Abstract
Diabetic nephropathy is one of the major complications of diabetes. Oxidative stress is implicated as an important mechanism by which diabetes causes nephropathy. The aim of the study was to examine the involvement of oxidative stress in the progression of nephropathy in STZ diabetic animals and to evaluate the potential of polyphenolic extract (PPE) in the treatment of diabetes mellitus. In this study, we examined whether prolonged oral administration of polyphenolic extract of Ichnocarpus frutescens could prevent the progress or improve the outcome of diabetic nephropathy induced by oxidative stress in STZ diabetic rats. Intraperitoneal glucose tolerance test (IPGTT) revealed a significant decrease in blood glucose levels at 180 min after glucose loading in Wistar albino rats fed with PPE. During the eight weeks of experimental period, diabetic rats exhibited wide range of symptoms, including loss of body weight, hyperglycemia, polyuria, proteinuria, renal enlargement, and total renal dysfunction. A significant increase in TBARS level was observed in diabetic kidney, which was accompanied by a significant decrease in enzymatic and non-enzymatic antioxidant levels. After eight weeks, PPE-treated groups showed a lower level of blood glucose compared with non-treated STZ diabetic rats. The increases in urinary albumin and protein after eight weeks of treatment were significantly inhibited by prolonged treatment with PPE. In addition, PPE attenuates the adverse effects on hepatic biomarkers. We found that PPE can effectively protect against aldose reductase activity and protein damage (albumin glycation), and showed that its action was mainly due to enriched polyphenolic content. Our results also showed that treatment with PPE normalized the increase in hyperalgesia (i.e., the response to thermal stimuli) associated with the induction of diabetes by STZ. PPE administration in diabetic rats clearly ameliorated diabetic complications, suggesting not only a natural antioxidant but also supportive therapy for the treatment of type II diabetes.
INTRODUCTION
Diabetic nephropathy (DN) is one of the most frequent causes of renal dysfunction. Approximately 30% of patients with diabetes experience diabetic nephropathy, which gradually develops to final renal failure (ESRD).Citation[1] Large-scale studies have established that the defining metabolic derangements (i.e., hyperglycemia, hyperlipidemia, and hyperinsulinemia), systemic and glomerular hypertension, oxidative stress, and advanced glycation end products (AGEs) increase the risk for diabetic renal disease.Citation[2] In addition, the persistence of hyperglycemia has been reported to increase the production of reactive oxygen species (ROS) through glucose auto-oxidation, abnormal metabolism of prostaglandins, and high polyol pathway flux. The prevention or retardation of diabetic nephropathy (DN) has become a major goal in biomedical research.Citation[3] Current methods used in clinical practice to slow progression of diabetic nephropathy include angiotensin system inhibition, blood pressure, glycemic control, and isocaloric protein restriction.Citation[4],Citation[5] Despite these remedies, the incidence of diabetic end-stage renal disease (ESRD) steadily increased over the past three decades, reaching epidemic proportions.
An increase in albumin excretion rate leading to micro-albuminuria is widely acknowledged as the earliest index of diabetic nephropathy and as a risk factor for the development of overt diabetic renal disease.Citation[6] First, although protein restriction delays aging-related glomerulosclerosis, animal studies showed that limiting calorie intake is by far more effective.Citation[7] Furthermore, some plant foods have advantageous properties, apparently unrelated to macronutrient composition. For example, polyphenolics extracted from tea-inhibited mesangial proliferation and significantly prolonged renal survival in experimental models of glomerulosclerosis.Citation[8] Polyphenol intake delayed ESRD in animal models of chronic renal failure.Citation[9] Previous studies have documented that hyperglycemia is associated with excessive free radical generation and oxidant stress and poor antioxidant status.Citation[10] A number of structurally diverse naturally occurring polyphenolic compounds have been studied in vivo to clarify their effectiveness in the prevention of diabetic nephropathy in experimental animals.Citation[11],Citation[12] Studies have shown that antioxidant treatment and good metabolic control is beneficial in slowing the progression of nephropathy in diabetes, and if the duration of diabetes is prolonged before reinstitution of normoglycemia, nephropathy is not easily reversed.Citation[13]
The leaves of Ichnocarpus frutescens are used extensively in the form of decoction for its antidiabetic activity by the tribal Karnataka and Utter Pradesh states for treating diabetes and jaundice.Citation[14] In our laboratory, anti-inflammatory, antioxidant activity and alpha glucosidase inhibitory activity of this plant have been reported.Citation[15] Based on our previous findings, the current study was designed to establish the biochemical and renal histopathological abnormalities that occur with the evolution of streptozotocin-induced diabetes in rats and to appreciate their possible reversal after the establishment of good metabolic control with a polyphenolic extract of Ichnocarpus frutescens. The aim of the current study was to identify a polyphenolic treatment pattern capable of slowing the progression of diabetic nephropathy more effectively.
MATERIALS AND METHODS
Chemicals
Streptozotocin was purchased from Sigma Chemical Co. (St. Louis, Missouri, USA). Folin-Ciocalteu reagent, pyrocatechol, quercetin, pentobarbitone sodium, bovine serum albumin (BSA), thiobarbituric acid, sulphosalicylic acid, reduced glutathione, nitroblue tetrazolium, NADH, sulfanilic acid, N-(1-naphthyl) ethylenediamine, vanadium trichloride, dimethyl sulphoxide β-mercaptoethanol, diammoniun sulphate (NH4)2SO4, NADPH, lithium sulphate (LiSO4), dl-glyceraldehyde, gentamicin, ethylene diamine tetra acetic acid (EDTA), trichloro acetic acid, oxalic acid, D-glucose, and Ellman's reagent (5,5′-dithiobis-(2-nitrobenzoic acid) were purchased from SISCO Research Laboratories Private Limited, Mumbai, India. All other chemicals and solvents were purchased locally and were of analytical grade.
Plant Materials
The fresh leaves of Ichnocarpus frutescens (L.) R.Br. (Apocynaceae) were collected from the Delta region of Cauvery River, Thiruchirappalli, India, in February 2004, and was authenticated at Botanical Survey of India (BSI), Central National Herbarium (CNH), Howrah, India (Ref. no.: CNH/I-I/87/2005-TECH/1326). An authentic voucher specimen was deposited in the Herbarium of Division of Pharmacognosy, Department of Pharmaceutical Technology, Jadavpur University, Kolkata, India.
Preparation of Polyphenolic Extract
Dried leaves of Ichnocarpus frutescens (500 g) were finely powdered, mixed with 70% methanol, and kept at room temperature for five days. After five days, it was filtered, and the solvent was evaporated. The residue was dissolved in water, and the aqueous layer was washed with petroleum ether several times until a clear upper layer of petroleum ether was obtained. The lower layer was then treated with ethyl acetate containing glacial acetic acid (10 mL/L). The extraction of polyphenol was carried out for 36 h at room temperature, and the combined ethyl acetate layer was concentrated. The residue was lyophilized and stored at −70°C. The total polyphenolic content and flavonoid of the extract were assayed using the standard methods. Total soluble phenolics in the PPE were determined with Folin-Ciocalteu reagent according to the standard method.Citation[16] Flavones and flavonols in polyphenolic extract (PPE) were expressed as quercetin equivalent.Citation[17] Flavanone in polyphenolic extract (PPE) was expressed as naringin equivalent.Citation[17]
Animals and Ethical Approval
Male and female Swiss albino rats, weighing about 200–250 g, were used in the present study. Animals were collected from the breeding colony and acclimatized to the laboratory condition for two weeks. They were housed in macrolon cages under standard laboratory conditions (light period 7:00 a.m. to 7:00 p.m., 21 ± 2°C, and relative humidity 55–70%). The animals were fed a commercial diet from Hindustan Lever Ltd. (Bangalore, India) and free access to water during the experiments. Experiments were performed that complied with the rulings of the Committee for the Purpose of Control and Supervision of Experiments on Animals (CPCSEA), New Delhi, India (Registration no.: 0367/01/C/CPCSEA), and the study was permitted by the institutional ethical committee of Jadavpur University.
Acute Toxicity Study
Acute oral toxicity study was performed as per OECD-423 guidelines (OECD 2002; acute toxic class method). Albino rats (n = 6) of both sexes selected by random sampling technique were used for acute toxicity study.Citation[18] The animals were kept fasting for overnight providing only water, after which the extracts were administered orally at the dose level 5 mg/kg body weight by gastric intubation and observed for 14 days. If mortality was observed in two out of three animals, then the dose administered was assigned as a toxic dose. If mortality was observed in one animal, then the same dose was repeated again to confirm the toxic dose. If mortality was not observed, the procedure was repeated for further higher doses such as 50, 300, and 2000 mg/kg body weight.
Intraperitoneal Glucose Tolerance Test (IPGTT)
After PPE administration (150 and 300 mg/kg), 20% D-glucose solution (2 g/kg body weight) was injected intraperitoneally to the animals (n = 6 for each experimental group) in the fasting state. Blood samples were obtained from the tail vein at 0, 15, 30, 60, and 120 min after the injection. Glucose measurement was made with Accu-check Touch Glucometer (Roche Diagnostics, Indianapolis, Indiana, USA).
Induction of Experimental Diabetic Nephropathy
All animal experiments were performed in accordance with the guidelines established by the Jadavpur University Committee for Animal Experimentation and the Committee for the Purpose of Control and Supervision of Experiments on Animals (CPCSEA), New Delhi, India. Diabetes was induced in eight-week-old male Wistar albino rats by administering an intraperitoneal injection of streptozotocin (50 mg/kg) dissolved in a 0.1 M citrate–phosphate buffer (pH 4.2). The rats were randomly divided into four groups of six animal each: control rats (A group), untreated diabetic rats (B group), and diabetic rats treated with polyphenolic extract at a daily dose of 150 and 300 mg/kg (C and D groups, respectively). Polyphenolic extract was dissolved in distilled water (total volume = 1 mL/kg). Two days after the injection of streptozotocin or vehicle, the induction of diabetes was confirmed by measuring urinary glucose with Diastix, reagent strips for the urinary glucose analysis (Bayer Diagnostics, Baroda, India). In every rat, polyphenolic extract or vehicle was received daily four weeks after the injection of streptozotocin. All groups were kept under identical conditions and had access to food and drinking water ad libitum. Eight weeks after the induction of diabetes, the rats were killed with an overdose of pentobarbital (60 mg i.p.). Blood samples were collected from the direct cardiac puncture, and the kidney was removed from each animal.
Plasma Analyses for Glycemia and Dyslipidemia
Glycemia was monitored by measurement of blood glucose. Blood glucose and body weights were assessed at two, four, six, and eight weeks after the induction of diabetes. Capillary blood samples were collected from the caudal vein after a daytime physiological fast to measure glycemia using commercial glucose strips kits as described earlier based on an enzymatic method (glucose oxidase). At the end of treatment period, animals were weighed and anesthetized with ether. Blood was obtained from cardiac puncture in the heparinized microhematocrit tubes, and the plasma was recovered by centrifugation at 4°C. Total plasma cholesterol and triglycerides, creatinine, total protein, and urea were measured using a commercially available reagent kit (Span Diagnostic Kit, Mumbai, India).
Biochemical Measurements in Urine
At the end of the study, each rat was weighed and placed in a metabolic cage for a 24 h urine collection. Albumin and protein concentrations were determined similarly in urine with Span Diagnostic kits (Mumbai, India). For urinary albumin and protein concentrations, rats were housed in metabolic cages for 24 h, and urine was collected in a collection beaker with several drops of toluene to inhibit microbial growth.
Assay of Renal Oxidative Stress
Preparation of Renal Homogenates
After blood sampling, the animals were sacrificed by cervical dislocation. Kidneys were excised, and then immediately frozen at −20°C until used for the estimation of GSH, MDA, CAT, and SOD levels. Immediately after rats were killed, the right/left kidney was dissected, rinsed with isotonic saline solution, dried, and weighed. After weighing, the kidney tissue was minced, and a homogenate was prepared with 10% (W/V) phosphate buffered saline (0.1 m/L, pH 7.4) using a homogenizer. The renal homogenate was used to estimate the malondialdehyde (MDA) and reduced glutathione (GSH). Post-mitochondrial supernatant was obtained by centrifugation at 10000 × g for 20 min at 4°C. This preparation was used further to estimate superoxide dismutase (SOD) and catalase (CAT).
Assay of Renal Lipid Peroxidation
Kidney cortex homogenates (10%, w/v) were mixed with an equal volume of ice cold 10% trichloroacetic acid (TCA). After centrifugation, a volume of the supernatant was added to an equal volume of 0.67% thiobarbituric acid (TBA), and the mixture was kept in a boiling water bath for 15 min. Samples were cooled to room temperature, and absorbance at 532 nm were recorded. The results were expressed as MDA equivalents using a molar extinction coefficient of 1.56 × 105M−1 cm−1.Citation[19]
Assay of Renal Glutathione
Reduced GSH in kidney was assayed by the method of Jollow et al.Citation[20] An aliquot of 1.0 ml of renal homogenate (10% w/v) was precipitated with 1.0 ml of sulphosalicylic acid (4% w/v). The samples were kept at 4°C for at least 1 h and then subjected to centrifugation at 1200 g for 15 min at 4°C. The assay mixture contained 0.1 ml filtered aliquot, 2.7 ml phosphate buffer (0.1 M, pH 7.4), and 0.2 ml DTNB (40 mg/10 ml of phosphate buffer 0.1 M, pH 7.4) in a total volume of 3.0 ml. The yellow color that developed was read immediately at 412 nm on a spectrophotometer.
Assay of Renal Superoxide Dismutase
Superoxide dismutase activity was assayed according to the method of Kono.Citation[21] The reduction of nitroblue tetrazolium (NBT), which was initiated by the superoxide dismutase, is measured at 560 nm. Briefly, the reaction was initiated by the addition of hydroxylamine hydrochloride to the reaction mixture containing NBT and kidney homogenate. The results were calculated as units/mg protein, where one unit of enzyme is defined as the amount of enzyme inhibiting the rate of reaction by 100%.
Assay of Renal Catalase
Catalase was assayed following AebiCitation[22] by the rate of decomposition for H2O2 at 240 nm. H2O2 (10 mM) was used as reagent, with the rate of dismutation of H2O2 to water and molecular oxygen being proportional to the concentration of catalase and with maximum absorbance at 240 nm.
Renal Histopathology
The right rat kidney was immediately fixed with 10% formalin. After fixation, the tissues were embedded in paraffin, and five micron-thick tissue sections were cut from the paraffin blocks. All of the kidney specimens were stained using hematoxylin and eosin, and each section was viewed under a light microscope at a magnification of ×10, 40–400.
Effect on Diabetic Neuropathy
Induction and Assessment of Diabetes
A single dose of 200 mg/kg STZ prepared in citrate buffer (pH 4.4, 0.1 M) was injected intraperitoneally to induce diabetes. The age-matched control mice received an equal volume of citrate buffer and used along with diabetic animals. Diabetes was confirmed after 48 h of STZ injection, the blood samples were collected through tail vein, and blood glucose levels were estimated by as described earlier. The mice having blood glucose levels more than 250 mg/dL were selected and used for the present study.Citation[11] Body weight and plasma glucose levels were also measured before and at the end of the experiment to see the effect of polyphenolic extract on these parameters.
Experimental Protocol
In the hot plate and tail immersion tests, pretreatment latencies were determined three times with an interval of 24 h starting three days before the injection of streptozotocin or saline, and the mean was calculated in order to obtain stable pre-drug response latency. On day 7 following the injection of streptozotocin, the polyphenolic extract given orally and the effect of their acute administration on nociception were determined at different time intervals (0, 30, 60 and 180 min). The mice were divided into the following groups:
control group, treated with saline;
group treated with streptozotocin (200 mg/kg, i.p., once);
group treated with streptozotocin + PPE (50 mg/kg, p.o.); and
group treated with streptozotocin + PPE (100 mg/kg, p.o).
Each group comprised six mice. A cutoff time of 10 s was used to prevent injury.
Assessment of Nociception
While nociception is defined as the normal electrophysiological response of peripheral sensory organs to noxious (tissue-damaging) stimuli, mediated by C and Ay nocireceptors, hyperalgesia is an exaggerated response to the same stimuli evoked by a hypersensitization of peripheral nociceptors and a central facilitation of pain transmission at the level of the dorsal horn neurones and thalamus. Pain was measured in the response of both healthy and diabetic mice to two different thermal stimuli.
Tail Immersion Test
The tails of mice were immersed in a warm water bath (52.5 ± 0.5°C) until tail withdrawal (flicking response) or signs of struggle were observed (cut-off 12 s). Shortening of the tail withdrawal time indicates hyperalgesia and is attributed to central mechanisms.Citation[23]
Hot Plate Test
The hyperalgesic response on the hot plate is considered to result from a combination of central and peripheral mechanisms.Citation[24] In this test, animals were individually placed on a hot plate (Eddy's hot plate) with the temperature adjusted to 55 ± 1°C. The latency to the first sign of paw licking or jump response to avoid the heat was taken as an index of the pain threshold; the cut-off time was 10 s in order to avoid damage to the paw.
Aldose Reductase Inhibitory Assay
Isolation Aldose Reductase Enzyme
Crude aldose reductase enzyme was prepared from rat lenses. The lenses were removed from the eyes of Wistar albino rats (150–200 g) and kept frozen at −20°C until the day of the experiment. The lenses were removed by lateral incision of the eye, washed with cold distilled water and kept cold. They were then deposited in a cold glass tube and then homogenized using a Teflon pestle in three volumes of cold 135 mM phosphate buffer (pH 7.0), containing 10 mM β- mercaptoethanol. The homogenate was centrifuged at 13,369 rpm (10 000 × g) for 15 min. The supernatant fluid was placed in a plastic 50 mL conical centrifuge tube, and the protein in this fluid was then precipitated by the addition, drop by drop, of a solution 75% (NH4)2SO4. The pellet obtained by centrifugation was dissolved in the same buffer and used as enzyme preparation. All of the procedures were carried out at 4°C. This solution containing enzyme was analyzed using the Biuret reaction. Its protein concentration was 4 mg/mL.
Enzyme Inhibitory Activity
The bioassay procedure with some modifications was followed according to Haraguchi et al.Citation[25] Bioassay solutions of plant extracts were prepared by dissolving 2 mg of the extract in 50 μL DMSO. After 30 min or more, a 1 mL volume was completed by adding phosphate buffer pH 6.2. The reaction mixture was prepared at 25°C, with a total volume of 1.8 mL, containing 50 mM Na-phosphate buffer (pH 6.2), 0.125 mM NADPH, 400 mM LiSO4, and enzyme preparation equivalent to 4 mg protein and 3 mM dl-glyceraldehyde as a substrate with or without plant extract. The reaction was initiated by the addition of NADPH and continued by 30 min. Absorbance readings were conducted at 340 nm absorption at the beginning and end of the reaction during this incubation time. NaHCO3 1M was added at the end of the 30 minute incubation period. Quercetin (3.3 × 10−1 to 33.3 mM), a known aldose reductase inhibitor, was used as positive control to compare the plant extracts inhibitory activity. A negative control was prepared using 5% DMSO in phosphate buffer (pH 6.2). The bioassays were run in triplicate, and the average inhibitory activities are shown on as percentages. At the end, the inhibitory activity of the extracts was calculated using the following formula:
Table 1 Aldose reductase inhibitory activity of polyphenolic extract of I. frutescens on rat isolated lens enzyme
Inhibition of in Vitro Albumin Glycation
The Albumin Glycation Reaction
One ml of 3000 mg/100mL glucose solution was added to 1 ml of 5 g/dL albumin solution. For the prevention of any environmental contamination, gentamicin with a concentration of 20 mg/100 ml was added to 0.01 mol/L phosphate buffer (pH 7.4) and incubated 72 hours in room temperature in constant position. Then, after the incubation period, it was dialyzed in phosphate buffer (the dialyzed bag was prepared in 10 mM/L EDTA before this process).Citation[26]
Measuring the Level of Albumin Glycation
For approving the albumin glycation, the TBA test was used, as follows. One ml 20% trichloro acetic acid (TCA) was added to above solution and then centrifuged for 10 minutes at 3000 rpm. The supernatant was discharged. This function was done twice. One ml phosphate buffer with above specification and 0.5 ml 0.3N oxalic acid were added to the sediment and put in boiling conditions in water bath. After the compound got cold in lab temperature, 0.5 ml 40% TCA was added to each sample. After centrifugation for 10 min at 3000 rpm, the supernatant was separated, 0.5 ml 5% M TBA was added to 1 ml of supernatant solution, and the whole solution was set in 40° water bath for half an hour. At the end, the absorbance of the sample was measured in 443 nm.Citation[27]
Preparing the Stock Solution of PPE
0.1 g PPE was dissolved in 10 ml double distilled water and used as PPE stock solution.
Determining the Effect of PPE on Albumin Glycation
Three different concentrations—1g/dL, 0.2 g/dL, and 0.1 g/dL—from PPE stock solution were prepared, and 0.1 ml from different concentrations of PPE was added to a solution having 5% albumin and 3000 mg/100ml glucose (in gentamicin phosphate buffer solution) and incubated in lab temperature for 72 hours. To determine the effect level of each concentration of PPE, TBA reaction was done as described previously. All of the experiment stages and each concentrations of PPE were done in triplicate, and for achieving acceptable results, the tests were repeated.
Statistical Analysis
The experimental data were expressed as mean ± S.E.M. The significance of difference among the various treated groups and control group were analyzed by means of one-way ANOVA followed by Dunnett's multiple comparison test using Graphad Instat Software (San Diego, California, USA). p < 0.05 and p < 0.01 were considered statistically significant. IC50 (inhibitor concentration that produces 50% inhibition) was estimated using linear regression method of plots of the percent inhibitory activity against the concentration of the tested compounds using Microsoft Excel.
RESULTS
Preliminary phytochemical screening of PPP was carried out for the detection of phytoconstituents, using standard chemical tests. Flavonoids, simple phenolic acids, anthocyanins, and tannins were detected in PPE. Total phenolic contents of PPE were expressed as mg of pyrocatechol equivalent per gram of dry weight of PPE extract. 1000 μg of PPE was used to determine the amount of total polyphenolic contents. The level of total polyphenolic compounds was 184.52 mg of pyrocatechol equivalent per gram of PPE. The present study showed the flavonoid content determined by two independent colorimetric methods, one for the determination of flavones and flavonols and other for the determination of flavanone, as reported previously. The contents of total flavonoids in the PPE of I. frutescens were expressed as the sum of two complementary methods for the determination of flavones, flavonols, and flavanone, and the results were found to be 36.42 mg of quercetin and naringin equivalent per gram of PPE. Major types of phenolic constituents identified in the leaves of I. frutescens are simple phenolic acids, flavonol, flavones, flavanone, and flavonoid glycosides.
Acute toxicity studies revealed the non-toxic nature of the polyphenolic extract of I. frutescens. After the administration of polyphenolic extract, rats were immediately observed for 2 h for behavioral, neurological, and autonomic profiles for any changes or lethality for the next 48 h. There was no lethality or any toxic reactions found at any of the doses selected until the end of the study period. However, at the above doses, PPE did not show any untoward effects on behavioral response, normal reflexes, etc. According to the Organization for Economic Co-operation and Development (OECD) guidelines for acute oral toxicity, an LD50 dose of 2000 mg/kg and greater is characterized as unclassified, and hence the drug is found to be safe.
To determine the effect of PPE on the function of pancreatic β-cells (insulin sensitivity) in Wistar albino rats, we carried out IPGTT by the administration of 2 g D-glucose/kg body weight. While the fasting blood levels in PPE-supplemented rats were not significantly different from those in control groups, the peak level of blood glucose in PPE-treated rats were significantly lower than glucose-loaded rats. The blood glucose levels at 120 min in PPE-treated rats were significantly lower than control rats (see ).
Figure 1. Effect of polyphenolic extract on intrperitoneal tolerance text (IPGTT) on glucose (2 g/kg) loaded rats. Values are expressed mean SEM ± of six animals. *P < 0.05, **P < 0.001 compared with glucose loaded rats.
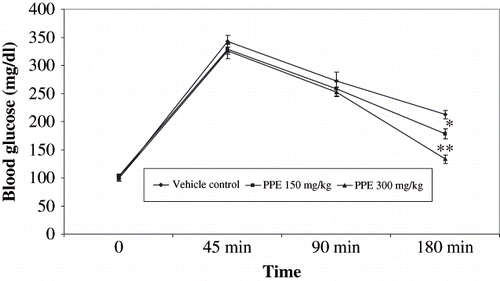
The body weight of the normal healthy rats increased during the eight-week experimental period (see ), while that of the control rats with diabetic nephropathy was lower than that of normal rats. The difference between the normal and diabetic nephropathy groups became more distinct as time progressed. There were no statistical differences at any time point between the body weight of the control and PPE-treated groups of rats with diabetic nephropathy. There was a significant decrease in rat kidney weight in the PPE-treated group compared to rats with diabetic nephropathy. shows blood glucose in all study groups. Diabetic rats had significantly elevated blood glucose compared to non diabetic rats. Blood glucose levels were significantly lowered by oral administration of PPE in diabetic rats. In diabetic rats given vehicle, serum cholesterol, triglycerides, and creatinine levels were increased in respect to controls (see ). PPE treatment significantly reduced hyperlipidemia. More remarkably, total cholesterol and triglycerides in the group given PPE accounted for values with in control range.
Table 2 Effect of oral administration of polyphenolic extract (PPE) of I. frutescens on serum biochemical and urinary profiles of vehicle-treated and streptozotocin (STZ)-induced diabetic nephropathic rats after the eight-week treatment
Figure 2. Body weight (g) in polyphenolic extract (PPE) treated diabetic rats. Values are expressed as mean ± SEM (n = 6). * * P < 0.01 with respect to theire initial values.
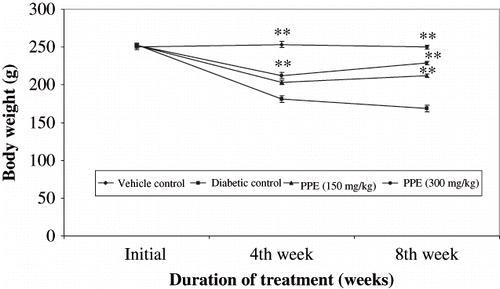
Figure 3. Effect of polyphenolic extract on blood glucose levels in normal and diabetic rats for 8 weeks. Data are mean of six rats for normal and experimental groups, respectively. **P < 0.01, *P < 0.05 compared with diabetic control of respective week.
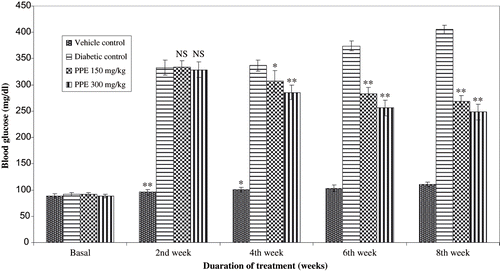
In the rats with diabetic nephropathy, mean values of proteinuria and albuminuria exceeded 86.01 mg/dL and 254.34 mg/dL, respectively, in all groups. Urinary microalbumin contents indicated glomerular damage. As shown in , the urinary microalbumin contents were reduced by increasing PPE supplementation. The administration of PPE maintained over time protein and albumin excretion at values comparable to vehicle group. Marked antiproteinuric effect with respect to treatment with PPE significantly differs from vehicle group. Renal functions, as also evaluated by serum creatinine levels, were progressively impaired in diabetic treated rats given vehicle (see ). In rats treated with PPE, mean value of serum creatinine was lower than in rats given vehicle.
The effect of PPE treatment on lipid peroxidation and antioxidant levels (GSH, SOD, and CAT) from normal, diabetic control and PPE-treated rats renal cortex are shown in and . TBARS were higher in the renal cortex of the non-treated diabetic healthy controls (see ). STZ diabetic rats with diabetic nephropathy had lower levels of renal antioxidants than healthy vehicle treated rats. PPE tended to increase renal antioxidant levels in diabetic rats, a difference that was statistically significant compared with rats of diabetic nephropathy.
Figure 4. Effect of polyphenolic extract (PPE) on renal antioxidant enzymes from normal and strptozotocin treated diabetic rats. Values are expressed as mean SEM of six animasl in each group. **P < 0.01 and *P < 0.05 compare with diabetic animals.
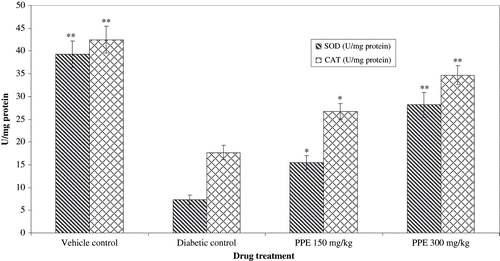
Figure 5. Effect of polyphenolic extract (PPE) on reanl reduced glutethione (GSH) from normal and diabetic rats. Values are expressed as mean ± SEM of six animals each. **P < 0.01 compared with diabetic control.
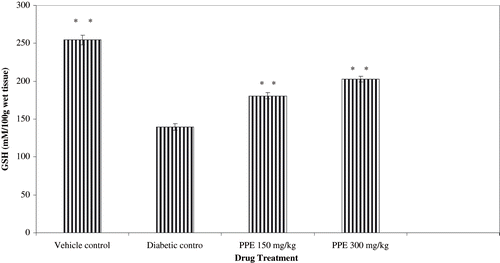
Figure 6. Effect of polyphenolic extract (PPE) on renal lipid peroxidation (LPO) from normal and diabetic rats. Values are expressed as mean ± SEM of six animals each. ** P < 0.01 compared with diabetic control.
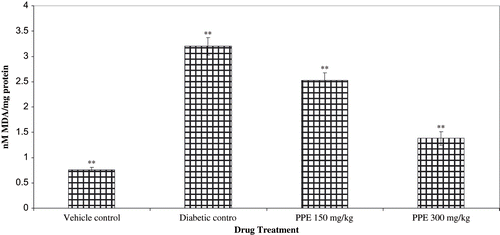
Histological examinations of the sections of kidney from normal control rat are shown in . The circular area observed on this photograph is renal glomeruli. Numerous tubules (proximal and distal) lie in the area adjacent to glomeruli. depicts the kidney section of STZ diabetic control rats that shows tubular vacuolations, glomerulosclerosis and irregular basement membrane. The incidence of hypertrophy, tubular vacuolations, and other derangements were much lower in STZ diabetic rats treated with polyphenolic extract (see ). shows the normal tubulointerstitial structure of vehicle treated rat kidneys. Interstitial damage, tubular hypertrophy, inflammatory cell infiltration, and thickening of tubular basement membrane were prominent in the STZ diabetic control rats (see and ). In contrast, polyphenolic extract-treated rat kidney showed improved structure of tubulointerstitial with minimal injury (see and ).
Figure 7. Light microscopes of the glomeruli. Paraffin-impeded sections of the renal cortex were stained with hematoxylin. Representative light microscope (magnification ×200) from each of the rat groups are shown: (A) Normal glomerulus from non-diabetic rat at eight weeks; (B) glomerulus from untreated STZ diabetic rats at eight weeks showing hypertrophy; irregular, denued glomerular basement membrane, mesangial expansion; and global glomerulosclerosis; with the symptoms of early diabetic nephropathy symptoms; (C) glomerulus from rats treated with eight weeks of PPE, depicting partial reversal of glomerulosclerosis.
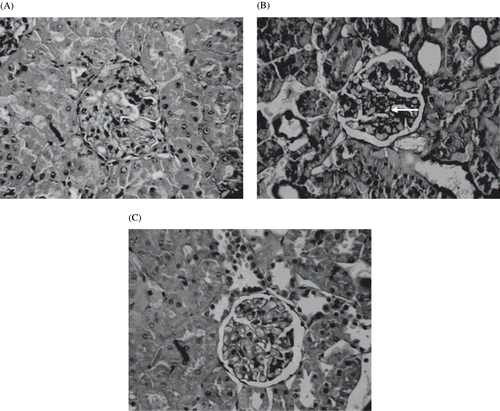
Figure 8. Panel D shows the normal tubulointerstitial structure of vehicle-treated rat kidneys. Panels E and F show prominent interstitial damage, tubular hypertrophy, inflammatory cell infiltration, and thickening of tubular basement membrane in the STZ diabetic control rats. Panels G and H from polyphenolic extract-treated rat kidney show improved structure of tubulointerstitial with minimal injury.
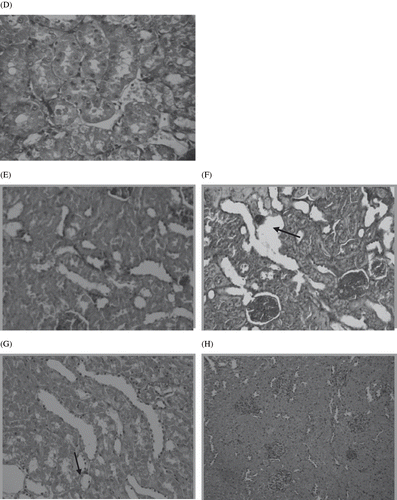
Diabetes was induced in mice by single high dose of streptozotocin (200 mg/kg, i.p.), and the nociception was assessed using hot plate and tail immersion one week following injection of STZ. The administration of PPE normalized the reaction time (s) of various thermal stimuli in diabetic rats (see and ). shows the percentage inhibition values of aldose reductase by PPE. Among three different concentrations, PPE (150 μg/mL) was found to be best inhibitory (63.43%) activity. In this research, the effects of various concentrations of PPE ion albumin glycation were studied. Three different concentration of PPE (62.75, 125, and 250 g/mL) and aminoguanidine (25 μg/mL) were used in the test, and by using the TBA method, the level of albumin glycation was determined. Percent inhibition was calculated to be 44.33%, 54.67%, and 63.33% for PPE at 62.75, 125, and 250 μg/mL, respectively; thus, PPE was comparable to aminoguanidine (66.67%), a known glycation inhibitor that traps reactive dicarbonyl impeding conversion to AGE. The results showed that the PPE had potential inhibitory effect on the albumin glycation reaction.
DISCUSSION
Diabetic nephropathy will soon represent the leading cause of end stage renal disease worldwide, with a consequent unbearable cost for patient healthcare systems even for developed countries.Citation[28],Citation[29] The need to reduce the incidence of diabetic nephropathy is urgent. Previous studies demonstrated that treatment with antioxidants partially reduced proteinuria and renal damage in unnephrectomized rats with overt diabetic nephropathy.Citation[30], Citation[, 31] Diabetic nephropathy is characterized by hypertrophy of glomerulus and tubules, basement membrane thickening of glomerulus and tubule, mesangial extra cellular matrix accumulation, and tubule interstitial fibrosis. Hypertrophy of epithelial cells and tubular basement membrane thickening have already occurred in early stage of diabetic nephropathy, and the extent of tubulointerstitial injury is associated closely with diabetic nephropathy.
Streptozotocin diabetic rats showed symptoms of renal nephropathy such as kidney hypertrophy that were attributed to an increase in blood glucose and polyuria. In addition, STZ diabetic rats showed elevated blood glucose, serum triglycerides, total cholesterol, urea, and creatinine, as demonstrated in previous reports.Citation[32], Citation[,33] Treatment with polyphenolic extract prevented renal enlargement and attenuated polyuria. Meanwhile, elevated blood glucose and serum biochemical parameters in STZ diabetic rats were also normalized to control values after eight weeks of treatment with PPE. This was consistent with other reports using natural antioxidants of plants to normalize diabetic nephropathy in STZ diabetic rats.Citation[34–36] Several plant extracts and their components per gram of extract were examined for their nephroprotective action against STZ-induced oxidative damage in diabetic rats.Citation[37],Citation[38] These data indicate that the renal protective effect of PPE might be due to high polyphenolic contents and antioxidant activity. PPE has 84.52 mg of polyphenol and 36.42 mg of total flavonoids, all of which protect against oxidative damage in diabetic kidney.
In diabetic nephropathy, the activation of neutrophils and monocytes causes the generation of ROS; the overproduction of ROS may cause an imbalance between oxidation and antioxidation in the body.Citation[39] Oxidative stress, recognized as a key component in the development of diabetic complications and increase in proportion to the AGEs accumulation, causes mitochondrial dysfunction.Citation[40] Other findings show that AGEs increase intracellular generation of ROS and decrease intracellular GSH levels in a time-dependent manner, and also that AGEs can augment the formation of ROS through the depletion of superoxide dismutase and other antioxidant enzymes.Citation[41] These suggest that ROS may be an important mediator of oxidative stress-induced renal damage in diabetic nephropathy. The intracellular generation of ROS suggests that there is a synergistic relationship between oxidative stress and high glucose. Oxidative stress-induced renal injury might be accelerated in hyperglycemia states. There may be agents that act to inhibit the generation, propagation, or activity of free radicals, such as preventive antioxidants, which include CAT, SOD, GSH-Px, polyphenol, and chain terminators such as vitamins E and C and glutathione.Citation[42],Citation[43] Therefore, the measurement of oxidative stress could be useful to investigate its role in the initiation and development of chronic diabetic complications, especially diabetic nephropathy, and also to evaluate preventive actions of exogenous and endogenous antioxidant therapy.
The mechanisms underlying the effects of PPE in diabetic nephropathy correlate by acting as an antioxidant, free radical scavenger, and inhibiter of lipid peroxidation.
In this study, we provide the first evidence that PPE can inhibit the ROS formation in a dose-dependent manner by increasing enzymatic and non-enzymatic antioxidants level in renal cortex. This oxidative stress was further assessed by the increase in renal MDA as a LPO marker.Citation[44] We observed an increased LPO when expressed TBARS showed in all diabetic groups as a result of oxidative stress. These suggest that the attenuation of oxidative stress induced by STZ through the scavenging of free radicals and inhibition of the oxidative stress may be one of the mechanisms underlying PPE in the prevention of diabetic nephropathy. Several studies reported that polyphenol could alleviate the decreased hepatic glutathione level in diabetic kidney. The overproduction of superoxide radicals by mitochondria activates the major pathways of hyperglycemia through the AGEs, and high glucose expression accelerates the oxidative stress in diabetic kidney. In STZ-induced treated rats, the activities of superoxide dismutase and catalase were notably elevated after the treatment with PPE. PPE, effective polyphenolic components of which are flavonoids (apigenin, quercetin, luteolin, and kaempferia) and phenolic acids (vanillic acid, protocatechuic acid, sinapic acid, and syringic acid), are known for their antioxidant and antidiabetic activity.Citation[45],Citation[46] Antioxidant therapy, especially polyphenolic extract of I. frutescens treatment, shows promise in preventing oxidative stress and renal damage in diabetic nephropathy. Further studies are necessary to establish the relationship between hepatic markers of oxidative stress and diabetic complications and elucidate whether treatment with polyphenolic extract reduces oxidative stress or reverses the adverse effects associated with diabetic nephropathy.
Diabetic neuropathy is the most common complication of diabetes mellitus with autonomic/peripheral neural components.Citation[47] Peripheral neuropathy may be either painful or painless. Diabetic neuropathy is associated with a decrease in nerve conduction velocity. Diabetes-induced oxidative stress and the generation of superoxide may be responsible in part for the development of vascular and neural complications.Citation[48] In our study, polyphenolic extract treatment prevented the diabetes-induced impairment in the hot plate and tail immersion reaction time. The decomposition products of STZ alter the cellular membrane proteins and thus initiate the autoimmune inflammatory process associated with the overproduction of cytokines, resulting in the destruction of neuronal cells. Our results showed that treated animals developed a well-defined hyperalgesia that was evaluated in two types of thermal noxious tests, the hot plate test and tail immersion test. The diabetes-induced hyperalgesia was abolished by the PPE. An alternative mechanism could be the inhibition of prostaglandin or other inflammatory mediators released from the neuronal cells by the activation of inflammatory mediators and oxidative stress. Although the exact mechanism of action of PPE is unclear, the anti-hyperalgesic effect of PPE seems to result from its inhibitory action on inflammatory mediators, which was consistent with our earlier reports on the anti-inflammatory activity of this plant.Citation[15]
Cataractogenesis is the most common complication that occurs in diabetes mellitus. Aldose reductase is a lens enzyme probably involved in the development of this eye problem.Citation[49] It is the first enzyme involved in the polyol pathway, which seems to play an important role in the development of degenerative complications of diabetes such as diabetic nephropathy, neuropathy, and retinopathy. Compounds inhibit aldose reductase activity, and therefore would be potential agents in the prevention of one of these complications. A large variety of structure diverse compounds have been identified to date as potent aldose reductase inhibitors. There have been numerous reports of aldose reductase inhibitors obtained from extracts, mostly plant materials. Flavonoids and related compounds are the most widely studied natural products family with aldose reductase inhibitory activity.Citation[50],Citation[51] Polyphenolic extract of I. frutescens were assessed for inhibitory activity against lens aldose reductase isolated from Wistar albino rats. Polyphenolic extract significantly inhibits the aldose reductase activity in vitro and is primarily caused by the presence of phenolic compounds and flavonoids in PPE. It has been well acknowledged that plant-derived extracts and polyphenolic compounds, especially flavonoids, are potential alternatives to synthetic inhibitors against aldose reductase activity.
Protein oxidative damage is directly involved in the pathogenesis of many diseases. Free radicals can produce protein mediators, which can cause the loss of various protein functions, such as enzyme activity, receptor affinity, and membrane transporters—each of which may result in biological dysfunctions.Citation[52] Proteins in the body are also modified by glucose through a glycation reaction. This reaction finally produces AGEs. The carbonyl carbons of reducing sugars non-enzymatically reacts with amino residues to form a Schiff base, a process known as glycation. This Schiff base rearrangement can form Amadori products, which are converted to AGEs through various processes. The formation of AGEs is known to cause diabetic complications such as nephropathy and retinopathy. In the presence of various concentration of PPE, albumin glycation was glycated and evaluated using the thiobarbituric acid method. The results showed that PPE significantly inhibited/and decreased the reaction of albumin glycation. PPE containing a number of antioxidant flavonoids and phenolic compounds may responsible for the inhibition of AGEs formation through the radical scavenging activity. Hence, formations of some kind of AGEs are thought to be due to free radical generation. Phenolic compounds known as free radical scavengers inhibit the formation of AGEs.Citation[53]
The aim of the study was to examine the involvement of oxidative stress in the progression of diabetic nephropathy and neuropathy in STZ diabetic animals and evaluate the potential of polyphenolic extract in the treatment of diabetes mellitus. Recently, a relationship between diabetic nephropathy and neuropathy and oxidative stress was reported, suggesting that oxidative stress enhances the progress of diabetic complications. Therefore, antioxidant treatment could ameliorate these complications. Based on the present preliminary results, we can conclude that PPE can be used as a therapeutic option in the treatment of diabetic complications such as kidney damage and neuropathic pain associated with diabetes mellitus. Further studies are needed to explore the possible mechanism of action of PPE in the treatment of diabetic complications.
ACKNOWLEDGMENT
The authors grateful to the All India Council of Technical Education (AICTE), New Delhi, Government of India, for providing financial support to carrying out this work.
REFERENCES
- Kikkawa R, Koya D, Haneda M. Progression of diabetic nephropathy. Am J Kidney Dis. 2003; 41: S19–S21
- Ha H, Kim KH. Role of oxidative stress in development of complications in diabetic nephropathy. Kidney Int. 1995; 48: S18–S21
- Remuzzi G, Schieppati A, Ruggenenti P. Nephropathy in patients with type 2 diabetes. N Engl J Med. 2002; 346: 1145–1151
- Lewis EJ, Hunsicker LG, Bain RP, Rohde RD. The effect of angiotensin enzyme inhibition on diabetic nephropathy. N Engl J Med. 1993; 329: 1456–1462
- Parving HH. The impact of hypertension and antihypertensive treatment on the course and prognosis of diabetic nephropathy. J Hypertens. 1990; 8: S187–S191
- Mogensen CE, Keane WF, Bennett PH, Jerums G, Parving HH, Passa P, Steffes MW, Striker GE, Viberti GC. Prevention of diabetic renal disease with special reference to micro-albuminuria. Lancet. 1995; 346: 1080–1084
- Masoro EJ, Iwasaki K, Gleiser CA, McMahan A, Seo EJ, Yu BP. Dietary modulation of the progression of nephropathy in aging rats: An evaluation of the importance of protein. Am J Clin Nutr. 1989; 49: 1217–1227
- Yokozawa T, Chung HY, He LQ, Oura H. Effectiveness of green tea tannin on rats with chronic renal failure. Biosci Biotech Biochem. 1996; 60: 1000–1005
- Bravo L. Polyphenols: Chemistry, dietary sources, metabolism and nutritional significance. Nutr Rev. 1998; 56: 317–333
- Yaqoob M, McCleeland P, Patrick AW, Syevenson A, Mason H, White MC, Bell GM. Evidence of oxidant injury and tubular damage in early diabetic nephropathy. QJM. 1994; 87: 601–607
- Anjaneyulu M, Chopra K. Quercetin, an anti-oxidant bioflavonoid, attenuates diabetic nephropathy in rats. Clin Exper Pharmacol Physiol. 2004; 31: 244–248
- Rhee SJ, Kim NJ, Kwag OG. Effects of green tea catechin on prostaglandin synthesis of renal glomerular and renal dysfunction in streptozotocin-induced diabetic rats. Asia Pacific J Clin Nutr. 2002; 11: 232–236
- Renu A, Saiyada NA, Odenbach S. Effect of reinstitution of good metabolic control on oxidative stress in kidney of diabetic rats. J Diabetes Compl. 2004; 5: 282–288
- Bhandary MJ, Chandrashekar KR, Kaveriappa KM. Medical ethno-botany of the siddis of Uttara Kannada District, Karnataka, India. J Ethnopharmacol. 1995; 47: 149–158
- Kumarappan CT, Chandra R, Mandal SC. Anti-inflammatory activity of Ichnocarpus frutescens. Pharmacologyonline. 2006; 3: 201–216
- Singleton VL, Orthofer RM, Ramuela-Raventos RM. Analysis of total phenols and antioxidants and other substrates by means of Folin-Ciocalteu reagent. Methods Enzymol. 1999; 29: 152–178
- Chang CC, Yang MH, Wen HM, Chern JC. Estimation of total flavonoid content in propolis by two complementary colorimetric methods. J Food Drug Anal. 2002; 10: 178–182
- Organization for Economic Co-operation and Development. Section 4: Health Effects Test No. 423: Acute Oral Toxicity—Acute Toxic Class Method. OECD Guidelines for the Testing of Chemicals. July, 1997; 1(4)1–14
- Ohkawa H, Ohishi N, Yagi K. Assay for lipid peroxidation in animal tissues by thiobarbituric acid reaction. Anal Biochem. 1979; 44: 276–278
- Jollow DJ, Mitchell JR, Zampaglione N, Gillete JR. Bromobenzene induced liver necrosis: Protective role of glutathione and evidence for 3,4 bromobenzeneoxide as the hepatotoxic intermediate. Pharmacology. 1974; 11: 151–169
- Kakkar P, Das B, Viswanathan PN. A modified spectrophotometric assay of superoxide dismutase. Indian J Biochem Biophys. 1984; 21: 130–132
- Aebi H. Catalase in vitro. Methods Enzymol. 1984; 34: 479–500
- Ramabadran K, Bansinath M, Turndorf H, Puig MM. The hyperalgesic effect of naloxone is attenuated in streptozotocin-induced diabetic mice. Psychopharmacology. 1989; 97: 169–174
- Kanaan SA, Saade NE, Haddad JJ, Abdelnoor AM, Atweh SF, Jabburet SJ, Garabelian BS. Endotoxin induced local inflammation and hyperalgesia in rats and mice: a new model for inflammatory pain. Pharmacology. 2003; 66: 373–379
- Haraguchi H, Ohmi I, Masuda H, Tamura Y, Mizutani K, Tanaka O, Chou WH. Inhibition of aldose reductase by dihydroflavonols in Engelhardtia chrysolepsis and effects on other enzymes. Experiencia. 1997; 52: 564–567
- Dolhfer R, Wieland OH. Improvement of the thiobarbituric acid assay for serum glycosylprotein determination. Clin Chim Acta. 1981; 112: 197–204
- Work TS, Work E. Laboratory Techniques in Biochemistry and Molecular Biology. Appleton and Lange, Amsterdam 1969; 423–428
- Hostetter T. Prevention of end-stage renal disease due to type 2 diabetes. N Engl J Med. 2001; 345: 910–911
- King H, Aubert R, Herman W. Global burden of diabetes, 1995–2025: Prevalence, numerical estimates, and projection. Diabetes Care. 1998; 21: 1414–1431
- Montilla P, Barcos M, Munoz MC, Bujalance I, Munoz-Castaneda JR, Tunez I. Red wine prevents brain oxidative stress and nephropathy in streptozotocin-induced diabetic rats. J Biochem Mol Biol. 2005; 38: 539–544
- Afshari AT, Shirpoor A, Farshid A, Saadatian R, Rasmi Y, Saboory E, Ilkhanizadeh B, Allameh A. The effect of ginger on diabetic nephropathy, plasma antioxidant capacity and lipid peroxidation in rats. Food Chem. 2007; 101: 148–153
- Grover JK, Yadav SP, Vats V. Effect of feeding Murraya koeingii and Brassica juncea diet kidney functions and glucose levels in streptozotocin diabetic mice. J Ethnopharmacol. 2003; 85: 1–5
- Yanardag R, Bolkent S, Karabulut-Bulan O, Tunali S. Effects of vanadyl sulfate on kidney in experimental diabetes. Biol Trace Elem Res. 2003; 95: 73–85
- Yildirim O, Buyukbingol Z. In vivo effect of vitamin C with cobalt on oxidative stress in experimental diabetic rat kidney. Diabetes Nutr Metab. 2003; 16: 208–213
- Orrosova IG, Fathallah L, Liu E, Nourooz-Zadeh J. Early oxidative stress in the diabetic kidney effect of DL-lipoic acid. Free Rad Bio Med. 2003; 34: 186–195
- Yamabe N, Yokozawa T, Oya T, Kim M. Therapeutic potential of (-)-epigallocatechin 3-O-gallate on renal damage in diabetic nephropathy model rats. J Pharmacol Exp Ther. 2006; 319: 228–236
- Tedong L, Dimo T, Dzeufiet PDD, Asongalem AE, Sokeng DS, Callard P, Flejou JF, Kamtchouing P. Anti-hyperglycemic and renal protective activities of Anacardium occidentale (anacardiaceae) leaves in streptozotocin-induced diabetic rats. Afr J Trad CAM. 2006; 3: 23–35
- Yanardag R, Bolkent S, Ozsoy-Sac O, Karabulut-Bulan O. The effects of chard (Beta vulgaris L. var. cicla) extract on the kidney tissue, serum urea and creatinine levels of diabetic rats. Phytother Res. 2002; 16: 758–761
- Brownlee M. Biochemistry and molecular cell biology of diabetic complications. Nature. 2001; 414: 813–820
- Wautier MP, Chappey O, Corda S, Stern DM, Schmidt AM, Wautier JL. Activation of NADPH oxidase by AGE links oxidant stress to altered gene expression via RAGE. Am J Physiol Endocrinol Metab. 2001; 280: E685–E694
- Yan H, Li X, Xie J, Li M. Effects of advanced glycation end products on renal fibrosis and oxidative stress in cultured NRK-49F cells. Chin Med J. 2007; 120: 787–79
- Boran M, Kucukaksu C, Balk M, Cetin S. Red cell lipid peroxidation and antioxidant system in hemodialysed patients: Influence of recombinant human erythropoietin (r- HuEPO) treatment. Int Urol Nephrol. 1998; 30: 507–512
- Du Thie G, Crozier A. Plant derived polyphenolic antioxidants. Curr Opin Nutr Metab. 2000; 3: 447–451
- Altomare E, Vendemiale G, Chicco D, Procacci V, Cirelli F. Increased lipid peroxidation in type 2 poorly controlled diabetic patients. Diabetes Metab. 1992; 18: 264–271
- Zarzuelo A, Jimenez L, Gomez MJ, Utrilla P, Fernaddez I, Torres MI, Osuna I. Effects of luteolin 5-O-β-rutinoside in STZ-induced diabetic rats. Life Sci. 1996; 58: 2311–2316
- Masella R, Catafora A, Modesti D, Cardilli A, Gennavo L, Bocca A, Coni E. Antioxidant activity 3,4-DHPEA-EA and protocatechuic acid: A comparative assessment with other olive biophenols. Redox Rep. 1999; 4: 113–121
- Ward JD. Diabetic neuropathies. Current concepts in prevention and treatment. Drugs. 1986; 32: 279–289
- Coopey LJ, Gellett JS, Davidson EP, Dunlap JA, Lund DD, Yorec MA. Effect of antioxidant treatment of streptozotocin-induced diabetic rats in endoneurial blood flow, motor nerve conduction velocity, and vascular reactivity of epineurial arterioles of the sciatic nerve. Diabetes. 2001; 50: 1927–1937
- Kinoshita JH, Datiles MB, Kador PF, Robison WG. Aldose reductase and diabetic eye complications. Diabetes Mellitus Theory and Practice., H Rifkin, D Porte. Elsevier, New York 1990; 264–278
- Sawada H, Hamatake M, Hara A, Nakagawa M, Nakayama T. Inhibition of human placenta aldose reductase by tannic acid. Chem Pharm Bull. 1989; 37: 1662–1664
- Haraguchi H, Ohmi I, Fukuda A, Tamura Y, Mizutani K, Tanaka O, Chou WH. Inhibition of aldose reductase and sorbitol accumulation by astibin and taxifolin dihydroflavonols in Engelhardtia chrysolepsis. Biosci Biotechnol Biochem. 1997; 61: 651–654
- Ulrich P, Cerami A. Protein glycation, diabetes, and aging. Recent Prog Horm Res. 2001; 56: 1–21
- Nagaraj RH, Sarkar P, Mally A, Biemel KM, Lederer MO, Padayatti PS. Effect of pyridoxamine on chemical modification of proteins by carbonyls in diabetic rats: Characterization of a major product from the reaction of pyridoxamine and methylglyoxal. Arch Biochem Biophys. 2002; 402: 110–119