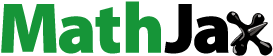
ABSTRACT
This study is the first to develop food supply and demand projections over the 21st century for Uzbekistan by considering the combined effects of climate change and soil salinization. The study results suggest that rising summer temperatures and soil salinity will considerably reduce wheat production. Projections indicate that a large wheat supply–demand gap will emerge in the midterm, particularly under the SSP3-RCP7.0 scenario. For the two more pessimistic scenarios, supply losses of about 24–29% are expected by the end of the century. Supply–demand gaps of up to 2.7 million tons of wheat would pose serious challenges to national food security.
Introduction
Global climate change poses a serious threat to the ecosystems, food production and industries in many parts of the world (Intergovernmental Panel on Climate Change [IPCC], Citation2022). Uzbekistan is one of the hotspots of global warming, with numerous studies having made evident that global warming is causing a reduction in river runoff and increasing water scarcity in the region (Gosling & Arnell, Citation2016; Reyer et al., Citation2017). Irrigated agriculture, which accounts for about 90% of total water withdrawals in Central Asia, is the most vulnerable to temperature variations and reduced water supply (Frenken, Citation2013). Increasing crop water consumption requirements and evaporation losses from irrigation systems have already added to the water deficit challenge in the region (Reyer et al., Citation2017). Recent research has focused on the impact of climate change on total factor productivity growth in agriculture (Kirui et al., Citation2023; Ortiz-Bobea et al., Citation2021). Climate change-induced water supply reductions in the region will lead to considerable financial losses within and outside agriculture (Bekchanov, Citation2014; Bekchanov & Lamers, Citation2016). Previous studies have suggested that climate change might have a positive impact on certain subregions in the short term (Mirzabaev, Citation2013; Sommer et al., Citation2013); however, widespread negative effects are inevitable in the midterm (Bobojonov & Aw-Hassan, Citation2014; Bobojonov et al., Citation2016).
Another serious challenge for Uzbek agriculture is soil salinization, which has resulted in considerable decreases in agricultural production (Khasanov et al., Citation2023). Soil salinization and land degradation have become the most pressing problems in the Aral Sea region of Central Asia, particularly in Uzbekistan, with 73.8% of the area around the South Aral Sea being moderately saline and 7.5% being slightly saline (Duan et al., Citation2022). The primary causes of soil salinization in Uzbekistan are poor land and groundwater management (Eswar et al., Citation2021; Ibrakhimov et al., Citation2007), and the use of phosphorus-based mineral fertilizers (Khasanov et al., Citation2023). Soil salinization is intensified due to changes in weather variability and water availability in dry regions, with shallow groundwater levels under water-scarce conditions (Corwin, Citation2021; Greene et al., Citation2016). Higher temperatures due to climate change reduce water availability, increase evapotranspiration and consequently require additional irrigation water applications, when saline water from groundwater aquifers or other alternative sources is often used to meet crop water demand (Mukhopadhyay et al., Citation2021; Okur & Örçen, Citation2020; Schlenker et al., Citation2005). If irrigation water applications are reduced due to lowered water supplies, capillary increases due to high evaporation induced by climate change also lead to higher soil salinity around the crop root zones.
Despite this vicious circle, the effects of soil salinity have not been fully considered in most of the previous projections on the effects of climate change on crop yields (e.g., Hatfield et al., Citation2015; Lobell et al., Citation2011). Our study contributes to ongoing research by using granular data and implementing panel data analysis to model the combined effects of soil salinization and climate change on the wheat supply of Uzbekistan, one of the hotspots of climate change. This approach allows for a detailed spatial analysis of the climate change impacts on wheat yields, a strategic staple crop across Uzbekistan. Differing from other approaches, such as the Ricardian approach, the panel data approach allows for controlling time-invariant omitted variables beyond temperature and precipitation (Deschenes & Greenstone, Citation2012). Moreover, by regressing crop yields on biophysical factors, we developed a statistical crop yield model based on empirical data, which is more reliable than biophysical crop simulation models (Ortiz-Bobea & Tack, Citation2018). Additionally, we applied a ‘shared socio-economic pathways–radiative concentration pathways’ (SSP-RCP) climate scenarios framework (van Vuuren et al., Citation2014) to the context of Uzbekistan and integrated it with the estimated ‘climate-wheat yield’ function to assess the future climate change impacts on food demand and supply for the first time in Uzbekistan.
According to our projections, the supply of wheat is anticipated to decrease considerably due to heat and salinity stress, resulting in substantial food demand supply gaps after 2040. Given the limitedness of available croplands and water resources, significant improvements in fertilizer-use efficiency, soil management and the development of high-yielding varieties will be necessary to ensure local food security in the future.
Data and methods
Study area
Uzbekistan is a double land-locked country located in the centre of Central Asia (). Over 40% of its 35.6 million people reside in rural areas (State Statistics Committee of Uzbekistan [SSCU], Citation2022). Due to consistent population growth, national food security has become a matter of significant political importance in the country.
The climate of the country is extremely continental, with an average monthly temperature of 13.7°C, peaking at 28.5°C in July (). Temperatures can plunge to −35°C in the north of the country during winter and soar to 50°C in the south during summer (as observed from 1991 to 2020). The country experiences a wide range of annual precipitation, varying between 200 and 550 mm in most areas. Yet, areas in the desert and steppe zones receive less than 200 mm, while mountainous areas receive more than 900 mm (Khalikulov et al., Citation2016).
Figure 2. Average monthly temperature and rainfall in Uzbekistan, 1991–2020.

Agricultural production plays a pivotal role in the national economy, contributing 16% of gross domestic product (GDP) and employing 44% of the labour force (Djanibekov et al., Citation2010; SSCU, Citation2022). Wheat production, in particular, is crucial for national food security, as flour-based food products provide over 52% of the dietary caloric intake (Abdullaev et al., Citation2009; Chabot & Tondel, Citation2011). Following the collapse of the former Soviet Union’s unified food supply and distribution system in 1991, Uzbekistan’s access to strategic food imports became limited and insecure. Consequently, the government introduced agricultural reforms to increase domestic wheat production and enhance ‘independence in cereals’ through policies such as setting wheat production targets, subsidizing inputs (irrigation water, mineral fertilizers, fuel, machinery), and regulating wheat and flour prices (Lombardozzi & Djanibekov, Citation2021).
Thanks to the reforms, the area of national wheat production increased rapidly from about 627,000 to over 1.3 million ha between 1992 and 1996, allowing the country to achieve independence in cereals (). The expansion of wheat production was achieved through reducing areas previously used for fodder production and some cotton areas as well. The country’s wheat yields have gradually increased over the years, reaching 4900 kg/ha in 2009 due to the introduction of suitable wheat varieties based on each region’s soil and climatic conditions. Another driver was farmers’ improved knowledge and experience with cultivation practices and wheat varieties. Currently, wheat and cotton are the most essential crops and are widely cultivated throughout the country.
Figure 3. Wheat yields and harvested area in Uzbekistan, 1992-2020.

Wheat cultivation areas that are irrigated account for 85%, while the remaining 15% are areas of rainfed farming. However, almost all the rainfed wheat farming areas are located in the provinces of Kashkadarya, Jizzakh and Samarkand. Irrigated wheat farming takes place in late autumn, winter and spring, thus winter and spring precipitation is crucial for the performance of irrigated wheat farming. During the winter and spring, the ample precipitation saturates the soil with moisture, reducing the need for additional irrigation.
Analytical approach and data
To assess wheat supply and demand projections, we calculated the production elasticities for important production and climate factors in the first step. These calculated production elasticities were then used to develop the supply projections.
Production elasticities
For our analysis in the first step, we employed a Cobb–Douglas production function in log form:
where represents the total output in district i and at time t; b is the slope coefficient; the variable Land stands for total harvested land (thousands ha); Labour is the labour value used; Fert is total (chemical) fertilizer; IW represents the total amount of irrigation water; T represents the average temperature for each season (W, winter; Sp, spring; S, summer; A, autumn); P represents each season’s total precipitation; GWS represents groundwater salinity; SS represents soil salinity; and
is the error term.
A fixed effect model can capture the relationship between agricultural production and climate variables by considering that unobserved time-invariant factors or unobserved variables are constant (Allison, Citation2009). Many prior studies have used fixed-effect models to measure the impact of climate change on different crops within a panel data framework (Ahmad et al., Citation2014; Mirzabaev, Citation2013; Sadozai et al., Citation2019). Additionally, a Hausman test confirmed the suitability of a fixed effects model for our data.
To analyse the climate, biophysical and economic factors influencing wheat productivity, we constructed a panel dataset encompassing the years between 2008 and 2017, and 158 of the country’s 176 districts (SSCU, Citation2022). We obtained the necessary data from multiple sources, including Beaudoing et al. (Citation2020), Rodell et al. (Citation2004), SSCU (Citation2020), the Ministry of Water Resources of the Republic of Uzbekistan (MWRRU, Citation2020), and the Global Rainfall Map in Near Real-Time (GSMaP_NRT) (Citation2023) prepared by the JAXA Global Rainfall Watch of Japan’s Aerospace Exploration Agency.
Particularly, we collected province-level secondary data on the total wheat yields and harvested area across districts for both irrigated and rainfed farming from the SSCU (Citation2020). Data on the total costs of nitrogen and phosphorus and labour use were also obtained from the same source (SSCU, Citation2020). Data on district level irrigation water use for September–December and March–May were provided by the regional branches of the MWRRU (Citation2020). We excluded January and February as the soil is typically frozen in these months, and hence no irrigation or leaching takes place. Data on temperature were extracted from global climate databases (Beaudoing et al., Citation2020; Rodell et al., Citation2004). We used the mean temperature for the relevant period from September to June, omitting the (post-) harvest period of July–August. Data on precipitation, particularly cumulative rainfall across districts for the same period were derived from the GSMaP_NRT prepared by the JAXA Global Rainfall Watch of Japan’s Aerospace Exploration Agency (see also Eltazarov et al., Citation2021). Data on monthly soil and groundwater salinity levels were obtained from the regional branches of MWRRU (Citation2020), and we calculated annual salinity levels as the average of monthly levels.
provides summary statistics and definitions of the variables used in the regression model. The dependent variable in the model is the log of total wheat production. The average wheat production across the districts is 39,217 tons; the average wheat sown area is 8283 ha. Given the data availability, some intermediate inputs in wheat cultivation, such as labour and fertilizers, are measured in economic terms (in UZS), while irrigation water is measured in physical terms (thousands m3). The average fertilizer cost per district is 2.498 million UZS and the average labour cost per district is 2.357 million UZS. For 10 months, the district-level average irrigation water use is 134,000 m3.
Table 1. Summary statistics.
The average temperature across the districts in winter, spring, summer and autumn is 1.5, 16.5, 27.8 and 14.7°C, respectively. Precipitation almost does not occur in June, and irrigated areas receive about 80 mm of rain or snowfall per season in winter and spring on average.
Due to seasonal changes, the average salinity levels of soil and groundwater are reported as annual averages, ranging from 0.1 to 7.6 g/l. Lower soil salinity levels of 0.1–0.4 g/l are found in the regions of Samarkand and Namangan, while higher soil salinity levels of 2.1 g/l are detected in regions around the Aral Sea. The highest salinity level of 7.6 g/l is found in the regions of Karakalpakstan, Khorezm and Bukhara.
Wheat supply and demand projections
To estimate future wheat supply, we integrated the production model described above with existing climate projections’ data from the Global Climate Knowledge Portal (World Bank, Citation2022), which provides seasonal temperature and precipitation projections across Uzbekistan from 2020 to 2100 under various SSP-RCP scenarios. To develop wheat demand projections, we considered national population changes over time under SSP scenarios (IIASA, Citation2023) and average dietary wheat consumption requirements per capita. As the Uzbek wheat production area has not changed over the last decade (), we assumed it to remain constant in the future.
Representative concentration pathways (RCPs) are trajectories of atmospheric greenhouse gas concentrations as adopted by the IPCC (Citation2022). These pathways correspond to possible warming levels over the years triggered by greenhouse gas emissions (Van Vuuren et al., Citation2011). The RCPs considered here, including RCP2.6, RCP4.5, RCP7.0 and RCP8.5, are labelled based on a possible range of radiative forcing in 2100. Higher values of RCP stand for higher levels of greenhouse gas (GHG) emissions and thus higher levels of temperature increases by 2100, while lower levels of RCP are related to lower temperatures but require high climate change mitigation efforts.
Since the IPCC’s Fifth Assessment Report (Citation2022), RCPs have been considered together with so-called shared socio-economic pathways (SSPs). The SSPs represent socio-economic developments up to 2100 and are used to elaborate GHG emission scenarios under various climate policies. Five SSP scenarios are: SSP1 – Sustainability (‘Taking the Green Road’), SSP2 – ‘Middle of the Road’, SSP3 – Regional Rivalry (‘A Rocky Road’), SSP4 – Inequality (‘A Road Divided’), and SSP5 – Fossil-fuelled Development (‘Taking the Highway’) (Van Vuuren et al., Citation2014). In brief, SSP1 stands for low levels of barriers to climate change adaptation and mitigation, and corresponds to low levels of global temperature rises, being the most desirable among all SSPs; SSP2 and SSP3 considering moderate and high levels of barriers for climate change adaptation and mitigation, respectively. SSP4 represents low levels of difficulty for introducing mitigation options but strong barriers to adaptation efforts. SSP5 considers high levels of difficulty to implement mitigation measures but low levels of challenges for adopting adaptation options.
Four combinations of climatic (RCP) and socio-economic development pathways (SSPs) are globally agreed among climate experts as standard scenarios (‘Tier 1’) to conduct climate policy research, namely SSP1-RCP2.6, SSP2-RCP4.5, SSP3-RCP7.0 and SSP5-RCP8.5 (O’Neill et al., Citation2016). These four standard scenarios were also adopted in our analysis of wheat supply and demand projections.
Results
The impact of climate and resource availability on wheat yields
The results of our fixed effects regression analysis are presented in . As expected, land, labour and fertilizer were found to significantly contribute to agricultural production. Since wheat yields are higher in irrigated areas than in rain-fed areas, irrigation water was found to have a significantly positive effect on productivity, even though the sample included non-irrigated areas. A 1% increase was associated with a 0.05% increase in production output across the whole sample. However, excluding non-irrigated areas would have resulted in a considerably larger slope coefficient.
Table 2. Results of the fixed effect panel model estimates.
Temperature also positively contributed to wheat productivity, but only in spring and autumn/fall. A 1% increase in spring temperatures was associated with an increase in crop output by 0.7%, while a 1% increase in autumn temperatures translated into a 0.4% increase in crop output. Conversely, temperature in the summer had a negative effect on productivity as excessive heat dries up the soil and inhibits plant growth. A 1% increase in summer temperatures was associated with a 1.9% drop in production output.
The analysis revealed that precipitation levels have no significant impact on productivity, which can be attributed to the fact that the majority of the sample consisted of irrigated areas. Soil salinity was found to have a negative relationship with production, with a 1% increase in soil salinity resulting in a 0.09% decrease in production. Additionally, the study found that groundwater salinity had a positive impact on productivity, as high groundwater salinity is likely a sign for lower water tables and reduced salt accumulation near the plant roots. A 1% increase in groundwater salinity was associated with a 0.11% increase in production. The results were also found to be robust to modifications in the selected time period and to an inclusion of a random effects model (see Table A1 in the supplemental data online).
National wheat supply and demand projection under various climatic SSP-RCP pathways
displays projections for future wheat supply and demand, considering future temperature and precipitation changes under SSP-RCP scenarios, as well as anticipated shifts in population.
Figure 4. Wheat supply and demand projections (without considering losses in trade and transportation) in Uzbekistan, 2020–2100, under various climate and socio-economic change (SSP-RCP) scenarios.

The SSP3-RCP7.0 (‘Regional rivalry’) scenario represents strong barriers to climate change mitigation and adaptation and leads to global mean temperature increases of above 5°C. Under this scenario, wheat demand is expected to increase enormously, reaching about 7.5 million by the last decade of the 21st century. However, wheat supply is projected to decrease substantially due to heat and salinity stress, falling short of 2020 production levels of 6.2 million tons by 24% (or 1.5 million tons) by the end of the century. Surging demand and dwindling harvests together would lead to a steadily growing demand–supply gap of about 2.7 million tons per year after 2040.
In the SSP5-RCP8.5 scenario (‘Taking the Highway’), both wheat supply and demand steadily decrease. Wheat supply continues to exceed demand until the end of the century. However, this supply surplus is only due to significant decreases in wheat demand that outweigh the substantial supply losses of 1.8 million tons or 29% compared with 2020.
The SSP2-RCP4.5 (‘Middle of the Road’) scenario implies moderate barriers to the adoption of climate adaptation and mitigation measures. In this case, wheat demand is expected to surpass wheat supply in the middle of the century by about 0.6 million tons. However, the water supply–demand gap will shrink once again at the end of the century due to the impact of a declining population.
The SSP1-RCP2.6 scenario (‘Sustainability’) implies substantial investment in mitigation and adoption measures. Under this scenario, the national wheat supply is expected to meet national dietary wheat demand until 2050, after which the supply will exceed demand due to a declining population.
Although SSP1-RCP2.6 and SSP2-RCP4.5 scenarios assume reduced population growth, current trends indicate that population growth in Uzbekistan is likely to increase due to cultural factors, which are not considered projections by IIASA (Citation2023). Additionally, financial and institutional challenges for adopting renewable energies and sustainable adaptation options remain high despite governmental support for sustainable transformation. Therefore, current tendencies signal a high likelihood of a SSP3-RCP7.0 scenario, which implies higher population growth and consequent increases in wheat demand that would lead to a wheat deficit after 2050 in Uzbekistan.
Discussion and policy implications
In this study, we aimed to estimate the effects of ongoing climate change and increasing soil salinization on future wheat supply changes in Uzbekistan. To achieve this, we constructed a panel data projection approach based on a fixed effects estimation of production elasticities, taking into account factors such as climate change and soil salinization. Our production function approach supports the assumption of strong interaction effects between climate change and salinization in the country. Under the two most pessimistic scenarios, we estimate that these interaction effects could result in yield losses of 24–29%. To assess the impact on food security, we compared these expected yield losses with projected changes in wheat demand under various scenarios. Our projections show that the only scenario where food security is guaranteed without substantial changes in demand is the sustainability scenario (SSP1-RCP2.6). In all other cases, a large supply–demand gap of up to 2.7 million tons would need to be covered on international wheat markets.
To maintain current levels of wheat supply, given the limited potential for cropland adjustments in Uzbekistan, optimizing both water and fertilizer inputs is essential. Our first-stage model implies that a 1% increase of irrigation water would result in a 0.045% increase in wheat production. Meanwhile, a 1% increase in fertilizer application would lead to an average increase of 0.39% in wheat production. However, these approaches are not sustainable, as increased areas with shallow groundwater tables would lead to increased soil salinization and further yield losses, as well as postpone other structural adjustments, as has occurred in irrigated areas of the US (Cui, Citation2020). Regarding fertilizer uses, as most crops in the region are cultivated using high amounts of fertilizer due to poor soil quality (Bobojonov & Aw-Hassan, Citation2014; Kienzler et al., Citation2011), excess fertilizer application could lead to a vicious cycle of further decreases in soil health and water quality, as shown by the example of China (Guo et al., Citation2010; Zhu & Chen, Citation2002).
An alternative approach to conventional agriculture is conservation agriculture, which comprises a set of practices, including zero or low-tillage, permanent soil organic cover, and the diversification of plant species. This approach not only reduces soil moisture losses, but also requires less fertilizer compared with conventional practices (Devkota et al., Citation2015). Additionally, it improves soil organic matter and soil fertility, consequently increasing resilience to salinization. Another approach could be the introduction of heat-tolerant plants (Bita & Gerats, Citation2013) with lower water needs and wheat varieties with a short germination and vegetation period. Such varieties would make it possible to cope with or even avoid high temperatures and high irrigation needs during the summer.
To achieve sustainability, this paper proposes several political measures. First, the identification of the most suitable solution for a particular region and farm structure, however, requires detailed analysis and agronomic advice. Agricultural policy in Uzbekistan plays a crucial role in creating a conducive environment for the development of extension services and increased management knowledge among agricultural producers. Through these services, local farmers can access information on suitable adaptation strategies, as presented above. The country has focused on traditional state extension services for too long in the past. Recently, a number of private extension services have emerged, and participatory forms of extension services in particular can bring about changes towards the modernization of farming systems (Djuraeva et al., Citation2023). In the future, the government should facilitate the exchange between private and state extension services and the use of synergies in cooperation. Additionally, input suppliers, both national and international, together with science specialists on seed breeding, can further the development and distribution of more drought-tolerant varieties.
Second, to improve evidence-based policy evaluation in the country, policies are needed to enhance access to high-quality farm-level data, which is currently lacking. For instance, the Uzbek government implemented a policy on the subsidization of drip irrigation technologies in 2022, but without detailed information on irrigation facilities at the farm level, empirical policy evaluation will remain challenging. Improving the infrastructure and human capacity of national statistical agencies and expanding national research funding would also be helpful for building detailed and reliable databases.
Finally, most proposed solutions require considerable investment. Yet, considerable production risk due to insecure land tenure and controlled production markets may deter farmers taking on agricultural credit and instead opt for a low-input strategy (Kuhn & Bobojonov, Citation2023). To incentivize investments in sustainable production, the government should prioritize refining institutional settings and developing financial risk management options, such as credit-bundled weather index insurance (Bryla-Tressler et al., Citation2011; Lu et al., Citation2022).
Conclusions
This study is one of the few approaches to investigate the combined ex-post effects of climate change and soil salinity on wheat yields, as well as future domestic food security in terms of wheat, which is a critical issue for many regions worldwide. Our results indicate that Uzbekistan has heavily relied on irrigation water use and is vulnerable to climate change, particularly considering already high levels of soil salinization. Furthermore, the population growth and increasing demand for food in the future are expected to result in a considerable wheat supply–demand gap, as shown under the SSP3-RCP4.5 scenario. To avoid this gap, structural adjustments and dedicated policy approaches are essential for achieving sustainability.
This study has several limitations. First, the underlying database is relatively short, covering only a period from 2008 to 2017, which limits the ability of studying the effects of long-term climatic trends. Access to more historical data would enable a more differentiated analysis. Second, the study uses aggregated data at the district level, which does not allow for regional-specific features in climatic conditions or even farm-level differences in agricultural practices to be examined. Future research could focus on analysing the non-linear impacts of some climatic variables and exploring the effects of other climatic variables, such as solar radiation, wind, CO2 and humidity, on crop yields and total factor productivity (TFP).
Our study underlines the need for immediate adaptation measures to narrow the future wheat supply–demand gap, despite smaller discrepancies in regional and temporal projections. To safeguard the future of agricultural activities and ensure food security in the region, advancements in water and soil management are crucial. Furthermore, development of new seeds and varieties, and reassessment of suitable wheat production areas are essential. These adjustments require foremost improvement in extension services, provision of precise farm-level data and modern risk management.
Author contribution statement
Mashkhura Babadjanova: investigation, methodology, formal analysis, software, writing, original draft. Ihtiyor Bobojonov: conceptualization, methodology, writing, review and editing. Maksud Bekchanov: conceptualization, formal analysis, writing, review and editing. Lena Kuhn: conceptualization, validation, resources, writing, review and editing. Thomas Glauben: writing, review and editing, supervision, funding acquisition.
Supplemental Material
Download MS Word (30.3 KB)Acknowledgements
The authors acknowledge and thank the organizers of the ADBI conference entitled ‘Water Resource Management in Agriculture for Achieving Food and Water Security under Climate Change in Asia’, 26–27 October 2022, for the opportunity to present our paper, and we thank the discussants for their useful feedback. We are also grateful for the valuable comments we received from the anonymous reviewers.
Disclosure statement
No potential conflict of interest was reported by the authors.
Supplementary material
Supplemental data for this article can be accessed at https://doi.org/10.1080/07900627.2023.2290523.
Additional information
Funding
References
- Abdullaev, I., De Fraiture, C., Giordano, M., Yakubov, M., & Rasulov, A. (2009). Agricultural water use and trade in Uzbekistan: Situation and potential impacts of market liberalization. International Journal of Water Resources Development, 25(1), 47–63. https://doi.org/10.1080/07900620802517533
- Ahmad, M., Nawaz, M., Iqbal, M., & Javed, S. (2014). Analyzing the impact of climate change on rice productivity in Pakistan. https://mpra.ub.uni-muenchen.de/72861/
- Allison, P. D. (2009). Fixed effects regression models. SAGE publications.
- Beaudoing, H., & Rodell, M. NASA/GSFC/HSL. (2020). GLDAS Noah land surface model L4 monthly 0.25 x 0.25 degree V2.1. Goddard Earth Sciences Data and Information Services Center (GES DISC). https://doi.org/10.5067/SXAVCZFAQLNO
- Bekchanov, M. (2014). Efficient water allocation and water conservation policy modeling in the Aral Sea Basin [ PhD thesis]. University of Bonn.
- Bekchanov, M., & Lamers, J. (2016). Economic costs of reduced irrigation water availability in Uzbekistan (Central Asia). Regional Environmental Change, 16(8), 2369–2387. https://doi.org/10.1007/s10113-016-0961-z
- Bita, C. E., & Gerats, T. (2013). Plant tolerance to high temperature in a changing environment: Scientific fundamentals and production of heat stress-tolerant crops. Frontiers in Plant Science, 4, 273. https://doi.org/10.3389/fpls.2013.00273
- Bobojonov, I., & Aw-Hassan, A. (2014). Impacts of climate change on farm income security in Central Asia: An integrated modeling approach. Agriculture Ecosystems and Environment, 188, 245–255. https://doi.org/10.1016/j.agee.2014.02.033
- Bobojonov, I., Berg, E., Franz-Vasdeki, J., Martius, C., & Lamers, J. P. (2016). Income and irrigation water use efficiency under climate change: An application of spatial stochastic crop and water allocation model to Western Uzbekistan. Climate Risk Management, 13, 19–30. https://doi.org/10.1016/j.crm.2016.05.004
- Bryla-Tressler, D., Syroka, J., Dana, J., Manuanorm, O. P., Lotsch, A., & Dick, W. (2011). Weather index insurance for agriculture: Guidance for development practitioners (Agriculture and Rural Development Discussion Paper, 50).
- Chabot, P., & Tondel, F. (2011). A regional view of wheat markets and food security in Central Asia: With a focus on Afghanistan and Tajikistan. United Stated Agency for International Development Famine Early Warning Systems Network FEWS NET, United States.
- Corwin, D. L. (2021). Climate change impacts on soil salinity in agricultural areas. European Journal of Soil Science, 72(2), 842–862. https://doi.org/10.1111/ejss.13010
- Cui, X. (2020). Climate change and adaptation in agriculture: Evidence from US cropping patterns. Journal of Environmental Economics and Management, 101, 102306. https://doi.org/10.1016/j.jeem.2020.102306
- Deschenes, O., & Greenstone, M. (2012). The economic impacts of climate change: Evidence from agricultural output and random fluctuations in weather: Reply. American Economic Review, 102(7), 3761–3773. https://doi.org/10.1257/aer.102.7.3761
- Devkota, M., Martius, C., Gupta, R. K., Devkota, K. P., McDonald, A. J., & Lamers, J. P. A. (2015). Managing soil salinity with permanent bed planting in irrigated production systems in Central Asia. Agriculture Ecosystems and Environment, 202, 90–97. https://doi.org/10.1016/j.agee.2014.12.006
- Djanibekov, N., Rudenko, I., Lamers, J., & Bobojonov, I. (2010). Pros and cons of cotton production in Uzbekistan. Case Study #7-9. In P. Pinstrup-Andersen & F. Cheng (Eds.), Food policy for developing countries: Case studies. Cornell University.
- Djuraeva, M., Bobojonov, I., Kuhn, L., & Glauben, T. (2023). The impact of agricultural extension type and form on technical efficiency under transition: An empirical assessment of wheat production in Uzbekistan. Economic Analysis and Policy, 77, 203–221. https://doi.org/10.1016/j.eap.2022.11.008
- Duan, Z., Wang, X., & Sun, L. (2022). Monitoring and mapping of soil salinity on the exposed seabed of the Aral Sea, Central Asia. Water, 14(9), 1438. https://doi.org/10.3390/w14091438
- Eltazarov, S., Bobojonov, I., Kuhn, L., & Glauben, T. (2021). Mapping weather risk– Amulti-indicator analysis of satellite-based weather data for agricultural index insurance development in semi-arid and arid zones of Central Asia Climate services, 23, 100251. https://doi.org/10.1016/j.cliser.2021.100251
- Eswar, D., Karuppusamy, R., & Chellamuthu, S. (2021). Drivers of soil salinity and their correlation with climate change. Current Opinion in Environmental Sustainability, 50, 310–318. https://doi.org/10.1016/j.cosust.2020.10.015
- FAO. (2023). FAOSTAT. Value of agricultural production. https://www.fao.org/faostat/en/#data/QV
- Frenken, K. (2013). Irrigation in Central Asia in Figures: AQUASTAT survey – 2012 (FAO Water Reports, 39).
- Global Rainfall Map in Near-Real-Time (GSMaP_NRT). (2023). JAXA global rainfall watch’ was produced and distributed by the earth observation research center. Japan Aerospace Exploration Agency.
- Gosling, S. N., & Arnell, N. W. (2016). A global assessment of the impact of climate change on water scarcity. Climate Change, 134(3), 371–385. https://doi.org/10.1007/s10584-013-0853-x
- Greene, R., Timms, W., Rengasamy, P., Arshad, M., & Cresswell, R. (2016). Soil and aquifer salinization: Toward an integrated approach for salinity management of groundwater. In A. J. Jakeman, O. Barreteau, R. J. Hunt, J. D. Rinaudo, & A. Ross (Eds.), Integrated groundwater management: Concepts, approaches and challenges (pp. 377–412). Springer.
- Guo, J. H., Liu, X. J., Zhang, Y., Shen, J. L., Han, W. X., Zhang, W. F., Zhang, Y., Zhang, W. F., Christie, P., Goulding, K. W. T., Vitousek, P. M., & Zhang, F. S. (2010). Significant acidification in major Chinese croplands. Science, 327(5968), 1008–1010. https://doi.org/10.1126/science.1182570
- Hatfield, G. L., Stanish, W. D., & Hubley-Kozey, C. L. (2015). Relationship between knee adduction moment patterns extracted using principal component analysis and discrete measures with different amplitude normalizations: Implications for knee osteoarthritis progression studies. Clinical Biomechanics, 30(10), 1146–1152. https://doi.org/10.1016/j.clinbiomech.2015.08.011
- Ibrakhimov, M., Khamzina, A., Forkutsa, I., Paluasheva, G., Lamers, J. P. A., Tischbein, B., Vlek, P. L. G., & Martius, C. (2007). Groundwater table and salinity: Spatial and temporal distribution and influence on soil salinization in Khorezm region (Uzbekistan, Aral Sea Basin). Irrigation and Drainage Systems, 21(3), 219–236. https://doi.org/10.1007/s10795-007-9033-3
- IIASA. (2023). SSP database (Online). International Institute for Applied Systems Analysis. Retrieved July 4, 2023, from https://secure.iiasa.ac.at/web-apps/ene/SspDb
- Intergovernmental Panel on Climate Change. (2022). Climate change 2022. Impacts, adaption and vulnerability. Summary for Policymakers. Retrieved June 31, 2023, from https://www.ipcc.ch/
- Khalikulov, Z., Sharma, R. C., Amanov, A., & Morgounov, A. (2016). The history of wheat breeding in Uzbekistan (Vol. 3). World Wheat Book.
- Khasanov, S., Kulmatov, R., Li, F., van Amstel, A., Bartholomeus, H., Aslanov, I., Sultonov, K., Kholov, N., Liu, H., & Chen, G. (2023). Impact assessment of soil salinity on crop production in Uzbekistan and its global significance. Agriculture Ecosystems and Environment, 342, 108262. https://doi.org/10.1016/j.agee.2022.108262
- Kienzler, K. M., Djanibekov, N., & Lamers, J. P. (2011). An agronomic, economic and behavioral analysis of N application to cotton and wheat in post-Soviet Uzbekistan. Agricultural Systems, 104(5), 411–418. https://doi.org/10.1016/j.agsy.2011.01.005
- Kirui, O., Kornher, L., & Bekchanov, M. (2023). Productivity growth and the role of mechanisation in African agriculture. Agrekon, 62(1), 80–97. https://doi.org/10.1080/03031853.2023.2176894
- Kuhn, L., & Bobojonov, I. (2023). The role of risk rationing in rural credit demand and uptake: Lessons from Kyrgyzstan. Agricultural Finance Review, 83(1), 1–20. https://doi.org/10.1108/AFR-04-2021-0039
- Lobell, D. B., Schlenker, W., & Costa-Roberts, J. (2011). Climate trends and global crop production since 1980. Science, 333(6042), 616–620. https://doi.org/10.1126/science.1204531
- Lombardozzi, L., & Djanibekov, N. (2021). Can self-sufficiency policy improve food security? An inter-temporal assessment of the wheat value-chain in Uzbekistan. Eurasian Geography and Economics, 62(1), 1–20. https://doi.org/10.1080/15387216.2020.1744462
- Lu, Y., Yu, L., Li, W. J., & Aleksandrova, M. (2022). Impacts and synergies of weather index insurance and microcredit in rural areas: A systematic review. Environmental Research Letters, 17(10), 103002. https://doi.org/10.1088/1748-9326/ac9244
- Ministry of Water Resources of the Republic of Uzbekistan. (2020). Data on irrigation and salinity.
- Mirzabaev, A. (2013). Impacts of weather variability and climate change on agricultural revenues in Central Asia. Quarterly Journal of International Agriculture, 52(892–2016–65182), 237–252. https://doi.org/10.22004/ag.econ.173648
- Mukhopadhyay, R., Sarkar, B., Jat, H. S., Sharma, P. C., & Bolan, N. S. (2021). Soil salinity under climate change: Challenges for sustainable agriculture and food security. Journal of Environmental Management, 280, 111736. https://doi.org/10.1016/j.jenvman.2020.111736
- Okur, B., & Örçen, N. (2020). Soil salinization and climate change. In Climate change and soil interactions (pp. 331–350). Elsevier. https://doi.org/10.1016/B978-0-12-818032-7.00012-6
- O’Neill, B. C., Tebaldi, C., van Vuuren, D. P., Eyring, V., Friedlingstein, P., Hurtt, G., Knutti, R., Kriegler, E., Lamarque, J.-F., Lowe, J., Meehl, G. A., Moss, R., Riahi, K., & Sanderson, B. M. (2016). The scenario model intercomparison project (ScenarioMIP) for CMIP6. Geoscientific Model Development, 9(9), 3461–3482. https://doi.org/10.5194/gmd-9-3461-2016
- Ortiz-Bobea, A., Ault, T. R., Carrillo, C. M., Chambers, R. G., & Lobell, D. B. (2021). Anthropogenic climate change has slowed global agricultural productivity growth. Nature Climate Change, 11(4), 306–312. https://doi.org/10.1038/s41558-021-01000-1
- Ortiz-Bobea, A., & Tack, J. (2018). Is another genetic revolution needed to offset climate change impacts for US maize yields? Environmental Research Letters, 13(12), 124009. https://doi.org/10.1088/1748-9326/aae9b8
- Reyer, C. P. O., Otto, I. M., Adams, S., Albrecht, T., Baarsch, F., Cartsburg, M., Coumou, D., Eden, A., Ludi, E., Marcus, R., Mengel, M., Mosello, B., Robinson, A., Schleussner, C. F., Serdeczny, O., & Stagl, J. (2017). Climate change impacts in Central Asia and their implications for development. Regional Environmental Change, 17(6), 1639–1650. https://doi.org/10.1007/s10113-015-0893-z
- Rodell, M., Houser, P. R., Jambor, U., Gottschalck, J., Mitchell, K., Meng, C.-J., Arsenault, K., Cosgrove, B., Radakovich, J., Bosilovich, M., Entin, J. K., Walker, J. P., Lohmann, D., & Toll, D. (2004). The global land data assimilation system. Bulletin of the American Meteorological Society, 85(3), 381–394. https://doi.org/10.1175/BAMS-85-3-381
- Sadozai, K. N., Khan, N. P., Jan, A. U., & Hameed, G. (2019). Assessing the impact of climate change on wheat productivity in Khyber Pakhtunkhwa, Pakistan. Sarhad Journal of Agriculture, 35(2), 594–601.
- Schlenker, W., Hanemann, W. M., & Fisher, A. C. (2005). Will US agriculture really benefit from global warming? Accounting for irrigation in the hedonic approach. American Economic Review, 95(1), 395–406. https://doi.org/10.1257/0002828053828455
- Sommer, R., Glazirina, M., Yuldashev, T., Otarov, A., Ibraeva, M., Martynova, L., Bekenov, M., Kholov, B., Ibragimov, N., Kobilov, R., Karaev, S., Sultonov, M., Khasanova, F., Esanbekov, M., Mavlyanov, D., Isaev, S., Abdurahimov, S., Ikramov, R., Shezdyukova, L., & de Pauw, E. (2013). Impact of climate change on wheat productivity in Central Asia. Agriculture Ecosystems and Environment, 178, 78–99. https://doi.org/10.1016/j.agee.2013.06.011
- State Statistics Committee of Uzbekistan. (2020). Data on cropland area, crop yields, crop output.
- State Statistics Committee of Uzbekistan. (2022). Online open data. Agriculture, demography. https://stat.uz/en/official-statistics/agriculture; https://stat.uz/en/official-statistics/demography
- Van Vuuren, D. P., Edmonds, J. A., Kainuma, M., Riahi, K., Thomson, A. M., Hibbard, K. A., Hurtt, G., Kram, T., Krey, V., Lamarque, J.-F., Masui, T., Meinshausen, M., Nakicenovic, N., Smith, S. J., & Rose, S. K. (2011). The representative concentration pathways: An overview. Climatic Change, 109(1–2), 5–31. https://doi.org/10.1007/s10584-011-0148-z
- Van Vuuren, D. P., Kriegler, E., O’Neill, B. C., Ebi, K. L., Riahi, K., Carter, T. R., Edmonds, J., Hallegatte, S., Kram, T., Mathur, R., & Winkler, H. (2014). A new scenario framework for climate change research: Scenario matrix architecture. Climatic Change, 122(3), 373–386. https://doi.org/10.1007/s10584-013-0906-1
- World Bank. (2022). Climate change knowledge portal. Retrieved August 6, 2022, from https://climateknowledgeportal.worldbank.org/country/uzbekistan/climate-data-historical
- Zhu, Z. L., & Chen, D. L. (2002). Nitrogen fertilizer use in China–Contributions to food production, impacts on the environment and best management strategies. Nutrient Cycling in Agroecosystems, 63(2/3), 117–127. https://doi.org/10.1023/A:1021107026067