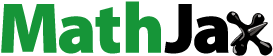
Abstract
Nundorite is a unique and enigmatic rock type, known only from its type locality within the Koonenberry Belt of western NSW. The rock is highly anomalous in geochemical composition being peralkaline, with low silica and high alumina, but enriched in rare metals such as Zr, Nb and rare earth elements. This study presents a comprehensive mineralogical and geochemical study of nundorite to resolve its origins and its potential to inform on the geological processes that lead to critical metal concentration in the crust. Nundorite is found only at a single outcrop but shows a progression of alteration and deformation over ∼250 m from the northwest to southeast. The least deformed varieties consist of coarse aegirine grains within a matrix composed predominantly of natrolite and microcline with minor albite, nepheline and schizolite. With increasing deformation, the proportion of albite and aegirine decreases, the primary zeolite changes from natrolite to analcime, and the pyroxene and schizolite shift towards the aegirine and serandite (Mn-rich) endmember compositions, respectively. These transitions can be related to progressive hydration and recrystallisation of the rock in response to hydrothermal alteration associated with deformation. Nundorite also contains a diverse suite of accessory rare metal phases such as zircon, monazite, cerite-group minerals, fergusonite, fersmite, natroniobite, galgenbergite, epidote–allanite and a range of Zr alkali silicate minerals.
Samarium–Nd isotopic compositions of nundorite (εNd ∼+5.5 at 585 Ma) are comparable with mafic volcanic rocks of the surrounding Mount Arrowsmith Volcanics and are consistent with derivation from a mantle source. These data, together with the uniform peralkaline composition, depletion in Ti and P, and enrichment in incompatible elements, are consistent with an origin as highly fractionated phonolite. Phonolites of similar composition are rare but have been reported from continental rift and intraplate alkaline provinces worldwide. Nundorite, therefore is not just a geological oddity, but also provides reaffirmation of the continental rift setting for the Mount Arrowsmith Volcanics and highlights the critical mineral resource potential of the region, as extensive crystal fractionation is a key mechanism to produce ore grade levels of rare metals in alkaline magmatic systems.
KEY POINTS
Nundorite is a geological oddity found only in the Koonenberry Belt of western NSW.
Nundorite has a high-alumina, peralkaline composition and is enriched in Zr, Nb and rare earth elements.
Nundorite has a complex accessory mineral assemblage reflecting its unusual composition and variable alteration.
The protolith of nundorite is phonolite formed after extensive crystal fractionation in a continental rift setting.
Introduction
Rare and unusual rock types, or geological ‘oddities’, have long held fascination with geologists and the general public alike, and therefore serve as important avenues to promote Earth science education and outreach. They also can provide unique insights into geological processes. For example, the orbicular granites located on Boogardie station, Western Australia, not only make spectacular dimension stone, but also provide petrological information on magma cooling and crystallisation rates (Zhang & Lee, Citation2021). Jadeitite deposits are important sources of cultural artefacts and gemstones, but also inform on metasomatic processes associated with subduction-zone metamorphism (Harlow et al., Citation2015). Geological oddities are also commonly linked with economic mineral deposits (e.g. tourmalinites of Broken Hill; Slack et al., Citation1993) and thus can be used as pathfinders or indicators of mineralisation. Therefore, despite their relative obscurity in the geological record, geological oddities commonly punch well above their weight in terms of their scientific, educational and economic significance.
This paper outlines the geological characteristics and origins of a particularly rare geological oddity, called nundorite, known only from a single locality within the Grey Range Group of the Koonenberry Belt, northwestern NSW. Nundorite is a fine-grained, tan- to brown-coloured granoblastic rock with distinctive coarse grains of pale green aegirine (). Cut and polished pieces of nundorite have been sold since the 1970s for decorative and spiritual purposes.
Figure 1. Visual and chemical features of nundorite. (a) Photo of a polished cut slab of ‘outcrop’ nundorite with green aegirine crystals evident. (b) Zirconium vs Al2O3/SiO2 of peralkaline igneous rocks from the global igneous geochemistry database of Gard et al. (Citation2019). The data were filtered for intermediate to felsic composition (>50 wt% SiO2) and evolved compositions with P2O5 <0.04 wt% and TiO2 <0.46 wt% to match the characteristics of nundorite. Note, very few rocks globally compare to the composition of nundorite.
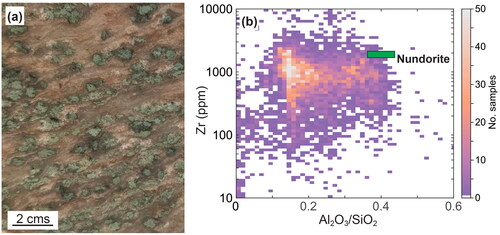
As well as its visual appeal, nundorite has an unusual chemical composition, being peralkaline ([Na + K]/Al >1), with relatively low SiO2 (∼54 wt%) but enriched in Al2O3 (>20 wt%), and rare metals such as Zr, Hf, Nb, Ta and rare earth elements (REE) (). These characteristics are unlike other rocks of the Grey Range Group, or indeed any common igneous or sedimentary protolith. For example, concentrations of Zr and Nb in nundorite are, respectively, more than one and two orders of magnitude higher than that of typical crustal rock types. Despite several previous geological studies, the mineralogy and genetic origins of nundorite remain highly contentious. It has been proposed to have originated as an intrusion (Edwards & Neef, Citation1979), pyroclastic rock (Buckley & Mills, Citation2006; Gilmore et al., Citation2009; Zhou, Citation1992, as cited in Barron, Citation2000), or to be the product of seafloor metasomatism (Gilmore et al., Citation2009) or fenitisation (metasomatism by alkali-rich fluids released from alkaline magmas) (Edwards, Citation1980). An alternative hypothesis attributes the concentration of alkalis and incompatible elements to magmatic evolution via extreme crystal fractionation (Barron, Citation2000).
Increasing demand for critical metals, such as Zr, Nb, Ta and REE, for modern technology and renewable energy is driving efforts to discover new ore deposits. Australia’s potential for deposits rich in these critical metals is demonstrated by the Toongi deposit near Dubbo, NSW (Spandler & Morris, Citation2016) and the Peak Ranges prospects in Central Queensland (Chandler & Spandler, Citation2020). In both cases, mineralisation is hosted in intraplate volcanic rocks of peralkaline composition. Nundorite also features enrichment in these metals and therefore may contribute to understanding of how critical metal ore deposits form and to evaluation of the ore potential of the Grey Range Group.
Here we present a detailed petrological evaluation of nundorite and its nearby deformed and recrystallised variants, using field observations, petrography, mineralogy and bulk-rock and Nd isotope geochemistry. Our datasets allow for a robust assessment of the origin and evolution of nundorite, which in turn contributes to a greater understanding of the understudied Grey Range Group and to understanding processes of critical mineral ore formation in alkaline rocks.
Background
Geological context
The outcrop of nundorite occurs on Nundora station in the Packsaddle district of northwestern NSW (). The outcrop lies within regional exposures of the Neoproterozoic Grey Range Group, which consists of a rift-related package of shallow marine sedimentary sequences of the Kara Formation intercalated with rocks of the Mount Arrowsmith Volcanics (MAV), a series of transitional to alkaline shallow marine to subaerial volcanic units (basalt–trachybasalt–trachyandesite–trachyte–alkali rhyolite) and shallow intrusive rocks (gabbro, diorite and syenite) (Crawford et al., Citation1997). Based on geological and geochemical characteristics, Crawford et al. (Citation1997) concluded that the MAV formed in a continental rift setting, somewhat akin to the northern sector of the East African Rift. The diversity of igneous rock types was generated primarily owing to extensive fractional crystallisation within the crust, but also in part owing to variable degrees of melting of an enriched mantle source, as well as minor levels of crustal assimilation (Crawford et al., Citation1997).
Figure 2. (a) Location, (b) geological setting of the northern Koonenberry Belt of western NSW and (c) local geological setting of the nundorite outcrop, adapted from Greenfield and Reid (Citation2010) and Mills et al. (Citation2015). Sample locations are presented by the coloured symbols, and nundorite sample locations are numbered (c).
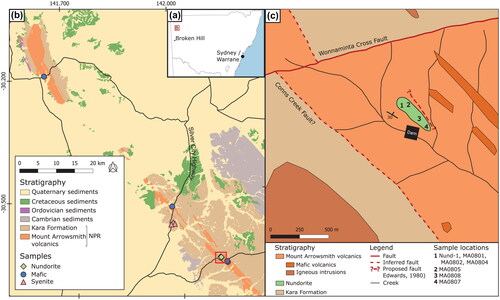
The MAV was age-dated via SHRIMP U–Pb geochronology of zircon from a trachytic tuff to ca 585 Ma (Greenfield et al., Citation2011) and is considered to be equivalent to the alkaline basalts in the Farnell Group (∼65 km to the southwest) and the Neoproterozoic Tasmanian alkaline dolerites, which intrude the Rocky Cape Group and Oonah Formation (Greenfield et al., Citation2010). More broadly, the Grey Range Group is thought to have formed in a failed continental rift environment associated with the final breakup of the supercontinent Rodinia (Crawford et al., Citation1997).
The Grey Range Group, together with the younger Cambrian sequences of the Mount Wright Arc, comprise the Wonnaminta Zone of the NW–SE-trending Koonenberry Belt (Greenfield et al., Citation2011) (). The Group has since experienced prehnite–pumpellyite to lower greenschist-facies metamorphism and multiple deformation events (Greenfield et al., Citation2010), including the Cambrian Delamerian Orogeny, which produced tight macroscopic folding, subvertical slaty cleavage and likely thrust faulting. Subsequent events included mesoscopic folding and localised higher-grade metamorphism attributed to the Silurian Benambran deformation, which was mainly concentrated in a zone through Nundora, Packsaddle and Milpa (Greenfield et al., Citation2010).
Past research on nundorite
Prior research on nundorite is limited to unpublished reports (e.g. Barron, Citation2000), theses (Edwards, Citation1980; Zhou, Citation1992) and field guides (e.g. Buckley & Mills, Citation2006), all of which highlight the enigmatic nature of the rock. Data and interpretations drawn from these documents are both inconclusive and inconsistent.
Nundorite is only confirmed to occur at a single locality (), mainly from outcrop encompassing a zone some 20 m long, up to 4 m wide and 1–1.5 m high. This outcrop is oriented parallel to the northwest–southeast-trending regional strike of the Koonenberry Belt. There is limited exposure of nundorite in surrounding subcrop extending at least 60 m to the northeast and 280 m to the southeast. Historical reports also indicate the presence of another outcrop ∼3.5 km northwest along-strike (Barron, Citation2000), but despite an extensive search, this outcrop was not found during our fieldwork. Exposure of the surrounding Grey Range Group rocks is also sparse, limited to subcrop in the surrounding flat gibber plain.
Nundorite has been subjected to regional greenschist-facies metamorphism (Greenfield et al., Citation2010) and therefore is interpreted to have formed during, or shortly after, deposition of the Grey Range Group. Previous geochemical analysis of nundorite emphasised the low silica content (<55 wt%), the anomalously high NaO2 and Al2O3 contents (Edwards, Citation1980) and enrichment in incompatible trace elements such as Zr, Nb and REEs (Greenfield et al., Citation2010). These chemical attributes are not shared by other units of the Grey Range Group. Whether this unusual composition is a primary feature or a result of alteration/metasomatism remains unknown to date.
Owing to its fine-grained nature and unusual composition, the mineralogy of nundorite is not well defined. It was initially described as a cordierite–anthophyllite rock (cited in Buckley & Mills, Citation2006) but has since been established to be an aegirine-bearing rock. Characterisation of the alkaline-silicate groundmass remains contentious, with the proposed mineralogy variably including albite, nepheline, natrolite, analcime, microcline and sanidine (Barron, Citation2000; Buckley & Mills, Citation2006; Edwards, Citation1980; Edwards & Neef, Citation1979; Gilmore et al., Citation2009). The groundmass shows a clear fabric in thin-section, although it is debated whether this is a tectonic foliation (Edwards, Citation1980) or a primary magmatic feature (Barron, Citation2000). The aegirine crystals are described as ragged and zoned (Edwards, Citation1980) and as having a reaction intergrowth with an unnamed alkali-amphibole (Barron, Citation2000). Epidote, zeolites, opaque minerals and sulfides were listed as a secondary mineral assemblage (Barron, Citation2000).
Variation in the rock is observed across the outcrop from west to east over ∼20 m. To the east of the main outcrop, the rock matrix darkens, and the foliation intensifies. Edwards (Citation1980) reported compositional variation in the pyroxenes, with higher proportions of Fe and Ca in the west, and higher Al and Na to the east. The increase in foliation was used to infer shearing along the eastern margin of the outcrop () (Edwards, Citation1980), which would be consistent with significant faulting and deformation in the area (Greenfield et al., Citation2010). Alternately, Barron (Citation2000) proposed that the variable alteration intensity reflects variations in the geochemistry of the rock sequence.
Analytical methods
Sample collection
Samples were collected from the Packsaddle and Mount Arrowsmith areas in May 2022 (). Nundorite was collected from the known nundorite outcrop, and along a southeast traverse (∼280 m) following the regional strike. Six nundorite samples were collected and studied in detail, each visually distinct through variations in colour and texture. An additional sample (Nund-1) collected from the nundorite outcrop on a prior field trip was also analysed in this study. Continuation of exposures of nundorite was investigated northwest along-strike, but no outcrops were identified. To characterise the geological origins and setting, three basanite samples and two basalt samples from the MAV were collected. Details of all samples are presented in the supplemental data (Appendix 1 A).
XRD
Identification and modal estimates of the major minerology of the samples were determined by XRD analysis using a Bruker D8 ADVANCE Powder X-ray Diffractometer with a Cu-radiation source, housed in Mawson Analytical Spectrometry Services, The University of Adelaide. The instrument was operated at 40 kV and 40 amps, scanning 2𝜽 from 5 to 65°, with sample rotation set to 30 rotations per minute. Approximately 800 mg of finely powdered sample was loaded into the XRD sample holder with vibration to minimise orientation of grains. Data were processed using Bruker DIFFRAC.EVA software, and reference spectra from the Crystallography Open Database were used to identify mineral phases. Semi-quantitative mineral modes were calculated using TOPAS 4.2 software.
Petrography
Petrographic analysis of nundorite was conducted using a combination of traditional optical petrography on thin-sections, and backscattered electron (BSE) imaging with energy-dispersive spectroscopy (EDS) via a scanning electron microscope (SEM). For SEM analysis, rock chips were set in epoxy mounts, polished and carbon coated. SEM analysis was completed using an FEI Quanta 450 FEG Environmental SEM and Hitachi SU7000 SEM with AZtec software. A 20 kV electron beam was used in both cases. Mineral maps were generated using a Hitachi SU3800 Automated Mineralogy SEM for Mineral Liberation Analysis with AMICS software. Mapping was completed using a 25 kV electron beam and spot size of ∼1 µm. All analytical instruments are housed at Adelaide Microscopy at The University of Adelaide.
Mineral geochemistry
Quantitative elemental data on major, minor and accessory minerals across the nundorite series were acquired using a Cameca SXFive Electron Microprobe at Adelaide Microscopy, The University of Adelaide. Beam conditions were set to 15 kV and 20 nA, and a beam diameter of 5 µm for silicate minerals and 2 µm for Zr and REE minerals. Peak counting times were 15–25 seconds for elements in major silicate minerals (Si, Ti, Al, Fe, Mn, Mg, Ca, Na, K, P) and 30–50 seconds for Zr, Nb, Th, Sr, Y and REE. A set of well-characterised synthetic and natural mineral (oxide, silicate, and phosphate) standards were used for calibration (supplemental data, Appendix 2). Feldspars, pyroxene and nepheline returned good analyses, as revealed by stable beam conditions during analysis, good analytical totals and stoichiometry. By contrast, the quality of analyses for many accessory phases is variable, as the analyses were complicated by multiple factors, such as suspected beam damage to hydrated and carbonated minerals, the complicated elemental composition and the very fine grainsize (2–10 μm) of accessory mineral phases. Therefore, the results for many accessory minerals are semi-quantitative.
Bulk-rock geochemistry
Samples were cut to remove weathered and altered material and analysed for major- and trace-element bulk-rock analysis at Bureau Veritas commercial laboratories in Adelaide. The analytical procedure involved lithium metaborate fusion of weighed sample aliquots at high temperatures in a Pt crucible. The fused glass was digested in nitric acid, therefore providing complete dissolution, albeit with some potential loss of highly volatile elements owing to the high fusion temperatures (Totland et al., Citation1992). The major element suite was determined using inductively coupled plasma (ICP) optical emission spectrometry, whereas the trace elements (REE, Nb, Rb, Sr, Ta, Th, U, Y and Zr) were measured using ICP-MS. Details of the detection limits are provided in the supplemental data (Appendix 1B). To determine the accuracy of the method, a total of five certified reference materials were used, OREAS542 (iron oxide Cu–Au ore), OREAS101a (uranium ore), OREAS91 (siltstone reference material), OREAS120 (uranium ore) and OREAS66a (Au–Ag–Cu ore). All of the analysed values fell within 95% confidence limits, thus demonstrating the accuracy of the method.
Sm–Nd isotope analysis
Samarium–Nd isotope analysis of the rock powders was performed at the University of Adelaide with a Phoenix Isotopx thermal ionisation mass spectrometer, following the routine described in Meeuws et al. (Citation2019). Between 50 and 200 mg of the samples was digested in PARR acid (HNO3–HF acid mix) digestion bombs at 190 °C for 96 hours, then evaporated to dryness and bombed with 6 M HCl overnight. The samples were then spiked with a 150Nd–147Sm spike. The measurements were corrected for mass fractionation by normalisation to 146 Nd/144Nd = 0.7219. The Nd reference material JNdi-1a was processed and yielded an average 143Nd/144Nd value of 0.512123 with a 2σ uncertainty of ± 0.000010 (n = 10; standard reference value = 0.512115 ± 0.000007; Tanaka et al., Citation2000), whereas the USGS rock standard G-2 (n = 3) returned a 143Nd/144Nd value of 0.512226 ± 0.000006 (reference value 0.512233 ± 0.00001; GeoReM preferred value).
Results
Field description
The main outcrop consists of weakly foliated nundorite that has been the source of nundorite specimens circulated for trade. The colour and texture of the rock vary across the outcrop. The rock matrix varies from pale pinky-beige at the northwest end of the outcrop (sample MA0802) to tan (samples MA0801, Nund-1) at the southeast end of the outcrops and to dark chocolate brown (samples MA0804) in subcrop on the north side of the outcrop. Elliptical pale green aegirine grains subtly define a foliation-aligned northwest–southeast with the regional strike. Nundorite varieties with the paler matrix tend to become darker approaching (i.e. within a few centimetres) weathered surfaces and fractures.
Discontinuous subcrop extends ∼280 m along-strike (southeast) and at least to 60 m to the east (). A gneissic foliation develops towards the eastern margin and increases in intensity along-strike to the southeast. Samples collected along this traverse (MA0805, MA0807) have a dark brown matrix with pale banding and dark elongated aegirine grains (up to 15 mm long) (). The southernmost sample (MA0808) has foliation developed to a fine mylonitic texture, with clear S and C fabrics comprising dark brown and pale beige banding wrapping around 1–3 mm aegirine grains ().
Figure 3. Textural and mineralogical changes across nundorite sampling traverse from outcrop to foliated varieties from left to right. (a) Hand-sample photos of nundorite. Note, the prominent green aegirine in the outcrop nundorite samples and increasing degree of foliation across the sample series. (b) MLA mineral maps of nundorite samples (note, the MLA technique could not be used to distinguish natrolite from analcime). The colours refer to minerals shown in (c). (c) Modal mineralogy across the sample series based on MLA mapping result, EDS and WDS analysis, thin-section petrography and XRD analysis.
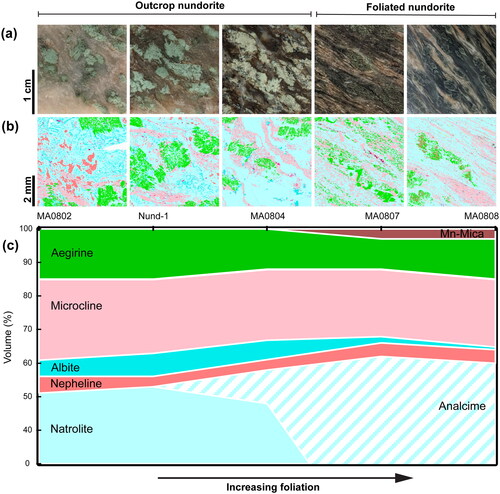
The progressive development of foliation intensity coincides with changes in the mineral assemblage (); therefore, we classify two mineral assemblages for nundorite, herein labelled ‘outcrop’ nundorite from the main outcrop, and ‘foliated’ nundorite from the surrounding subcrop.
Petrography
Outcrop nundorite: major and minor mineral assemblage
The outcrop nundorite appears as a granular porphyroblastic rock consisting of light green aegirine (∼8 mm) within a finer pinky-tan to light brown matrix. Aegirine (∼15 modal %) forms rounded to elliptical grains that appear to be subhedral in hand sample. However, optical petrography reveals these grains to be fractured and fragmented with ragged grain boundaries, although they remain optically continuous and display various degrees of zoning (supplemental data, Appendix 3). Inclusions are consistently oriented parallel within the aegirine (), but at an angle to the fabric of the rock. Inclusions consist of albite, nepheline, natrolite, microcline and zirconium alkali silicate and REE mineral phases, as described below. Sample MA0804 has larger aegirine grains (10–12 mm) that are visibly fragmented and elongated, even in hand sample, giving them a cloud like appearance.
Figure 4. Backscattered electron images of outcrop nundorite samples. (a) Major and minor minerals in sample MA0802. (b) Monazite in interstices of aegirine, sample MA0804. (c) Euhedral schizolite and skeletal albite intergrown with matrix natrolite of sample MA0802. (d) Accessory REE and Zr phases in sample Nund-1. (e) Zr alkali silicate intergrown with aegirine in sample Nund-1. (f) Complexly zoned Zr alkali silicate associated with microcline and cerite-group minerals in sample MA0802. Mineral abbreviations: Aeg, aegirine; Crt, cerite-group mineral; Mnz, monazite; Nb, unidentified Nb-rich mineral; Ntr, natrolite; Mic, microcline; ZAS, zirconium alkali silicate.
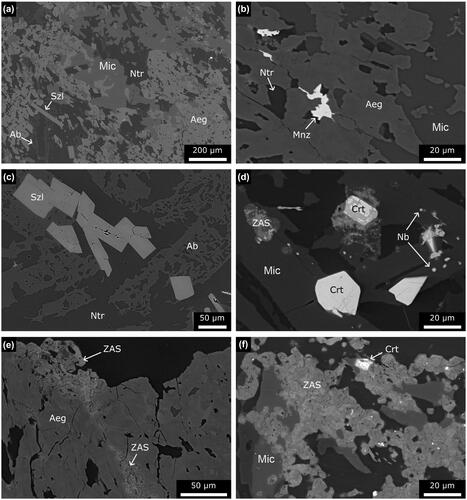
Combined XRD and petrographic methods established the bulk of the rock matrix (∼50% of the rock) to be the zeolite mineral natrolite [Na2Al2Si3O10·2(H2O)]. Natrolite has a variable crystal habit, ranging from radial aggregates up to 300 µm across, to subhedral grains ranging from 10 to 100 µm. The larger grains concentrate in ‘pressure shadow’ zones around aegirine. Natrolite is also present as inclusions and fracture fillings in aegirine and nepheline. Analcime was also detected during EDS and XRD analysis but is only present as a minor to accessory phase. Microcline defines the foliation and is also present as inclusions in aegirine () and overgrowing nepheline. In the lightest variants of nundorite on the western side of the outcrop (e.g. sample MA0802), the microcline (∼25 modal %; ; Appendix 3) is subhedral, prismatic to tabular and 50–100 µm long, whereas in tan (Nund-1, MA0801) and dark (MA0804) coloured nundorite varieties further to the east, the microcline (20 modal %) is more euhedral and up to 400 µm in size.
Nepheline and albite are both minor phases in the outcrop nundorite (). Nepheline forms 0.1–1 mm anhedral blobs in the pinkish and tan samples, commonly with small inclusions of natrolite and in places aegirine (). Patches of fine (tens of micrometres) nepheline flecks are present in the tan samples, whereas the darkest outcrop sample has scattered flecks of nepheline with microcline in foliation bands. Nepheline is aligned with foliation and commonly surrounded by a corona of natrolite that separates nepheline from albite (Appendix 3). Albite is unfoliated and forms radiating skeletal laths up to 500 µm long in the lightest samples () and relatively pristine subhedral tabular clusters in the darker sample MA0804.
Outcrop nundorite: accessory mineral assemblage
The outcrop nundorite contains a diversity of accessory minerals. Schizolite (NaCaMnSi3O8—a wollastonite group mineral; Lussier et al., Citation2021) is found as euhedral 100–200 µm, tabular to prismatic grains () that can occur as individual grains, as grain clusters in the matrix or with microcline in foliation bands. The dark nundorite sample MA0804 contains notably less and fine-grained (∼50 µm) schizolite than other samples.
Zirconium in the light and tan samples is largely hosted in Zr–Na silicate and Zr–Ca silicate phases (; Appendix 2), which are tentatively identified as either elpidite, catapleiite, lemoynite or armstrongite. These form blebby anhedral clusters (up to 100 µm) within the aegirine and within the bands of microcline, following foliation ().
Table 1. Representative major element oxide (wt%) composition of rare metal-bearing accessory phases found in nundorite.
A range of REE- and Nb-rich phases were found within the samples (; Appendix 2). The predominate REE mineral is a solid solution between cerite-group minerals (REE-rich hydrated silicate minerals; Atencio & de Almeida Azzi, Citation2020). The cerite-group mineral (herein, cerite) occurs as small (10–50 µm) euhedral grains () or as overgrows on, and fractures in, aegirine. Other REE- and Nb-rich minerals include galgenbergite–(Ce) [Ca(REE)2(CO3)4·(H2O)], fersmite [(Ca,Ce,Na)(Nb,Ta,Ti)2(O,OH,F)6], natroniobite (NaNbO3), an unidentified REE-rich fluorcarbonate and an unidentified Nb-silicate. These minerals are generally 2–10 µm and occur peppered throughout the matrix, associated with the Zr alkali silicate clusters, clustered between microcline grains or associated with aegirine (either replacing or along fractures). Monazite was found only in sample (MA0804) as 25–50 µm euhedral grains and in thin veins within and overgrowing aegirine ().
In places, euhedral sphalerite and galena are also present. Traces of quartz are present in MA0804, in veins cross-cutting foliation (micrometre and millimetre scale) and in small patches associated with an unidentified Mn-rich mineral.
Foliated nundorite: major, minor and accessory mineral assemblage
A major difference between the foliated nundorite found to the east and southeast of the outcrop nundorite is the higher degree of foliation and rock matrix mineralogy dominated by analcime rather than natrolite. The analcime forms gneissic banding along with microcline (), which has a large size range (50–800 µm tabular crystals). Small (20 µm) fragmented grains of nepheline are also concentrated in the foliated bands. There is little albite in these samples, but small fragments are peppered throughout the fabric (Appendix 3). In the southernmost sample (MA0808), the fabric develops into a mylonitic texture with S and C fabrics, and microcline is concentrated in sigmoidal shear bands.
Aegirine (∼10 modal %) is dark green in the foliated nundorite. In samples closer to the outcrop (MA0805, MA0807) the grains are highly fragmented and smeared along the fabric. This gives the appearance of larger, elongated grains in hand sample (up to 15 mm). The aegirine fragments are varied in size (<10–500 µm), and few have inclusions but feature some complex zoning to aegirine-augite and are partially replaced by zircon, cerite and hematite (). The habit of aegirine is different in the most deformed sample (MA0808), being rounded and smaller (∼2 mm) and commonly with a poikilitic texture with equant inclusions of microcline, nepheline and analcime ().
Figure 5. Backscattered electron images of mineral textures in foliated nundorite. (a) Poikiolitic aegirine with nepheline inclusions in sample MA0808. (b) Intergrown aegirine and aegirine-augite surrounded, and partly replaced by, hematite, sphalerite and clustered cerite-group minerals; sample MA0807. (c) Fine zircon and mica grains rimming microcline in sample MA0807. (d) Schizolite associated with zircon and cerite in sample MA0807. Mineral abbreviations: Aeg, aegirine; Aeg-Aug, aegirine-augite; Crt, cerite; Hem, hematite; Or, microcline; Sp, sphalerite; Szl, schizolite; Zrn, zircon.
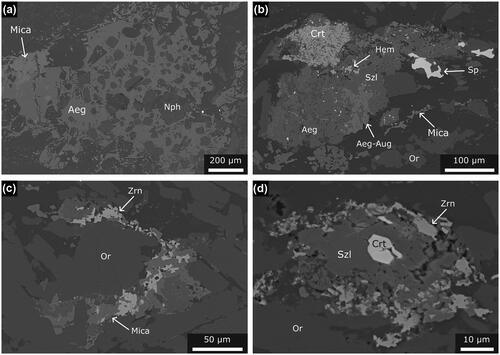
Compared with outcrop nundorite, there is less schizolite in MA0807 and MA0808. Grains are subhedral and intergrown with microcline, aegirine and clusters of cerite (). A K–Fe–Mn silicate is tentatively identified as a Mn-rich mica. However, electron microprobe data returned inconsistent data with variable totals, making identification challenging. This mineral forms thin bands along foliation, commonly surrounding and flecked through fragmented aegirine. In places, the ‘mica’ is overgrowing aegirine and is associated with the breakdown of schizolite.
The main Zr-phase in these samples is zircon (), although there is also a Na–Ca–Zr silicate phase, possibly catapleiite, present. Clusters of anhedral to subhedral zircon are foliated within the microcline banding (). Zircon also overgrows aegirine and schizolite.
Like the outcrop nundorite, the foliated nundorite had a range of REE and Nb-rich minerals (). These phases formed in clusters along foliation and tend to be larger in size (up to 100 µm) than in the outcrop nundorite. REEs are mostly hosted in silicate phases, which are generally Ca-rich and include cerite-group minerals and epidote–allanite. These congregate around aegirine, schizolite and the Mn-mica (). An unidentified REE-fluorcarbonate is also present. Niobium is hosted in fergusonite-(Ce) [(Ce,La,Y)NbO4], as well as an unidentified Nb-silicate.
Mineral geochemistry
Quantitative mineral chemistry determined by electron microprobe was used to examine mineral composition across the nundorite sample set. The matrix minerals have relatively stable compositions across nundorite samples. Albite is near endmember (Ab97–100). The composition of microcline is also consistently close to the pure endmember (Or96–99) across all samples. Nepheline is near ideal composition (Na0.75 K0.25AlSiO4) ().
Table 2. Representative major silicate mineral compositions (wt%) of nundorite samples.
Aegirine has compositional variations both within grains and throughout the series. This appears on mineral maps as patchy zoning. Although previously reported as aegirine-augite (Gilmore et al., Citation2009), there is very little Mn, Mg or Ca, especially from the outcrop samples, and compositional variation is mostly Al substitution for Fe (). Pyroxenes tend to separate into two compositional clusters; low Al aegirine (Aeg83–95) and aluminous aegirine (Aeg63–80) (). The outcrop nundorite contains both compositions but is predominantly the aluminous aegirine. Aegirine analyses in the foliated nundorite were generally more Fe- and Ca-rich, with fewer aluminous analyses (; ). Mineral maps do indicate this composition may still be present in small fragments, but these are more difficult to analyse.
Figure 6. Changes in endmember proportions of aegirine (in clinopyroxene) and pectolite (in schizolite) across the nundorite alteration series.
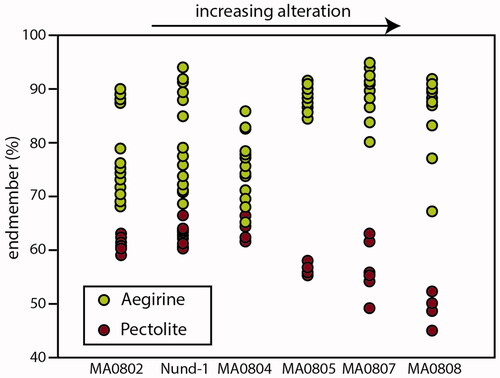
The composition of schizolite changes throughout the series (). Schizolite is the intermediate member of the serandite [NaMn2Si3O8(OH)] to pectolite [NaCa2Si3O8(OH)] series of the wollastonite group. In the outcrop nundorite, the composition trends toward the pectolite endmember, whereas the schizolite from the foliated nundorite is closer to ideal schizolite, although there is also a larger compositional range in the most foliated sample ().
Bulk-rock geochemistry
The bulk-rock geochemistry was determined for seven samples across the sampling traverse. This series represents the full range of nundorite types encountered in the field ().
Table 3. Major and trace-element geochemistry and Nd isotope composition of samples of nundorite and associated mafic volcanic rocks.
All nundorite samples analysed here have similar bulk-rock geochemical compositions and are similar to the chemical compositions documented in previous studies (Edwards, Citation1980; Greenfield et al., Citation2011). Nundorite is unusually enriched in alkalis (9.44–11.7 wt% Na2O, 3.78–4.69 wt% K2O) and Al2O3 (20.2–22.9 wt%) with relatively low SiO2 contents (53.1–55.4 wt%). All samples plot as phonolites on a TAS diagram () and have appreciable Fe2O3 contents (3.62–3.93 wt%), but low contents of CaO (0.17–0.71 wt%) and MgO (0.02–0.21 wt%). Titanium and P contents are anomalously low, as depicted by their very prominent negative anomalies on multi-element plots (). The peralkaline index [molar (Na + K)/Al] ranges from 1.00 to 1.13, establishing them as peralkaline rocks.
Figure 7. Total-alkali vs silica diagram (from Le Maitre et al., Citation2002) of nundorite and basanite samples, and MAV rocks from Greenfield et al. (Citation2011) and references therein. The data have been filtered to exclude analyses with >8 wt% LOI, and anomalously low Na2O (<1 wt%) and K2O (<0.10 wt%), indicative of modification via alteration. Diamond symbols are data from the literature, and circle symbol are data from this study.

Figure 8. NMORB-normalised multi-trace element plots of nundorite (n = 7) compared with; (a) syenites (n = 2) and basanites (n = 3) of the Mount Arrowsmith Volcanics, and (b) evolved, high alumina phonolites from the Apiau Alkaline Complex (AAC), Guyana Shield (n = 13; Figueiredo et al., Citation2022) and the Dunedin volcano (n = 11; Pontesilli et al., Citation2022; Price et al., Citation2003). Samples normalised to NMORB values of Sun and McDonough (Citation1989).
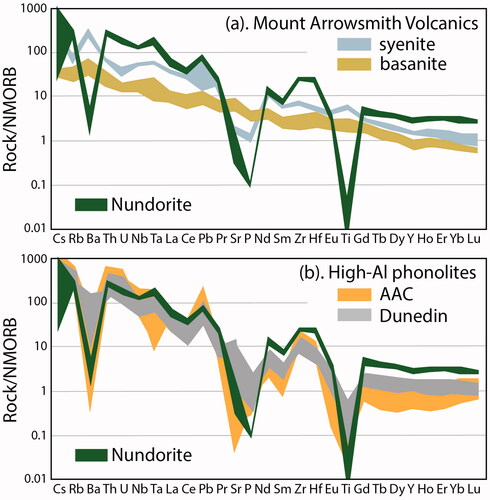
Multi-element plots normalised to NMORB show the nundorite to have enriched incompatible element (positive slope on diagram) compositions somewhat similar, albeit to much higher concentrations, to alkali-rich mafic volcanics of the MAV. Notable exceptions are the pronounced negative anomalies for Ba and Sr (in addition to Ti and P), as well as a positive Ta anomaly (i.e. Nb/Ta ratio lower than NMORB). Concentrations of rare metals such as Zr (1720–1880 ppm) and Nb (245–320 ppm) are notably elevated. Nundorite has LREE-enriched REE patterns with a modest negative Eu anomaly.
Loss on ignition (LOI) ranges from 4.28 to 5.63 wt%, and generally increases along the sampling traverse to the southeast. Otherwise, there is no systematic chemical variation across the sampling traverse, with the possible exceptions of Rb that decreases slightly, and Sr that increases, with alteration/foliation intensity. These elements are known to be fluid mobile, and therefore their concentrations may have been modified via hydrothermal overprinting of nundorite towards the southwest of the sampling traverse.
Sm–Nd isotopes
Two samples of outcrop nundorite (Nund-1 and MA0802) and one sample of the foliated nundorite (MA0808) analysed for Sm–Nd isotopes returned similar compositions, with 147Sm/144Nd of 0.11176–0.11011, and 143Nd/144Nd of 0.5121689–0.5121639 (). Initial εNd values corrected to an age of 585 Ma are +5.46 to +5.56. For comparative purposes, we also analysed the isotopic composition of two trachybasalt samples collected from outcrops ∼1.5 km southeast along-strike from the nundorite locality. These mafic rocks returned initial εNd values (T = 585 Ma) of +5.24 and +5.28 (), which are similar to the nundorite samples and other mafic volcanic rocks of the MAV (mostly +4 to +6; ; Zhou & Whitford, Citation1994). Single-stage depleted mantle model ages for both the nundorite and mafic volcanic samples are between 900 and 820 Ma.
Figure 9. εNd vs bulk rock Zr concentration on nundorite and rocks of the Mount Arrowsmith Volcanics (MAV). Note the similar εNd of nundorite and the MAV rocks. Circles are data from this study, and diamonds are data from Crawford et al. (Citation1997), Zhou and Whitford (Citation1994), and Greenfield et al. (Citation2011). Symbol size is larger than measurement uncertainties.
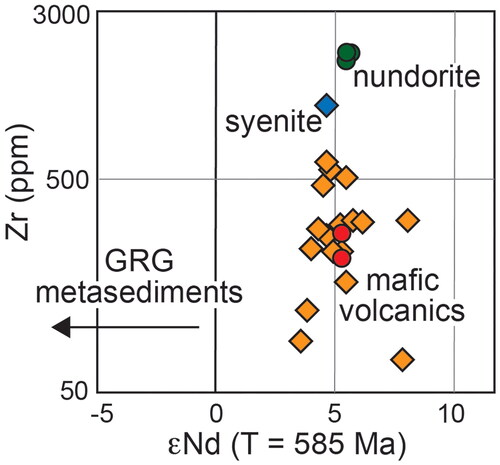
Discussion
Nundorite as a geological oddity
Despite a known provenance of only a single small outcrop, nundorite has seen wide circulation across Australia as specimens of ornamental stone owing to its distinctive appearance of green coarse aegirine crystals set within a fine matrix that can vary in colour from pink to tan to brown. Beyond the visual appeal, nundorite has a highly unusual bulk chemical composition and unique mineralogy, and so certainly qualifies as a geological oddity. Rocks of high-alumina peralkaline composition are extremely rare in the rock record (or at least are very rarely reported), as are rocks with Zr contents exceeding 1500 ppm; nundorite has all of these features. The unusual composition manifests in a diverse assemblage of rare accessory minerals including schizolite and Mn-rich mica, a range of Na–Zr silicate phases, cerite, REE (fluor)carbonates, fergusonite, fersmite and other Nb minerals.
The bulk-rock composition is remarkably uniform across the sampling traverse, despite the changes in rock colour and texture, and mineralogy (). The increasing intensity of foliation along the traverse is an indication that the variation is due to increasing degrees of deformation and hydrothermal alteration approaching a possible fault zone to the southeast. The isochemical nature of this alteration allows us to examine relative mineral stability relations.
The mineral assemblage of nundorite is dominated by the zeolite minerals natrolite and analcime, which are known to form under relatively low temperature (<300 °C) metamorphic conditions (Chipera & Apps, Citation2001). This is consistent with the expected Delamerian regional metamorphism of prehnite–pumpellyite to lower greenschist-facies (Greenfield et al., Citation2010). Mineralogical changes across the traverse are marked by: (1) a transition from natrolite to analcime, (2) a decrease in modal pyroxene and a shift in composition from jadeite towards endmember aegirine, (3) decreasing abundance of nepheline and albite, (4) increasing abundance of microcline, (5) a transition from Ca-rich schizolite to Ca-poor schizolite plus Mn-rich mica, (6) a transition from Na–Zr silicates to zircon and Ca–Zr silicates, and (7) a transition from REE silicates to Ca-bearing REE silicates.
The major mineralogical change of natrolite to analcime can be explained by an increase in the silica activity (Chipera & Apps, Citation2001). A generalised reaction for the alkali aluminosilicate phase changes observed across the sampling traverse can be represented as:
(1)
(1)
The decreasing mode of pyroxene is largely accounted for by a loss of the jadeite component, which can simply be related to analcime growth via:
(2)
(2)
The breakdown of schizolite to Mn-rich mica is proposed to provide the additional silica to drive the natrolite to analcime reaction, and to account for the presence of zircon and trace quartz in the foliated samples, rather than Zr–Na silicate. The transition from relatively Ca-rich to Ca-poor schizolite () also explains the higher Ca contents of aegirine-rich pyroxenes and Ca-rich REE and Zr minerals in the foliated samples. The only additional component required for these mineral reactions is introduction of H2O via a hydrothermal fluid influx, which is consistent with the increasing LOI content across the sampling traverse.
Origin of nundorite
The enigmatic nature of nundorite is reflected in the diverse range of primary origins proposed, from intrusive (Edwards & Neef, Citation1979) or pyroclastic rock (Buckley & Mills, Citation2006; Gilmore et al., Citation2009), to being the product of hydrothermal alteration on the seafloor (Gilmore et al., Citation2009) or via fenitisation (Edwards, Citation1980). Although the low-grade metamorphic overprint complicates interpretation of the protolith of nundorite, the bulk geochemical and Nd isotope compositions, together with the geological setting of the surrounding units, are instructive for protolith evaluation. In particular, the initial Nd isotopic composition of nundorite (εNd ∼+5.5) is similar to mafic volcanic rocks of the MAV () and is consistent with a mantle source. Metasedimentary rocks of the Grey Range Group contain significant Mesoproterozoic components (Johnson et al., Citation2016), and therefore would have significantly more evolved (non-radiogenic) Nd isotope compositions. Therefore, a sedimentary or anatectic origin for nundorite is not supported by the isotopic data and is inconsistent with the peralkaline composition and highly elevated content of rare metals such as Zr, Hf, Nb and Ta. A hydrothermal alteration origin is a feasible explanation for the enrichment of alkali elements, as these are known to be fluid-mobile, but it does not conform with high Al2O3 and the very high contents of Zr, Hf, Nb and Ta. These elements are recognised to be among the most fluid immobile (e.g. Humphris & Thompson, Citation1978), only being concentrated by metasomatic processes in rare and unusual cases (Jiang et al., Citation2005). Moreover, intense hydrothermal alteration tends to result in spatially resolvable geochemical variability (e.g. zonation around faults or shear zones), whereas the nundorite is remarkably uniform in chemical composition across the study area.
Ruling out sedimentary or hydrothermal origins, we conclude that nundorite is of an igneous protolith. In this case, nundorite can be assigned to the MAV, as previously asserted (e.g. Greenfield et al., Citation2010). The metamorphic overprint complicates determination of plutonic vs volcanic origins, although we note that zeolites, such as those that dominate nundorite, are common products of alteration of glass in volcanic rocks such as phonolites (Chipera & Apps, Citation2001). Indeed, the aegirine–zeolite dominant mineralogy of nundorite has also been reported for many phonolites (e.g. Goble et al., Citation1993; Weisenberger et al., Citation2014). Nundorite and MAV mafic rocks share similar Nd isotopic compositions (), but there are no known volcanic rocks of the MAV with a geochemical composition comparable with nundorite. However, Crawford et al. (Citation1997) documented alkaline rhyolites of the MAV, with high Zr (∼1600 ppm) and Nb (∼130 ppm) that are approaching the values of nundorite. More broadly, we are unaware of any phonolitic rocks in Australia with compositions comparable with that of nundorite (e.g. Ewart et al., Citation1985). Nevertheless, rare occurrences of evolved phonolites with compositions very similar to that of nundorite have been described from the Dunedin volcano, New Zealand (Pontesilli et al., Citation2022; Price et al., Citation2003), and from continental rift volcanic systems of Mt Kenya (Price et al., Citation1985), Mt Kilimanjaro (Nonnotte et al., Citation2011), Cameroon (Pouclet et al., Citation2014), central Namibia (Marsh, Citation2010) and the Guyana Shield (Figueiredo et al., Citation2022). Specifically, all of these rocks have high alkalis (Na2O + K2O >13%), anomalously high alumina (∼19–23 wt% Al2O3), very low TiO2 and P2O5, and distinctive, but comparable, trace-element compositions (), including high contents of Zr (700 to ∼2000 ppm), Nb (100 to ∼350 ppm) and many other incompatible elements.
With the assertion that nundorite is of high-alumina phonolitic origin, we examine the possible petrogenic processes that may have generated this unusual composition. The comparable mantle-like Nd isotopic signatures of both nundorite and local mafic volcanic rocks preclude any significant role of assimilation of old crustal material during magma genesis. High-alumina phonolite melts could be produced via low-degree melting of mantle lherzolite at P < 1.5 GPa (Laporte et al., Citation2014). However, this origin does not conform with the very low in MgO and CaO contents, and degree of enrichment in incompatible trace-element composition of nundorite. Moreover, the widespread presence of (likely coeval) MAV primitive mafic volcanics indicates that degrees of mantle melting were likely to be significantly higher than that required for primary phonolite generation.
Crawford et al. (Citation1997) concluded that the spectrum of volcanic rock compositions preserved in the MAV were largely the result of magmatic differentiation via crystal fractionation; herein, we propose that nundorite is also a product of (hyper)extended crystal fractionation of a mafic alkali parental melt. This model is supported by isotopic data, and comparison with other rare metal-rich peralkaline complexes that form via extensive fractionation of mafic parental melts (Chandler & Spandler, Citation2020; Figueiredo et al., Citation2022; Pontesilli et al., Citation2022; Spandler & Morris, Citation2016). Notably, the prominent negative anomalies for Ba, Ti, P and Sr can be related to extensive fractionation of K-feldspar (Ba), Ti oxides (Ti) and apatite (Sr, P), as also observed in other highly fractionated phonolites (e.g. Figueiredo et al., Citation2022; Pontesilli et al., Citation2022; ). Importantly, rare metals (Zr, Hf, Nb and Ta) remain highly incompatible, owing to their high solubility in peralkaline melts (Markl et al., Citation2010; Spandler & Morris, Citation2016) and therefore can become highly enriched in the residual melt fraction. The moderate negative Eu anomaly indicates either a limited role of plagioclase fractionation or that the magmas were relatively oxidised such that Eu behaved in a similar manner to other REE (i.e. all trivalent). Detailed fractional crystallisation modelling is beyond the scope of this paper; however, simple mineral removal and melt evolution models are unlikely to be able to reproduce the unusual composition of nundorite (e.g. see Spandler & Morris, Citation2016). Instead, more complex magma evolutional pathways, such as the replenish–mix–tap–crystallise model of O’Neill and Jenner (Citation2016) may be important to consider, especially to account for the high degree of enrichment in incompatible elements.
To summarise, we propose that nundorite is the low-grade metamorphic equivalent of a high-alumina phonolite produced by extensive fractionation of an alkali-rich mafic parent melt generated in a developing continental rift zone. Depleted mantle model ages of 890–830 Ma may correspond to an episode of mantle enrichment related to an impinging mantle plume that produced the widespread Gairdner Large Igneous Province (Huang et al., Citation2015). This plume activity, or intra-continental rifting associated with the Adelaide Superbasin (900–820 Ma; Lloyd et al., Citation2022) may also have imparted structural and compositional features on the lithosphere that influenced the location of subsequent continental rifting and formation of the Koonenberry Belt.
Significance for critical mineral exploration
The widespread mafic/ultramafic igneous intrusions of the MAV are currently a major exploration target for orthomagmatic Ni (±Cu, PGE) mineralisation (Gilmore et al., Citation2007). By contrast, there has been little to no attention on the potential for rare metal mineralisation in the MAV, despite alkaline intrusive and volcanic systems being important sources of rare metals both in Australia (Spandler et al., Citation2020) and overseas (Chakhmouradian & Zaitsev, Citation2012). Australian deposits that could be considered the closest analogues of nundorite are the Toongi deposit in NSW (Spandler & Morris, Citation2016) and some rhyolite bodies of the Peak Ranges Volcanics of Central Queensland (Chandler & Spandler, Citation2020). In both cases, rare metal mineralisation is hosted in peralkaline volcanic rocks, and mineralisation was primarily driven by extreme crystal fractionation in the absence of crustal contamination, processes that are also implicit in nundorite formation. Ore mineral assemblages are also broadly similar between the three rock units, with Zr hosted in alkali Zr silicates, Nb in Nb-oxide phases, and REE in diverse carbonate and silicate phases (Chandler & Spandler, Citation2020; Spandler & Morris, Citation2016). While nundorite itself is not enriched in rare metals to ore grades (compare ∼15 000 ppm Zr and ∼3300 ppm Nb of Toongi, with ∼1800 ppm Zr and ∼290 ppm Nb for nundorite), its evolution and preservation in the MAV highlight that the essential magma evolution processes for ore formation were in operation during development of the MAV. Given the limited previous detailed geological work on the MAV and lack of attention for critical mineral prospectively, this area may well be fertile hunting ground for discovery of rare metal mineral deposits.
Conclusions
Despite its unique occurrence, nundorite can be assigned an origin as a high-Al phonolite based on geochemical and Nd isotope composition, and its association with the continental rift-related MAV. The unusual assemblage of accessory minerals rich in Mn, REE, Zr and Nb is attributed to high concentrations of these elements in the rock, and to recrystallisation during low-grade metamorphism and variable levels of hydrothermal alteration and deformation. The high levels of Al2O3 and incompatible trace elements, as well as very low P2O5 and TiO2, are considered a primary magmatic feature related to extreme levels of crystal fractionation, which is consistent with the proposed origin of similar rock types found in other continent rift and intraplate volcanic settings. Although a geological oddity, the occurrence of nundorite demonstrates that magmatic processes crucial for rare metal mineralisation—namely melting of an enriched mantle source during continental rifting, followed by magma evolution via extended crystal fractionation—were in operation during the formation of the MAV, so this belt should be considered prospective for critical mineral ore deposits.
Supplemental Material
Download PDF (7.6 MB)Appendix 1
Download MS Excel (17.6 KB)Appendix 2
Download MS Excel (96.9 KB)Acknowledgements
The authors thank two reviewers for their constructive comments that greatly improved the manuscript, and Chris Yeats for editorial handling. We thank Phil Gilmore for providing some initial information on nundorite, Yanbo Cheng for assistance with the fieldwork and Ben Wade for assistance with mineral chemistry analysis.
Disclosure statement
No potential conflict of interest was reported by the author(s).
Data availability statement
The authors confirm that the data supporting the findings of this study are available within the article and its supplementary materials.
Additional information
Funding
References
- Atencio, D., & de Almeida Azzi, A. (2020). Cerite: A new supergroup of minerals and cerite–(La) renamed ferricerite–(La). Mineralogical Magazine, 84(6), 928–931. https://doi.org/10.1180/mgm.2020.86
- Barron, L. M. (2000). Some alkaline rocks and sediments, far west New South Wales [unpublished report]. Geological Survey of New South Wales.
- Buckley, P. M., & Mills, K. J. (2006). Excursion guide for the Koonenberry Belt Field Trip (BHEI2006). Broken Hill Exploration Initiative.
- Chakhmouradian, A. R., & Zaitsev, A. N. (2012). Rare earth mineralization in igneous rocks: Sources and processes. Elements, 8(5), 347–353. https://doi.org/10.2113/gselements.8.5.347
- Chandler, R., & Spandler, C. (2020). The igneous petrogenesis and rare metal potential of the peralkaline volcanic complex of the southern Peak Range, Central Queensland, Australia. Lithos, 358–359, 105386. https://doi.org/10.1016/j.lithos.2020.105386
- Chipera, S. J., & Apps, J. A. (2001). Geochemical stability of natural zeolite. Reviews in Mineralogy and Geochemistry, 45(1), 117–161. https://doi.org/10.2138/rmg.2001.45.3
- Crawford, A. J., Stevens, B. P. J., & Fanning, M. (1997). Geochemistry and tectonic setting of some Neoproterozoic and Early Cambrian volcanics in western New South Wales. Australian Journal of Earth Sciences, 44(6), 831–852. https://doi.org/10.1080/08120099708728358
- Edwards, A. C., & Neef, G. (1979). The Geology of Mt. Arrowsmith, Wonominta Block, Koonenberry Gap, Kayrunnera and Wertago (GS 1981/183). Geological Survey of New South Wales.
- Edwards, A. C. (1980). The geology and petrology of the igneous rocks of Mt. Arrowsmith and related areas of north western New South Wales [unpublished PhD thesis]. The University of New South Wales.
- Ewart, A., Chappell, B. W., & Le Maitre, R. W. (1985). Aspects of the mineralogy and chemistry of the intermediate‐silicic Cainozoic volcanic rocks of eastern Australia. Part 1: Introduction and geochemistry. Australian Journal of Earth Sciences, 32(4), 359–382. https://doi.org/10.1080/08120098508729339
- Figueiredo, R. F., Azzone, R. G., & dos Santos, T. J. S. (2022). The Roraima Alkaline Province: A Cretaceous alkaline province in the Amazonian Craton. Geochemistry, 82(3), 125900. https://doi.org/10.1016/j.chemer.2022.125900
- Gard, M., Hasterok, D., & Halpin, J. A. (2019). Global whole-rock geochemical database compilation. Earth System Science Data, 11(4), 1553–1566. https://doi.org/10.5194/essd-11-1553-2019
- Gilmore, P. J., Greenfield, J. E., & Mills, K. J. (2009). Field guide for the Koonenberry Belt geological history and mineral systems excursion, October 2009 (GS 2009/0694). Broken Hill Exploration Initiative.
- Gilmore, P., Greenfield, J., Reid, W., & Mills, K. (2007). Metallogenesis of the Koonenberry Belt. Bulletin of the Australian Institute of Geoscientists, 46, 49–64.
- Goble, R. J., Treves, S. B., & Ghazi, A. M. (1993). Comparison of the Rainy Ridge analcime phonolite sill and the Crowsnest Volcanics, Alberta, Canada. Canadian Journal of Earth Sciences, 30(8), 1644–1649. https://doi.org/10.1139/e93-142
- Greenfield, J. E., Gilmore, P. J., & Mills, K. J. (2010). Explanatory notes for the Koonenberry Belt geological maps (Bulletin 35). Geological Survey of New South Wales.
- Greenfield, J. E., Musgrave, R. J., Bruce, M. C., Gilmore, P. J., & Mills, K. J. (2011). The Mount Wright Arc: A Cambrian subduction system developed on the continental margin of East Gondwana, Koonenberry Belt, Eastern Australia. Gondwana Research, 19(3), 650–669. https://doi.org/10.1016/j.gr.2010.11.017
- Greenfield, J. E., & Reid, W. J. (2010). Mount Arrowsmith 1:100 000 Geological Sheet 7237 (1st ed.). Geological Survey of New South Wales.
- Harlow, G. E., Tsujimori, T., & Sorensen, S. S. (2015). Jadeitites and plate tectonics. Annual Review of Earth and Planetary Sciences, 43(1), 105–138. https://doi.org/10.1146/annurev-earth-060614-105215
- Huang, Q., Kamenetsky, V. S., McPhie, J., Ehrig, K., Meffre, S., Maas, R., Thompson, J., Kamenetsky, M., Chambefort, I., Apukhtina, O. B., & Yongbin, H. (2015). Neoproterozoic (ca. 820–830 Ma) mafic dykes at Olympic Dam, South Australia: Links with the Gairdner large igneous province. Precambrian Research, 271, 160–172. https://doi.org/10.1016/j.precamres.2015.10.001
- Humphris, S. E., & Thompson, G. (1978). Hydrothermal alteration of oceanic basalts by seawater. Geochimica et Cosmochimica Acta, 42(1), 107–125. https://doi.org/10.1016/0016-7037(78)90221-1
- Jiang, S. Y., Wang, R. C., Xu, X. S., & Zhao, K. D. (2005). Mobility of high field strength elements (HFSE) in magmatic-, metamorphic-, and submarine-hydrothermal systems. Physics and Chemistry of the Earth, 30(17–18), 1020–1029. https://doi.org/10.1016/j.pce.2004.11.004
- Johnson, E. L., Phillips, G., & Allen, C. M. (2016). Ediacaran–Cambrian basin evolution in the Koonenberry Belt (eastern Australia): Implications for the geodynamics of the Delamerian Orogen. Gondwana Research, 37, 266–284. https://doi.org/10.1016/j.gr.2016.04.010
- Laporte, D., Lambart, S., Schiano, P., & Ottolini, L. (2014). Experimental derivation of nepheline syenite and phonolite liquids by partial melting of upper mantle peridotites. Earth and Planetary Science Letters, 404, 319–331. https://doi.org/10.1016/j.epsl.2014.08.002
- Le Maitre, R. W., Streckeisen, A., Zanettin, B., Le Bas, M. J., Bonin, B., & Bateman, P. eds. (2002). Igneous rocks. A classification and glossary of terms: Recommendations of the International Union of Geological Sciences Subcommission on the Systematics of Igneous Rocks (2nd edi.). Cambridge University Press. https://doi.org/10.1017/CBO9780511535581
- Lloyd, J. C., Collins, A. S., Blades, M. L., Gilbert, S. E., & Amos, K. J. (2022). Early evolution of the Adelaide Superbasin. Geosciences, 12(4), 154. https://doi.org/10.3390/geosciences12040154
- Lussier, A. J., Grice, J. D., Friis, H., & Poirier, G. G. (2021). Insights into the crystal chemistry of the serandite–schizolite–pectolite series. The Canadian Mineralogist, 59(3), 551–572. https://doi.org/10.3749/canmin.1900097
- Markl, G., Marks, M. A., & Frost, B. R. (2010). On the controls of oxygen fugacity in the generation and crystallization of peralkaline melts. Journal of Petrology, 51(9), 1831–1847. https://doi.org/10.1093/petrology/egq040
- Marsh, J. S. (2010). The geochemistry and evolution of Palaeogene phonolites, central Namibia. Lithos, 117(1–4), 149–160. https://doi.org/10.1016/j.lithos.2010.02.012
- Meeuws, F. J. E., Foden, J. D., Holford, S. P., & Forster, M. A. (2019). Geochemical constraints on Cenozoic intraplate magmatism and their relation to Jurassic dolerites in Tasmania, using Sr–Nd–Pb isotopes. Chemical Geology, 506, 225–273. https://doi.org/10.1016/j.chemgeo.2018.12.025
- Mills, K. G., Hicks, M. G., & Cooper, I. B. (2015). Wonnaminta 1:100 000 Geological Sheet 7336 (2nd ed.) Geological Survey of NSW.
- Nonnotte, P., Benoit, M., Le Gall, B., Hémond, C., Rolet, J., Cotten, J., Brunet, P., & Makoba, E. (2011). Petrology and geochemistry of alkaline lava series, Kilimanjaro, Tanzania: New constraints on petrogenetic processes. Geological Society of America Special Paper, 478, 127–158. https://doi.org/10.1130/2011.2478(07)
- O’Neill, H. S. C., & Jenner, F. E. (2016). Causes of the compositional variability among ocean floor basalts. Journal of Petrology, 57(11–12), 2163–2194. https://doi.org/10.1093/petrology/egx001
- Pontesilli, A., Brenna, M., Mollo, S., Masotta, M., Nazzari, M., Le Roux, P., & Scarlato, P. (2022). Trachyte–phonolite transition at Dunedin Volcano: Fingerprints of magma plumbing system maturity and mush evolution. Lithos, 408–409, 106545. https://doi.org/10.1016/j.lithos.2021.106545
- Pouclet, A., Kagou Dongmo, A., Bardintzeff, J-M., Wandji, P., Chakam Tagheu, P., Nkouathio, D., Bellon, H., & Ruffet, G. (2014). The Mount Manengouba, a complex volcano of the Cameroon Line: Volcanic history, petrological and geochemical features. Journal of African Earth Sciences, 97, 297–321. https://doi.org/10.1016/j.jafrearsci.2014.04.023
- Price, R. C., Cooper, A. F., Woodhead, J. D., & Cartwright, I. A. N. (2003). Phonolitic diatremes within the Dunedin Volcano, South Island, New Zealand. Journal of Petrology, 44(11), 2053–2080. https://doi.org/10.1093/petrology/egg070
- Price, R. C., Johnson, R. W., Gray, C. M., & Frey, F. A. (1985). Geochemistry of phonolites and trachytes from the summit region of Mt. Kenya. Contributions to Mineralogy and Petrology, 89(4), 394–409. https://doi.org/10.1007/BF00381560
- Slack, J. F., Palmer, M. R., Stevens, B. P., & Barnes, R. G. (1993). Origin and significance of tourmaline-rich rocks in the Broken Hill district, Australia. Economic Geology, 88(3), 505–541. https://doi.org/10.2113/gsecongeo.88.3.505
- Spandler, C., & Morris, C. (2016). Geology and genesis of the Toongi rare metal (Zr, Hf, Nb, Ta, Y and REE) deposit, NSW, Australia, and implications for rare metal mineralization in peralkaline igneous rocks. Contributions to Mineralogy and Petrology, 171(12), 104. https://doi.org/10.1007/s00410-016-1316-y
- Spandler, C., Slezak, P., & Nazari-Dehkordi, T. (2020). Tectonic significance of Australian rare earth element deposits. Earth-Science Reviews, 207, 103219. https://doi.org/10.1016/j.earscirev.2020.103219
- Sun, S. S., & McDonough, W. F. (1989). Chemical and isotopic systematics of oceanic basalts: Implications for mantle composition and processes. In A. D. Saunders & M. J. Norry (Eds.), Magmatism in the ocean basins (pp. 313–345). Geological Society. Special Publications. No. 42. https://doi.org/10.1144/GSL.SP.1989.042.01.19
- Tanaka, T., Togashi, S., Kamioka, H., Amakawa, H., Kagami, H., Hamamoto, T., Yuhara, M., Orihashi, Y., Yoneda, S., Shimizu, H., Kunimaru, T., Takahashi, K., Yanagi, T., Nakano, T., Fujimaki, H., Shinjo, R., Asahara, Y., Tanimizu, M., & Dragusanu, C. (2000). JNdi-1: A neodymium isotopic reference in consistency with LaJolla neodymium. Chemical Geology, 168(3–4), 279–281. https://doi.org/10.1016/S0009-2541(00)00198-4
- Totland, M., Jarvis, I., & Jarvis, K. E. (1992). An assessment of dissolution techniques for the analysis of geological samples by plasma spectrometry. Chemical Geology, 95(1–2), 35–62. https://doi.org/10.1016/0009-2541(92)90042-4
- Weisenberger, T. B., Spürgin, S., & Lahaye, Y. (2014). Hydrothermal alteration and zeolitization of the Fohberg phonolite, Kaiserstuhl Volcanic Complex, Germany. International Journal of Earth Sciences, 103(8), 2273–2300. https://doi.org/10.1007/s00531-014-1046-1
- Zhang, J., & Lee, C. T. A. (2021). Disequilibrium crystallization and rapid crystal growth: A case study of orbicular granitoids of magmatic origin. International Geology Review, 63(7), 900–916. https://doi.org/10.1080/00206814.2020.1734975
- Zhou, B., & Whitford, D. J. (1994). Geochemistry of the Mt Wright Volcanics from the Wonominta Block, northwestern New South Wales. Australian Journal of Earth Sciences, 41(4), 331–340. https://doi.org/10.1080/08120099408728142
- Zhou, B. (1992). Petrological, geochemical and Sr–Nd isotopic studies of metamorphosed mafic rocks in the Wonominta Block, north-western New South Wales [unpublished PhD thesis]. The University of Sydney.