abdelhameed A. A., Mergani A., Rahoud S., Elagib K. E., et al. Susceptibility to periportal (Symmers) fibrosis in human schistosoma mansoni infections: evidence that intensity and duration of infection, gender, and inherited factors are critical in disease progression. J Infect Dis 1999; 180: 1298–306
- Colley D. G., Garcia A. A., Lambertucci J. R., Parra J. C., Katz N., Rocha R. S., et al. Immune responses during human schistosomiasis. XII. Differential responsiveness in patients with hepatosplenic disease. Am J Trop Med Hyg 1986; 35: 793–802
- Tweardy D. J., Osman G. S., el Kholy A., Ellner J. J. Failure of immunosuppressive mechanisms in human Schistosoma mansoni infection with hepatosplenomegaly. J Clin Microbiol 1987; 25: 768–73
- Bahia‐Oliveira L. M., Gazzinelli G., Eloi‐Santos S. M., Cunha‐Melo J. R., ves‐Oliveira L. F., Silveira A. M., et al. Differential cellular reactivity to adult worm antigens of patients with different clinical forms of schistosomiasis mansoni. Trans R Soc Trop Med Hyg 1992; 86: 57–61
- Jankovic D., Cheever A. W., Kullberg M. C., Wynn T. A., Yap G., Caspar P., et al. CD4+ T cell‐mediated granulomatous pathology in schistosomiasis is downregulated by a B cell‐dependent mechanism requiring Fc receptor signaling. J Exp Med 1998; 187: 619–29
- Grogan J. L., Kremsner P. G., Deelder A. M., Yazdanbakhsh M. Antigen‐specific proliferation and interferon‐gamma and interleukin‐5 production are down‐regulated during Schistosoma haematobium infection. J Infect Dis 1998; 177: 1433–7
- Satoguina J., Mempel M., Larbi J., Badusche M., Loliger C., Adjei O., et al. Antigen‐specific T regulatory‐1 cells are associated with immunosuppression in a chronic helminth infection (onchocerciasis). Microbes Infect 2002; 4: 1291–300
- Doetze A., Satoguina J., Burchard G., Rau T., Loliger C., Fleischer B., et al. Antigen‐specific cellular hyporesponsiveness in a chronic human helminth infection is mediated by T(h)3/T(r)1‐type cytokines IL‐10 and transforming growth factor‐beta but not by a T(h)1 to T(h)2 shift. Int Immunol 2000; 12: 623–30
- Babu S., Blauvelt C. P., Kumaraswami V., Nutman T. B. Regulatory networks induced by live parasites impair both Th1 and Th2 pathways in patent lymphatic filariasis: implications for parasite persistence. J Immunol 2006; 176: 3248–56
- Kullberg M. C., Pearce E. J., Hieny S. E., Sher A., Berzofsky J. A. Infection with Schistosoma mansoni alters Th1/Th2 cytokine responses to a non‐parasite antigen. J Immunol 1992; 148: 3264–70
- Hartgers F. C., Yazdanbakhsh M. Co‐infection of helminths and malaria: modulation of the immune responses to malaria. Parasite Immunol 2006; 28: 497–506
- Specht S., Hoerauf A. Does helminth elimination promote or prevent malaria?. Lancet 2007; 369: 446–7
- Nacher M., Singhasivanon P., Traore B., Dejvorakul S., Phumratanaprapin W., Looareesuwan S., et al. Short report: Hookworm infection is associated with decreased body temperature during mild Plasmodium falciparum malaria. Am J Trop Med Hyg 2001; 65: 136–7
- Nacher M., Gay F., Singhasivanon P., Krudsood S., Treeprasertsuk S., Mazier D., et al. Ascaris lumbricoides infection is associated with protection from cerebral malaria. Parasite Immunol 2000; 22: 107–13
- Lyke K. E., Dicko A., Dabo A., Sangare L., Kone A., Coulibaly D., et al. Association of Schistosoma haematobium infection with protection against acute Plasmodium falciparum malaria in Malian children. Am J Trop Med Hyg 2005; 73: 1124–30
- Briand V., Watier L., LE Hesran J. Y., Garcia A., Cot M. Coinfection with Plasmodium falciparum and schistosoma haematobium: protective effect of schistosomiasis on malaria in senegalese children?. Am J Trop Med Hyg 2005; 72: 702–7
- Yan Y., Inuo G., Akao N., Tsukidate S., Fujita K. Down‐regulation of murine susceptibility to cerebral malaria by inoculation with third‐stage larvae of the filarial nematode Brugia pahangi. Parasitology 1997; 114: 333–8
- Actor J. K., Shirai M., Kullberg M. C., Buller R. M., Sher A., Berzofsky J. A. Helminth infection results in decreased virus‐specific CD8+ cytotoxic T‐cell and Th1 cytokine responses as well as delayed virus clearance. Proc Natl Acad Sci U S A 1993; 90: 948–52
- Sabin E. A., Araujo M. I., Carvalho E. M., Pearce E. J. Impairment of tetanus toxoid‐specific Th1‐like immune responses in humans infected with Schistosoma mansoni. J Infect Dis 1996; 173: 269–72
- Cooper P. J., Espinel I., Paredes W., Guderian R. H., Nutman T. B. Impaired tetanus‐specific cellular and humoral responses following tetanus vaccination in human onchocerciasis: a possible role for interleukin‐10. J Infect Dis 1998; 178: 1133–8
- Elias D., Akuffo H., Pawlowski A., Haile M., Schon T., Britton S. Schistosoma mansoni infection reduces the protective efficacy of BCG vaccination against virulent Mycobacterium tuberculosis. Vaccine 2005; 23: 1326–34
- Eloi‐Santos S., Olsen N. J., Correa‐Oliveira R., Colley D. G. Schistosoma mansoni: mortality, pathophysiology, and susceptibility differences in male and female mice. Exp Parasitol 1992; 75: 168–75
- Pearce E. J., MacDonald A. S. The immunobiology of schistosomiasis. Nat Rev Immunol 2002; 2: 499–511
- Grzych J. M., Pearce E., Cheever A., Caulada Z. A., Caspar P., Heiny S., et al. Egg deposition is the major stimulus for the production of Th2 cytokines in murine schistosomiasis mansoni. J Immunol 1991; 146: 1322–7
- Matricardi P. M., Ronchetti R. Are infections protecting from atopy?. Curr Opin Allergy Clin Immunol 2001; 1: 413–9
- Mosmann T. R. T lymphocyte subsets, cytokines, and effector functions. Ann N Y Acad Sci 1992; 664: 89–92
- Henderson G. S., Nix N. A., Montesano M. A., Gold D., Freeman G. L Jr., McCurley T. L., et al. Two distinct pathological syndromes in male CBA/J inbred mice with chronic Schistosoma mansoni infections. Am J Pathol 1993; 142: 703–14
- Boros D. L. Immunopathology of Schistosoma mansoni infection. Clin Microbiol Rev 1989; 2: 250–69
- Fanning M. M., Peters P. A., Davis R. S., Kazura J. W., Mahmoud A. A. Immunopathology of murine infection with Schistosoma mansoni: relationship of genetic background to hepatosplenic disease and modulation. J Infect Dis 1981; 144: 148–53
- Wilson M. S., Taylor M. D., Balic A., Finney C. A., Lamb J. R., Maizels R. M. Suppression of allergic airway inflammation by helminth‐induced regulatory T cells. J Exp Med 2005; 202: 1199–212
- Kitagaki K., Businga T. R., Racila D., Elliott D. E., Weinstock J. V., Kline J. N. Intestinal helminths protect in a murine model of asthma. J Immunol 2006; 1628–35
- Bashir M. E., Andersen P., Fuss I. J., Shi H. N., Nagler‐Anderson C. An enteric helminth infection protects against an allergic response to dietary antigen. J Immunol 2002; 177: 3284–92
- Schopf L., Luccioli S., Bundoc V., Justice P., Chan C. C., Wetzel B. J., et al. Differential modulation of allergic eye disease by chronic and acute ascaris infection. Invest Ophthalmol Vis Sci 2005; 46: 2772–80
- Wohlleben G., Trujillo C., Muller J., Ritze Y., Grunewald S., Tatsch U., et al. Helminth infection modulates the development of allergen‐induced airway inflammation. Int Immunol 2004; 16: 585–96
- Lima C., Perini A., Garcia M. L., Martins M. A., Teixeira M. M., Macedo M. S. Eosinophilic inflammation and airway hyper‐responsiveness are profoundly inhibited by a helminth (Ascaris suum) extract in a murine model of asthma. Clin Exp Allergy 2002; 32: 1659–66
- Negrao‐Correa D., Silveira M. R., Borges C. M., Souza D. G., Teixeira M. M. Changes in pulmonary function and parasite burden in rats infected with Strongyloides venezuelensis concomitant with induction of allergic airway inflammation. Infect Immun 2003; 71: 2607–14
- Wang C. C., Nolan T. J., Schad G. A., Abraham D. Infection of mice with the helminth Strongyloides stercoralis suppresses pulmonary allergic responses to ovalbumin. Clin Exp Allergy 2001; 31: 495–503
- Enobe C. S., Araujo C. A., Perini A., Martins M. A., Macedo M. S., Macedo‐Soares M. F. Early stages of Ascaris suum induce airway inflammation and hyperreactivity in a mouse model. Parasite Immunol 2006; 28: 453–61
- Strait R. T., Morris S. C., Smiley K., Urban J. F Jr., Finkelman F. D. IL‐4 exacerbates anaphylaxis. J Immunol 2003; 170: 3835–42
- Wynn T. A., Thompson R. W., Cheever A. W., Mentink‐Kane M. M. Immunopathogenesis of schistosomiasis. Immunol Rev 2004; 201: 156–67
- Cumberbatch M., Dearman R. J., Kimber I. Characteristics and regulation of the expression on interleukin 1 receptors by murine Langerhans cells and keratinocytes. Arch Dermatol Res 1998; 290: 688–95
- Umetsu D. T., DeKruyff R. H. Immune dysregulation in asthma. Curr Opin Immunol 2006; 18: 727–32
- Akdis M., Verhagen J., Taylor A., Karamloo F., Karagiannidis C., Crameri R., et al. Immune responses in healthy and allergic individuals are characterized by a fine balance between allergen‐specific T regulatory 1 and T helper 2 cells. J Exp Med 2004; 199: 1567–75
- Francis J. N., Till S. J., Durham S. R. Induction of IL‐10+CD4+CD25+ T cells by grass pollen immunotherapy. J Allergy Clin Immunol 2003; 111: 1255–61
- Jutel M., Akdis M., Budak F., Aebischer‐Casaulta C., Wrzyszcz M., Blaser K., et al. IL‐10 and TGF‐beta cooperate in the regulatory T cell response to mucosal allergens in normal immunity and specific immunotherapy. Eur J Immunol 2003; 33: 1205–14
- McKee A. S., Pearce E. J. CD25+CD4+ cells contribute to Th2 polarization during helminth infection by suppressing Th1 response development. J Immunol 2004; 173: 1224–31
- Taylor J. J., Mohrs M., Pearce E. J. Regulatory T cell responses develop in parallel to Th responses and control the magnitude and phenotype of the Th effector population. J Immunol 2006; 176: 5839–47
- Baumgart M., Tompkins F., Leng J., Hesse M. Naturally occurring CD4+Foxp3+ regulatory T cells are an essential, IL‐10‐independent part of the immunoregulatory network in Schistosoma mansoni egg‐induced inflammation. J Immunol 2006; 176: 5374–87
- Huehn J., Siegmund K., Lehmann J. C., Siewert C., Haubold U., Feuerer M., et al. Developmental stage, phenotype, and migration distinguish naive‐ and effector/memory‐like CD4+ regulatory T cells. J Exp Med 2004; 199: 303–13
- Hesse M., Piccirillo C. A., Belkaid Y., Prufer J., Mentink‐Kane M., Leusink M., et al. The pathogenesis of schistosomiasis is controlled by cooperating IL‐10‐producing innate effector and regulatory T cells. J Immunol 2004; 172: 3157–66
- Sadler C. H., Rutitzky L. I., Stadecker M. J., Wilson R. A. IL‐10 is crucial for the transition from acute to chronic disease state during infection of mice with Schistosoma mansoni. Eur J Immunol 2003; 33: 880–8
- van der Kleij D., Latz E., Brouwers J. F., Kruize Y. C., Schmitz M., Kurt‐Jones E. A., et al. A novel host‐parasite lipid cross‐talk. Schistosomal lyso‐phosphatidylserine activates toll‐like receptor 2 and affects immune polarization. J Biol Chem 2002; 277: 48122–9
- Wynn T. A., Cheever A. W., Williams M. E., Hieny S., Caspar P., Kuhn R., et al. IL‐10 regulates liver pathology in acute murine Schistosomiasis mansoni but is not required for immune down‐modulation of chronic disease. J Immunol 1998; 160: 4473–80
- Harris D. P., Haynes L., Sayles P. C., Duso D. K., Eaton S. M., Lepak N. M., et al. Reciprocal regulation of polarized cytokine production by effector B and T cells. Nat Immunol 2000; 1: 475–82
- Mizoguchi A., Bhan A. K. A case for regulatory B cells. J Immunol 2006; 176: 705–10
- Mangan N. E., Fallon R. E., Smith P., van Rooijen N., McKenzie A. N., Fallon P. G. Helminth infection protects mice from anaphylaxis via IL‐10‐producing B cells. J Immunol 2004; 173: 6346–56
- Mangan N. E., van Rooijen N., McKenzie A. N., Fallon P. G. Helminth‐modified pulmonary immune response protects mice from allergen‐induced airway hyperresponsiveness. J Immunol 2006; 176: 138–47
- Velupillai P., Secor W. E., Horauf A. M., Harn D. A. B‐1 cell (CD5+B220+) outgrowth in murine schistosomiasis is genetically restricted and is largely due to activation by polylactosamine sugars. J Immunol 1997; 158: 338–44
- Akira S., Takeda K. Toll‐like receptor signalling. Nat Rev Immunol 2004; 4: 499–511
- Kapsenberg M. L. Dendritic‐cell control of pathogen‐driven T‐cell polarization. Nat Rev Immunol 2003; 3: 984–93
- Chen C. C., Louie S., McCormick B. A., Walker W. A., Shi H. N. Helminth‐primed dendritic cells alter the host response to enteric bacterial infection. J Immunol 2006; 176: 472–83
- Trottein F., Pavelka N., Vizzardelli C., Angeli V., Zouain C. S., Pelizzola M., et al. A type I IFN‐dependent pathway induced by Schistosoma mansoni eggs in mouse myeloid dendritic cells generates an inflammatory signature. J Immunol 2004; 172: 3011–7
- Layland L. E., Wagner H., da Costa C. U. Lack of antigen‐specific Th1 response alters granuloma formation and composition in Schistosoma mansoni‐infected MyD88‐/‐ mice. Eur J Immunol 2005; 35: 3248–57
- Aksoy E., Zouain C. S., Vanhoutte F., Fontaine J., Pavelka N., Thieblemont N., et al. Double‐stranded RNAs from the helminth parasite Schistosoma activate TLR3 in dendritic cells. J Biol Chem 2005; 280: 277–83
- Faveeuw C., Mallevaey T., Paschinger K., Wilson I. B., Fontaine J., Mollicone R., et al. Schistosome N‐glycans containing core alpha 3‐fucose and core beta 2‐xylose epitopes are strong inducers of Th2 responses in mice. Eur J Immunol 2003; 33: 1271–81
- Okano M., Satoskar A. R., Nishizaki K., Harn D. A Jr. Lacto‐N‐fucopentaose III found on Schistosoma mansoni egg antigens functions as adjuvant for proteins by inducing Th2‐type response. J Immunol 2001; 167: 442–50
- van Die I., van Vliet S. J., Nyame A. K., Cummings R. D., Bank C. M., Appelmelk B., et al. The dendritic cell‐specific C‐type lectin DC‐SIGN is a receptor for Schistosoma mansoni egg antigens and recognizes the glycan antigen Lewis x. Glycobiology 2003; 13: 471–8
- Thomas P. G., Carter M. R., Atochina O., Da'Dara A. A., Piskorska D., McGuire E., et al. Maturation of dendritic cell 2 phenotype by a helminth glycan uses a Toll‐like receptor 4‐dependent mechanism. J Immunol 2003; 171: 5837–41
- van Liempt E., van Vliet S. J., Engering A., Garcia Vallejo J. J., Bank C. M., Sanchez‐Hernandez M., et al. Schistosoma mansoni soluble egg antigens are internalized by human dendritic cells through multiple C‐type lectins and suppress TLR‐induced dendritic cell activation. Mol Immunol 2007; 44: 2605–15
- Balic A., Harcus Y., Holland M. J., Maizels R. M. Selective maturation of dendritic cells by Nippostrongylus brasiliensis‐secreted proteins drives Th2 immune responses. Eur J Immunol 2004; 34: 3047–59
- Holland M. J., Harcus Y. M., Riches P. L., Maizels R. M. Proteins secreted by the parasitic nematode Nippostrongylus brasiliensis act as adjuvants for Th2 responses. Eur J Immunol 2000; 30: 1977–87
- Schramm G., Falcone F. H., Gronow A., Haisch K., Mamat U., Doenhoff M. J., et al. Molecular characterization of an Interleukin‐4‐inducing factor from Schistosoma mansoni eggs. J Biol Chem 2003; 278: 18384–92
- Wuhrer M., Balog C. I., Catalina M. I., Jones F. M., Schramm G., Haas H., et al. IPSE/alpha‐1, a major secretory glycoprotein antigen from schistosome eggs, expresses the Lewis X motif on core‐difucosylated N‐glycans. FEBS J 2006; 273: 2276–92
- Tawill S., Le Goff L., Ali F., Blaxter M., Allen J. E. Both free‐living and parasitic nematodes induce a characteristic Th2 response that is dependent on the presence of intact glycans. Infect Immun 2004; 72: 398–407
- Silva S. R., Jacysyn J. F., Macedo M. S., Faquim‐Mauro E. L. Immunosuppressive components of Ascaris suum down‐regulate expression of costimulatory molecules and function of antigen‐presenting cells via an IL‐10‐mediated mechanism. Eur J Immunol 2006; 36: 3227–37
- Sutmuller R. P., den Brok M. H., Kramer M., Bennink E. J., Toonen L. W., Kullberg B. J., et al. Toll‐like receptor 2 controls expansion and function of regulatory T cells. J Clin Invest 2006; 116: 485–94
- Liu H., Komai‐Koma M., Xu D., Liew F. Y. Toll‐like receptor 2 signaling modulates the functions of CD4+ CD25+ regulatory T cells. Proc Natl Acad Sci U S A 2006; 103: 7048–53
- Gordon S. Alternative activation of macrophages. Nat Rev Immunol 2003; 3: 23–35
- Raes G., de Baetselier P., Noel W., Beschin A., Brombacher F., Hassanzadeh G. G. Differential expression of FIZZ1 and Ym1 in alternatively versus classically activated macrophages. J Leukoc Biol 2002; 71: 597–602
- Raes G., Brys L., Dahal B. K., Brandt J., Grooten J., Brombacher F., et al. Macrophage galactose‐type C‐type lectins as novel markers for alternatively activated macrophages elicited by parasitic infections and allergic airway inflammation. J Leukoc Biol 2005; 77: 321–7
- Piessens W. F., Ratiwayanto S., Tuti S., Palmieri J. H., Piessens P. W., Koiman I., et al. Antigen‐specific suppressor cells and suppressor factors in human filariasis with Brugia malayi. N Engl J Med 1980; 302: 833–7
- Todd C. W., Goodgame R. W., Colley D. G. Immune responses during human schistosomiasis mansoni. V. Suppression of schistosome antigen‐specific lymphocyte blastogenesis by adherent/phagocytic cells. J Immunol 1979; 122: 1440–6
- Loke P., MacDonald A. S., Robb A., Maizels R. M., Allen J. E. Alternatively activated macrophages induced by nematode infection inhibit proliferation via cell‐to‐cell contact. Eur J Immunol 2000; 30: 2669–78
- Rodriguez‐Sosa M., Satoskar A. R., Calderon R., Gomez‐Garcia L., Saavedra R., Bojalil R., et al. Chronic helminth infection induces alternatively activated macrophages expressing high levels of CCR5 with low interleukin‐12 production and Th2‐biasing ability. Infect Immun 2002; 70: 3656–64
- Reece J. J., Siracusa M. C., Scott A. L. Innate immune responses to lung‐stage helminth infection induce alternatively activated alveolar macrophages. Infect Immun 2006; 74: 4970–81
- Taylor M. D., Harris A., Nair M. G., Maizels R. M., Allen J. E. F4/80+ alternatively activated macrophages control CD4+ T cell hyporesponsiveness at sites peripheral to filarial infection. J Immunol 2006; 176: 6918–27
- Herbert D. R., Holscher C., Mohrs M., Arendse B., Schwegmann A., Radwanska M., et al. Alternative macrophage activation is essential for survival during schistosomiasis and downmodulates T helper 1 responses and immunopathology. Immunity 2004; 20: 623–35
- van Vliet S. J., Gringhuis S. I., Geijtenbeek T. B., van Kooyk Y. Regulation of effector T cells by antigen‐presenting cells via interaction of the C‐type lectin MGL with CD45. Nat Immunol 2006; 7: 1200–8
- Terrazas L. I., Walsh K. L., Piskorska D., McGuire E., Harn D. A Jr. The schistosome oligosaccharide lacto‐N‐neotetraose expands Gr1(+) cells that secrete anti‐inflammatory cytokines and inhibit proliferation of naive CD4(+) cells: a potential mechanism for immune polarization in helminth infections. J Immunol 2001; 167: 5294–303
- Brys L., Beschin A., Raes G., Ghassabeh G. H., Noel W., Brandt J., et al. Reactive oxygen species and 12/15‐lipoxygenase contribute to the antiproliferative capacity of alternatively activated myeloid cells elicited during helminth infection. J Immunol 2005; 174: 6095–104
- Summers R. W., Elliott D. E., Urban J. F Jr., Thompson R. A., Weinstock J. V. Trichuris suis therapy for active ulcerative colitis: a randomized controlled trial. Gastroenterology 2005; 128: 825–32