Abstract
Despite the success of drug-eluting stents (DES) in reducing restenosis and the need for target vessel revascularization, several deficiencies have been unraveled since their first clinical application including the risk of stent thrombosis, undesired effects due to the stent polymer as well as the stent itself, and incomplete inhibition of restenosis (especially in complex lesions). Several novel stent systems are being investigated in order to address these issues. In second-generation DES, the rapamycin analogues zotarolimus and everolimus (and more recently biolimus) have been most extensively studied. Furthermore, special stent-coatings to actively promote endothelial healing (in order to reduce the risk of stent thrombosis) and to further reduce restenosis have been employed. To avoid undesirable effects of currently applied (durable) polymers, biocompatible and bioabsorbable polymers as well as DES delivery systems without the need for a polymer have been developed. Bioabsorbable stents, both polymeric and metallic, were developed to decrease potential late complications after stent implantation. Although most of these innovative novel principles intuitively seem appealing and demonstrate good results in initial clinical evaluations, long-term large-scale studies are necessary in order to reliably assess whether these novel systems are truly superior to first-generation DES with respect to safety and efficacy.
Introduction
One of the main mid- to long-term complications of percutaneous transluminal coronary angioplasty (PTCA) is the development of restenosis with rates reported to be as high as 50% Citation1. With the introduction of balloon-expandable bare-metal stents (BMS), restenosis rates and the need for target vessel revascularization (TVR) were greatly reduced as compared to angioplasty alone Citation2, Citation3. As the risk of restenosis remained around 30%, however, drug-eluting stents (DES) were designed which release pharmacological agents after deployment in order to inhibit vascular smooth muscle cell proliferation and in turn restenosis.
First-generation DES were coated with either rapamycin (sirolimus, Cypher® stent) or paclitaxel (Taxus® stent). After implantation, restenosis rates and the need for TVR dropped to below 10% (and even to 0% in some initial patient populations) as compared to BMS Citation4, Citation5. Already in the first series of patients treated with BMS, however, stent thrombosis emerged as a severe complication, especially after cessation of dual antiplatelet therapy, with a high morbidity and mortality Citation6. Meanwhile, some studies have implied that the use of DES may be associated with an even higher risk for stent thrombosis as compared with BMS Citation7–10. Moreover, a number of reports imply that thrombosis rates of DES may be higher in ‘real-world’ patients than in clinical trials Citation11, Citation12. Although other studies did not find an increased risk of stent thrombosis after DES implantation as compared to BMS Citation13, Citation14, the fear of it remains high owing to the oftentimes catastrophic clinical consequences.
Key messages
Despite the success of drug-eluting stents, several deficiencies have been unraveled since their first clinical application.
Several novel stent systems have been developed in order to address these issues.
Although most of these innovative novel principles show good results in initial clinical evaluations, long-term large-scale studies are necessary in order to reliably assess their safety and efficacy.
The substances that are eluted from DES primarily inhibit migration and proliferation of vascular smooth muscle cells (VSMC), which represent the crucial events in the development of in-stent restenosis Citation10. However, these agents not only inhibit VSMC migration and proliferation, but also affect other cell types such as endothelial cells. Among the available substances, rapamycin and paclitaxel have been studied extensively. Indeed, it was shown that both agents decrease migration and proliferation of mature endothelial cells Citation15, Citation16 as well as proliferation, differentiation, and homing of endothelial progenitor cells Citation17, Citation18. Furthermore, rapamycin and paclitaxel induce the expression of tissue factor, a key factor in the initiation of coagulation and thrombus formation Citation19, Citation20. All of these effects appear particularly important, as they may be responsible for the possibly increased risk for stent thrombosis with DES. Moreover, the polymer used for DES has been associated with hypersensitivity reactions, which may favor stent thrombosis as well Citation21, Citation22.
Hence, several aspects of currently available DES need improvement:
Further reduction in restenosis, especially after stenting of complex lesions
Avoidance of unwanted effects due to the stent polymer (and the stent itself)
Reduction of the risk of stent thrombosis.
Numerous different technological advances are currently under investigation or are already used in clinical practice to achieve these goals (). This review focuses on recent improvements in drug-eluting stent technology, particularly with respect to novel antiproliferative agents, polymers, and stent designs (; ). The discussion of technical improvements in strut technology (e.g. open- versus closed-cell; thin versus thick struts) is beyond the scope of this paper and is reviewed elsewhere Citation23.
Figure 2. Simplified, schematic overview over some of the novel strategies in coronary stent systems. First-generation DES consist of a stent strut and a polymer, on which the specific agents (e.g. sirolimus, paclitaxel) are loaded (A). In the next generation of coronary stents, several novel approaches were undertaken to overcome the limitations of first-generation DES. For example, novel drugs, biocompatible polymers, and more flexible stents were developed (B). Furthermore, systems using bioresorbable polymers (which are absorbed after elution of the specific agent) are being tested (C). Another approach uses micro- or nanoporous surfaces, into which agents are loaded without the need for a polymer (D). Similarly, ‘reservoir’ stents contain pores, in which the specific agent (+/ − a biocompatible polymer) is loaded, leaving a ‘clean’ surface, thereby facilitating deployment and potentially reducing thrombogenicity. Moreover, bidirectional, differential elution would be feasible with the use of this system (E). ‘Bioactive’ stents are coated with specific substances to facilitate re-endothelialization in order to tackle the doom of stent thrombosis (F). Finally, a novel system of stents that are absorbed after a certain time following deployment (‘bioresorbable stent’) is being developed (G). See text for further details. Of note, some of these systems combine several of the above-shown principles.
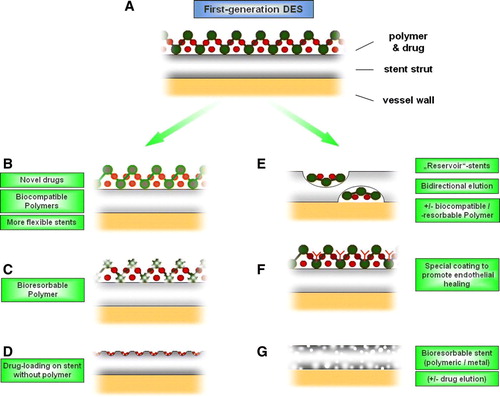
Table I. List of the novel stent systems discussed in this review, including the (proposed) main improvement as compared to first-generation DES.
The next generation of coronary stents
Novel drugs
Several novel DES systems have been developed and tested since the first introduction of sirolimus- and paclitaxel-eluting stents; in this regard, not only the agent loaded on the stent but also the polymer used for coating and the stent platform were subject to improvement. Novel substances include rapamycin analogues (e.g. everolimus, zotarolimus, biolimus) as well as other substances such as pimecrolimus and tacrolimus. Moreover, loading of more than one agent is being attempted, e.g. pimecrolimus + paclitaxel (SymBio® stent) or zotarolimus + dexamethasone (Zodiac program).
Zotarolimus-eluting stents (Endeavor®, ZoMaxx®)
On the Endeavor® stent, zotarolimus (an analogue of rapamycin) is loaded on a Driver-BMS, a cobalt-chromium alloy stent known for its enhanced flexibility as compared to stainless steel stents; furthermore, the drug is eluted from a phosphorylcholine polymer, which has the (theoretical) advantage of causing less hypersensitivity reactions owing to its better biocompatibility. In the ENDEAVOR II study, the primary end point of target vessel failure at 9 months was reduced by 52% (7.9% versus 15.1%, P = 0.0001) with the Endeavor stent as compared to the Driver-BMS, and the rate of major adverse cardiac events (MACE) was reduced by 51% (7.3% versus 14.4%, P = 0.0001); the differences in clinical outcome were maintained at 12 and 24 months (P < 0.0001) Citation24.
The ENDEAVOR III follow-up study, however, failed to meet non-inferiority as compared to the Cypher first-generation DES with respect to the primary end point of angiographic in-segment late lumen loss (0.34 + / − 0.44 mm versus 0.13 + / − 0.32 mm, respectively; P<0.001) at 9 months; moreover, binary angiographic restenosis (11.7% versus 4.3%, P = 0.04) and total (clinically and non-clinically driven) target lesion revascularization (TLR) rates (9.8% versus 3.5%, P = 0.04) were higher in the Endeavor as compared to the Cypher group Citation25. At 24 months, however, both the rates of MACE and target lesion revascularizations were not statistically different for the two DES (data presented at the ACC Meeting 2007). This may have occurred because the lesions were anatomically simple, and a difference might not be detected with the number of patients included in this study. Just recently Medtronic reported at the TCT meeting 2007 that the ENDEAVOR IV trial (a single-blind trial comparing the safety and efficacy of the Endeavor® DES to the Taxus paclitaxel-eluting coronary Stent) met its primary end point of target vessel failure (defined as a composite of cardiac death, myocardial infarction, and target vessel revascularization) at 9 months.
The ZoMaxx® stent equally elutes zotarolimus from a biocompatible phosphorylcholine coating but with an additional cap resulting in a different drug elution profile (complete elution at 30 days as compared to 10 days in the Endeavor stent). As the ZOMAXX I and ZOMAXX II trials comparing the novel system with the paclitaxel-eluting Taxus® stent yielded mixed results (data presented at the ACC Meeting 2007), the manufacturer recently withdrew this stent system from the market.
Everolimus-eluting stent (Xience®)
The ‘Xience V’® DES is loaded with everolimus, another analogue of rapamycin; the stent is designed to be more flexible than first-generation DES, by eluting the substance from a thin cobalt-chromium strut, and to be more biocompatible due to the novel fluoropolymer used for coating. In a 60-patient safety and effectiveness study (SPIRIT FIRST), the angiographic in-stent late luminal loss (primary end point) was 0.10±0.23 mm in the group receiving the everolimus-eluting stent versus 0.84±0.36 in the control BMS arm. At 2 years, clinically driven TLR rate was 7.7% in the Xience stent group vs. 21.4% in the control BMS group; MACE were also lower in the treatment group (3.8% versus 25%) (data presented at the EuroPCR Meeting 2006). The SPIRIT III trial, which was designed as the US pivotal approval study for the Xience V stent, showed a 50% reduction in the primary end point of late loss at 8-month angiographic follow-up as compared to the Taxus stent (0.14±0.41 mm versus 0.28±0.48 mm, P<0.0001 for non-inferiority and P=0.004 for superiority); furthermore, the trial demonstrated non-inferiority with respect to target vessel failure at 9 months and a reduction in MACE with the Xience V stent (data presented at the ACC Meeting 2007). After 12 months patients treated with the everolimus-eluting stent had significantly fewer major cardiac adverse events than patients treated with the paclitaxel-eluting stent (5.8 versus 9.9%, P < 0.01), mainly due to a lower target vessel revascularization rate (3.3% versus 5.6%, P<0.05) (data presented at the TCT meeting 2007).
Novel polymers
Biocompatible polymers
The (durable) polymers used for coating of first-generation DES have been accused of causing hypersensitivity reactions in some patients, which may lead to a pronounced inflammatory reaction and, in some cases, to stent thrombosis Citation21, Citation22. In order to overcome this limitation of first-generation DES, biocompatible co-polymer coatings were developed.
On the Endeavor Resolute® stent, the novel BioLinxTM polymer is used for drug elution. This system is composed of three elements: One hydrophobic polymer (‘C10’) to retain the drug and control drug release, another polymer (‘C19’) to provide improved biocompatibility, and finally (on the outer-most side of the stent) a polyvinyl pyrrolidinone (PVP) hydrophilic polymer which increases the initial drug burst and further enhances biocompatibility. This particular setup was chosen to optimize the drug elution profile while at the same time minimizing the risk of eliciting an inflammatory response of the vessel wall; therefore, this stent should prove particularly valuable when used in patients with complex medical conditions (e.g. diabetes) and difficult lesions. In the RESOLUTE trial, no TLR or TVR was observed with a MACE rate of 7.0% after 9 months in a patient population with an overall high percentage of complex lesions and patient characteristics (including small vessels and long lesions); the study's primary end point, in-stent late lumen loss, was 0.22 mm (data presented at the EuroPCR Meeting 2007).
Bioabsorbable polymers
Using bioabsorbable polymers for coating represents another attempt to overcome the problems encountered with first-generation DES polymers mentioned above. However, absorption of the polymer may per se led to an increased inflammatory reaction in the vessel wall Citation26. Hence, only an inert bioabsorbable polymer, which is absorbed slowly and in and of itself does not elicit an inflammatory reaction in the vessel wall, is suitable.
On the BioMATRIX® stent, the agent (biolimus, a rapamycin analogue specifically designed for use on DES) is loaded on such a biodegradable polymer. Furthermore, the coating is only eluted from the abluminal stent surface leaving a naked surface exposed to the luminal side with the potential advantage of a reduced prothrombotic potential of the stent. In the first-in-human evaluation of the BioMATRIX® DES (STEALTH-I trial), in-stent late lumen loss was significantly decreased after 6 months as compared to the control uncoated stent (0.26 + / − 0.43 versus 0.74 + / − 0.45 mm, P<0.001) Citation27. Follow-up studies, such as the LEADERS trial (comparing the BioMatrix® with the Cypher® stent) and the STEALTH II trial (comparing the biolimus-eluting stent with the Taxus stent) are ongoing.
In the SIMPLE trial, examination of the Infinnium® stent using a similar system composed of two biodegradable polymers with different paclitaxel-release kinetics appeared safe and effective in reducing restenosis at 6 months (data presented at the EuroPCR Meeting 2006).
In the FUTURE I and FUTURE II trials, the everolimus-eluting Champion® stent was compared to BMS. After 6 months, patients receiving the everolimus-eluting stent had decreased in-stent late lumen loss as compared with BMS implantation (decreased rate range of 78%–94%) with no cases of in-stent restenosis (as compared to five cases after BMS implantation) Citation28. Although promising, larger studies and long-term data are awaited, especially to evaluate the frequency of hypersensitivity reactions and stent thrombosis with these novel systems.
Elution without a polymer
While using biocompatible or bioabsorbable polymers represents one possibility to reduce hypersensitivity reactions and/or stent thrombosis encountered in first-generation DES systems, avoidance of the polymer altogether represents a potentially even more promising approach to tackle this problem. However, putting a drug on a ‘normal’, unprepared platform does not seem feasible, and several such attempts did not turn out to be efficacious.
On the Yukon® stent, the stainless steel BMS is modified to a microporous surface with 106 pores per cm3, onto which the agent is loaded without the need for a polymer; moreover, drug coating can be performed directly in the catheterization lab with this system, and differential coating of the luminal and of the vessel side of the stent is possible. In the ISAR-TEST study, the mean difference in in-stent late lumen loss between patients treated with a rapamycin-coated Yukon® stent compared with patients treated with a ‘conventional’ paclitaxel stent was 0.002 mm (P = 0.02 for non-inferiority); angiographic restenosis rates (14.2% versus 15.5%) and target lesion revascularization rates due to restenosis (9.3% in both groups) were similar in both groups, consistent with non-inferiority of the Yukon® stent Citation29. Currently, several other studies are ongoing with the same system in higher-risk populations as well as with different drug concentrations.
A similar system is used to elute tacrolimus from a nanoporous aluminium stent; after initially favorable results in animal models Citation30, however, clinical trials demonstrated both lack of safety and efficacy, probably due to particle debris being dislodged from the stent system (PRESENT I & II study) Citation31.
On the V-Flex Plus® coronary stents, paclitaxel is directly applied to the abluminal surface of the stent by a proprietary process. In the ELUTES trial, restenosis was reduced in the highest dose of paclitaxel in the V-Flex Plus group as compared to controls at 6 month angiographic follow-up (33.9% versus 14.2%, P = 0.006), with no difference in MACE and no occurrence of stent thrombosis up to 12 months Citation32.
In the prospective, randomized, blinded, multicenter DELIVER trial, implantation of the non-polymer-based paclitaxel-coated ACHIEVE® stent was compared to BMS implantation. While late loss, restenosis, and target vessel failure were all reduced with the novel system, the results were insufficient to achieve the study's prespecified end point, i.e. 40% reduction in target vessel failure and 50% reduction in binary restenosis of the novel stent system as compared to the BMS Citation33. Taken together, drug elution without the use of a polymer appears feasible, but not all systems will work. Large-scale studies will be necessary to determine the viability of this technology.
Reservoir stents
In first-generation DES, the polymer containing the drug was more or less uniformly spread over the stent. On the one hand, this led to difficulties in deployment due to the ‘stickiness’ of the stent (as compared to ‘naked’ BMS); on the other hand it was uncertain in how far microfragmentation of the polymer and/or the drug occurred and how this affects the distal coronary vasculature. To overcome this drawback, reservoir-containing stents were designed. In theory, a different drug can be loaded on the luminal and on the surface side of the stent allowing for differential drug elution; moreover, different drugs could be loaded into adjacent wells of the same side allowing for dual local drug elution.
The CoStar® stent represents one such stent, in which the agent is loaded in reservoirs and is subsequently released towards both the luminal and abluminal side of the vessel; the drug, paclitaxel, is loaded on a bioresorbable polymer, which is located only within the wells in the stent, resulting in a polymer-free stent surface. Although initial trials were positive, the recently presented results of the COSTAR II study did not meet the prespecified primary end point, but instead demonstrated significantly higher rates of clinically driven TVR (8.1% versus 4.3%, P = 0.002), TLR (6.6% versus 3.1%, P = 0.002) and MACE (11.0% versus 6.9%, P = 0.005) with the use of the CoStar® as compared to the Taxus® stent (data presented at the EuroPCR Meeting 2007). As a result of these outcomes, ongoing clinical trials with the CoStar® stent were terminated, and the submission of the stent's premarket approval application to the Food and Drug Administration (FDA) was not concluded Citation34. The reason for the failure in efficacy may have occurred due to inclusion of more complex lesions and multivessel stenting on the one hand, as well as by insufficient dosage of paclitaxel on the other; further studies will among others examine the efficacy and safety of the same system with elution of sirolimus.
A similar reservoir design is used on the Janus® stent, where the immunosuppressive drug tacrolimus is eluted from wells located on the outer surface of the stent without the use of a polymer. In the JUPITER II trial, implantation of the Janus® stent resulted in similar rates of MACE and TLR as compared to the Technic® BMS; no cases of subacute or late thrombosis occurred in the 327 patients (data presented at the ESC Meeting 2005). A recent study in ‘real-world’ patients showed a reduction in MACE (6% versus 15%, P = 0.038) after Janus stent as compared to BMS implantation after 8 months Citation35.
Coating with pro-endothelial agents (‘bioactive stents’)
As mentioned, one major problem encountered with both conventional bare-metal stents and (possibly even to a slightly higher degree) with first-generation drug-eluting stents is the risk of stent thrombosis. In order to overcome this problem, several novel approaches use coating strategies to enhance endothelial healing thereby reducing thrombogenicity of the stent and, consequently, the risk of stent thrombosis; because the ‘pro-healing’ substances are in fact not eluted from the stent but instead attract endothelial cells, this type of stent may be referred to as a ‘bioactive stent’ Citation36.
One promising approach to diminish the thrombogenicity of the stent is attempted by using titanium-nitride-oxide for coating; in vitro, this setup is associated with diminished platelet adhesion and fibrinogen binding as compared to BMS. In the first human study, performed with an unmounted stent coated with titanium-nitride-oxide and compared to the unmounted bare metal stent, titanium-nitride-oxide coating led to lower late loss (0.55±0.63 versus 0.90±0.76 mm, P = 0.03) and percent diameter stenosis (26%±17% versus 36%±24%, P=0.04) at 6 months as compared to BMS implantation; this translated into a reduced MACE rate in the titanium-nitride-oxide-coated (Tinox) stent group (7% versus 27%, P = 0.02), which was primarily driven by a reduced need for TLR (7% versus 23%, P = 0.07) Citation37. In a second study comparing the titanium-nitride-coated TitanR-stent (Hexacath®) to a paclitaxel-eluting stent system in routine clinical practice, the MACE rate was 0% versus 4.9% 30 days after implantation as compared to the paclitaxel stent; TVR was also significantly lower (0% versus 2.9%), an observation which was apparently driven by less stent thrombosis Citation38. Although the difference in MACE had disappeared by 12 months, there was less myocardial infarction after implantation of the titanium-nitride-oxide-coated stent Citation38.
Endothelial progenitor cells (EPCs) are involved in re-endothelialization after angioplasty Citation39, Citation40. Antiproliferative agents on DES, such as rapamycin, inhibit proliferation, migration, and differentiation of human endothelial progenitor cells in vitroCitation17, Citation41, potentially resulting in impaired endothelial healing. Indeed, a decrease in circulating CD34-positive cells is observed after rapamycin- as compared to bare-metal stent implantation Citation18. In an attempt to promote re-endothelialization, the ‘Genous capture R stent®’ is covered with a CD34-binding antibody to capture endothelial progenitor cells. In a first human trial in 16 patients (HEALING-FIM), the 9-month composite MACE rate was 6.3% despite only 1 month of clopidogrel treatment, and the mean angiographic late luminal loss was 0.63 + / − 0.52 mm by 6 months Citation42. In the HEALING II multicenter trial, in-stent late loss was 0.78 mm at 6 months and 0.59 mm at 18 months (data presented at the TCT Meeting 2005).
A similar approach is used by loading the stent with an integrin-binding cyclic Arg-Gly-Asp peptide to facilitate recruitment of circulating EPCs and, in turn, accelerate re-endothelialization; in a porcine coronary artery stenting model, stents loaded with this peptide indeed reduced neointima formation and enhanced endothelial coverage Citation43. Whether this approach is feasible also in human remains to be determined.
Initially, also estradiol was shown to promote rapid re-endothelialization of coronary stents in animal models. It was consequently used on the ERES® stent in conjunction with rapamycin to both reduce restenosis and accelerate endothelial healing. When compared to a polymer-free rapamycin-eluting stent, however, no difference was observed in late lumen loss, the incidence of binary angiographic restenosis, target lesion revascularization, death and myocardial infarction, or stent thrombosis Citation44.
Coating with other anti-restenotic agents
The application of DES is associated with a marked reduction of restenosis as compared to BMS implantation or PTCA alone. However, even currently available DES do not completely prevent restenosis, and the latter still remains a problem especially after stenting in multivessel disease and complex lesions. Hence, the quest for novel substances which further reduce restenosis is ongoing; in this section, some potential strategies are discussed, most of which, however, are still ‘in development’.
As inflammation plays an important role in acute coronary syndromes, it was conceivable to employ anti-inflammatory agents on DES. The STRIDE study demonstrated the feasibility and safety of a dexamethasone-eluting stent in patients with de novo single-vessel disease with a MACE rate of 3.3% after 6 months and binary restenosis rate of 13.3% Citation45. In a recent study of patients with acute coronary syndromes (ACS), however, implantation of a dexamethasone-eluting stent was not associated with a reduction in TVR, myocardial infarction (MI) and death Citation46.
3-hydroxy-3-methylglutaryl coenzyme A (HMG-CoA)-reductase-inhibitors (statins) have a pronounced antiproliferative effect on vascular smooth muscle cells Citation47; furthermore, they inhibit tissue factor expression in endothelial cells Citation48, and may thus represent an interesting candidate for DES coating. Animal studies assessing the effect of a simvastatin-eluting stent are currently ongoing Citation49.
Mycophenolic acid, like rapamycin, has long been used as an immunosuppressive agent after organ transplantation. In humans with de-novo coronary lesions, implantation of two mycophenolic acid-eluting stents (with different release kinetics) showed no difference as compared to BMS implantation with respect to luminal diameter or MACE Citation50.
Implantation of advanced c-myc antisense (AVI-4126)-eluting stents in a porcine coronary artery restenosis model was associated with complete vascular healing and reduced neointimal formation Citation51. In the AVAIL trial, the rate of restenosis was 39% in the control group, 44% in the low-dose AVI-4126 group, and 18.5% in the high-dose AVI-4126 group (data presented at the AHA Meeting 2004).
Angiopeptin reduces in-stent restenosis in various animal models, and implantation of an angiopeptin-eluting stent appears safe and effective in a small study of patients with native de-novo coronary lesions Citation52.
Dimethyl sulfoxide (DMSO) may also represent an interesting drug for DES coating. We recently demonstrated that DMSO not only prevents vascular smooth muscle cell proliferation and migration, but equally reduces upregulation of tissue factor in endothelial cells, vascular smooth muscle, and monocytes; furthermore, DMSO prevented thrombotic occlusion in vivo in a mouse carotid artery injury model Citation53. As such, DMSO may have the potential to inhibit both neointima formation and stent thrombosis when applied on a drug-eluting stent. Whether these promising first results hold true, however, remains to be assessed in a larger animal model and, eventually, in humans.
Bioabsorbable stents
As the stent itself represents a foreign body in the vasculature with an inherent prothrombogenic potential, employment of a biodegradable stent which dissolves after the initial phase of vessel recoil and constrictive remodeling appears to be a logical and attractive approach. Another advantage of such a stent would be the fact that it would not interfere with non-invasive imaging modalities (in particular computer tomography and magnetic resonance imaging), especially after implantation of a large number of stents (‘full metal jacket’); also, bypass implantation, if required, would potentially be more feasible. Polymeric (e.g. Igaki-Tamai, BVS®, Sahajanand®, REVA®) and metallic bioabsorbable/biodegradable stent systems (e.g. magnesium, iron) have been developed.
Polymeric
The Igaki-Tamai stent, which is constructed from a poly-L-lactic acid polymer, was one of the first bioabsorbable stents employed in humans. In the first clinical study in the year 2000, 25 stents were successfully implanted in 19 lesions in 15 patients with angiographic success in all procedures; on follow-up coronary angiography, both restenosis rate and target lesion revascularization rate per lesion were 10.5%, and the rates per patient were 6.7% at 6 months Citation54, demonstrating the general feasibility of this approach.
In contrast to this passive stent, the BVS® stent releases everolimus from a bioresorbable polylactic acid polymer. In the ABSORB trial, in-stent late loss was 0.44±0.35 mm at 6-month angiographic follow-up after implantation of the BVS® stent; the binary restenosis rate was 11.5%, and there was only one non-Q-wave infarction requiring TVR: (corresponding to a MACE rate of 3.3%); no late stent thromboses were recorded. The percentage of acute stent recoil was 6.85%±6.96%, and acute incomplete apposition was observed in 6 of 26 patients (data presented at the ACC Meeting 2007); after 12 months, no further MACE occurred Citation55.
The principal drawbacks of polymeric biodegradable stents are early recoil after implantation due to their lower radial strength compared to metal stents; with respect to the implantation procedure, both limited flexibility as well as missing radio-opacity can make accurate placement difficult. Furthermore, severe inflammatory reactions have been observed during degradation, which may in turn favor the development of restenosis Citation26. Hence, larger studies, long-term follow-ups of presently available trials, as well as further technical improvements are required.
Metal
To overcome the principal drawbacks of bioresorbable polymeric stents, resorbable metallic stents have been proposed and tested both in preclinical and clinical studies.
The Absorbable Metal Stent (AMS®) is an alloy stent made of 93% magnesium and 7% rare-earth metals, which induces rapid endothelialization, has low thrombogenicity, and a degradation time of 2–3 months. In the first-in-man study (PROGRESS-AMS), its clinical feasibility was assessed for the treatment of a single de-novo lesion in a native coronary artery. A late loss of 1.08 mm (± 0.49 mm) was observed resulting in a 38% rate of ischemia-driven TLR; no cases of cardiac death, non-fatal MI, or stent thrombosis were reported, and the primary study end point (MACE < 30%) was met with a MACE rate of 23.8% at 4 months. At 12 months, the MACE rate had risen to 26.7% Citation56. The 45% rate of all TLR, however, was rather high and remained in the range of that seen with balloon angioplasty alone; hence, the current version of this system, although apparently safe and technically applicable in patients, requires modifications in order to increase its efficacy, including a prolongation of degradation time of the stent (in order to reduce TLR rates) and/or drug elution.
Another option is the use of absorbable iron as a stent platform, which has been successfully tested in animal models Citation57.
Conclusions
Despite the success of first-generation DES (Cypher®, Taxus®) in reducing restenosis and the need for target vessel revascularization, several weaknesses have arisen since their first application, including the risk of stent thrombosis, undesired effects due to the stent polymer as well as the stent itself, and incomplete inhibition of restenosis (especially in complex lesions). Several novel approaches have been introduced to overcome these limitations (summarized in ); while most of the above-mentioned modalities are still under investigation, others have already reached daily clinical application. Many of these innovative novel principles intuitively seem appealing and demonstrate good results in initial clinical evaluations; however, as most of the clinical trials have only been performed in a small number of highly selected patients, large-scale studies as well as long-term follow-up will be required before it is possible to determine their place in the field of interventional cardiology. The need for such investigations was strikingly demonstrated in first-generation DES, as their potential of causing (late) stent thrombosis only became evident after evaluation of several large-scale studies and registries in ‘real-world’ patients. Until such studies are available with the new stent systems, caution with respect to their long-term efficacy and safety is warranted.
Outlook—‘the ideal stent’
The ideal drug-eluting stent entirely inhibits restenosis while at the same time expediting re-endothelialization, all on the basis of a bioabsorbable (and fully biocompatible) polymer. Of the systems currently investigated, elution of an anti-restenotic agent combined with a ‘pro-healing’ platform to enhance re-endothelialization appears to be one of the possible and feasible options. It is moreover conceivable that different types of stents could be used depending on the specific clinical situation in which they are needed: for example, particularly enhanced biocompatibility (e.g. by the use of special elution profiles and/or combination of different polymers) are warranted in complex, small, and long lesions as encountered in diabetics in order not to elicit a severe inflammatory response; on the other hand, a stent placed in the setting of an acute coronary syndrome would first and foremost need to possess potent anti-inflammatory as well as potent antithrombotic properties owing to the highly inflammatory and prothrombotic environment encountered in this situation Citation58. Overall, the wealth of innovative novel developments in the field of DES promises to yield exciting future treatment modalities for interventional cardiology.
Acknowledgements
Research by the authors was supported by the Swiss National Research Foundation (grant No. 3100-068118.02/1 to TFL and 3200B0-113328/1 to FCT) as well as by the European Union (grant no. G5RD-CT-2001-00532 to TFL), the Swiss Heart Foundation, the Wolfermann-Nägeli Foundation, the Bonizzi-Theler Foundation, the Velux Foundation, and the Center for Integrative Human Physiology of the University of Zurich. The authors received unrestricted research grants from Cordis, Biotronik, Boston Scientific, and Medtronic (TFL and FRE). Drs. Lüscher and Tanner are co-holders of a patent on the potential clinical applications of dimethyl sulfoxide.
References
- Horlitz M, Sigwart U, Niebauer J. Fighting restenosis after coronary angioplasty: contemporary and future treatment options. Int J Cardiol. 2002; 83: 199–205
- Serruys PW, de Jaegere P, Kiemeneij F, Macaya C, Rutsch W, Heyndrickx G, et al. A comparison of balloon-expandable-stent implantation with balloon angioplasty in patients with coronary artery disease. Benestent Study Group. N Engl J Med. 1994; 331: 489–95
- Fischman DL, Leon MB, Baim DS, Schatz RA, Savage MP, Penn I, et al. A randomized comparison of coronary-stent placement and balloon angioplasty in the treatment of coronary artery disease. Stent Restenosis Study Investigators. N Engl J Med. 1994; 331: 496–501
- Morice MC, Serruys PW, Sousa JE, Fajadet J, Ban Hayashi E, Perin M, et al. A randomized comparison of a sirolimus-eluting stent with a standard stent for coronary revascularization. N Engl J Med. 2002; 346: 1773–80
- Moses JW, Leon MB, Popma JJ, Fitzgerald PJ, Holmes DR, O'Shaughnessy C, et al. Sirolimus-eluting stents versus standard stents in patients with stenosis in a native coronary artery. N Engl J Med. 2003; 349: 1315–23
- Shaknovich A. Complications of coronary stenting. Coron Artery Dis. 1994; 5: 583–9
- McFadden EP, Stabile E, Regar E, Cheneau E, Ong AT, Kinnaird T, et al. Late thrombosis in drug-eluting coronary stents after discontinuation of antiplatelet therapy. Lancet. 2004; 364: 1519–21
- Stone GW, Moses JW, Ellis SG, Schofer J, Dawkins KD, Morice MC, et al. Safety and efficacy of sirolimus- and paclitaxel-eluting coronary stents. N Engl J Med. 2007; 356: 998–1008
- Camenzind, E, Steg, PG, Wijns, W. Stent thrombosis late after implantation of first-generation drug-eluting stents: a cause for concern. Circulation. 2007;115:1440–55; discussion 1455.
- Luscher TF, Steffel J, Eberli FR, Joner M, Nakazawa G, Tanner FC, et al. Drug-eluting stent and coronary thrombosis: biological mechanisms and clinical implications. Circulation. 2007; 115: 1051–8
- Iakovou I, Schmidt T, Bonizzoni E, Ge L, Sangiorgi GM, Stankovic G, et al. Incidence, predictors, and outcome of thrombosis after successful implantation of drug-eluting stents. JAMA. 2005; 293: 2126–30
- Ong AT, McFadden EP, Regar E, de Jaegere PP, van Domburg RT, Serruys PW. Late angiographic stent thrombosis (LAST) events with drug-eluting stents. J Am Coll Cardiol. 2005; 45: 2088-9-2
- Spaulding C, Daemen J, Boersma E, Cutlip DE, Serruys PW. A pooled analysis of data comparing sirolimus-eluting stents with bare-metal stents. N Engl J Med. 2007; 356: 989–97
- Mauri L, Hsieh WH, Massaro JM, Ho KK, D'Agostino R, Cutlip DE. Stent thrombosis in randomized clinical trials of drug-eluting stents. N Engl J Med. 2007; 356: 1020–9
- Marx SO, Jayaraman T, Go LO, Marks AR. Rapamycin-FKBP inhibits cell cycle regulators of proliferation in vascular smooth muscle cells. Circ Res. 1995; 76: 412–7
- Poon M, Marx SO, Gallo R, Badimon JJ, Taubman MB, Marks AR. Rapamycin inhibits vascular smooth muscle cell migration. J Clin Invest. 1996; 98: 2277–83
- Butzal M, Loges S, Schweizer M, Fischer U, Gehling UM, Hossfeld DK, et al. Rapamycin inhibits proliferation and differentiation of human endothelial progenitor cells in vitro. Exp Cell Res. 2004; 300: 65–71
- Inoue T, Sata M, Hikichi Y, Sohma R, Fukuda D, Uchida T, et al. Mobilization of CD34-positive bone marrow-derived cells after coronary stent implantation: impact on restenosis. Circulation. 2007; 115: 553–61
- Steffel J, Latini RA, Akhmedov A, Zimmermann D, Zimmerling P, Luscher TF, et al. Rapamycin, but not FK-506, increases endothelial tissue factor expression: implications for drug-eluting stent design. Circulation. 2005; 112: 2002–11
- Stahli BE, Camici GG, Steffel J, Akhmedov A, Shojaati K, Graber M, et al. Paclitaxel enhances thrombin-induced endothelial tissue factor expression via c-Jun terminal NH2 kinase activation. Circ Res. 2006; 99: 149–55
- Joner M, Finn AV, Farb A, Mont EK, Kolodgie FD, Ladich E, et al. Pathology of drug-eluting stents in humans: delayed healing and late thrombotic risk. J Am Coll Cardiol. 2006; 48: 193–202
- Nebeker JR, Virmani R, Bennett CL, Hoffman JM, Samore MH, Alvarez J, et al. Hypersensitivity cases associated with drug-eluting coronary stents: a review of available cases from the Research on Adverse Drug Events and Reports (RADAR) project. J Am Coll Cardiol. 2006; 47: 175–81
- Hara H, Nakamura M, Palmaz JC, Schwartz RS. Role of stent design and coatings on restenosis and thrombosis. Adv Drug Deliv Rev. 2006; 58: 377–86
- Fajadet J, Wijns W, Laarman GJ, Kuck KH, Ormiston J, Munzel T, et al. Randomized, double-blind, multicenter study of the Endeavor zotarolimus-eluting phosphorylcholine-encapsulated stent for treatment of native coronary artery lesions: clinical and angiographic results of the ENDEAVOR II trial. Circulation. 2006; 114: 798–806
- Kandzari DE, Leon MB, Popma JJ, Fitzgerald PJ, O'Shaughnessy C, Ball MW, et al. Comparison of zotarolimus-eluting and sirolimus-eluting stents in patients with native coronary artery disease: a randomized controlled trial. J Am Coll Cardiol. 2006; 48: 2440–7
- van der Giessen WJ, Lincoff AM, Schwartz RS, van Beusekom HM, Serruys PW, Holmes DR, Jr, et al. Marked inflammatory sequelae to implantation of biodegradable and nonbiodegradable polymers in porcine coronary arteries. Circulation. 1996; 94: 1690–7
- Costa RA, Lansky AJ, Abizaid A, Mueller R, Tsuchiya Y, Mori K, et al. Angiographic results of the first human experience with the Biolimus A9 drug-eluting stent for de novo coronary lesions. Am J Cardiol. 2006; 98: 443–6
- Tsuchiya Y, Lansky AJ, Costa RA, Mehran R, Pietras C, Shimada Y, et al. Effect of everolimus-eluting stents in different vessel sizes (from the pooled FUTURE I and II trials). Am J Cardiol. 2006; 98: 464–9
- Mehilli J, Kastrati A, Wessely R, Dibra A, Hausleiter J, Jaschke B, et al. Randomized trial of a nonpolymer-based rapamycin-eluting stent versus a polymer-based paclitaxel-eluting stent for the reduction of late lumen loss. Circulation. 2006; 113: 273–9
- Wieneke H, Dirsch O, Sawitowski T, Gu YL, Brauer H, Dahmen U, et al. Synergistic effects of a novel nanoporous stent coating and tacrolimus on intima proliferation in rabbits. Catheter Cardiovasc Interv. 2003; 60: 399–407
- Grube E, Buellesfeld L. Rapamycin analogs for stent-based local drug delivery. Everolimus- and tacrolimus-eluting stents. Herz. 2004; 29: 162–6
- Gershlick A, De Scheerder I, Chevalier B, Stephens-Lloyd A, Camenzind E, Vrints C, et al. Inhibition of restenosis with a paclitaxel-eluting, polymer-free coronary stent: the European evaLUation of pacliTaxel Eluting Stent (ELUTES) trial. Circulation. 2004; 109: 487–93
- Lansky AJ, Costa RA, Mintz GS, Tsuchiya Y, Midei M, Cox DA, et al. Non-polymer-based paclitaxel-coated coronary stents for the treatment of patients with de novo coronary lesions: angiographic follow-up of the DELIVER clinical trial. Circulation. 2004; 109: 1948–54
- Conor Medsystems, LLC Reports COSTAR II Pivotal Drug-Eluting Stent Trial Conclusions. Available at: http://www.jnj.com/news/jnj_news/20070507_080915.htm, , (accessed 6 July 2007).
- Han YL, Wang SL, Jing QM, Yu HB, Wang B, Ma YY, et al. Midterm outcomes of prospective, randomized, single-center study of the Janus tacrolimus-eluting stent for treatment of native coronary artery lesions. Chin Med J (Engl) 2007; 120: 552–6
- Windecker S, Billinger M, Hess OM. Stent coating with titanium-nitric-oxide for prevention of restenosis. EuroIntervention. 2006; 2: 146–8
- Windecker S, Simon R, Lins M, Klauss V, Eberli FR, Roffi M, et al. Randomized comparison of a titanium-nitride-oxide-coated stent with a stainless steel stent for coronary revascularization: the TiNOX trial. Circulation. 2005; 111: 2617–22
- Karjalainen PP, Ylitalo A, Airaksinen JK. Titanium and nitride oxide-coated stents and paclitaxel-eluting stents for coronary revascularization in an unselected population. J Invasive Cardiol. 2006; 18: 462–8
- Urao N, Okigaki M, Yamada H, Aadachi Y, Matsuno K, Matsui A, et al. Erythropoietin-mobilized endothelial progenitors enhance reendothelialization via Akt-endothelial nitric oxide synthase activation and prevent neointimal hyperplasia. Circ Res. 2006; 98: 1405–13
- Griese DP, Ehsan A, Melo LG, Kong D, Zhang L, Mann MJ, et al. Isolation and transplantation of autologous circulating endothelial cells into denuded vessels and prosthetic grafts: implications for cell-based vascular therapy. Circulation. 2003; 108: 2710–5
- Chen TG, Chen JZ, Wang XX. Effects of rapamycin on number activity and eNOS of endothelial progenitor cells from peripheral blood. Cell Prolif. 2006; 39: 117–25
- Aoki J, Serruys PW, van Beusekom H, Ong AT, McFadden EP, Sianos G, et al. Endothelial progenitor cell capture by stents coated with antibody against CD34: the HEALING-FIM (Healthy Endothelial Accelerated Lining Inhibits Neointimal Growth-First In Man) Registry. J Am Coll Cardiol. 2005; 45: 1574–9
- Blindt R, Vogt F, Astafieva I, Fach C, Hristov M, Krott N, et al. A novel drug-eluting stent coated with an integrin-binding cyclic Arg-Gly-Asp peptide inhibits neointimal hyperplasia by recruiting endothelial progenitor cells. J Am Coll Cardiol. 2006; 47: 1786–95
- Adriaenssens T, Mehilli J, Wessely R, Ndrepepa G, Seyfarth M, Wieczorek A, et al. Does addition of estradiol improve the efficacy of a rapamycin-eluting stent? Results of the ISAR-PEACE randomized trial. J Am Coll Cardiol. 2007; 49: 1265–71
- Liu, X, Huang, Y, Hanet, C, Vandormael, M, Legrand, V, Dens, J, , et al. Study of antirestenosis with the BiodivYsio dexamethasone-eluting stent (STRIDE): a first-in-human multicenter pilot trial. Catheter Cardiovasc Interv. 2003;60:172–8; discussion 179.
- Ribichini F, Tomai F, Paloscia L, Di Sciascio G, Carosio G, Romano M, et al. Steroid-eluting stents in patients with acute coronary syndrome: the dexamethasone eluting stent Italian registry. Heart. 2007; 93: 598–600
- Lefer AM, Scalia R, Lefer DJ. Vascular effects of HMG CoA-reductase inhibitors (statins) unrelated to cholesterol lowering: new concepts for cardiovascular disease. Cardiovasc Res. 2001; 49: 281–7
- Eto M, Kozai T, Cosentino F, Joch H, Luscher TF. Statin prevents tissue factor expression in human endothelial cells: role of Rho/Rho-kinase and Akt pathways. Circulation. 2002; 105: 1756–9
- Vaina S, Serruys PW. Progressive stent technologies: new approaches for the treatment of cardiovascular diseases. Expert Opin Drug Deliv. 2006; 3: 783–97
- Abizaid A, Albertal M, Ormiston J, Londero H, Ruygrok P, Seixas AC, et al. IMPACT Trial: angiographic and intravascular ultrasound observations of the first human experience with mycophenolic acid-eluting polymer stent system. Catheter Cardiovasc Interv. 2005; 66: 491–5
- Kipshidze NN, Iversen P, Kim HS, Yiazdi H, Dangas G, Seaborn R, et al. Advanced c-myc antisense (AVI-4126)-eluting phosphorylcholine-coated stent implantation is associated with complete vascular healing and reduced neointimal formation in the porcine coronary restenosis model. Catheter Cardiovasc Interv. 2004; 61: 518–27
- Kwok OH, Chow WH, Law TC, Chiu A, Ng W, Lam WF, et al. First human experience with angiopeptin-eluting stent: a quantitative coronary angiography and three-dimensional intravascular ultrasound study. Catheter Cardiovasc Interv. 2005; 66: 541–6
- Camici GG, Steffel J, Akhmedov A, Schafer N, Baldinger J, Schulz U, et al. Dimethyl sulfoxide inhibits tissue factor expression, thrombus formation, and vascular smooth muscle cell activation: a potential treatment strategy for drug-eluting stents. Circulation. 2006; 114: 1512–21
- Tamai H, Igaki K, Kyo E, Kosuga K, Kawashima A, Matsui S, et al. Initial and 6-month results of biodegradable poly-l-lactic acid coronary stents in humans. Circulation. 2000; 102: 399–404
- Ormiston JA, Serruys PW, Regar E, Dudek D, Thuesen L, Webster MW, et al. A bioabsorbable everolimus-eluting coronary stent system for patients with single de-novo coronary artery lesions (ABSORB): a prospective open-label trial. Lancet. 2008; 371: 899–907
- Erbel R, Di Mario C, Bartunek J, Bonnier J, de Bruyne B, Eberli FR, et al. Temporary scaffolding of coronary arteries with bioabsorbable magnesium stents: a prospective, non-randomised multicentre trial. Lancet. 2007; 369: 1869–75
- Peuster M, Wohlsein P, Brugmann M, Ehlerding M, Seidler K, Fink C, et al. A novel approach to temporary stenting: degradable cardiovascular stents produced from corrodible metal-results 6-18 months after implantation into New Zealand white rabbits. Heart. 2001; 86: 563–9
- Steffel J, Luscher TF, Tanner FC. Tissue factor in cardiovascular diseases: molecular mechanisms and clinical implications. Circulation. 2006; 113: 722–31