Abstract
In the present study, we hypothesized that changes in perfusion pressure result in altered expression of mRNA and protein encoding for the ETA‐, ETB‐, AT1‐ and AT2‐receptors in rat mesenteric vessels. Segments of the rat mesenteric artery were cannulated with glass micropipettes, pressurized and luminally perfused in a perfusion chamber. After either exposure to no (“organ culture” (0 mmHg)), normal (85/75 mmHg) or high pressure (160/150 mmHg) at constant flow for 1–17 h, the vessel segments were snap frozen and real‐time polymerase chain reaction was performed to quantify the ET‐ and AT‐receptor mRNA content, or immersed in a fixative solution, dehydrated, frozen, cut in a cryostat and immunohistology stained for ET‐ and AT‐receptor protein. The mRNA expressions of ETB and of AT2 were significantly enhanced in vessels exposed to high perfusion pressure, compared with normal and no perfusion pressure at 4 h. In concordance, AT1‐, AT2‐ and ETB‐receptor proteins were up‐regulated at 17 h of high perfusion pressure. In conclusion, the results from our rat perfusion model suggest a more important role of shear stress than pure pressure alone and may serve as a surrogate model for studies designed to investigate hypertension mechanisms.
Introduction
Changes of the endothelin‐1 (ET) and the angiotensin II (AT) systems are considered to play important roles in the pathophysiology of cardiovascular disease. The relationship between the AT and the ET systems is complex, and their participation in pathophysiology of hypertension remains to be clarified. One example of the complexity is illustrated by the fact that AT succeeds in stimulating ET‐1 expression by both endothelium and smooth muscle cells (SMC) in the vascular wall in vivo as well as in vitro Citation[1],Citation[2]. ET‐1 is the most potent endogenous vasoconstrictive substance yet known. It exerts its effects through the ETA‐ and ETB‐receptors. ETA‐ and ETB‐receptors on SMC induce contraction and stimulate proliferation and cell hypertrophy Citation[3]. Endothelial ETB‐receptors stimulate the production of NO and prostacyclin and, accordingly, elicit vasorelaxation Citation[4]. In physiology, the administration of ET‐1 elicits first a transient relaxation followed by sustained contraction. However, in pathophysiology this pattern may be altered toward a more constrictive phenotype, hence the vasoconstrictor action of ET‐1 is the predominant effect. A similar relationship is thought to exist between the AT1‐ and AT2‐receptors; whereas AT II induces vasoconstriction via AT1‐receptors on SMC Citation[5], it promotes vasodilatation via AT2‐receptors on endothelial cells Citation[6].
ET‐1 is over‐expressed in the vascular wall in certain rat models of experimental hypertension, whereas in others it is not Citation[7]. It has been shown that elevated perfusion pressure up‐regulates ET‐1 and ETB‐receptor expression in the rabbit carotid artery Citation[8]. Examination of the expression of prepro‐ET‐1 mRNA in small arteries from gluteal subcutaneous biopsies, normotensive subjects and mildly hypertensive patients revealed a similar expression of endothelial prepro‐ET‐1 mRNA, whereas in moderately to severely hypertensive patients, the ET‐1 expression in the endothelium of small arteries was significantly increased Citation[9]. The contractile ETB‐receptor is up‐regulated in atherosclerosis, congestive heart failure, subarachnoidal hemorrhage and ischemic stroke Citation[10–13]. In these conditions, it might contribute to pathological vasomotor function. The AT1‐ and AT2‐receptors have been shown to be down‐regulated in heart failure Citation[14] and the AT1‐receptor was also reduced in ischemic heart disease Citation[15].
The data regarding the regulation of ET‐1 synthesis and release by shear stress forces on endothelial cells are controversial, since some reports have described a shear stress‐dependent induction of ET‐1 production, others have found no significant changes in the ET‐1 release, and still others have shown down‐regulation. Long‐term arterial laminar shear stress has been found to up‐regulate the ETB‐receptor Citation[16]. In yet another study, elevated pressure without shear stress showed enhanced ET‐1 release from human umbilical vein endothelial cells Citation[17]. The effects on AT‐receptors by shear stress are to us largely unknown.
We have demonstrated that in rat and man there is G‐protein coupled receptor (GPCR) up‐regulation of vascular SMC in hypertension Citation[18]. The receptor up‐regulation may contribute to the development of hypertension and its complications. Both transcriptional and posttranscriptional mechanisms are involved in the receptor up‐regulation in vascular SMC. However, molecular mechanisms that lead to GPCR receptor up‐regulation are still not fully understood.
The present study was carried out to examine whether shear stress is essential for transcriptional up‐regulation of GPCR receptors in hypertension (elevation of intraluminal pressure).
Methods
Tissue preparation
Sprague–Dawley rats (body weight 250–350 g) were anesthetized with CO2 and exsanguinated. The mesenteric artery, including primary and secondary branches, was gently removed, immersed into cold buffer solution (for composition, see below) and freed of adhering tissue under a dissection microscope. The Lund University Animal Ethic's Committee approved the experimental protocol.
Buffer solutions
Standard buffer solution (mM): NaCl 119; NaHCO3 15; KCl 4.6; MgCl 1.2; NaH2PO4 1.2; CaCl2 1.5; glucose 5.5. Analytical grade chemicals and double distilled water were used for preparing all solutions.
In vitro perfusion pressure and shear stress
Segments of the primary or secondary branch from the rat mesenteric artery were cannulated with glass micropipettes, luminally perfused in a temperature‐controlled (37°C) perfusion chamber and either exposure to no pressure (“organ culture”, n=6; normal, 85/75 mmHg, n=6; or high pressure, 160/150 mmHg, n=6) and at constant flow. One experiment was also performed without any flow, but with pure pressure alone (160/150 mmHg).
Real‐time reverse transcription polymerase chain reaction (RT‐PCR)
Artery segments were homogenized in 1 ml of RNApro solution (Q‐BIOgene, CA, USA). In order to do so a FastPrep instrument from the same manufacturer was used. Following a protocol from the FastRNA Pro kit supplier the total RNA was extracted and checked by a spectrophotometer, GeneQuant Pro (Amersham Biosciences, Cambridge, UK). Using TaqMan Reverse Transcription Reagents (PE Applied Biosystems, CA, USA) in a Perkin–Elmer 2400 PCR (Perkin–Elmer, MA, USA) machine at 42°C for 30 min, reverse transcription (RT) of total RNA to cDNA was carried out. The GeneAmp SYBR Green PCR kit in a GeneAmp 5700 sequence detection system (Perkin–Elmer) was used in order to perform real‐time quantitative PCR. The system automatically monitors the binding of fluorescent dye to double‐strand DNA by real‐time detection of the fluorescence during each cycle of PCR amplification.
The PCR reaction was performed in a 50 µl volume and started at a temperature of 50°C for 2 min, 95°C for 10 min and the following 40 PCR cycles with 95°C for 15 s and 60°C for 1 min. After the real‐time PCR, dissociation curves were run to identify the specific PCR products. The primers were designed using the Primer Express 2.0 software (PE Applied Biosystems) and synthesized by TAG Copenhagen A/S (Copenhagen, Denmark). Specific primers for the rat ETA (DQ839234), ETB (GeneBank accession no. X57764), AT1a (GeneBank accession no. NM030985) and AT2 receptor mRNA (GeneBank accession no. D16840) were designed as follows:
ETA receptor:
Forward: 5′‐GTCGAGAGGTGGCAAAGACC‐3′
Reverse: 5′‐ACAGGGCGAAGATGACAACC‐3′
ETB receptor:
Forward: 5′‐GATACGACAACTTCCGCTCCA‐3′
Reverse: 5′‐GTCCACGATGAGGACAATGAG‐3′
AT1a receptor:
Forward: 5′‐CACAGTGTGCGCGTTTCATT‐3′
Reverse: 5′‐TGGTAAGGCCCAGCCCTAT‐3′
AT2 receptor
Forward: 5′‐TCTGTTAGTGGGATGCATGTAATCA‐3′
Reverse: 5′‐TGTGGGCCTCCAAACCATT‐3′
Elongation factor‐1 (EF‐1, GeneBank accession no. BC111707) and glyceraldehyde 3‐phosphate dehydrogenase (GAPDH, GeneBank accession no. DQ403053) mRNA were used as references, since they are continuously expressed to a constant amount in cells. The EF‐1 and GAPDH primers were designed as follows:
EF‐1:
Forward: 5′‐GCAAGCCCATGTGTGTTGAA‐3′
Reverse: 5′‐TGATGACACCCACAGCAACTG‐3′
GAPDH:
Forward: 5′‐GGCCTTCCGTGTTCCTACC‐3′
Reverse: 5′‐CGGCATGTCAGATCCACAAC‐3′
Data were analyzed with the comparative cycle threshold (CT) method. To evaluate the amount of mRNA in the sample, EF‐1 and GAPDH mRNA were assessed in the same sample simultaneously. The CT values of EF‐1 and GAPDH mRNA were used as a reference to quantify the relative amount of ETA, ETB, AT1, AT2 receptor mRNA. The relative amount of mRNA was calculated with the CT values of ETA, ETB, AT1, AT2 receptor mRNA in relation to the CT values of EF‐1 and GAPDH mRNA in the sample.
mRNA stability
To study if a role of mRNA stability was involved in the up‐regulation of ETB‐ and AT2‐receptors, the mesenteric artery segments were perfused with Actinomycin D (AcD) for 2 h (n=6) and 4 h (n=6) added to the buffer solution; thus the AcD was both in the perfusion chamber (extraluminally) and intraluminally. The administration of AcD results in inhibition of any de novo synthesis of receptor mRNA. Real‐time RT‐PCR was used to determine the amount of the receptor mRNA degradation.
Immunohistochemistry
After the mesenteric artery segments had been exposed to organ culture, normal or high perfusion pressure, they were immersed in a fixative solution consisting of 4% paraformaldehyde in 0.1 M phosphate buffer (pH 7.4) for 3 h at 4°C. After fixation, the specimens were dehydrated in a 20% sucrose phosphate buffer (0.1 M, pH 7.4) for 24 h at 4°, and then frozen in Tissue‐Tek (Sakura Finetek Europe B.V., Zoeterwoude, Netherlands) and stored at −80°C. Sections were cut at 10‐µm thickness in a cryostat and mounted on SupperFrost Plus slides (Menzel GMBH & COKG, Braunschweig, Germany). Immunohistochemistry were carried out using standard protocols, i.e. the sections were incubated with the primary antibody overnight at 4°C and the secondary antibody for 1 h at room temperature in the dark. Primary and secondary antibodies as well as the dilutions were used as follows: AT1 receptor (1:50, mouse, Abcam plc, Cambridge, UK), AT2 receptor (1:200, rabbit, Abcam plc), ETA receptor (1:50, sheep, Alexis Biochemical, Lausen, Switzerland), ETB receptor (1:100, rabbit, Abcam plc). The appropriate secondary antibodies, goat anti‐mouse IgG H&L (1:200, Abcam plc), goat anti‐rabbit IgG H&L (1:200, Abcam plc), or donkey anti‐sheep IgG H&L (1:200, Abcam plc) conjugated to fluorescein isothiocynate (FITC) was used for fluorescence microscopic imaging. In the control experiments, either the primary antibody or the secondary antibody was omitted. The stained specimens were observed under a confocal microscope (Nikon, C1plus, Nikon Instruments Inc., NY, USA). The fluorescence intensity was measured and analyzed by Image J software (http://rsb.info.nih.gov/ij). For each specimen, six randomly selected sections were studied. In each section, the fluorescence intensity was measured at six preset areas and the mean value of fluorescence intensity was obtained.
Data analysis
All data are expressed as mean±SEM. The amount of receptor mRNA is expressed as percentage of control group or amount of CT values in relation to the house keeping genes. The unpaired Student's t‐test was used to compare two sets of data. When three or more sets of data were compared, the two‐way ANOVA‐test was implemented. A p‐value less than 0.05 was considered significant.
Results
Receptor mRNA expression and mRNA stability
The expression of ETB (p<0.05) and AT2 (p<0.05) mRNA was significantly increased in artery segments exposed to high perfusion pressure, compared with normal perfusion pressure and fresh vessels after 4 h (). The 2‐h perfusion did not result in a significant change in receptor mRNA expression (data not shown).
Figure 1. ETB and AT2 mRNA expression from rat mesenteric artery. No perfusion pressure (C), normal perfusion pressure (NP) and high perfusion pressure (HP) for 4 h. *p<0.05.
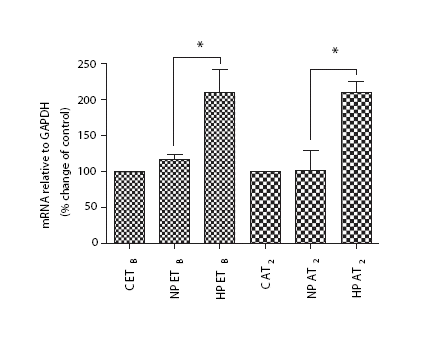
In order to understand the reasons behind the enhanced expression of ETB and AT2, we analyzed if the expression could be the result of altered mRNA stability. We found that the high perfusion pressure had no influence on the mRNA stability of the receptors, compared with normal pressure ( and ). Thus, the observed increase was true in .
Figure 2. (a) ETB mRNA expression from rat mesenteric artery perfused with Actinomycin D. Organ culture (OC), normal perfusion pressure (NP) and high perfusion pressure (HP) for 2 and 4 h of incubation. (b) AT2 mRNA expression from rat mesenteric artery perfused with Actinomycin D. Organ culture (OC), normal perfusion pressure (NP) and high perfusion pressure (HP) for 2 and 4 h of incubation.
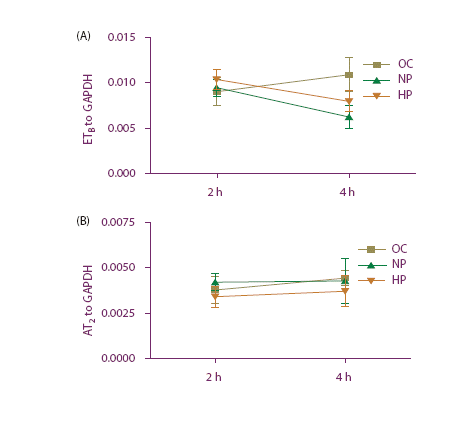
ETA (p=0.60) and AT1 (p=0.45) expressions were not significantly altered when exposed to high perfusion pressure compared with normal perfusion pressure for 4 h. When the vessels were exposed to pressure only without perfusion, no significant alteration in expression was seen for any of the receptors (p>0.05).
Receptor protein expression
In order to study if the receptor mRNA translates to receptor protein, we carried out a series of experiments at both short (1‐ and 3 h) and long‐term (17 h) pressure exposure. ETB‐receptor protein expression was up‐regulated after 17 h of high perfusion pressure compared with 1 h of high perfusion pressure, and there was a clear tendency towards up‐regulation of ETB‐receptor protein compared with 17 h of normal perfusion pressure (). There was no difference in ETB‐receptor expression between normal pressure and high pressure at 1 and 3 h of exposure (). High pressure for 17 h resulted in a significantly elevated ETB‐receptor expression (p<0.05) as compared with normal pressure or high pressure for 1 h (). Normal pressure for 17 h did not alter the ETB‐receptor expression (p>0.05). There was no significant change in the ETA‐receptor protein expression (data not shown).
Figure 3. ETB protein expression from rat mesenteric artery. Normal perfusion pressure (NP) and high perfusion pressure (HP) for 1, 3 and 17 h. *p<0.05.
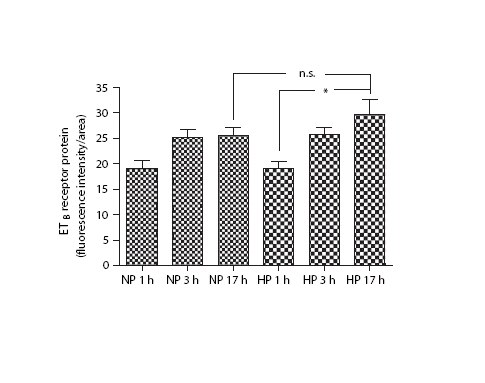
There was enhanced expression of both AT1 and AT2 receptors at 17 h of elevated perfusion pressure ( and ). The normal pressure exposure for 1–17 h did not alter AT1 or AT2 receptor expression; high‐pressure exposure for only 1 or 3 h was apparently too short to alter the expression ().
Figure 4. (a) AT1 protein expression from rat mesenteric artery. Normal perfusion pressure (NP) and high perfusion pressure (HP) for 1, 3 and 17 h. *p<0.05. (b) AT2 protein expression from rat mesenteric artery. Normal perfusion pressure (NP) and high perfusion pressure (HP) for 1, 3 and 17 h. *p<0.05.
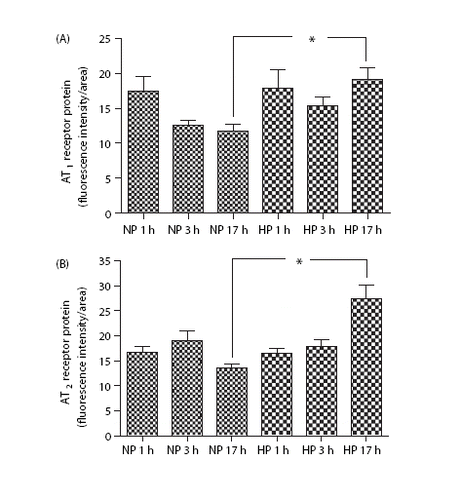
Localization of receptor expression
We evaluated the precise site of the enhanced expression of endothelin and angiotensin receptors with high‐power confocal microscopy. In , the immunohistochemistry results are shown for AT1, AT2 and ETB for both normal perfusion pressure (NP) and high perfusion pressure (HP) and 1 h and 17 h. Clear up‐regulation can be seen. With co‐localization studies using SMC actin, we verified that the up‐regulation takes place in the cytoplasm of the vessel wall SMC (); that the changes take place in the SMC is also apparent, since we had removed the adventitial layer before mounting the vessels on the pipettes and did not see any clear receptor alterations in the endothelium.
Figure 5. (a) Immunohistochemistry. Left top: AT1 protein expression during normal perfusion pressure (NP) and 1 h. Right top: AT1 protein expression during NP and 17 h. Left bottom: AT1 protein expression during high perfusion pressure (HP) and 1 h. Right bottom: AT1 protein expression during HP and 17 h. (b) Immunohistochemistry. Left top: AT2 protein expression during NP and 1 h. Right top: AT2 protein expression during NP and 17 h. Left bottom: AT2 protein expression during HP and 1 h. Right bottom: AT2 protein expression during HP and 17 h. (c) Immunohistochemistry. Left top: ETB protein expression during NP and 1 h. Right top: ETB protein expression during NP and 17 h. Left bottom: ETB protein expression during HP and 1 h. Right bottom: ETB protein expression during HP and 17 h.
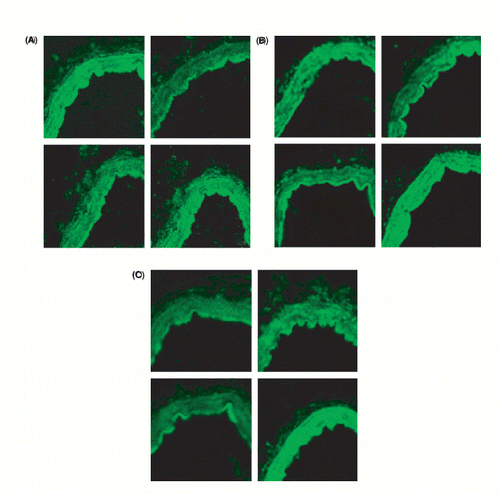
Discussion
The present study is the first to show that enhanced perfusion pressure at constant flow (elevated shear stress) results in enhanced expression, first of ET and AT receptor mRNA (4 h), and later in receptor protein expression (17 h). Thus, the present study has revealed that wall shear stress of flowing fluid is essential for transcriptional up‐regulation of GPCR receptors putatively occurring in hypertension, and that shear stress‐induced activation of intracellular signal transductions is involved in this process. We showed that there was an up‐regulation of the ETB‐ and AT2‐receptor mRNA in the mesenteric artery SMC when exposed to high perfusion pressure, but not when the vessels were exposed to normal pressure. Studies of ET‐receptors and shear stress have before shown conflicting results. Our study focused mainly on the ET‐receptors, ETB and ETA, while previous experiments have shown enhanced synthesis of the ET‐1 peptide and of prepro‐ET‐1 mRNA expression Citation[19],Citation[20] or made use of cultured SMC instead of whole vessels Citation[21]. If one assumes that ET‐1 up‐regulation leads to the up‐regulation of the ETB‐receptor, then our study might be in line with these previous results. Alternatively, the enhanced ET‐1 production will lead to enhanced contraction per se, but also via the increased presence of contractile ETB‐receptors. Unfortunately, the problem still exists that different methods and different definitions of shear stress have been used that cannot easily be translated into effective direct comparisons between studies. Furthermore, there are studies that have shown an early transient up‐regulation of the ET‐1 gene expression followed by a sustained suppression, making the release of ET‐1 to shear stress biphasic Citation[22]. Also, it is important to be aware of factors other than high perfusion pressure alone that can alter the expression of the endothelin system Citation[23]. Our results bring novel insight into the role of shear stress in whole mesenteric artery segments and closer to the setting of hypertension. When it comes to the AT‐receptors, hypertension and wall shear stress, studies are lacking and our study is novel in showing that the AT2‐receptor mRNA is up‐regulated under these circumstances and that this occurs, as for the ETB‐receptors, also in the SMC.
Our results have shown that there is an activation of the angiotensin/endothelin systems at high perfusion pressure that differs from that seen in “normal perfusion pressure”. This is in line with previous animal and human studies Citation[8],Citation[9]. We observed a specific up‐regulation of the ETB‐ and AT2‐receptor mRNA in high perfusion pressure compared with normal perfusion pressure after 4 h. Because the up‐regulation was sensitive to Actinomycin D, it suggests that it is controlled at the level of mRNA synthesis. We speculate whether these receptors are activated in an early phase of a blood pressure increase to cause/worsen hypertension or act as a negative feedback system. The AT1‐receptor showed up‐regulation but not the ETA‐receptor, which are known to cause vasoconstriction. Both the ETB‐ and AT2‐receptors were up‐regulated and are known to mediate endothelium‐dependent vasodilatation when present on the endothelium. However, here the expression was seen on the SMC, which is novel and potentially important. As previously noted, the ETB‐receptors can change phenotype to a contractile receptor located in the SMC. It is possible that the ETB vasodilatation is predominant at normotension, but hypothetically, a contractile phenotype is expressed at the same time, which alters the balance of effects at the vascular ETB‐receptor from a favored vasodilatation in healthy men Citation[24] to a vasoconstrictor in hypertension. It is less clear whether this would be the case in a high‐pressure environment. Experiments measuring possible alterations of receptor expression when perfusing vessels with and without an endothelium might be helpful in this regard.
The immunohistology results showed a significant up‐regulation of the AT1‐ and AT2‐receptor protein after 17 h of high perfusion pressure, as well as that of the ETB‐receptor protein as compared with normal pressure. This can be especially appreciated since the ETB‐receptor protein showed a significant up‐regulation from 1 to 17 h of exposure to a high perfusion pressure state. The time‐endpoints (1, 3 and 17 h) in this study were partly designed on the basis of previous study results. There was no significant change in protein expression between normal and high perfusion pressure of any of the receptors at 1 and 3 h of perfusion at either pressure. This was largely expected, since it was unlikely for translation to have had enough time to produce sufficient amounts of finished protein structure at this time. The enhanced ETB‐receptor mRNA and protein expression in hypertension shown in our study is in line with the up‐regulation of this receptor in atherosclerosis, congestive heart failure, subarachnoidal hemorrhage and ischemic stroke (Citation[10–13)]. In heart failure, for instance, levels of ET‐1 have been observed to be elevated. It is possible that these high levels of ET‐1, acting on the ETB‐receptor, may contribute to the increase in vascular tone seen in heart failure as well as in hypertension. It is important to keep in mind that in the present study we examined the effects of high perfusion pressure in the rat mesenteric artery, whereas in other studies human vessels from the coronary and cerebral arteries have been examined. The enhanced AT1‐ and AT2‐receptor protein expression seen in our study is in opposition to the reduced expression of these receptors seen in heart failure and ischemic heart disease Citation[14],Citation[15].
In conclusion, we have demonstrated a specific up‐regulation of the ETB‐ and AT2‐receptor mRNA at high perfusion pressure compared with normal perfusion pressure. Furthermore, this resulted in up‐regulation of the ETB‐receptor protein and enhanced AT1‐ and AT2‐receptor protein expressions after high perfusion pressure a few hours later when the mRNA had time to produce the protein. This demonstrates that there might be an occurrence of GPCR changes in early hypertension.
Acknowledgments
The Swedish Research Council (grant no. 5958) and The Heart–Lung Foundation.
Declaration of interest: The authors report no conflicts of interest. The authors alone are responsible for the content and writing of the paper.
References
- Rajagopalan S, Laursen JB, Borthayre A, Kurz S, Keiser J, Haleen S, et al. Role for endothelin‐1 in angiotensin II‐mediated hypertension. Hypertension 1997; 30: 29–34
- Ito H, Hirata Y, Adachi S, Tanaka M, Tsujino M, Koike A, et al. Endothelin‐1 is an autocrine/paracrine factor in the mechanism of angiotensin II‐induced hypertrophy in cultured rat cardiomyocytes. J Clin Invest 1993; 92: 398–403
- De Nucci G, Thomas R, D' Orleans‐, Juste P, Antunes E, Walder C, Warner TD, et al. Pressor effects of circulating endothelin are limited by its removal in the pulmonary circulation and by the release of prostacyclin and endothelium‐derived relaxing factor. Proc Natl Acad Sci USA 1988; 85: 9797–9800
- Schiffrin EL. Endothelin: Potential role in hypertension and vascular hypertrophy. brief review. Hypertension 1995; 25: 1135–1143
- Ytterberg H, Edvinsson L. Evidence for a cyclic AMP‐dependent pathway in AT1‐receptor activation of human omental arteries. JRAAS 2001; 2: 42–47
- Carey RM, Wang ZQ, Siragy HM. Update: role of the angiotensin type‐2 (AT (2)) receptor in blood pressure regulation. Curr Hypertens Rep 2000; 2: 198–201
- Schiffrin EL. Role of endothelin‐1 in hypertension. Hypertension 1999; 34: 876–881
- Lauth M, Berger M, Cattaruzza M, Hecker M. Elevated perfusion pressure up regulates endothelin‐1 and endothelin B receptor expression in the rabbit carotid artery. Hypertension 2000; 35: 648–654
- Schiffrin EL, Deng LY, Sventek P, Day R. Enhanced expression of endothelin‐1 gene in endothelium of resistance arteries in severe human essential hypertension. J Hypertens 1997; 15: 57–63
- Dagassan PH, Breu V, Clozel M, Künzli A, Vogt P, Turina M, et al. Up‐regulation of endothelin‐B receptors in atherosclerotic human coronary arteries. J Cardiovasc Pharmacol 1996; 27: 147–53
- Cannan CR, Burnett JC, Lerman A. Enhanced coronary vasoconstriction to endothelin‐B‐receptor activation in experimental congestive heart failure. Circulation 1996; 93: 646–651
- Roux S, Loffler BM, Gray GA, Sprecher U, Clozel M, Clozel JP. The role of endothelin in experimental cerebral vasospasm. Neurosurgery 1995; 37: 78–85
- Stenman E, Malmsjo M, Uddman E, Gidö G, Wieloch T, Edvinsson L. Cerebral ischemia upregulates vascular endothelin ET (B) receptors in rat. Stroke 2002; 33: 2311–2316
- Wackenfors A, Pantev E, Emilson M, Edvinsson L, Malmsjo M. Angiotensin II receptor mRNA expression and vasoconstriction in human coronary arteries: effects of heart failure and age. Basic Clin pharmacol Toxicol 2004; 95: 266–272
- Wackenfors A, Emilson M, Ingemansson R, Edvinsson L, Malmsjo M. Ischemic heart disease down‐regulates angiotensin type 1 receptor mRNA in human coronary arteries. Eur J Pharmacol 2004; 503: 147–153
- Morawietz H, Talanow R, Szibor M, Rueckschloss U, Schubert A, Bartling B, et al. Regulation of the endothelin system by shear stress in human endothelial cells. J Physiology 2000; 523(3)761–770
- Hishikawa K, Nakaki T, Marumo T, Suzuki H, Kato R, Saruta T. Pressure enhances endothelin‐1 release from cultured human endothelial cells. Hypertension 1995; 25: 449–452
- Li J, Cao YX, Liu H, Xu CB. Enhanced G‐protein coupled receptors‐mediated contraction and reduced endothelium‐dependent relaxation in hypertension. Eur J Pharmacol 2007; 557: 186–194
- Macarthur H, Warner TD, Wood EG, Corder R, Vane JR. Endothelin‐1 release from endothelial cells in culture is elevated both acutely and chronically by short periods of mechanical stretch. Biochem Biophys Res Commun 1994; 200: 395–400
- Wang DL, Wung BS, Peng YC, Wang JJ. Mechanical strain increases endothelin‐1 gene expression via protein kinase C pathway in human endothelial cells. J Cell Physiol 1995; 163: 400–406
- Cattaruzza M, Eberhardt I, Hecker M. Mechanosensitive transcription factors involved in endothelium B receptor expression. J Biol Chem 2001; 276: 36999–37003
- Kuchan MJ, Frangos JA. Shear stress regulates endothelin‐1 release via protein kinase C and cGMP in cultured endothelial cells. Am J Physiol 1993; 264: H150–H156
- Marsden PA, Brenner BM. Transcriptional regulation of the endothelin‐1 gene by TNF‐alpha. Am J Physiol 1992; 262: C854–C861
- Strachan FE, Spratt JC, Wilkinson IB, Johnston NR, Gray GA, Webb DJ. Systemic blockade of the endothelin‐B receptor increases peripheral vascular resistance in healthy men. Hypertension 1999; 33: 581–585