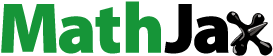
Abstract
Cereal production in Australia is severely impacted by stubble-borne pathogens which can survive multiple seasons within cereal residues (stubble). Microwave radiation may be able to reduce or eliminate the pathogens, but the energy requirements first need defining. Hence, the dielectric properties of wheat and barley stubble with different pathogen loads were investigated at 10%, 15%, 30% and 100% moisture content using an open-ended coaxial probe in a spectral band covering 915, 2450, and 5800 MHz. A significant increase in dielectric constant and loss factor was observed with increasing stubble moisture. The dielectric constant and loss factor were lower in the crown (basal) section of stubble compared with the stem (20 cm from base), due to differences in density. When stubble moisture was 100%, the loss factor of barley was higher than wheat. Infection of stubble by different pathogens did not affect the dielectric properties. Microwave heating could therefore be an effective method to heat cereal stubble to eradicate a range of cereal pathogens, especially at lower frequencies and high moisture content, at which the loss factor is high. This research serves as a starting point to define requirements for further development of effective microwave radiation treatments under field conditions.
Introduction
Fusarium crown rot, yellow leaf (tan) spot, and common root rot are significant diseases of cereal crops in Australia. The fungal pathogens responsible for each of these diseases can survive across seasons in cereal stubble or residues. In Australia, cost to the wheat industry alone, exceeds AUD 90 million for Fusarium crown rot (caused primarily by Fusarium pseudograminearum), and AUD 30 million for common root rot (caused by Bipolaris sorokiniana) (Murray and Brennan Citation2009b). Net blotches (caused by Pyrenophora teres f. teres and P. teres f. maculata) cause AUD 62 million damage to barley crops (Murray and Brennan Citation2009a). Conservation agriculture practises such as retention of cereal stubble are increasing the incidence of these diseases due to the preservation of inoculum in the previous years’ rows of cereal (Simpfendorfer et al. Citation2019).
Previous management options such as tillage and burning were effective at reducing levels of stubble-borne fungal pathogens, such as inoculum of Fusarium crown rot, common root rot, and net blotches. However, these practices are incompatible with current conservation-agriculture practices, because they are associated with reduced soil water storage, poorer soil health, and increased erosion (Ugalde et al. Citation2007). Disease often recurs due to the persistence of background inoculum or growers returning to susceptible crops prematurely. Complete elimination of inoculum from cereal stubble and soil is needed to break the disease cycle. Heat treatment is an effective method for eliminating pathogens from cereal stubble and soil (Bottomley et al. Citation2017), but no commercially available options currently suit conservation-agriculture systems.
Microwave radiation has recently been identified as a promising new system to heat-kill pathogens in cereal stubble whilst still allowing retention of these residues. Microwave radiation has been shown to eliminate conidia (i.e. spores) of B. sorokiniana and macroconidia of F. pseudograminearum and F. cerealis from spore solutions (Petronaitis et al. Citation2022), and F. pseudograminearum mycelia from infected durum wheat stubble (Petronaitis et al. Citation2018). Similarly, populations of various seed-borne pathogens, including Fusarium species in cereals, have been reduced by using microwave radiation, but at the expense of seed viability (Knox et al. Citation2013; Taheri et al. Citation2019; Citation2020). These studies support the concept of using microwave radiation as a fast and effective control strategy for a range of cereal pathogens. Extending microwave treatments from laboratory experiments to cereal stubble in the field will first require knowledge of the permittivity of cereal stubble to microwave radiation and heat uniformity within stubble, as these qualities will affect microwave efficiency, resources (such as energy and time) and consequently cost.
The suitability of microwaves as a heat-delivery system for cereal stubble has not been investigated. To do this, the dielectric properties of cereal stubble at microwave frequencies must be investigated, as the dielectric properties determine the rate and extent to which a material can heat at these frequencies (Nelson Citation2010). The dielectric properties of wheat straw (the portion of stem cut at grain harvest) have been described several times, but only in the radio frequency range of 1 kHz to 1000 kHz for the purpose of developing a capacitance-based moisture sensing method for straw and/or grain (Ko and Zoerb Citation1970; Guo et al. Citation2013; Jafari et al. Citation2020). In all cases, the dielectric properties were a function of the frequency of the electric field, as well as the moisture content, density, and temperature of the wheat straw. As such, the dielectric properties of cereal straw or stubble requires defining in the microwave frequency range, as microwaves are less expensive and not limited to material of regular or simple shape, as is the case with radio frequency heating (Mazima et al. Citation2018).
The dielectric properties of a material, generally expressed as relative complex permittivity, can be used to describe how the material will behave within an electromagnetic field, including microwave fields. Subsequently, these properties can be used to estimate the rate at which the material, for example cereal stubble, can be heated. To measure the relative complex permittivity () of a material, both the real (dielectric constant) and imaginary (loss factor) components must be determined. These are given by
where
represents the dielectric constant,
and
represents the loss factor. The dielectric constant is related to the material’s ability to store electromagnetic energy, with a higher
indicating that more energy can be stored, but the dielectric constant can also influence reflection (i.e. the amount of energy reflecting from the surface and the amount of energy entering the material) of the electric field. The loss factor represents the material’s ability to convert electromagnetic energy, for example from the electric field imposed by microwaves, into heat energy. In practical terms, a higher
means more field energy is transformed into heat energy where it is absorbed in the material (i.e. volumetric heating) and can be transferred to other parts of the material (via conduction). These factors are influenced by frequency, but also the temperature, moisture content, composition and bulk density of the material (Nelson Citation2010).
Various techniques for measuring the dielectric properties of agricultural materials have been described (reviewed in Nelson Citation2010). The open-ended coaxial line technique has been used on a large range of fruits, vegetables, and grains (reviewed by Guo et al. Citation2010), with the technology developed commercially and most notably used as a rapid moisture probe for grain and seed (Nelson Citation2010). More recently, this method has been used to determine the dielectric properties of legume grains (Guo et al. Citation2010; Jiao et al. Citation2011; Taheri et al. Citation2018). An open-ended coaxial (line) probe is used to measure the reflected signal of a given material at a range of frequencies (i.e. broadband), calibrated with samples of known permittivity (e.g. air open circuit, water and a short circuit), from which the permittivity of the material of interest can be calculated (Nelson Citation2010). This method is a reliable way of measuring dielectric properties and has been adopted by both industry and the scientific community, making it a sensible option for measuring materials such as cereal stubble whose dielectric properties are yet to be described.
The dielectric properties of cereal stubble infected with F. pseudograminearum, B. sorokiniana, and P. tritici-repentis were measured. An open-ended coaxial probe was used to measure the reflected signal of relative permittivity of cereal stubble in the microwave spectrum. Given that the relationship between moisture and dielectric properties is strongly correlated, we hypothesised that the dielectric properties of wheat and barley stubble will be higher with increased moisture content at microwave frequencies. In addition, we assessed whether the fungal pathogen infection status and crop species affected dielectric properties of cereal stubble, as these are important considerations in designing methods for broadacre-scale microwave application for controlling all three of our target fungal pathogens.
Materials and methods
Dielectric stubble experiment
Cereal stubble collection
Wheat (cv. Lancer) and barley (cv. Spartacus) stubble, infected with B. sorokiniana (Bs) or F. pseudograminearum (Fp) or co-infected (Bs + Fp), were collected post-harvest from inoculated field plots located in Gurley, New South Wales in 2020 (-29.82837, 149.99848). Barley stubble (cv. Spartacus) naturally infected with P. teres f. maculata and P. teres f. teres (Pte) was collected post-harvest from an experimental field at Tamworth Agricultural Institute, Tamworth, New South Wales in 2020 (-31.152353, 150.984708). All stubble samples had naturally senesced and dried at the time of collection. Cereal stubble was prepared by separating whole plants into individual tillers, removing soil, and cutting into 20 cm lengths (from crown). A subsample of 10 tillers was submitted to the South Australian Research and Development Institute, Urrbrae, Australia to verify the presence of the pathogen via quantitative polymerase chain reaction (qPCR). Quantification of pathogens within each stubble type, collected from the two locations, is outlined in . Tillers that were asymptomatic to Fusarium crown rot (i.e. free of sub-crown internode, crown, or stem browning) were deliberately selected for use in the nil treatments, as there were background F. pseudograminearum populations in the non-inoculated (nil) field plots ().
Table 1. Mean pathogen DNA levels present in a sub-sample of each cereal cultivar, cereal type and pathogen combination, aquired via quantitative polymerase chain reaction (qPCR) and expressed as pgDNA/g stubble for F. pseudograminearum (Fp) and B. sorokiniana (Bs) or kDNA copies/g stubble (P. teres f. maculata and P. teres f. teres), averaged across two replicates.
Cereal stubble preparation
Four moisture content (MC) levels for each pathogen-cereal stubble combination were prepared to analyse the change in dielectric properties associated with stubble moisture content. The selected moisture contents were based on expected field equilibrium (10% and 15%), timber fibre saturation (30%), and wet-weather saturation (100%). Final moisture content (MCf) was achieved by adding a predetermined mass of distilled water (mw (g)) to the sample dry mass (ms (g)) as determined by the following equation:
where MCf represents the final moisture content and MCi represents the initial moisture content. Samples were dried in a dehydrator at 40 °C for 48 h to achieve sample dry mass (ms) without affecting the viability of target fungal pathogens. Deionised and autoclaved water was sprayed onto the cereal stubble (by weight), in polyethylene bags, to achieve final moisture contents. Sealed bags were refrigerated at 5 °C to prevent pathogen overgrowth and mixed twice daily for 5-6 days to ensure moisture was evenly distributed among the cereal stubble. After the experiment, samples were weighed and dried again at 105 °C for 48 h to determine the true MC of each sample using wet and dried weights of the samples.
Measurement of dielectric properties
Dielectric properties were measured using an open-ended coaxial probe with network analyser (E8364B PNA network analyser, frequency range 10 MHz − 50 GHz) using Agilent software (Agilent Technologies 85070, Agilent, Santa Clara, California, USA). Stubble pieces were measured at frequencies between 700 and 7000 MHz, which include the allowed microwave Industrial, Scientific, and Medical (ISM) frequencies of 915, 2450, and 8500 MHz. Initial calibrations were performed using open circuit (air), a short circuit (aluminium foil sheet), and tap water at 22 °C.
Stubble pieces were brought to room temperature (22 °C) prior to experimentation. Tillers were cut lengthways and flattened to create a flat surface for the open-ended coaxial probe, with the exterior surface of the tiller in contact with the probe. Measurements were taken from two locations on the tiller, at 5 cm and 15-20 cm above the crown, hereafter referred to as “crown” and “stem” portions, respectively. Leaf sheath was generally present on the outside of the 15-20 cm samples and was left intact, given that preliminary readings did not appear to differ based on the presence or absence of leaf sheath.
Experimental design
For the dielectric experiment, two tillers per sample were measured, with mean values of each sample being used in the analysis. Two replicates of all treatments were conducted. The experiment was conducted over two days and the order of treatments was randomised within each replicate day (replicate one was conducted on day one and replicate 2 was conducted on day two).
The study was set up as a 5-factor experiment with the following factors: pathogen (Fp, Bs, combined Fp and Bs, Ptr, and a nil control), cultivar (Lancer and Spartacus), moisture content of the stubbles (10%, 15%, 30%, and 100% moisture content on a dry weight basis), location of the measurement along the stubble (i.e. stubble portion: crown or stem), and the frequency of the electromagnetic fields (700, 1000, 2000, 3000, 4000 5000, 6000, and 7000 MHz). Analysis of variance (ANOVA) was performed on the data. Data was tested for symmetry around the grand mean (normality), prior to applying the ANOVA. Factor and interaction means were compared using Least Significant Differences (p = 0.05) to determine significant differences in the data. Data means were exported to MatLab software (Mathworks Inc., USA), for surface plotting to visualise the data.
Stubble characterisation experiments
Additional stubble characterisation experiments explored differences between stem and crown portions of the stubble and between and wheat and barley stubble. This allowed us to determine whether differences in dielectric properties between these traits were primarily due to structural differences (i.e. stubble microstructure density, cell size, and arrangement) or non-structural differences (i.e. using powdered stubble tissue). The nil-pathogen Lancer wheat and Spartacus barley specimens were used in these experiments, as pathogen status did not significantly affect dielectric properties.
CT scanning
X-ray computed tomography (CT) scanning was used to measure the tissue volume of the stubble. Five replicates of each combination of cultivar × stubble portion were wet to 50% MC (as described previously) and scanned in a fully randomised replicate block sequence. Samples were weighed before and after scanning, and then again after drying at 60 °C for 72 h, to estimate the MC of each stubble segment during scanning. Dry weight per unit length (mg/mm) was further determined by weighing a 5 mm subsection of the dried stubble measured with a digital calliper.
Stubble pieces were scanned using a GE-Phoenix V|tome|xs 240 micro-CT scanner (manufactured 2010, GE Sensing & Inspection Technologies GmbH, Niels-Bohr-Straße 7, 31515 Wunstorf, Germany) located at the University of New England, Armidale, NSW Australia, with “Datos” acquisition software (version 2.2.1.695) and Phoenix datos|x 2 Reconstruction (version 2.2.1.716) software. Individual stubble pieces were mounted vertically on the rotating stage and imaged using the predetermined optimal X-ray tube settings (80 kV, 120 µA, 600 ms integration time per projection, focal spot 4 µm diameter). A 1000 × 1000-pixel detector array (DXR-250) was used to capture 3200 projections (full 360-degree rotation) using the GE constant rotation CT function, with a resultant isotropic voxel side length of 6.94 µm and tomographs were projected across the 16-bit depth dynamic range when reconstructed.
Reconstructed tomographs were imported directly into FIJI image J -version 1.53j (Schindelin et al. Citation2012). A 5.00 mm section of the stem was selected by extracting 720 slices from the centremost section of the scan. The volume was smoothed using a 2 D gaussian filter (sigma = 1.5) to remove the noise associated with the generally low attenuation of x-rays by the low electron dense material. Voxels with a density greater than that of the air were manually selected using a bi-level threshold tool and the volume classified as dry matter voxels was determined using a voxel counting method. The structured density of the 5 mm scanned section was determined from the scan volume and the samples mass. The R statistical package was used to analyse the measured parameters (Lenth Citation2016). Linear models were fitted with species and stem portions as predictors and model diagnostics were used to ensure model assumptions were upheld. An ANOVA was performed and post-hoc multiple comparisons were carried out using Tukey’s method (R package:lsmeans) (Lenth Citation2016).
Light microscopy
Light microscopy was used to evaluate the stubble microstructure, specifically the thickness and intensity of the epidermis, parenchyma and vascular bundles of the crown and stem sections. Using the same experimental design as the CT scanning experiment, dried stubble pieces were fixed in formalin-acetic acid-alcohol (FAA) (formaldehyde-ethanol-acetic acid-water; 10:50:5:35). Samples were dehydrated in a Leica TP 1020 tissue processor (Leica Biosystems, IL, USA) through a graded ethanol series: 70, 80, 90, 95% ethanol for 2 h in each bath, two series of 100% ethanol, and one of ethanol-xylene (50:50) 1 h per bath; subsequently, xylene for 1 h, then xylene for 2 h, and finally two baths of paraffin tissue embedding medium (Leica Paraplast Plus, IL, USA) of 2 h each.
The dried samples were embedded in paraffin wax with a Leica EG1150 Tissue Embedder (Leica Biosystems, IL, USA) and transverse sections were cut to 20 µm with a Leica SM 2010 slide microtome (Leica Biosystems, IL, USA). Sections were de-wrinkled in a water bath at 41 °C and transferred to slides which were laid onto a histoplate at 47 °C for at least 1.5 h. The slides were dewaxed in two xylene baths and subsequently hydrated through an ethanol series of 100, 90, 70, 50% ethanol, and distilled water for 5 min in each bath (O'Brien and McCully Citation1981; Retamales and Scharaschkin Citation2014). Slides were stained with Safranin O (Matheson Coleman & Bell) and counterstained with Astra Blue (Glentham Life Sciences) (Kraus et al. Citation1998; Warwick et al. Citation2017) as follows: 6 min in Safranin O, two baths of distilled water of 1 min each, 2 min in Astra Blue, 1 min in distilled water, an ethanol series at 50, 70, 90, and 100%, 30 s in each bath, and finally, 30 s in SolV21C (xylene substitute) (Muraban Laboratories, NSW). The samples were mounted with Euparal (Agar Scientific, UK) (Warwick et al. Citation2017).
All slides were scanned in a Nanozoomer Hamamatsu 2.0 RS (Hamamatsu Photonics K.K., Japan), set at 40x magnification, with a Z-stacking of 7 layers at 1 µm. The samples were measured with NDP.view2 (Hamamatsu Photonics K.K., Japan) viewing software. Five measurements were taken per image of the culm thickness (measured from the cuticle to the end of the ground tissue), the outer ring thickness (cuticle, epidermis, and hypodermis), the inner ring thickness (ground tissue) and the number of vascular bundles of the outer ring, inner ring, and total vascular bundles.
The mean values for each trait were analysed using a linear mixed model framework. Fixed effects were included to account for crop species and stubble portion, along with their interaction. Replicate was fitted as a random effect and individual samples within replicates accounted for residual error. The model was fitted using the ASReml-R package (Butler et al. Citation2017) in the R statistical computing environment (R Core Team Citation2019) whereby all variance components were estimated using residual maximum likelihood (Patterson and Thompson Citation1971).
Dielectrics of ground material
Air-dried stubble samples were ground in a ball mill for 2 min (at 30 s−1 oscillations) each, resulting in a uniformly fine powder. The powder was then transferred into a custom made guarded parallel plate apparatus (as described in Agilent Technologies Citation2003) and packed to density of 0.495 g cm−3 with guarded plate diameter of 13.6 mm and depth of 1.6 mm for the material under test. Using the equation below we calculated complex relative permittivity of the material (or dielectric constant):
where
is the relative permittivity,
is the thickness of the material (m),
refers to the equivalent parallel capacitance of the material (F), A is the area of the guarded plate and
is the permittivity of free space (which we assumed to be 8.854 × 10−12 Fm−1) (Agilent Technologies Citation2003). Capacitance was determined using an Atlas LCR45 passive component impedance meter (Peak Electronic Design Ltd, UK) measured at a frequency of 200 kHz which provided the most optimal resolution with this experimental apparatus. The R statistical package was used to analyse the measured parameters (Lenth Citation2016). Linear models were fitted with species and stem portions as predictors and model diagnostics were used to ensure model assumptions were upheld. An ANOVA was performed and post-hoc multiple comparisons were carried out using Tukeys method (R package:lsmeans) (Lenth Citation2016).
Results
Dielectric constant (
) (real part of dielectric properties)
The dielectric properties of both the crown and stem portions generally increased with increasing MC and decreased with increasing frequency across the microwave spectrum (i.e. at 700, 1000, 2000, 3000, 5000, 6000 and 7000 MHz) (). Dielectric constant increased with MC on average across both crown and stem portions, which was evident by a main effect of MC (p < 0.01, ). The increase in dielectric constant in the stem portions (), however, was significantly greater than the increase observed in the crown portions at higher MCs, for example at 100% MC (p < 0.01, ). The dielectric constant of stem portions was also significantly higher at 30% MC at most frequencies (). This large difference between the dielectric constant of crown and stem portions at the higher MCs resulted in an additional main effect of stubble portion (crown versus stem) when averaged across all MCs (p < 0.01, ). Increasing stubble MC from 10% to 15% did not result in a significant increase in dielectric constant in the crown or stem portions individually () or when averaged across both stubble portions () (p < 0.01). Infection status (p = 0.52) and crop species (wheat cv. Lancer versus barley cv. Spartacus) (p = 0.65) also did not affect dielectric constant (at 2 GHz) (data not shown).
Figure 1. Surface plot showing the dielectric constant of the (a) crown and (b) stem portions of stubble (averaged across crop species) at a range of cereal stubble moisture contents for eight frequencies in the microwave spectrum.

Table 2. Main effect of moisture at four moisture contents (%) on the dielectric constant for eight frequencies in the microwave spectrum. There was a significant (p < 0.05) effect of moisture content at all frequencies.
Table 3. Main effect of cereal stubble portion (stem or crown) on the dielectric constant for eight frequencies in the microwave spectrum. There was a significant (p < 0.05) difference between stubble portions at all frequencies.
Dielectric loss factor (∈″) (imaginary part of dielectric properties)
Loss factor was affected by MC, stubble portion and crop species (p < 0.01). Loss factor increased with increasing MC in the stems, with the highest loss factor observed at 100% MC in stems for both the wheat and barley cultivar examined ( and ). In the crown portions, the loss factor increased significantly when the MC of stubble was 30% (compared with loss factor at 10% stubble moisture), but was the same (for barley cv. Spartacus, ) or decreased (for wheat cv. Lancer, ) in crown portions when further increased to 100% MC. Loss factor differed significantly between barley cv. Spartacus and wheat cv. Lancer within the stem tissue at 100% MC across all frequencies tested. Within these treatments (stem tissue at 100% MC), the loss factor generally decreased with increasing frequency in barley cv. Spartacus (6.03 at 700 to 3.74 at 7000 MHz, ) and increased with increasing frequency in wheat cv. Lancer (2.24 at 700 to 3.03 at 7000 MHz, ). No difference in loss factor was observed between the crown and stem portions at 10%, 15% or 30% MC in the interaction between MC, stubble portion and crop species (data not shown). Fungal pathogen infection status also did not affect the loss factor (p = 0.52, data not shown).
Stubble characterisation
The density of the intact stubble was significantly higher in the crown portions compared with the stem portions of stubble (p = 0.01, ). Once the physical structure of the stubble was removed by grinding into a powder, the dielectric of the ground material was also significantly higher in the crown than in the stem portions (p = 0.00, ). Overall, the dielectric constant of the ground material, measured without additional moisture, was higher than what was observed for the intact stubble, possibly due to more air in the intact stubble samples.
Table 4. Main effect of stubble portion (stem or crown portions) on the density of intact stubble (density) and the dielectric constant of the ground and compacted stubble (dielectric). There was a significant (p < 0.05) difference between stubble portions for both the density and dielectric measurements.
There were clear differences between the transverse structures of the two cereal stubbles examined. Barley cv. Spartacus had a significantly thicker (for crown tissue: 204.5 µm compared with 137.2 µm in wheat) or similarly sized (for stem tissue: 91.1 µm for barley and 77.8 µm for wheat) outer ring of the epidermis (p = 0.04) compared with the wheat cv. Lancer. However, the much larger inner ring thickness in Lancer wheat (635.2 µm) compared with Spartacus barley (296.4 µm, p = 0.00) means that the total culm thickness was still larger overall in wheat (740.5 µm), compared with barley (444.9 µm) (p < 0.01, ). Wheat cv. Lancer also contained a greater number of vascular bundles (54.2 bundles) compared with that of barley cv. Spartacus (40.5 bundles) (p < 0.01). When averaged across crop species, more vascular bundles were found in the crown (19.4) compared with the stem (15.8) in the outer ring (p < 0.01), but this difference was not evident in the inner ring (p = 0.85).
Figure 4. Transverse sections of stubble taken from the stem portion of (a) wheat cv. Lancer and (b) barley cv. Spartacus. Scale bar represents 500 µm.

Water loss during the 30-minute CT scan time was greatest in the stems of barley cv. Spartacus (-19.2%, p < 0.01). Water loss in the crowns of both crop species were similar (-12.6% in barley cv. Spartacus and −10.2% in wheat cv. Lancer). The least amount of water was lost from the stems of wheat cv. Lancer (-6.0%). This water loss characteristic may be important for field efficacy of microwaving, for example, barley cv. Spartacus may require more rapid treatment after a rain or a wetting event before too much moisture is lost from the stubble.
Discussion
This study is the first to measure the dielectric properties of wheat and barley stubble at different MCs in the microwave frequency range (700 to 7000 MHz). As expected, dielectric constant and loss factor increased with increasing MC. Different portions along the stubble length appeared to have varied dielectric properties indicating that the crown section of cereal stubble does not have as much potential for heating compared with the stem. Interestingly, barley (cv. Spartacus) also had a higher loss factor compared with wheat (cv. Lancer) when stubble MC was high, although these differences may be too small to make a practical difference. Our models suggest that microwave radiation would be effective for heating cereal stubble for the purpose of eradicating cereal pathogens, especially at lower frequencies and moderate to high MC, when the loss factor is high.
The dielectric properties, both dielectric constant and loss factor, increased with increasing moisture in the cereal stubble samples. This is not surprising, as the moisture relationship with dielectric properties is highly correlated, to a point where dielectric properties are used as a commercial method for moisture sensing as they indicate the polarity of a material (Nelson Citation2010). Similar findings have been observed in other crops such as legume (Guo et al. Citation2010; Jiao et al. Citation2011; Taheri et al. Citation2018) and wheat grains (reviewed by Mazima et al. Citation2018). These studies also observed a decrease in dielectric constant and loss factor with increasing frequency, which we observed similarly at 15%, 30% and 100% MC for the dielectric constant, and at 100% MC only for the loss factor. In these wetter stubble samples, dissolved solids from the stubble may have leached into water that was present on the stubble surface, and as such these dissolved solids in water would result in higher dielectric properties (Nelson Citation2001).
In this study, cereal stubble with a higher MC would have had a greater number of dipolar molecules (water) than the dry cereal stubble. These water molecules interact with electromagnetic fields and are subsequently more responsive to heating via microwave radiation. The dielectric constant of cereal stubble, even at 100% MC, was 7 (averaged across crown and stem), which is much closer to the dielectric properties of air or paper (dielectric constant of 1 and 3, respectively), than water (dielectric constant of 78) (Brodie Citation2021). The higher dielectric constant indicates a greater reflection of energy away from the surface of the material, reducing the overall amount of microwave energy entering the sample. This may reduce the heating effect, however, there is also better absorbance in the material provided by the higher loss factor. The increase in loss factor with MC subsequently indicates that there will be more heating in cereal stubble with a higher MC, as the field energy is converted into heat energy where it is absorbed by the material. Given these results, the microwave treatment of cereal stubble is still likely to be maximised in moist stubble (i.e. in 30-100% MC stubble). Microwave efficacy could therefore be maximised when cereal stubble is wet, for example following rainfall events, or by applying water to the stubble prior to microwaving.
Dielectric properties (both the dielectric constant and loss factor) of the stem portions increased significantly with moisture. Increases were also observed in the crown portions, but these were much lower. The dielectric constant, even at the highest MC, was approximately 3 for the crown segments and 11 for the stem segments. Additionally, loss factor did not increase from 30% to 100% MC in the crown segments but continued to increase in the stem segments at these MCs. It is likely that differences in the structure of these two plant parts can explain majority of this variation. The crown portions of stubble had a significantly higher density than the stems, and the density of a material is known to affect the interaction between a given material and a microwave field (Nelson Citation2010). Similar findings have shown wheat grains and straw to have different dielectric properties and also vary in their response to moisture (Jafari et al. Citation2020). The porosity of the different plant parts likely contributed to this result.
Interestingly, when stubble structure and density was removed via grinding of samples into a powdered form, there was still a difference between the dielectric of the crown and stem portions. The results were, however, more similar, with approximately 7.2 recorded for the crown segments and 6.7 for the stem segments (at ambient MC). This suggests there may be some inherent differences in chemical properties between the two plant parts. The only other observed difference between the crown and stem was that the crown had a higher number of vascular bundles in the outer ring of the epidermis, but it is not clear whether this can account for any of the observed differences. Practically, different treatment approaches may be required when heating the different portions of the cereal stubble, for example pathogens residing in the crown (targeting F. pseudograminearum and B. sorokiniana) may require more energy than pathogens residing in the stem (targeting P. teres).
Differences in dielectric properties between the two crop species was observed in stem sections at 100% MC. Specifically, the wheat cv. Lancer had a lower loss factor compared with the barley cv. Spartacus, suggesting that the stem portions of Lancer heat less than Spartacus under high moisture conditions and lower frequencies. There are two possible explanations for this observation. Lancer is a short plant height cultivar with relatively dense culms, and again, density may have an affect (Nelson Citation2010). However, no differences in density or stem wall thickness were observed between the two crop species, so this explanation may be reasonably excluded. Further, if crop species was influenced by density alone, we assume that a crop species effect would have been observed for both dielectric constant and loss factor across a larger range of frequencies and moisture contents (as was observed for differences between the crown and stem portions). It is therefore more likely that we detected differences in dissolved ions and other solids present in the moisture component of the wetted stubble, which are known to be more reactive at lower frequencies (Nelson Citation2001). Barley is naturally more salt tolerant and can accumulate more salt (NaCl) than bread wheat (Munns and Tester Citation2008), and higher energy loss due to ionic conduction can occur at low frequencies when there are more dissolved salts (i.e. larger number of potential hydrogen bonds) (Brodie Citation2021). This further supports the idea that there may be an overall difference in dielectric properties between wheat and barley, however, further study is needed to explore these lower frequency responses, as well as testing of more than one cultivar of each crop species.
No difference between infected and uninfected cereal stubble was observed in our experiments. It is important to consider that there was no true “nil infection” stubble available due to background infection of almost all stubble by F. pseudograminearum. The Spartacus barley stubble collected from the Tamworth site containing high levels of P. teres did have comparatively low levels of F. pseudograminearum, but there were still no differences in dielectric properties detected between this stubble and Spartacus barley stubble collected from the Gurley site. Ideally, our results would be confirmed using a control cereal stubble that is free of any pathogen, but this is inherently difficult when using field collected stubble due to the widespread prevalence of F. pseudograminearum in Northern NSW (Petronaitis et al. Citation2021). Our finding that the colonisation of cereal stubble by different pathogens did not affect dielectric properties in the microwave frequency range may, in fact, be an advantage when it comes to treating infected cereal stubble. The co-infection of cereal crops, for example by F. pseudograminearum and B. sorokiniana, is common in Australia (Simpfendorfer and McKay Citation2019). The presence of these two pathogens together did not appear to affect the heating potential of cereal stubble. Given that these pathogens can be eradicated using a similar amount of microwave energy (< 300 J g−1 in a 10 ml spore suspension) the treatment for one pathogen will likely eradicate both (Petronaitis et al. Citation2022).
Several other factors may also affect the dielectric properties of cereal stubble, and therefore the ability to heat stubble using microwaves. Advanced age and weathering of stubble, and subsequent decomposition, will likely reduce the density and probably increase porosity. Older stubble may therefore have higher dielectric properties, although this hypothesis should be tested experimentally. Further, temperature affects the strength of hydrogen bonds, so higher temperatures will subsequently reduce relaxation time (Brodie Citation2021). As such, temperature and other weather conditions would need to be considered in a field setting.
Our measurement of the dielectric properties of cereal stubble across the microwave frequency range demonstrates that microwaving could be an effective method of heating cereal stubble. Efficacy would be maximised when stubble MC is in the range of 30- to 100%, achieved in the field through timing with natural weather events (e.g. following rainfall, dew, or fog) or via water application (e.g. irrigation or spray) to the stubble prior to microwaving. Different treatment approaches may be required when heating the different portions of the cereal stubble due to the reduced heating efficacy of crown tissue predicted in our models for both dielectric constant and loss factor. Future research is required to investigate the dielectric properties of a broader range of crop species and varieties, also considering stubble age and other abiotic conditions. Although field validation will be important in the future, this study supports further development of microwave application for disease control at the broadacre scale.
Author contributions
This article is based primarily on a student thesis prepared by PhD candidate Toni Petronaitis. All authors contributed to the study conception. Experimentation and data collection was performed by Toni Petronaitis. Data analysis was conducted by Toni Petronaitis, Graham Brodie, and Richard Flavel. The draft manuscript was written by Toni Petronaitis and all authors commented on previous versions of the manuscript. All authors read and approved the final manuscript.
Acknowledgements
Ms Petronaitis thanks the GRDC and New South Wales Department of Primary Industries for co-funding her Grains Agronomy and Pathology Partnership PhD scholarship (BLG211). Technical assistance provided by Prof Mohan Jacob and Dr Kenneth Leong is gratefully acknowledged. The authors also thank Dr David Backhouse, Dr Narelle Moore, Dr Ahsan Asif and Dr Duy Le who reviewed the manuscript.
Disclosure statement
No potential conflict of interest was reported by the authors.
Additional information
Funding
Notes on contributors
Toni Petronaitis
Mrs Toni Petronaitis: Toni joined the NSW Department of Primary Industries as a research officer in cereal pathology in 2017 after completing her BSc (Hons) at the University of New England (UNE) in 2016. Toni works within the cereal disease management team who are recognised for developing and extending successful integrated disease management strategies. Toni is currently undertaking a Doctor of Philosophy (Grains Agronomy and Pathology Partnership PhD Scholarship, commenced 2019) under the supervision of Dr Steven Simpfendorfer (NSW DPI), Associate Professor David Backhouse (UNE) and Dr Graham Brodie (JCU). Toni is investigating the epidemiology and novel control of winter cereal pathogens in the northern grain region of Australia (New South Wales and Queensland). Toni is specifically interested in stubble-borne pathogens which survive between cropping seasons in cereal stubble/residues, which makes them inherently difficult to control in conservation agriculture systems. Toni is also investigating a novel approach to controlling these pathogens in the field using microwave radiation.
Graham Brodie
Dr Graham Brodie: Graham has 40 years of professional engineering experience, including 8 to 9 years of industrial engineering experience in the planning, design, construction, and maintenance of large-scale electrical distribution systems. Graham has also worked as an educator and academic with the Victorian Colleges of Agriculture and Horticulture for 7 years and the University of Melbourne for 25 years, as well as James Cook University as an adjunct professor since 2010. This has also involved the design, analysis, and development of high-powered microwave treatment systems, farm structures, automatic data acquisition systems, solar powered electrical and heating systems, and agricultural waste management systems. His research focuses on high powered microwave engineering applications in agriculture and forestry, improving water use efficiency in agriculture, small scale pump-storage electrical generation systems for remote homesteads, and applications of GIS and remote sensing technologies in agriculture and archaeology.
Steven Simpfendorfer
Dr Steven Simpfendorfer: Steven is based at the Tamworth Agricultural Institute (NSW Department of Primary Industries) where he leads research and extension programs on the integrated management of winter cereal diseases in northern NSW, Australia. He has 21 years’ experience in developing and extending integrated disease management strategies to support growers in limiting losses to winter cereal diseases. He is the recognised expert for developing regionally validated IDM strategies and providing diagnostic, surveillance and training support to industry, accompanied by a strong focus on the delivery and communication of outcomes to growers and advisors to facilitate rapid adoption. He was previously with NSW Department of Primary Industries at Wagga Wagga as a soil microbiologist from 1997-2000. Steven has currently published 42 peer reviewed journal articles, over 70 conference papers and over 200 extension articles from his research.
Richard J. Flavel
Dr Richard Flavel: Richard completed Rural Science at the University of New England (2008). He specialised in plant nutrition, with honours exploring the availability of sulfur from novel fertilizer sources in field. Following this he worked as a broadacre commercial agronomist based in Narrabri, Northern NSW before returning to UNE to complete a PhD (“Visualising and quantifying cereal root responses to phosphorus”). Richard has worked as a Research Fellow in plant-soil interactions for the Cooperative Research Centre for Polymers on non-wetting soils and the interactions between the wheat root systems, the soil physical structure and ‘functionalized polymers’ capable of moderating the soil moisture dynamics. In his current role as Crop Lecturer his research interest is in the soil physio-chemical interactions that occur at the root interface and the implications that mechanisms at this locus have for crop and plant productivity.
Nigel W. M. Warwick
Dr Nigel Warwick: Nigel is a Senior Lecturer in Plant and Crop Ecophysiology at the University of New England. His research has a strong focus on plant, crop, and horticultural ecophysiology. Nigel has successfully supervised Honours, Masters and Doctoral students from Australia, Bhutan, Ethiopia, Ghana, Jordan, India, Iran, Nepal and Timor Leste. He has postgraduates working on projects in the cereal, tomato and berry fruit industries. Currently, he has research collaborations with cereal breeders at the NSW DPI Tamworth Agricultural Institute and the Jodrell Laboratory at the Royal Botanic Gardens, Kew, in the United Kingdom. Prior to this, he worked on research projects on plant water use in horticultural systems, biochemistry of leaf senescence, salinity, wetland plant ecology and photosynthesis at Plant Physiology Division DSIR NZ, the School of Agriculture and Forestry at The University of Melbourne, the Institute of Biological Chemistry at Washington State University, USA, and the Department of Ecology and Evolutionary Biology/CRC for Freshwater Ecology at Monash University.
References
- Agilent Technologies. 2003. Application Note 1369-1: solutions for measuring permittivity and permeability with LCR meters and impedance analysers. USA: Agilent Technologies Inc.
- Bottomley P, Sutherland M, Percy C. 2017. Soil Solarisation as a method to deliver near zero level crown rot (Fusarium pseudograminearum) reference sites in the northern grain region of Australia (conference abstract). Sci Protect Plant Health, Brisbane, Australia:26–28.
- Brodie G. 2021. Modeling the very broad band dielectric properties of water. J Microw Power Electromagn Energy. 55(1):80–89.
- Butler D, Cullis B, Gilmour A, Gogel B, Thompson R. 2017. ASReml-R reference manual version 4. UK: VSN International Ltd, Hemel Hempstead, HP1 1ES.
- Guo W, Wang S, Tiwari G, Johnson JA, Tang J. 2010. Temperature and moisture dependent dielectric properties of legume flour associated with dielectric heating. LWT-Food Sci Technol. 43(2):193–201.
- Guo W, Yang J, Zhu X, Wang S, Guo K. 2013. Frequency, moisture, temperature, and density-dependent dielectric properties of wheat straw. Trans ASABE. 56(3):1069–1075.
- Jafari M, Chegini G, Rezaeealam B, Shaygani Akmal AA. 2020. Experimental determination of the dielectric constant of wheat grain and cluster straw in different moisture contents. Food Sci Nutr. 8(1):629–635.
- Jiao S, Johnson J, Tang J, Tiwari G, Wang S. 2011. Dielectric properties of cowpea weevil, black-eyed peas and mung beans with respect to the development of radio frequency heat treatments. Biosys Eng. 108(3):280–291.
- Knox OGG, McHugh MJ, Fountaine JM, Havis ND. 2013. Effects of microwaves on fungal pathogens of wheat seed. Crop Prot. 50:12–16.
- Ko R, Zoerb G. 1970. Dielectric constant of wheat straw. Trans ASAE. 13(1):42–47.
- Kraus JE, de Sousa HC, Rezende MH, Castro NM, Vecchi C, Luque R. 1998. Astra blue and basic Fuchsin double staining of plant materials. Biotech Histochem. 73(5):235–243.
- Lenth RV. 2016. Least-squares means: the R package lsmeans. J Stat Softw. 69:1–33.
- Mazima JK, Johnson A, Manasseh E, Kaijage S. 2018. An overview of electromagnetic radiation in grain crops. Food Sci Technol Int. 1(1):21–32.
- Munns R, Tester M. 2008. Mechanisms of salinity tolerance. Annu Rev Plant Biol. 59:651–681.
- Murray GM, Brennan JP. 2009a. Estimating disease losses to the Australian barley industry. Austral Plant Pathol. 39(1):85–96.
- Murray GM, Brennan JP. 2009b. Estimating disease losses to the Australian wheat industry. Austral Plant Pathol. 38(6):558–570.
- Nelson S. 2001. Radio-frequency: and microwave dielectric properties of insects. J Microw Power Electromagn Energy. 36(1):47–56.
- Nelson S. 2010. Fundamentals of dielectric properties measurements and agricultural applications. J Microw Power Electromagn Energy. 44(2):98–113.
- O'Brien TP, McCully ME. 1981. The study of plant structure: principles and selected methods. Melbourne, Australia: Termarcarphi Pty. Ltd.
- Patterson HD, Thompson R. 1971. Recovery of inter-block information when block sizes are unequal. Biometrika. 58(3):545–554.
- Petronaitis T, Forknall C, Brodie G, Simpfendorfer S, Backhouse D. 2022. Killer Joules”: spores of Bipolaris sorokiniana and Fusarium species are susceptible to microwave radiation. Australas Plant Pathol. 51:433–440.
- Petronaitis T, Forknall C, Simpfendorfer S. 2018. Microwave radiation reduces survival of Fusarium pseudograminearum in durum wheat stubble. Australasian Plant Pathol. 47(4):375–378.
- Petronaitis T, Simpfendorfer S, Hüberli D. 2021. Importance of Fusarium spp. in wheat to food security: a global perspective. In: Scott P, Strange R, Korsten L, Gullino ML, editors. Plant diseases and food security in the 21st century. Vol. 10. Cham: Springer Nature; p. 127–159.
- R Core Team. 2019. R: A language and environment for statistical computing. Vienna, Austria: R Foundation for Statistical Computing.
- Retamales HA, Scharaschkin T. 2014. A staining protocol for identifying secondary compounds in Myrtaceae. Appl Plant Sci. 2(10):1400063.
- Schindelin J, Arganda-Carreras I, Frise E, Kaynig V, Longair M, Pietzsch T, Preibisch S, Rueden C, Saalfeld S, Schmid B, et al. 2012. Fiji: an open-source platform for biological-image analysis. Nat Methods. 9(7):676–682.
- Simpfendorfer S, McKay A, Ophel-Keller K. 2019. New approaches to crop disease management in conservation agriculture. In: Pratley J, Kirkegaard J, editors. Australian agriculture in 2020: from conservation to automation. Wagga Wagga: Agronomy Australia and Charles Sturt University; p. 173–188.
- Simpfendorfer S, McKay A. 2019. What pathogens were detected in central and northern cereal crops in 2018? GRDC Update Paper Sourced from: https://grdccomau/resources-and-publications/grdc-update-papers/tab-content/grdc-update-papers/2019/03/what-pathogens-were-detected-in-central-and-northern-cereal-crops-in-2018 [accessed 2021 August 3].
- Taheri S, Brodie G, Gupta D. 2020. Microwave fluidised bed drying of red lentil seeds: drying kinetics and reduction of botrytis grey mold pathogen. Food Bioprod Process. 119:390–401.
- Taheri S, Brodie GI, Gupta D, Dadu RHR, Taheri S, Brodie GI, Gupta D, Dadu RHR. 2019. Effect of microwave radiation on internal inoculum of ascochyta blight in lentil seeds at different seed moisture contents. Trans ASABE. 62(1):33–43.
- Taheri S, Brodie G, Jacob MV, Antunes E. 2018. Dielectric properties of chickpea, red and green lentil in the microwave frequency range as a function of temperature and moisture content. J Microw Power Electromagn Energy. 52(3):198–214.
- Ugalde D, Brungs A, Kaebernick M, McGregor A, Slattery B. 2007. Implications of climate change for tillage practice in Australia. Soil Tillage Res. 97(2):318–330.
- Warwick NW, Hailey L, Clarke KL, Gasson PE. 2017. Climate trends in the wood anatomy of Acacia sensu stricto (Leguminosae: Mimosoideae). Ann Bot. 119(8):1249–1266.