Abstract
The renin-angiotensin-aldosterone system is known to play an essential role in controlling sodium balance and body fluid volumes, and thus blood pressure. In addition to the circulating system which regulates urgent cardiovascular responses, a tissue-localized renin-angiotensin system (RAS) regulates long-term changes in various organs. Many recognized RAS components have also been identified in the human eye. The highly vasoconstrictive angiotensin II (Ang II) is considered the key peptide in the circulatory RAS. However, the ultimate effect of RAS activation at tissue level is more complex, being based not only on the biological activity of Ang II but also on the activities of other products of angiotensinogen metabolism, often exerting opposite effects to Ang II action. In recent studies, orally administered angiotensin II type 1 receptor blockers and angiotensin-converting enzyme inhibitors lower intra-ocular pressure (IOP), likewise topical application of these compounds, the effect being more prominent in ocular hypertensive eyes. Based on previous findings and our own experimental data, it can strongly be suggested that the RAS not only regulates blood pressure but is also involved in the regulation of IOP.
Introduction
Open angle glaucoma (OAG) is one of the major causes of vision loss worldwide Citation1, Citation2. There will be 60.5 million people with glaucoma in 2010, increasing to 79.6 million by 2020, and, of these, 74% will have OAG Citation3. Among many predictors like age, cup-disc ratio of papilla, thickness of cornea Citation4, the major known risk factor for glaucoma is increased intra-ocular pressure (IOP), which is a net sum of the homeostatic balance of aqueous humour formation and outflow. A reduction in IOP by 30% reduces disease progression from about 10% to 33%, even in normal tension glaucoma patients Citation5, Citation6. Ocular hypotensive medication has been shown to prevent the onset of glaucoma in individuals with elevated IOP Citation7. Current pharmacotherapy comprises of drugs acting on adrenergic α- and β-receptors or on cholinergic muscarine receptors, prostaglandin analogues, and carbonic anhydrase inhibitors. They are administered mainly topically and targeted either to reduce the formation of aqueous humour in the ciliary body or to increase outflow through uveoscleral pathways. Evidence is accumulating to suggest that widely used antihypertensive drugs acting on the renin-angiotensin system (RAS) can also lower IOP. In recent human studies orally administered losartan (angiotensin type 1 receptor blocker (ARB)) Citation8 and captopril (angiotensin-converting enzyme (ACE) inhibitor) Citation9 are able to lower IOP in both normotensive and glaucomatous subjects, although blood pressure is reduced in only arterial hypertensive patients. Topical application of olmesartan (ARB) Citation10, Citation11, ACE Citation12, Citation13 and renin inhibitors Citation14 has been reported to lower IOP in animal studies. Topical ACE inhibitors have also been shown to prevent the visual field defect progression in the normotensive glaucoma Citation15. The effect is more prominent in ocular hypertensive animals Citation10, Citation11. Based on such findings it can strongly be suggested that the RAS not only regulates blood pressure but is also involved in the regulation of IOP. However, the exact mechanism of this action is yet unclear.
Abbreviations
In addition to the essential role of circulating RAS and aldosterone (RAAS) in controlling sodium balance, body fluid volumes, and hence blood pressure Citation16, Citation17, there is a tissue-localized RAS Citation18, Citation19 which regulates long-term changes in a number of organs. A local RAS has been demonstrated in the vasculature as well as in the adrenal gland, kidney, brain, testis, and ovary Citation20, Citation21 and also in the eye Citation22–26. The final effect of RAS activation is complex, being based on the biological activity of angiotensin II (Ang II) and the activities of the other products of angiotensinogen metabolism, often exerting opposite effects on Ang II action Citation19. In this review we first describe the plasma-localized RAS and then concentrate on the local RAS, especially in the anterior part of the eye.
The renin-angiotensin system: circulating RAS and tissue RAS
History
The scientists Tiegerstedt (Finnish) and Bergman found in 1898 that crude saline extracts from the kidney contained a pressor substance which they named renin. In 1934 Goldblatt and co-workers demonstrated that constriction of the renal arteries produced persistent hypertension in dogs. In 1940 groups under Braun-Menéndez and Page reported that renin was an enzyme acting on a plasma protein substrate to catalyse the formation of the actual pressor material, a peptide, first named hypertensin or angiotonin. Later the pressor substance was renamed angiotensin and the plasma substrate angiotensinogen. In 1958 Gross suggested that the renin-angiotensin system was involved in the regulation of aldosterone secretion in the kidneys. It was found that renin secretion increased with depletion of Na+. Thus the renin-angiotensin system came to be recognized as a mechanism to stimulating aldosterone synthesis and secretion and an important homeostatic mechanism in the regulation of blood pressure and electrolyte composition. In the 1970s the development of antihypertensive drugs started: first via inhibition of angiotensin II formation (ACE inhibitors) and later via angiotensin II receptor type 1 blocking Citation16, Citation17.
Key messages
Many recognized renin-angiotensin system (RAS) components have been identified in the human eye.
Evidence is accumulating to indicate that antihypertensive drugs antagonizing the RAS can also reduce intra-ocular pressure.
Compounds blocking RAS may prove to be potential antiglaucomatous drugs.
Our recent findings suggest the potentials of agents which increase angiotensin-converting enzyme-related carboxypeptidase (ACE2) activity and formation of angiotensin (1-7) or activate Mas receptors.
Circulating RAS
The complexity of the present knowledge of RAS is described in . The obligatory substrate of the RAS is angiotensinogen, an α-glycoprotein which is synthesized in and released from the liver and cleaved in the circulation by the enzyme renin secreted from the juxtaglomerular apparatus of the kidney to form the decapeptide angiotensin I (Ang I) Citation16, Citation18, Citation19. Importantly, renin has an inactive precursor, prorenin. Prorenin is released constitutively from the kidney, its plasma levels are higher than those of renin, and its action on RAS is probably marked not only via renin but also via renin receptor Citation27. Ang I is a weak vasoconstrictor and is cleaved to the more potent octapeptide Ang II by the ACE, membrane-bound proteinase which is predominantly expressed in high concentrations on the surface of endothelial cells in the pulmonary circulation Citation18. ACE is also known as kininase II; it has in addition a catalysing effect on the bradykinin cascade Citation28 and thus a degrading effect on the highly vasodilatative bradykinin Citation19.
Figure 1. Tissue RAS-angiotensin synthesis pathways. (ACE = angiotensin-converting enzyme; ACE2 = angiotensin-converting enzyme-related carboxypeptidase; Ang I,II,III,IV = angiotensin I,II,III,IV; Ang (1-10) = angiotensin (1-10); Ang (1-8) = angiotensin (1-8); Ang (2-8) = angiotensin (2-8); Ang (3-8) = angiotensin (3-8); Ang (1-9) = angiotensin (1-9); Ang (1-7) = angiotensin (1-7); Ang (1-5) = angiotensin (1-5); Ang (3-7) = angiotensin (3-7); AT1 = angiotensin II type 1 receptor; AT2 = angiotensin II type 2 receptor; AT4 = angiotensin II type 4 receptor; AP = aminopeptidase (-A,-N,-M,-B); B1/B2 = bradykinin receptors; CAGE = chymostatin-sensitive Ang II generating enzyme; Mas receptor = Ang (1-7) receptor type; Nep = neprilysin; PEP = prolyl endopeptidase; PCP = prolylcarboxypeptidase; tPA = tissue-type plasminogen activator.)
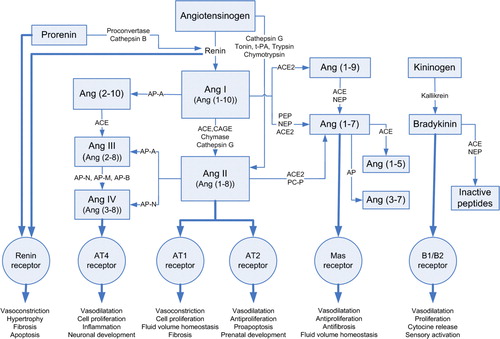
Angiotensin II (Ang II) is a potent vasoconstrictor and is considered the main effector peptide in the RAS. The mechanism of RAS-induced hypertension has generally been attributed to the direct effects of Ang II on Ang type 1 receptors in vascular smooth muscle and stimulation of the release of aldosterone, a mineralocorticoid from the adrenal cortex Citation18, Citation19. Ang II elevates blood pressure by releasing noradrenaline from adrenergic nerve endings, endothelin-1 (a potent vasoconstrictor) from the endothelium, and vasopressin (a vasoconstricting pituitary hormone), as well as by reducing baroreceptor activity Citation29. Additionally, Ang II stimulates free radical production, plasminogen activator inhibitor-1 release, and tissue factor and adhesion molecule expression. Ang II is considered to diminish beneficial effects of nitric oxide by inhibiting nitric oxide synthase. In blood vessels, it stimulates muscle cell proliferation and leukocyte activation Citation16, Citation19, Citation30. The half-life of Ang II is short, a couple of seconds Citation31, but its effects appear rapidly and are long-lasting.
Angiotensin III (Ang III) is formed from Ang II by aminopeptidase A. Similarly to Ang II, Ang III is also a vasoconstrictor, albeit less potent Citation16.
Angiotensin IV (Ang IV) is formed from Ang III or directly from Ang II by aminopeptidase activity. In contrast to Ang II, Ang IV is considered a vasorelaxing agent Citation16. It also has cell-proliferative properties and may be involved in vascular inflammatory responses Citation32. The precise mechanism and function of Ang IV is not clear, but its vasodilatatory effect is explained by activation of endothelial nitric oxide synthase Citation16, Citation19.
Angiotensin (1-9) (Ang (1-9)) is formed from Ang I by angiotensin-converting enzyme-related carboxypeptidase (ACE2). Ang (1-9) has recently been found, and its function is not yet clear, but it is a strong inhibitor of ACE. It enhances the activation of bradykinin, increases nitric oxide and arachidonic acid release, and is possibly involved in the inhibition of platelet function Citation19, Citation33.
Angiotensin (1-7) (Ang (1-7)), formed from Ang II by ACE-independent enzymes Citation19, Citation34–36, is one of the most extensively investigated products of the RAS. It can also be synthesized directly from Ang I or Ang (1-9), bypassing the synthesis of Ang II Citation35. It is a biologically active heptapeptide with high selectivity. In most situations, Ang (1-7) and Ang II exert opposing actions, suggesting a primary role for Ang (1-7) as a counter-regulatory component for the vascular and proliferative actions of Ang II Citation37–40. Ang (1-7) promotes release of prostanoids from endothelial and smooth muscle cells, release of nitric oxide, vasorelaxation, inhibition of vascular cell growth, and, less frequently, vasoconstriction Citation36, Citation41–44. Ang (1-7) also has an important stimulatory interaction with the kallikrein-kinin system, and thus has a vasodilatatory effect. This mechanism is complex, involving receptor activation, inhibition of ACE, and the release of nitric oxide and/or prostaglandins. On the other hand, Ang (1-7) may be part of the endogenous regulators of tissue growth Citation36.
The effects of angiotensins are exerted through specific heptahelical G-protein-coupled receptors Citation16, Citation37. Ang II receptors in the cardiovascular system are classically divided into two main subtypes: Ang type 1 (AT1) and 2 (AT2) receptors. In the rat and mouse, AT1 receptors are further divided into two subtypes, AT1a and AT1b Citation18, Citation37. There is also an AT4 receptor type which is involved especially in cardiovascular pathology Citation32 and the recently discovered Mas receptor Citation38. Most of the known biological effects of Ang II are mediated by the AT1 receptors, which are specifically blocked by AT1 receptor antagonists, widely used as antihypertensive drugs, ‘sartans’. AT2 receptors are less well characterized but are considered cardiovascular protective receptors which antagonize the effects of Ang II mediated via AT1 receptors. AT2 receptors may exert the antiproliferative, proapoptotic, vasodilatatory, and antihypertensive effects of angiotensins, and evidently have an important role in prenatal development Citation16, Citation37. The Mas receptor was first found in the mouse kidney and later in other organs, e.g. heart, brain, and vasculature Citation38, Citation39. Ang (1-7) is considered to be an endogenous ligand for this angiotensin receptor type Citation38, which is distinct from AT1 and AT2 receptors, and through it mediates a number of the positive cardiovascular effects of Ang (1-7).
Tissue RAS
In addition to the circulatory system there is a tissue-localized RAS Citation16, Citation18, Citation19 which regulates long-term changes in different organs. There is thus in tissues a system which can further be divided into an extrinsic and intrinsic RAS Citation13: angiotensin II is derived either from the circulation or from local biosynthesis. In tissues, Ang II production can be catalysed by enzymes other than the classical ACE. These actions are called renin-independent or ACE-independent pathways for Ang II production. Marked species differences in the local Ang II-forming pathways have been reported Citation45–47. Further, there is an important enzyme, ACE2, which can degrade Ang I and Ang II to form the biologically active Ang (1-7) acting in many respects opposite to Ang II. According to literature these alternative pathways are important both in physiological and pathophysiological conditions Citation48, Citation49.
Alternative pathways for angiotensin II biosynthesis
Chymostatin-sensitive Ang II generating enzyme (CAGE)-dependent pathway of Ang II production
CAGE is a protease able to convert Ang I to Ang II. It is found e.g. in human, monkey, and dog aorta distributed predominantly in adventitia, while ACE is found localized mainly in the endothelium. The distribution of these enzymes may reflect distinct functional roles Citation50. The exact role of CAGE in physiology is unknown Citation19.
Chymase-dependent pathway of Ang II production
Chymases (α- and β-chymase) are chymotrypsin-like serine proteases found in the heart, kidney, vascular smooth muscle, and secretory granules of mast cells Citation49, Citation51, Citation52. They are able to cleave Ang I to produce Ang II, but not to form Ang II directly from angiotensinogen Citation51. Chymase-mediated Ang II production may have an important role especially in pathological conditions Citation49, Citation52. Chymase may be associated with the development of diabetic and hypertensive nephropathy Citation53, vascular proliferative diseases Citation54, myocardial infarction Citation55, and atherosclerosis Citation56.
Cathepsin G-dependent pathway of Ang II production
Cathepsin G is a serine protease found in the granules of human neutrophil cells. It is able to convert Ang I to Ang II, but can also produce Ang II directly from angiotensinogen Citation57, Citation58. A serine protease, called tonin Citation59, as well as tissue-type plasminogen activator, trypsin, and chymotrypsin are also able to release Ang II directly from angiotensinogen Citation19, Citation60.
ACE2-dependent pathway of Ang metabolism
The human angiotensin-converting enzyme-related carboxypeptidase (ACE2) is a structurally related homologue of ACE with 42% protein sequence identity Citation33, Citation61, but it acts contrary to the carboxypeptidases, and increase Ang (1-9) and Ang (1-7) formation. Unlike ACE, ACE2 is not able to degrade bradykinin. ACE2 is mainly expressed in cardiac blood vessels, kidneys, and testis Citation33, Citation62. It is considered to be a balancing counter-regulator in the RAS, as it is able to cleave especially the bioactive Ang II to form Ang (1-7), and Ang I to form Ang (1-9) Citation33, Citation63. It is of importance in that both Ang (1-7) and Ang (1-9) have physiological effects opposite to those of Ang II. In the absence of ACE2, the predominant effects of Ang II lead to vasoconstriction and hypertension. In the light of these findings, ACE2 can be regarded as an important modulator of blood pressure Citation63.
Aqueous humour dynamics and IOP
IOP is maintained by a homeostatic balance of formation and outflow of aqueous humour (AH). AH is produced by the ciliary processes at approximately 2–3 µL/min, and the entire volume is replaced every 90–100 min. There are three essential steps in the formation of AH: the blood circulation must be sufficient in the ciliary processes, the plasma must be filtered into tissue spaces, and the filtrate must pass through the double-layered epithelium to enter the posterior chamber Citation64. The widely used oculohypotensive drug timolol maleate reduces the formation of AH via β-adrenergic receptor Citation65, though the exact mechanism here is not known. Carbonic anhydrase inhibitors administered as eye drops or orally also diminish the formation of AH, like the less potent α-adrenergic drugs. AH passes from the posterior chamber through the pupil into the anterior chamber and has several routes to exit from the eye. The main route (90%) of drainage in the normal eye is the route through the trabecular meshwork Citation66. Uveoscleral outflow constitutes approximately 10% of total outflow, but the potent antiglaucomatous prostaglandin analogues act by this route Citation67. The other alternative, albeit minor, pathways of outflow are those through iris vessels, corneal endothelium, or anterior vitreous body. Anticholinergics such as pilocarpine probably increase the trabecular outflow by contraction of the ciliary muscle, which leads to changes in the geometry of the trabecular meshwork.
RAS and the eye
RAS expression
Many of the recognized RAS components have already been identified in the human eye Citation18, Citation22–25, except for the recently described Mas receptor for Ang (1-7) and novel peptidases degrading angiotensins (). Prorenin, the precursor of renin, has been detected in the human non-pigmented ciliary epithelium Citation68. Renin mRNA has been detected in retinal pigment epithelium (but not in the neural retina) and choroid Citation24. Angiotensinogen has been found in the non-pigmented ciliary epithelium Citation23, and its gene expression has been demonstrated in retina and choroid Citation24, Citation25 and in sclera Citation24. ACE has been identified mainly in the human non-pigmented ciliary epithelium Citation25 but also in the retina and choroid. ACE2 is localized in the Müller cells and photoreceptors in the retina Citation69. In animal studies, active chymase has been detected in the anterior uveal tract, choroid and sclera Citation70. In human ocular tissues Ang II receptors (predominantly type 1) are most abundant in the retina. AT1 receptors are found in retinal Müller cells and blood vessels Citation71 and in ganglion cells and the cornea Citation25. AT2 receptors are also localized in Müller cells, in ganglion cells, as well as in the inner nuclear layer of the retina Citation71. Ang II has been detected in many human ocular tissues: in the non-pigmented ciliary epithelium, in cells of the cornea, in epithelial cells of the conjunctiva, and in trabecular meshwork cells, as well as in ganglion cells and photoreceptor cells and in the endothelial cells of retinal and choroidal vessels Citation25. Ang (1-7) has recently been found in the human retina Citation71.
Table I. Localization of the RAS components in intra-ocular tissues of different species. For abbreviations, see text.
There has been debate as to the effects of local RAS in the human eye: does intra-ocular angiotensin originate from local production or from the blood compartment Citation22? It has been shown that neither Ang I, Ang II, nor angiotensinogen is able to pass the blood-brain barrier Citation22, Citation72. The blood-retina barrier in the eye is comparable to this Citation73. If it is intact, circulating angiotensin cannot reach the vitreous fluid Citation22, while if the barrier is disrupted this becomes possible Citation74. It is therefore obvious that the levels of angiotensins and other RAS molecules in the eye are too high to have originated from blood-borne peptides. In porcine ocular tissues Ang I and II levels have proved 5- to 100-fold higher than could be accounted for by admixture with blood or diffusion from blood Citation22. Also ACE activity has been shown to be lower in plasma than in ocular tissues in the rabbit and pig Citation75, Citation76.
Glaucoma
The exact function of the RAS in the eye has not yet been clarified. As mentioned before, RAS activity has been shown in cultured non-pigmented human ciliary epithelial cells, the cells responsible for aqueous humour formation Citation77, Citation78. Ang II has been reported to activate a Ca2 + signalling system which increases potassium ion channel activity and triggers aldosterone production Citation79. These effects are accompanied by cell volume loss, indicating that Ang II acts as an operated secretagogue in the non-pigmented ciliary cells Citation77. Ang II has also been found to cause an increase in cytoplasmic sodium concentration due to activation of Na+/H+ exchange Citation80. In point of fact, mechanisms related to sodium handling are common pathogenetic factors in both ciliary and renal tubular epithelia, which may explain the coexistence of glaucoma and systemic hypertension Citation81.
ACE inhibitors have been shown to lower Ang II levels in aqueous humour Citation82. ACE inhibitors might decrease the production of aqueous humour by reducing blood flow in the ciliary body Citation83. On the other hand, ACE inhibitors promote synthesis of prostaglandins by preventing the breakdown of bradykinin, which in turn could lower IOP by increasing the uveoscleral outflow Citation84, Citation85. The precise mechanism underlying increased uveoscleral outflow is not known, but there would appear to be associations with increased biosynthesis of certain matrix metalloproteinases. This leads to relaxation of the ciliary muscle and reduction and compaction of extracellular matrix components within the ciliary muscle, the iris, and the sclera, and within tissues of the uveoscleral outflow pathway. All these effects might facilitate aqueous humour outflow and thus lower IOP Citation86. By preventing bradykinin breakdown, ACE inhibitors activate the nitric oxide pathway and reduce the formation of the vasoconstrictive peptide, endothelin-1. Endothelin-1 has been shown to elicit contraction e.g. in porcine ophthalmic and ciliary arteries and the human ophthalmic artery Citation87, Citation88.
There is as yet only limited evidence regarding the role of the RAS in aqueous humour outflow, but Ang II has been reported to be able to induce cell proliferation in bovine trabecular meshwork cells and increase the synthesis of collagen in vitro Citation89. It has been reported that Ang II administered intracamerally diminishes uveoscleral outflow Citation90. On the other hand, synthetic and natural Ang II have been reported to reduce IOP in in vivo studies with anaesthetized cats when administered intravenously Citation91. The same IOP-lowering effect was seen in the enucleated, arterially perfused cat and human eye. The mechanism behind the effect was considered to consist in vasoconstriction of the iris artery.
Our own studies
We evaluated the effects of exogenous angiotensin II and its breakdown metabolite, angiotensin (1-7) on IOP and on aqueous humour dynamics in ocular normotensive rabbits Citation92. Administered topically these two peptides did not alter IOP in conscious rabbits during 6 hours. However, intravitreally administered Ang (1-7) reduced IOP for 5 hours (P<0.05). This effect was abolished by a selective angiotensin (1-7) antagonist A-779, and partially by the selective angiotensin II type 2 receptor antagonist PD 123,319. On the other hand, in our outflow studies conducted by a two-level constant pressure method Citation93, Ang II reduced outflow facility (P<0.01) dose-dependently, while angiotensin (1-7) had no effect. Based on these findings, we propose that Ang (1-7) reduces IOP possibly via the recently described Mas receptors and probably via decrease of production of aqueous humour. Preliminary data indicate that also in rabbits with congenitally elevated IOP the oculohypotensive effect of Ang (1-7) is more pronounced than in normotensive animals (Vaajanen et al., unpublished data). We have also demonstrated very recently the expression of Mas receptor in the rat eye for the first time, but the precise localization is still under investigation (Vaajanen et al., unpublished data).
There are controversial findings on the relationship between high systemic blood pressure and IOP in man. We found a significant relationship between the development of hypertension and IOP in spontaneously hypertensive rats, a widely used animal model of human essential hypertension, during an 8-week follow-up Citation94. In double transgenic rats harbouring human renin and human angiotensinogen genes, arterial hypertension developed rapidly together with high IOP. In this study antihypertensive treatment with ARB appeared to reduce IOP slightly parallel to a lowering of blood pressure.
Future possibilities for drug treatment of glaucoma
Evidence is now accumulating to indicate that antihypertensive drugs acting on the RAS can also reduce IOP, and compounds blocking RAS may prove to be potential antiglaucomatous drugs in the future. Our recent findings suggest the potentials of agents increasing ACE2 activity and the formation of angiotensin (1-7) or activating Mas receptors. In addition to the oculohypotensive effects of ACE inhibitors and of AT1 receptor antagonists, chymase, an alternative Ang II-generating enzyme, may also influence the regulation of IOP. Intra-ocular chymase injection has resulted in an increase in IOP in rabbits, which effect was attenuated by a specific chymase inhibitor Citation95. The exact mechanism by which compounds acting on the RAS reduce IOP is not fully understood. In addition to their ocular hypotensive effect, blockade of the ocular RAS may also exert a neuroprotective effect in glaucoma, since angiotensin-induced vasoconstriction of ocular blood vessels has been considered a pathogenic mechanism in optic nerve damage Citation96. Compounds acting on the RAS may also have a potential in the treatment and prevention of diabetic retinopathy, a leading cause Citation97 of blindness in people of working age.
Summary
Compounds acting on the RAS may prove to be potential antiglaucomatous drugs. Especially agents increasing ACE2 activity and the formation of angiotensin (1-7) or activating Mas receptors are new options, in addition to classical ACE inhibitors and Ang II receptor type 1 blockers. However, the nature of the present experimental compounds amplifies significant pharmacokinetic challenges in the penetration to the inner parts of the eye.
Acknowledgements
This work was supported by grants from the Foundation of Päivikki and Sakari Sohlberg, Helsinki, Finland and the Eye Foundation Helsinki, Finland. We thank Ms Jaana Tuure for her secretarial help.
References
- Wu SY, Nemesure B, Leske MC. Observed versus indirect estimates of incidence of open-angle glaucoma. Am J Epidemiol. 2001; 15: 184–7
- Kroese M, Burton H, Vardy S, Rimmer T, McCarter D. Prevalence of primary open angle glaucoma in general ophthalmic practice in the United Kingdom. Br J Ophthalmol. 2002; 86: 978–80
- Quigley HA, Broman AT. The number of people with glaucoma worldwide in 2010 and 2020. Br J Ophthalmol. 2006; 90: 262–7
- Gordon MO, Beiser JA, Brandt JD, Heuer DK, Higginbotham EJ, Johnson CA, et al. The Ocular Hypertension Treatment Study: baseline factors that predict the onset of primary open-angle glaucoma. Arch Ophthalmol. 2002; 120: 714–20
- Comparison of glaucomatous progression between untreated patients with normal tension glaucoma and patients with therapeutically reduced intraocular pressures. Collaborative Normal-Tension Glaucoma Study Group. Am J Ophthalmol. 1998;126:487–97.
- The effectiveness of intraocular pressure reduction in the treatment of normal-tension glaucoma. Collaborative Normal-Tension Glaucoma Study Group. Am J Ophthalmol. 1998;126:498–505.
- Hirooka K, Baba T, Fujimura T, Shiraga F. Prevention of visual field defect progression with angiotensin-converting enzyme inhibitor in eyes with normal-tension glaucoma. Am J Ophthalmol. 2006; 142: 523–5
- Costagliola C, Verolino M, de Rosa ML, Iaccarino G, Ciancaglini M, Mastropasqua L. Effect of oral losartan potassium on intraocular pressure in normotensive and glaucomatous human subjects. Exp Eye Res. 2000; 71: 167–71
- Costagliola C, Di Benedetto R, De Caprio L, Verde R, Mastropasqua L. Effect of oral captopril (SQ 14225) on intraocular pressure in man. Eur J Ophthalmol. 1995; 5: 19–25
- Wang RF, Podos SM, Mittag TW, Yokoyoma T. Effect of CS-088, an angiotensin AT1 receptor antagonist, on intraocular pressure in glaucomatous monkey eyes. Exp Eye Res. 2005; 80: 629–32
- Inoue T, Yokoyoma T, Mori Y, Sasaki Y, Hosokawa T, Yanagisawa H, et al. The effect of topical CS-088, an angiotensin AT1 receptor antagonist, on intraocular pressure and aqueous humor dynamics in rabbits. Curr Eye Res. 2001; 23: 133–8
- Shah GB, Sharma S, Mehta AA, Goyal RK. Oculohypotensive effect of angiotensin-converting enzyme inhibitors in acute and chronic models of glaucoma. J Cardiovasc Pharmacol. 2000; 36: 169–75
- Watkins RW, Baum T, Cedeno K, Smith EM, Yuen PH, Ahn HS, et al. Topical ocular hypotensive effects of the novel angiotensin converting enzyme inhibitor SCH 33861 in conscious rabbits. J Ocul Pharmacol. 1987; 3: 295–307
- Giardina WJ, Kleinert HD, Ebert DM, Wismer CT, Chekal MA, Stein HH. Intraocular pressure lowering effects of the renin inhibitor ABBOTT-64662 diacetate in animals. J Ocul Pharmacol. 1990; 6: 75–83
- Kass MA, Heuer DK, Higginbotham EJ, Johnson CA, Keltner JL, Miller JP, et al. The Ocular Hypertension Treatment Study: a randomized trial determines that topical ocular hypotensive medication delays or prevents the onset of primary open-angle glaucoma. Arch Ophthalmol. 2002; 120: 701–13
- Jackson KE. Renin and Angiotensin. Goodman & Gilman's The pharmacological basis of therapeutics, JG Hardman, LE Limbird. McGraw-Hill, Medical Publishing Division, New York 2001; 809–42
- Hall JE. Historical perspective of the renin-angiotensin system. Mol Biotechnol. 2003; 24: 27–39
- Paul M, Poyan Mehr A, Kreutz R. Physiology of local renin-angiotensin systems. Review Physiol Rev. 2006; 86: 747–803
- Kramkowski K, Mogielnicki A, Buczko W. The physiological significance of the alternative pathways of angiotensin II production. J Physiol Pharmacol. 2006; 57: 529–39
- Deschepper CF, Mellon SH, Cumin F, Baxter JD, Ganong WF. Analysis by immunocytochemistry and in situ hybridization of renin and its mRNA in kidney, testis, adrenal, and pituitary of the rat. Proc Natl Acad Sci U S A. 1986; 83: 7552–6
- Derkx FH, Alberda AT, Zeilmaker GH, Schalekamp MA. High concentrations of immunoreactive renin, prorenin and enzymatically-active renin in human ovarian follicular fluid. Br J Obstet Gynaecol. 1987; 94: 4–9
- Danser AHJ, Derkx FHM, Admiraal PJJ, Deinum J, de Jong PTVM, Schalekamp MADH. Angiotensin levels in the eye. Invest Ophthalmol Vis Sci. 1994; 35: 1008–18
- Sramek SJ, Wallow IHL, Tewksbury DA, Brandt CR, Poulsen GL. An ocular renin-angiotensin system. Immunohistochemistry of angiotensinogen. Invest Ophthalmol Vis Sci. 1992; 33: 1627–32
- Wagner J, Jan Danser AH, Derkx FH, de Jong TV, Paul M, Mullins JJ, et al. Demonstration of renin mRNA, angiotensinogen mRNA, and angiotensin converting enzyme mRNA expression in the human eye: evidence for an intraocular renin-angiotensin system. Br J Ophthalmol. 1996; 80: 159–63
- Savaskan E, Loffler KU, Meier F, Muller-Spahn F, Flammer J, Meyer P. Immunohistochemical localization of angiotensin-converting enzyme, angiotensin II and AT1 receptor in human ocular tissues. Ophthalmic Res. 2004; 36: 312–20
- Van Haeringen NJ. The renin-angiotensin system in the human eye. Br J Ophthalmol. 1996; 80: 99–100
- Batenburg WW, Krop M, Garrelds IM, de Vries R, de Bruin RJ, Burcklé CA, et al. Prorenin is the endogenous agonist of the (pro)renin receptor. Binding kinetics of renin and prorenin in rat vascular smooth muscle cells overexpressing the human (pro)renin receptor. J Hypertens. 2007; 25: 2441–53
- Su JB. Kinins and cardiovascular diseases. Curr Pharm Des. 2006; 12: 3423–35
- Sealey JE, Laragh JH. The renin-angiotensin-aldosterone system for normal regulation of blood pressure and sodium and potassium homeostasis. Hypertension: Pathophysiology, diagnosis and management, JN Laragh, BM Brenner. Raven Press, New York 1990; 1287–311
- Buczko W, Matys T, Kucharewicz I, Chabielska E. The role of endothelium in antithrombotic effect of the renin-angiotensin system blockade. J Physiol Pharmacol. 1999; 50: 499–507
- Al-Merani SA, Brooks DP, Chapman BJ, Munday KA. The half-lives of angiotensin II, angiotensin II-amide, angiotensin III, Sar1-Ala8-angiotensin II and renin in the circulatory system of the rat. J Physiol. 1978; 278: 471–90
- Ruiz-Ortega M, Esteban V, Egido J. The regulation of the inflammatory response through nuclear factor-kappa b pathway by angiotensin IV extends the role of the renin angiotensin system in cardiovascular diseases. Trends Cardiovasc Med. 2007; 17: 19–25
- Donoghue M, Hsieh F, Baronas E, Godbout K, Gosselin M, Stagliano N, et al. A novel angiotensin-converting enzyme-related carboxypeptidase (ACE2) converts angiotensin I to angiotensin 1-9. Circ Res. 2000; 87: 1–9
- Welches WR, Brosnihan KB, Ferrario CM. A comparison of the properties and enzymatic activities of three angiotensin processing enzymes: angiotensin converting enzyme, prolyl endopeptidase and neutral endopeptidase 24,11. Life Sci. 1993; 52: 1461–81
- Kucharewicz I, Pawlak R, Matys T, Chabielska E, Buczko W. Angiotensin-(1-7): an active member of the renin-angiotensin system. J Physiol Pharmacol. 2002; 53: 533–40
- Santos RAS, Campagnole-Santos MJ, Andrade SP. Angiotensin-(1-7): an update. Regul Pept. 2000; 91: 45–62
- De Gasparo M, Catt KJ, Inagami T, Wright JW, Unger T. International union of pharmacology XXIII. @ The angiotensin II receptors. Pharmacol Rev. 2000; 52: 415–72
- Santos RA, Simoes e Silva AC, Maric C, Silva DM, Machado RP, de Buhr I, et al. Angiotensin-(1-7) is an endogenous ligand for the G-protein-coupled receptor Mas. Proc Natl Acad Sci U S A. 2003; 8: 8258–63
- Iwata M, Cowling RT, Gurantz D, Moore C, Zhang S, Yuan JX, et al. Angiotensin (1-7) binds to specific receptors on cardiac fibroblasts to initiate antifibrotic and antitrophic effects. Am J Physiol Heart Circ Physiol. 2005; 289: 2356–63
- Kostenis E, Milligan G, Christopoulos A, Sanchez-Ferrer CF, Heringer-Walther S, Sexton PM, et al. G-protein-coupled receptor Mas is a physiological antagonist of the angiotensin II type I receptor. Circulation. 2005; 111: 1806–13
- Brosnihan KB, Li P, Ferrario CM. Angiotensin-(1-7) dilates canine coronary arteries through kinins and nitric oxide. Hypertension. 1996; 27: 523–8
- Muthalif MM, Benter IF, Uddin MR, Harper JL, Malik KU. Signal transduction mechanisms involved in angiotensin-(1-7)-stimulated arachidonic acid release and prostanoid synthesis in rabbit aortic smooth muscle cells. J Pharmacol Exp Ther. 1998; 284: 388–98
- Jaiswal N, Diz DI, Chappell MC, Khosla MC, Ferrario CM. Stimulation of endothelial cell prostaglandin production by angiotensin peptides. Characterization of receptors. Hypertension. 1992; 19: 49–55
- Seyedi N, Xu X, Nasjletti A, Hintze TH. Coronary kinin generation mediates nitric oxide release after angiotensin receptor stimulation. Hypertension. 1995; 26: 164–70
- Okunishi H, Oka Y, Shiota N, Kawamoto T, Song K, Miyazaki M. Marked species-difference in the vascular angiotensin II-forming pathways: humans versus rodents. Japan J Pharmacol. 1993; 62: 207–10
- Balcells E, Meng QC, Johnson WH, Oparil S, Dell′Italia LJ. Angiotensin II formation from ACE and chymase in human and animal hearts: methods and species considerations. Am J Physiol Heart Circ Physiol. 1997; 273: 1769–74
- Akasu M, Urata H, Kinoshita A, Sasaguri M, Ideishi M, Arakawa K. Differences in tissue angiotensin II-forming pathways by species and organs in vitro. Hypertension. 1998; 32: 514–20
- Resende MM, Mill JG. Alternate angiotensin II-forming pathways and their importance in physiological or physiopathological conditions. Arq Bras Cardiol. 2002; 78: 432–8
- Miyazaki M, Takai S. Tissue angiotensin II generating system by angiotensin-converting enzyme and chymase. J Pharmacol Sci. 2006; 100: 391–7
- Okunishi H, Miyazaki M, Okamura T, Toda N. Different distribution of two types of angiotensin II-generating enzymes in the aortic wall. Biochem Biophys Res Commun. 1987; 149: 1186–92
- Urata H, Kinoshita A, Misono KS, Bumpus FM, Husain A. Identification of a highly specific chymase as the major angiotensin II-forming enzyme in the human heart. J Biol Chem. 1990; 265: 22348–57
- Bacani C, Frishman WH. Chymase: a new pharmacologic target in cardiovascular disease. Cardiol Rev. 2006; 14: 187–93
- Huang XR, Chen WY, Truong LD, Lan HY. Chymase is upregulated in diabetic nephropathy: implications for an alternative pathway of angiotensin II-mediated diabetic renal and vascular disease. J Am Soc Nephrol. 2003; 14: 1738–47
- Nishimoto M, Takai S, Kim S, Jin D, Yuda A, Sakaguchi M, et al. Significance of chymase-dependent angiotensin II-forming pathway in the development of vascular proliferation. Circulation. 2001; 104: 1274–9
- Jin D, Takai S, Yamada M, Sakaguchi M, Miyazaki M. Beneficial effects of cardiac chymase inhibition during the acute phase of myocardial infarction. Life Sci. 2002; 71: 437–46
- Ihara M, Urata H, Kinoshita A, Suzumiya J, Sasaguri M, Kikuchi M, et al. Increased chymase-dependent angiotensin II formation in human atherosclerotic aorta. Hypertension. 1999; 33: 1399–405
- Klickstein LB, Kaempfer CE, Wintroub BU. The granulocyte-angiotensin system. Angiotensin I-converting activity of cathepsin G. J Biol Chem. 1982; 257: 15042–6
- Reilly CF, Tewksbury DA, Schechter NM, Travis J. Rapid conversion of angiotensin I to angiotensin II by neutrophil and mast cell proteinases. J Biol Chem. 1982; 257: 8619–22
- Grisé C, Boucher R, Thibault G, Genest J. Formation of angiotensin II by tonin from partially purified human angiotensinogen. Can J Biochem. 1981; 59: 250–5
- Kokkonen JO, Saarinen J, Kovanen PT. Angiotensin II formation in the human heart: an ACE or non-ACE mediated pathway? Ann Med. 1998;309–13.
- Vickers C, Hales P, Kaushik V, Dick L, Gavin J, Tang J, et al. Hydrolysis of biological peptides by human angiotensin-converting enzyme-related carboxypeptidase. J Biol Chem. 2002; 277: 14838–43
- Tipnis SR, Hooper NM, Hyde R, Karran E, Christie G, Turner AJ. A human homolog of angiotensin-converting enzyme. Cloning and functional expression as a captopril-insensitive carboxypeptidase. J Biol Chem. 2000; 275: 33238–43
- Yagil Y, Yagil C. Hypothesis: ACE2 modulates blood pressure in the mammalian organism. Hypertension. 2003; 41: 871–3
- Brubaker RF. Clinical evaluation of circulation of aqueous humor flow. Duane's Clinical Ophthalmology.Vol 3, Ch 46, W Tasman, EA Jaeger. JB Lippincott Company, Philadelphia 1994; 1–11
- Brooks AM, Gillies WE. Ocular beta-blockers in glaucoma management. Clinical pharmacological aspects. Drugs Aging. 1992; 2: 208–21
- Kardon RH, Weingeist TA. Anatomy of the ciliary body and outflow pathways. Duane's Clinical Ophthalmology. Vol 3, Ch 43, W Tasman, EA Jaeger. JB Lippincott Company, Philadelphia 1994; 1–26
- Weinreb RN. Uveoscleral outflow: the other outflow pathway. J Glauc. 2000; 9: 343–5
- Sramek SJ, Wallow IH, Day RP, Ehrlich EN. Ocular renin-angiotensin: immunohistochemical evidence for the presence of prorenin in eye tissue. Invest Ophthalmol Vis Sci. 1988; 29: 1749–52
- Tikellis C, Johnston CI, Forbes JM, Burns WC, Thomas MC, Lew RA, et al. Identification of angiotensin converting enzyme 2 in the rodent retina. Curr Eye Res. 2004; 29: 419–27
- Shiota N, Saegusa Y, Nishimura K, Miyazaki M. Angiotensin II-generating system in dog and monkey ocular tissues. Clin Exp Pharmacol Ther. 1997; 24: 243–8
- Senanayake P, Drazba J, Shadrach K, Milsted A, Rungger-Brandle E, Nishiyama K, et al. Angiotensin II and its receptor subtypes in the human retina. Invest Ophthalmol Vis Sci. 2007; 48: 3301–11
- Schelling P, Ganten U, Sponer G, Unger T, Ganten D. Components of the renin angiotensin system in cerebrospinal fluid of rats and dogs with special consideration of the origin and fate of angiotensin II. Neuroendocrinology. 1980; 31: 297–308
- Cunha-Vaz J. The blood-ocular barriers. Surv Ophthalmol. 1979; 23: 279–96
- Danser AH, van der Dorpel MA, Deinum J, Derkx FH, Franken AA, Peperkamp E, et al. Renin, prorenin, and immunoreactive renin in vitreous fluid from eyes with and without diabetic retinopathy. J Clin Endocrinol Metab. 1989; 68: 160–7
- Geng L, Persson K, Nilsson SFE. Angiotensin converting enzyme (ACE) activity in porcine ocular tissue: effects of diet and ACE inhibitors. J Pharm Ther. 2003; 19: 589–96
- Ramirez M, Davidson EA, Luttenauer L, Elena P-P, Cumin F, Mathis GA, et al. The renin-angiotensin system in the rabbit eye. J Ocul Pharmacol Ther. 1996; 12: 299–312
- Culliane AB, Leung PS, Ortgo J, Coca-Prados M, Harvey BJ. Renin-angiotensin system expression and secretory function in cultured human ciliary body non-pigmented epithelium. Br J Ophthalmol. 2002; 86: 676–83
- Lin C, Stone RA, Wax MB. Angiotensin binding sites in rabbit anterior uvea and human ciliary epithelial cells. Invest Ophthalmol Vis Sci. 1990; 31: 147–52
- Capponi AM, Lew PD, Jornot L, Vallotton MB. Correlation between cytosolic free Ca2+ and aldosterone production in bovine adrenal glomerulosa cells. J Biol Chem. 1984; 259: 8863–9
- Hou Y, Delamere NA. Influence of Ang II on cytoplasmic sodium in cultured rabbit nonpigmented ciliary epithelium. Am J Physiol Cell Physiol. 2002; 283: 552–9
- Langman MJS, Lancashire RJ, Cheng KK, Stewart PM. Systemic hypertension and glaucoma: mechanisms in common and co-occurrence. Br J Ophthalmol. 2005; 89: 960–3
- Osusky R, Nussberger J, Amstutz C, Flammer J, Brunner HR. Individual measurements of angiotensin II concentrations in aqueous humor of the eye. Eur J Ophthalmol. 1994; 4: 228–33
- Reitsamer HA, Kiel JW. Relationship between ciliary body blood flow and aqueous production in rabbits. Invest Ophthalmol Vis Sci. 2003; 44: 3967–71
- Lotti VJ, Pawlowski N. Prostaglandins mediate the ocular hypotensive action of the angiotensin converting enzyme inhibitor MK-422 (enalaprilat) in African green monkeys. J Ocul Pharmacol. 1990; 6: 1–7
- Nilsson SF, Samuelsson M, Bill A, Stjernscantz J. Increased uveoscleral outflow as a possible mechanism of ocular hypotension caused by prostaglandin F2 alpha-1-isopropylester in the cynomolgus monkey. Exp Eye Res. 1989; 48: 707–16
- Weinreb RN, Toris CB, Gabelt BAT, Lindsey JD, Kaufman PL. Effects of prostaglandins on the aqueous humor outflow pathways. Surv Ophthalmol. 2002; 47: 53–64
- Haefliger IO, Flammer J, Luscher TF. Nitric oxide and endothelin-1 are important regulators of human ophthalmic artery. Invest Ophthalmol Vis Sci. 1992; 33: 2340–3
- Yao K, Tschudi M, Flammer J, Luscher TF. Endothelium-dependent regulation of vascular tone of the porcine ophthalmic artery. Invest Ophthalmol Vis Sci. 1991; 32: 1791–8
- Shen F, Zhang L, Liu T. Effects of angiotensin II on the 3H-TdR incorporation and synthesis of collagen in cultured bovine trabecular meshwork cells. Yan Ke Xue Bao. 2001;17:209–12 (in Chinese).
- Inoue T, Yokoyoma T, Koike H. The effect of angiotensin II on uveoscleral outflow in rabbits. Curr Eye Res. 2001; 23: 139–43
- Macri FJ. The action of angiotensin on intraocular pressure. Arch Ophthalmol. 1965; 73: 528–39
- Vaajanen A, Vapaatalo H, Kautiainen H, Oksala O. Angiotensin (1-7) reduces intraocular pressure in the normotensive rabbit eye. Invest Ophthalmol Vis Sci. 2008 Jan 25 (Epub ahead of print).
- Bárány EH. Simultaneous measurement of changing intraocular pressure and outflow facility in the vervet monkey by constant pressure infusion. Invest Ophthalmol Vis Sci. 1964; 3: 135–43
- Vaajanen A, Mervaala E, Oksala O, Vapaatalo H. Is there a relationship between blood pressure and intraocular pressure? An experimental study in hypertensive rats. Curr Eye Res. 2008 33:325–32.
- Konno T, Maruichi M, Takai S, Oku H, Sugiyama T, Uchibori T, et al. Effect of chymase on intraocular pressure in rabbits. Eur J Pharmacol. 2005; 524: 132–7
- Mabuchi F, Aihara M, Mackey MR, Lindsey JD, Weinreb RN. Regional optic nerve damage in experimental mouse glaucoma. Invest Ophthalmol Vis Sci. 2004; 45: 4352–8
- Sjølie AK, Chaturvedi N. The retinal renin-angiotensin system: implications for therapy in diabetic retinopathy. J Hum Hypertens. 2002; 16: 42–6
- Vita JB, Anderson JA, Hulem CD, Irving HL. Angiotensin-converting enzyme activity in ocular fluids. Invest Ophthalmol Vis Sci. 1981; 20: 255–7
- Weinreb RN, Sandman R, Ryder MI, Friberg TR. Angiotensin-converting enzyme activity in human aqueous humor. Arch Ophthalmol. 1985; 103: 34–6
- Maruichi M, Oku H, Takai S, Muramatsu M, Sugiyama T, Imamura Y, et al. Measurement of activities in two different angiotensin II generating systems, chymase and angiotensin-converting enzyme, in the vitreous fluid of vitreoretinal diseases: A possible involvement of chymase in the pathogenesis of macular hole patients. Curr Eye Res. 2004; 29: 321–5