Abstract
Diabetic nephropathy is a serious microvascular complication and one of the main causes of end-stage renal disease. L-Glutamine (LG) is naturally occurring amino acids with antidiabetic and antioxidant potential. The aim of present investigation was to evaluate the potential of LG against streptozotocin (STZ)-induced diabetic nephropathy (DN) in laboratory rats. DN was induced in male Wistar rats (200–220 g) by intraperitoneal administration of STZ (55 mg/kg). Animals were treated orally with either distilled water (10 mg/kg) or LG (250, 500, and 1000 mg/kg) or Sitagliptin (5 mg/kg). Various biochemical, molecular, and histological (hematoxylin–eosin and Masson’s trichrome stain) parameters were assessed. Administration of LG (500 and 1000 mg/kg) significantly inhibited (p < .05) STZ-induced alterations in serum and urine biochemistry (urine creatinine, uric acid, albumin, and BUN). It also significantly increased creatinine clearance rate. STZ induced increase in renal oxidonitrosative stress was significantly decreased (p < .05) by LG (500 and 1000 mg/kg) treatment. Upregulated renal KIM-1, NGAL, TGF-β1, and collagen-1 mRNA expression after STZ administration was significantly inhibited (p < .05) by LG (500 and 1000 mg/kg) treatment. Correlation analysis also revealed that antidiabetic potential of LG attenuates STZ-induced elevated renal KIM-1, NGAL, TGF-β1, and collagen-1 mRNA expression. Histopathological alteration induced by STZ in renal tissue was ameliorated by LG treatment. In conclusion, results of present investigation suggest that treatment with LG ameliorated STZ-induced DN via the inhibition of oxidonitrosative stress as well as downregulation of KIM-1, NGAL, TGF-β1, and collagen-1 mRNA expressions.
Introduction
Diabetic nephropathy (DN) is one of the most serious microvascular complication of type 1 and type 2 diabetes as well as it is one of the main causes of end-stage renal failure and death in diabetic patients.Citation1,Citation2 Evidence suggest that more than 30% of all diabetic patients develop DN within 10–20 years after the onset of diabetes.Citation3 In diabetic patients, the incidence of development of microalbuminuria is 20–40% if DN remains untreated. Moreover, within 20–25 years almost 20% of these patients will develop end-stage renal failureCitation4 and which further requires renal transplantation or chronic hemodialysis.Citation5
The pathogenesis of DN includes hyperglycemia-induced overproduction of free radicals that further activates all pathways involved in the pathogenesis of complications of diabetes.Citation1,Citation2 Therefore, elevated oxidative stress played a vital role in induction and maintenance of DN.Citation6 It has been reported that increased blood sugar level caused overproduction of hydrogen peroxide that in turn elevated lipid peroxidation.Citation7 It is also responsible for the glycosylation of proteins in blood and tissues, which leads to the production of advanced glycosylated end products (AGEs).Citation8,Citation9 Although, factors responsible for DN is not clear, it is argued that increased production of reactive oxygen species, renal polyol formation, advanced glycated end-products accumulation, and elevated level of transforming growth factor beta-1 (TGF-β1) contributes to increased renal albumin permeability and extracellular matrix (ECM) accumulation that leads to elevated proteinuria, glomerulosclerosis, and tubulointerstitial fibrosis.Citation10,Citation11
Available management options for DN are targeted toward glycemic control, but still most of the patients with diabetes prone to developing kidney disease. Consequently, there is an urgent need to identify additional therapeutic strategies to attenuate the progression of DN in this population. The streptozotocin (STZ)-induced diabetes is well established, widely used, highly reproducible animal model to study diabetic complications including nephropathy, retinopathy, and neuropathy.Citation12,Citation13 A single intraperitoneal (ip) administration of STZ leads to the development of DN, which is characterized by changes in both glomerular and tubular structure and function.Citation14,Citation15 This model mimics almost all pathobiological features of clinical DN including activation of protein kinase C,Citation16 deposition of AGEs,Citation17 mesangial cell activation, and podocyte injury.Citation12 Thus, STZ-induced DN animal model plays a vital role in the development of therapeutic moieties with antidiabetic potential.
Studies have documented the importance of nonessential amino acids in the management and treatments of various diseases. Glutamine is one of the 20 naturally occurring amino acids in dietary protein, specifically it is a conditionally essential amino acid. It is found very high in dietary meats, eggs, whey protein, and casein protein. Under normal conditions, glutamine serves as a main energy metabolism fuel for intestinal and renal enterocytes. Studies have validated the effectiveness of glutamine supplementation in the reduction of intestinal injuries and improving systemic cell immunity.Citation18,Citation19 It is an important precursor for the synthesis of glutathione, which is a non-enzymatic antioxidant. It also plays a vital role in glutathione metabolism as well as maintenance of the cellular redox potential during elevated cellular oxidative stress.Citation20 Accumulating biochemical and clinical evidence support the phenomenon where amino acids exert its antioxidant effects to attenuate oxidative stress-mediated disorders.Citation21,Citation22 Furthermore, administration of certain amino acids shown to attenuates DN in experimental animals.Citation23–25 Literature lucid with evidence showed that L-glutamine (LG) possesses potent antiulcer, antioxidant, anti-bacterial, cardioprotective, anticancer, hepatoprotective, and anti-apoptotic potential.Citation26–29 It has been reported that LG significantly attenuated cisplatin-induced genotoxicity.Citation30 However, its role in DN is yet to unravel. Hence, the aim of the present investigation was to evaluate the potential of LG against STZ-induced DN by assessing various biochemical, molecular, and histological changes in laboratory rats.
Materials and methods
Drugs and chemicals
LG (≥99%) and Streptozocin (97%) were purchased from Sigma-Aldrich Co., St Louis, MO. 1,1′,3,3′-Tetraethoxypropane, crystalline beef liver catalase, 5,5′-dithiobis (2-nitrobenzoic acid) were purchased from SD Fine Chemicals, Mumbai, India. Sulfanilamides, naphthylamine diamine HCl, and phosphoric acid were obtained from Loba Chemie Pvt. Ltd., Mumbai, India. Creatinine and BUN kits were purchased from Accurex Biomedical Pvt. Ltd., Mumbai, India. Total RNA Extraction kit and One-step RT-PCR kit was purchased from MP Biomedicals India Private Limited, India.
Experimental animals
Adult male Wistar rats (200–220 g) were purchased from the National Institute of Biosciences, Pune (India). They were maintained at 24 °C ± 1 °C with a relative humidity of 45–55% and 12:12 h dark/Light cycle. The animals had free access to standard pellet chow (Nutrivet Life Sciences, Manikbag, Sinhagad Road, Pune) and water throughout the experimental protocol. All experiments were carried out between 09:00 and 17:00 h. The experimental protocol (DYPCOP/IAEC/2016/2) was approved by the Institutional Animal Ethics Committee (IAEC) of Pad. Dr D. Y. Patil College of Pharmacy, Akurdi, Pune and performed in accordance with the guidelines of Committee for Control and Supervision of Experimentation on Animals (CPCSEA), Government of India on animal experimentation.
Induction and assessment of diabetes
A single dose of 55 mg/kg streptozotocin (STZ) prepared in citrate buffer (pH 4.4, 0.1 M) was injected intraperitoneally to induce diabetes.Citation31 The age‐matched control rats received an equal volume of citrate buffer and were used along with diabetic animals. Diabetes was confirmed 48 h after streptozotocin injection; the blood samples were collected via retro-orbital plexus technique using heparinized capillary glass tubes, and plasma glucose levels were estimated by the enzymatic GOD‐POD (glucose oxidase-peroxidase) diagnostic kit method. The rats having plasma glucose levels more than 250 mg/dL were selected and used for the present study. The body weight and plasma glucose levels were measured before and at the end of the experiment. Food intake and water intake were measured with by placing the animals in a metabolic cage (Metabolic cage, Biomedical, India) before and at the end of the experiment.
Experimental design
After a basal recording of nociceptive reaction at week four after streptozotocin injection, the control and diabetic rats were randomly selected and divided into seven groups of 8–10 animals each as follows:
(A) Non-diabetic animals
Group I: Normal non-diabetic (N): animals received a single injection of citrate buffer (vehicle) and oral gavage of double distilled water (10 mg/kg).
Group II: Per se: animals received LG (1000 mg/kg) in double distilled water by oral gavage.
(B) Diabetic animals
Group I: Diabetic control (DC): Diabetic animals received double-distilled water by oral gavage (10 mg/kg).
Group II: Diabetic (STZ) + Sita (5): Diabetic animals received Sitagliptin (5 mg/kg) in double-distilled water by oral gavage.
Group III: Diabetic (STZ) + LG (250): Diabetic animals received LG (250 mg/kg) in double-distilled water by oral gavage.
Group IV: Diabetic (STZ) + LG (500): Diabetic animals received LG (500 mg/kg) in double-distilled water by oral gavage.
Group V: Diabetic (STZ) + LG (1000): Diabetic animals received LG (1000 mg/kg) in double-distilled water by oral gavage.
Freshly prepared LG was administered daily in three different dosages (250, 500, and 1000 mg/kg).Citation32 The dose of Sitagliptin (5 mg/kg) was selected based on the previous study.Citation33 At the end of the study, whole blood samples were collected from retro-orbital plexus to obtain serum for renal function parameters. Body weights and kidney weights of all animals were recorded, and animals were sacrificed by cervical dislocation. Kidney tissues were harvested, fatty and conjunctive tissue layer were removed, rinsed in normal saline and stored in −80 °C freezer for further biochemicals and RT-PCR studies. A kidney of rat from each group was isolated and fixed in 10% formalin solution for histopathological examination.
Serum and urine biochemistry
The serum was separated by centrifugation using an Eppendorf Cytocentrifuge, maintained at 4 °C and run at a speed of 7000 rpm for 15 min. Serum as well as urine creatinine, uric acid, albumin, and BUN were measured by a spectrophotometer using reagent kits according to the procedure provided by the manufacturer (Accurex Biomedical Pvt. Ltd., Mumbai, India).
Biochemical estimation
Kidney tissue homogenate preparation, antioxidants, lipid peroxidation (MDA), NO, and hydroxyproline estimation
A known weight of the kidney tissue homogenates was prepared with 0.1 M tris-HCl buffer (pH 7.4), and supernatant of homogenates was employed to estimate superoxide dismutase (SOD), reduced glutathione (GSH), lipid peroxidation (MDA), nitric oxide (NO), and hydroxyproline level as described previously.Citation34–41
Determination of KIM-1, NGAL, TGF-β, and collagen-1 by reverse transcriptase-PCR in kidney
The levels of mRNA were analyzed in renal tissue using a reverse transcription (RT)-PCR approach as described previously.Citation34,Citation42–44 Briefly, single-stranded cDNA was synthesized from 5 μg of total cellular RNA using reverse transcriptase (MP Biomedicals India Private Limited, India) as described previously.Citation34 Amplification of β-actin served as a control for sample loading and integrity. The primer sequences for KIM-1, NGAL, TGF-β, collagen-1, and β-actin were selected according to the previously reported method.Citation45 () PCR products were detected by electrophoresis on a 1.5% agarose gel containing ethidium bromide. The size of amplicons was confirmed using a 100-bp ladder as a standard size marker. The amplicons were visualized, and images were captured using a gel documentation system (Alpha Innotech Inc., San Leandro, CA). Gene expression was assessed by generating densitometry data for band intensities in different sets of experiments, by analyzing the gel images on the Image J program (Version 1.33, Wayne Rasband, National Institutes of Health, Bethesda, MD) semi-quantitatively. The band intensities were compared with constitutively expressed β-actin. The intensity of mRNAs was standardized against that of the β-actin mRNA from each sample, and the results were expressed as PCR-product/β-actin mRNA ratio.
Table 1. Primer sequences for KIM-1, NGAL, TGF-β, collagen-1, and β-actin.
Histological examination
The dissected kidney tissue specimens were fixed in 10% formaldehyde, processed routinely for embedding in paraffin. Sections were stained with hematoxylin–eosin stain and Masson’s trichrome stain as described previously.Citation46 Kidney sections were analyzed qualitatively under a light microscope (40× and 100×) for various histopathological alterations.
Statistical analysis
Data were expressed as mean ± standard error mean (SEM). Data analysis was performed using Graph Pad Prism 5.0 software (Graph Pad, San Diego, CA). Data of biochemical parameters were analyzed using one-way analysis of variance (ANOVA), and Tukey’s multiple range test was applied for post hoc analysis. A value of p < .05 was considered to be statistically significant.
Results
Effect of L-glutamine on STZ-induced alterations in body weight and relative kidney weight in diabetic rats
There was significant decrease (p < .05) in body weight and significant increase (p < .05) in relative kidney weight of STZ control rats as compared to normal rats. These STZ-induced decrease in body weight was significantly halted by LG (500 and 1000 mg/kg) administration. Whereas, relative kidney weight was decrease significantly (p < .05) after LG (500 and 1000 mg/kg) treatment as compared to STZ control rats. Sitagliptin (5 mg/kg) treatment also significantly inhibited (p < .05) STZ induced an alteration in body weight and relative kidney weight as compared to STZ control rats. However, increase in relative kidney weight was more significantly attenuated (p < .05) by LG (1000 mg/kg) and sitagliptin (5 mg/kg) administration than LG (500 mg/kg) ().
Table 2. Effect of LG on STZ-induced alterations in body weight, blood glucose level, food intake, water intake, urine output, and relative kidney weight in diabetic rats.
Effect of L-glutamine on STZ-induced alterations in blood glucose level, food intake, water intake, and urine output in diabetic rats
There was a significant increase (p < .05) in plasma glucose level as well as food intake, water intake, and urine output in STZ control rats as compared to normal rats after four weeks of intraparietal STZ administration. These increased plasma glucose level, food intake, water intake, and urine output were significantly inhibited (p < .05) by LG (500 and 1000 mg/kg) treatment as compared to STZ control rats ().
Effect of L-glutamine on STZ induced alterations in serum and urine biochemical parameters in diabetic rats
When compared with normal rats, intraperitoneal administration of STZ caused significant elevated (p < .05) in serum BUN, creatinine, and uric acid levels in STZ control rats. Administration of LG (500 and 1000 mg/kg) significantly reduced (p < .05) these elevated levels of serum BUN, creatinine, and uric acid as compared to STZ control rats. Sitagliptin (5 mg/kg) treatment also showed a significant reduction (p < .05) in serum BUN, creatinine, and uric acid as compared to STZ control rats ().
Table 3. Effect of LG on STZ-induced alterations in serum and urine biochemical parameters in diabetic rats.
Effect of L-glutamine on STZ-induced alterations in creatinine clearance in diabetic rats
There was significant decreased (p < .05) in creatinine clearance rate in STZ control rats as compared to normal rats after four weeks of post-STZ administration. Whereas, treatment with LG (500 and 1000 mg/kg) significantly attenuated (p < .05) STZ induced an alteration in creatinine clearance rate as compared to STZ control rats. Administration of sitagliptin (5 mg/kg) also significantly increase (p < .05) the rate of creatinine clearance as compared to STZ control rats ().
Effect of L-glutamine on STZ induced alterations in renal SOD and GSH levels in diabetic rats
Intraperitoneal administration of STZ caused significant decrease (p < .05) in renal SOD and GSH levels in STZ control rats as compared to normal rats. Administration of LG (500 and 1000 mg/kg) significantly increased (p < .05) renal SOD and GSH levels as compared to STZ control rats. When compared with STZ control rats, sitagliptin (5 mg/kg) treatment also showed significant (p < .05) inhibition in STZ-induced decreased renal SOD and GSH levels ().
Table 4. Effect of LG on STZ-induced alterations in renal oxidonitrosative stress and hydroxyproline level in diabetic rats.
Effect of L-glutamine on STZ induced alterations in renal MDA and NO levels in diabetic rats
There was a significant increase (p < .05) in renal MDA and NO levels in STZ control rats as compared to normal rats after four weeks of post-STZ administration. Whereas, treatment with LG (500 and 1000 mg/kg) significantly inhibited (p < .05) STZ induced increased in renal MDA and NO levels as compared to STZ control rats. Administration of sitagliptin (5 mg/kg) also significantly decreased (p < .05) renal MDA and NO levels as compared to STZ control rats ().
Effect of L-glutamine on STZ induced alterations in renal hydroxyproline level in diabetic rats
When compared with normal rats, intraperitoneal administration of STZ caused a significant increase (p < .05) in renal hydroxyproline level in STZ control rats. Administration of LG (500 and 1000 mg/kg) significantly reduced (p < .05) these elevated levels of renal hydroxyproline as compared to STZ control rats. Sitagliptin (5 mg/kg) treatment also showed a significant reduction (p < .05) in renal hydroxyproline level as compared to STZ control rats ().
Effect of L-glutamine on STZ induced alterations in renal KIM-1, NGAL, TGF-β1, and collagen-1 mRNA expressions in diabetic rats
Intraperitoneal administration of STZ caused significant upregulation (p < .05) in renal KIM-1, NGAL, TGF-β1, and collagen-1 mRNA expressions in STZ control rats as compared to normal rats. Administration of LG (500 and 1000 mg/kg) significantly inhibited (p < .05) STZ-induced upregulated renal KIM-1, NGAL, TGF-β1, and collagen-1 mRNA expressions as compared to STZ control rats. When compared with STZ control rats, sitagliptin (5 mg/kg) treatment also showed significant downregulation (p < .05) in KIM-1, NGAL, TGF-β1, and collagen-1 mRNA expressions ().
Figure 1. (A) Effect of LG on STZ-induced alterations in renal KIM-1, NGAL, TGF-β, and Collagen-1 mRNA expression in diabetic rats. (B) Quantitative representation of the mRNA expression of KIM-1, (C) NGAL, (D) TGF-β, and (E) Collagen-1. Results are represented as mean ± SEM, (n = 4) data were analyzed by One-way ANOVA followed by Tukey’s multiple range test for each parameter separately. #p < .05 as compared with normal group, *p < .05 as compared with diabetic control (DC) group and $p < .05 as compared with each other. N: normal group; DC: diabetic control group; Sita (5): sitagliptin (5 mg/kg, p.o.)-treated group; LG (250): L-glutamine (250 mg/kg, p.o.)-treated group; LG (500): L-glutamine (500 mg/kg, p.o.)-treated group; LG (1000): L-glutamine (1000 mg/kg, p.o.)-treated group. Lane 1: Ladder 1000 bp; Lane 2: mRNA expression of normal group; Lane 3: mRNA expression of diabetic control group; Lane 4: mRNA expression of sitagliptin (5 mg/kg, p.o.) treated group; Lane 5: mRNA expression of L-glutamine (250 mg/kg, p.o.) treated group; Lane 5: mRNA expression of L-glutamine (500 mg/kg, p.o.) treated group; Lane 7: mRNA expression of L-glutamine (1000 mg/kg, p.o.) treated group and Lane 8: mRNA expression of per se group.
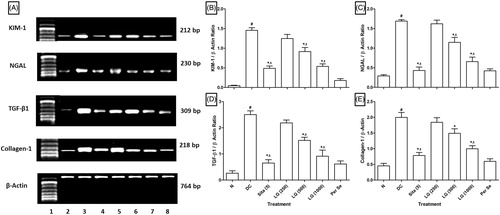
Correlation analyses of blood glucose level and KIM-1, NGAL, TGF-β1 as well as collagen-1 mRNA expressions in diabetic rats
Correlation coefficient by linear regression analyses was carried out between various parameters. Direct correlation was observed between blood glucose level and renal KIM-1, NGAL, TGF-β1 as well as collagen-1 mRNA expressions indicating that increased dose of LG significantly inhibited blood glucose as well as downregulated renal KIM-1 (r = 0.878523, p < .05), NGAL (r = 0.86497, p < .05), TGF-β1 (r = 0.919845, p < .05) and collagen-1 (r = 0.856981, p < .05) mRNA expression. Thus, all renal marker tested with respect to kidney showed high degree of correlation ().
Effect of L-glutamine on STZ-induced alterations in renal histopathology in diabetic rats
Renal tissue from normal rats showed the well-organized architecture of renal histology. It showed normal glomerulus surrounded by the Bowman’s capsule, proximal, and distal convoluted tubules without any inflammatory infiltration, fibrosis, and necrosis ( and ). Whereas, different grades of various pathological lesions were observed in the renal tissue of diabetic rats (). It showed glomerular necrosis with distorted glomerular symmetry, thickening of the basement membrane, mesangial cells proliferation and widened mesangial regions. It showed the presence of inflammatory infiltration and congestion. MT staining showed the presence of fibrosis in the renal tissue from STZ control rats (). All these changes were attenuated by the treatments with LG (500 and 1000 mg/kg) ( and ) and sitagliptin (5 mg/kg) (). However, LG (250 mg/kg) treatment failed to do so ().
Figure 3. Effect of LG on STZ-induced alterations in kidney histology. Photomicrograph of sections of kidney of (A) normal, (B) diabetic control rats, (C) Sitagliptin (5 mg/kg, p.o.) treated rats (C), LG (250 mg/kg, p.o.) treated rats (D), LG (500 mg/kg, p.o.) treated rats (E) and LG (1000 mg/kg, p.o.) treated rats. H & E staining at 100×. Increase in thickening of the basement membrane (black arrow), necrosis (white arrow) and inflammatory infiltration (red arrow).
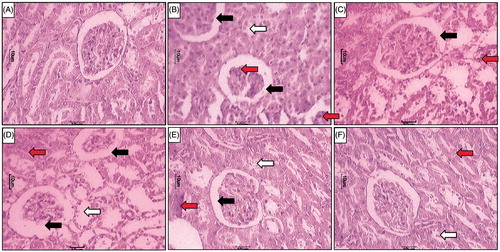
Figure 4. Effect of LG on STZ-induced alterations in kidney histology. Photomicrograph of sections of kidney of (A) normal, (B) diabetic control rats, (C) Sitagliptin (5 mg/kg, p.o.) treated rats (C), LG (250 mg/kg, p.o.)-treated rats (D), LG (500 mg/kg, p.o.)-treated rats (E) and LG (1000 mg/kg, p.o.)-treated rats. MT staining at 40×. Fibrosis (Black arrow).
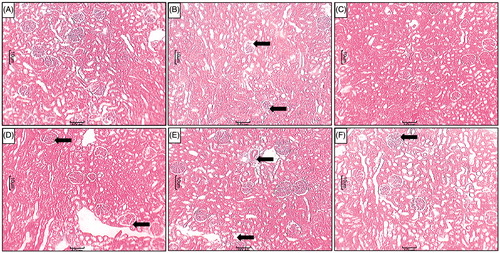
Discussion
DN is one of the major microvascular complications of diabetes mellitus and leading cause of end-stage renal disease.Citation47 Current treatments have been shown to attenuate the functional and structural abnormalities seen in DN disease. However, the associated side effect of these agents limits their use in the treatment of DN. Thus, in the present investigation, we have evaluated the effect of oral administration of LG in STZ-induced diabetic nephropathic rat as it exhibits clinicopathological features including biochemical, oxidative, and metabolic changes that were also presented in human.
Literature is punctuated with evidence that diabetes is associated with features like polydipsia, and polyphagia which may be due to decrease in the availability of glucose and amino acid to cell.Citation48 Impaired cellular biosynthesis and metabolism has been reported to be the underlying cause of diabetes-induced polydipsia, polyphagia, polyuria, and bodyweight loss. In the present investigation, rats administrated with STZ exhibited the features including a loss in body weight, increased food intake as well as water intake and urine output. These features have been investigated by other researchers also, and results of the present investigation are in line with these researchers.Citation48,Citation49 However, this STZ-induced polydipsia, polyphagia, polyuria, and loss in body weight was halted in LG-treated animals when compared with diabetic control animals. The result of present investigation is in accordance with findings of the previous investigation where the administration of glutamine reduced urine output in nephropathic animals.Citation50
It has been well documented that DN is associated with increased urinary albumin excretion thus microalbuminuria is served as a hallmark of DN. Furthermore, kidneys play vital role in the excretion of metabolic wastes including urea, uric acid, and creatinine. Hence, alteration in the levels of BUN, creatinine, and uric acid in serum, as well as urine, reflected renal dysfunction.Citation2,Citation51 Glucosuria induces an osmotic diuresis and dehydration that resulted in a condition of prerenal acute renal failure (ARF). The similar results were noted in a present investigation where 4-week post STZ administration resulted in significantly increased serum albumin, BUN, creatinine, and uric acid as well as urinary albumin excretion. These cumulative alterations in the biochemical parameter of serum and urine reflected in decreased glomerular filtration rate (GFR) which was assessed in terms of creatinine clearance. A study carried out by the previous researcher also showed alteration in these hallmarks of renal dysfunction in STZ-induced diabetic rats.Citation52 However, administration of LG resulted in significant attenuation in BUN, uric acid, creatinine, albumin, and GFR. The previous researcher also showed substantial attenuation in serum creatinine and creatinine clearance after the administration of LG in cisplatin-induced nephropathy.Citation50 Thus LG treatment attenuated this serum and urinary biochemical alteration suggesting it’s direct or indirect role in providing protection against STZ-induced DN or delay in its development.
It has been well documented that hyperglycemia caused abnormalities in ECM which intern caused increase in vascular permeability, abnormalities in blood flow, and inhibition in the formation of endothelial trophic factors.Citation53,Citation54 Elevated plasma glucose stimulates the production of reactive oxygen species (ROS) which in turn generates oxidative stress, these together cause the formation of edema, ischemia, and hypoxia that lead to DN.Citation55,Citation56 In glomerulus; hyperglycemia induces production of hydrogen peroxide that increases lipid peroxidation. Furthermore, cellular death causes generation of ROS, which ultimately lead to the generation of SOD and catalase. SOD indulge in the dismutation of superoxide into oxygen and hydrogen peroxide whereas, catalase involve in the cleavage of hydrogen peroxide into water and oxygen. These hydroxyl radicals pierce the membrane barriers and react mutagenically with DNA in the cell nucleus and show the toxic effect to the cells.Citation57 Intraperitoneal administration of STZ resulting in ROS generation causes lipid peroxidation followed by deterioration of membrane lipid bilayer arrangement and increased tissue permeability through the inactivation of membrane-bound enzymes and receptors which are an essential feature of oxidative stress.Citation58 GSH is a potent ubiquitous, non-enzymatic biological antioxidantCitation48,Citation59,Citation60 plays a vital role in the detoxification of free radical species such as hydrogen peroxide, superoxide, and alkoxy radicals to maintain the cell metabolism and integrity.Citation40 The level of glutamine is successfully reduced in renal tissue of rat under stressful conditions.Citation61 However, glutamine plays an important role in glutathione metabolism.Citation62 Thus, oral glutamine administration may provide ideal conditions for the synthesis of glutathione, which may induce a positive effect on diabetic metabolic abnormalities.
Previously it has been believed that focal segmental glomerulosclerosis is a main manifestation in DN followed by a similar fibrosing process in the tubulointerstitial region.Citation63,Citation64 However, recent studies showed that tubulointerstitial fibrosis could also occur in the early stage of DN and directly induce deterioration of renal function, independent of the glomerular lesions. It has been well documented that renal tissue of STZ-administered animals is associated with aberration of renal histology including thickening of capillary basement membrane, mesangial proliferation, and nodular glomerulosclerosis.Citation65 Researcher showed that hyperglycemia directly induces mesangial expansion via increased matrix production in renal tissue of STZ-treated rats.Citation66 Moreover, extracellular membrane (ECM) protein activation and matrix degradation subdue is correlated with TGF-β-induced fibrogenesis.Citation67,Citation68 Interestingly, TGF-β signal transduction is greatly connected with the Smads.Citation67,Citation68 TGF-β on binding with two specific type I and type II serine/threonine kinase receptor convey its signal across the plasma membrane. Clinical study also showed elevated serum and urinary TGF-β1 level in DN patients.Citation69 In the present study, the expression of TGF-β1 was upregulated in renal tissue of STZ control rats, which is in line with findings of the previous investigator, demonstrating the possible contribution of TGF-β1 to DN.Citation70,Citation71 Treatment with glutamine showed significantly downregulated renal TGF-β1 expression. This notion was further supported by histological findings of renal tissue from glutamine treated rats where thickening of the GBM and tubular necrosis markedly reduced which might be due to the inhibition of TGF-β1 expression.
In the past, the researchers have underlined the correlation between iNOS and DN.Citation72,Citation73 The peroxynitrite, which is a potent and aggressive cellular oxidant formed by the reaction of NO with O2−.Citation74 Nitrite/nitrate levels, as the end products of nitric oxide conversion that caused generation of vicious cycles leads to cell death.Citation75 It has been well documented that streptozotocin-induced diabetes caused an increase in the activity and expression of iNOS.Citation76 In the present investigation, intraperitoneal administration of STZ demonstrated the involvement of iNOS in the inflammatory process reflected by the elevated level of NO in renal tissue. LG treatment in results in inhibition of STZ-induced elevated NO level, and this result is in line with findings of the previous researcher.Citation26
Kidney Injury Molecule-1 (KIM-1), an extracellular protein which is the hallmark of acute kidney injury (AKI) and its expression is elevated during DN in patients with T1DM with or without albuminuria.Citation1,Citation77 Human neutrophil gelatinase-associated lipocalin (NGAL) is a ubiquitous lipocalin iron-carrying protein which bound to gelatinase in specific granules of the neutrophilCitation78 has been identified as an early biomarker involved in ischemic renal injury and repair processes.Citation79 Previously it has been reported that KIM-1 correlate positively with the high glucose level in diabetic patients.Citation80 Expression of KIM-1 and NGAL reported to be increased in renal tissue of rats during DN which is consistent with the results of our present investigation.Citation81 Administration of LG showed significant inhibition in upregulation of diabetes-induced KIM-1 and NGAL mRNA expression, which might be due to its antihyperglycemic potential.
Transforming growth factor-β1 (TGF-β1) is a multifunctional cytokine circulating in a biologically inactive form in human plasma.Citation82,Citation83 It is also known as key mediator of the sclerosing process in kidney, liver, skin, and other organs and its elevated production have been implicated in DN in both animal modelsCitation84,Citation85 and humans.Citation86 Research carried out over past decades showed that high glucose levels caused activation of TGF-β1 that leads to initial structural damage to glomeruli.Citation87,Citation88 Thus, the elevated expression of TGF-β1 serve as a predictor of early diabetic nephropathy. Furthermore, elevated levels of TGF-β1 is related to glomerulosclerosis and interstitial fibrosis in various renal diseases via increases in the synthesis of ECM components, including collagens, fibronectin, and laminin.Citation89 The similar findings were noted in the present investigation where elevated TGF-β1 is associated with upregulated collagen-1 mRNA expression as well as hydroxyproline level in renal tissue. LG treatment significantly inhibited elevated levels of TGF-β1, collagen-1 mRNA, and hydroxyproline which might attribute to its antidiabetic property. Correlation analysis also revealed that antidiabetic potential of LG might play an important role in the attenuation of STZ-induced elevated renal TGF-β1 and collagen-1 mRNA expressions.
Clinically efficacy of glutamine has been proven where administration of oral glutamine (15 g twice a day for seven consecutive days) significantly reduces oxaliplatin-induced neuropathy in colorectal cancer patients.Citation90 In conclusion, results of present investigation suggest that treatment with LG ameliorated STZ-induced DN via the inhibition of oxidonitrosative stress as well as downregulation of KIM-1, NGAL, TGF-β1, and collagen-1 mRNA expressions. This finding may open novel vistas in therapeutic option with amino acids like LG to treat diabetes-induced nephropathy.
Acknowledgements
The authors would like to acknowledge Col. S. K. Joshi, Director and Dr N. S. Vyawahare, Principal, Pad. Dr D. Y. Patil College of Pharmacy Akurdi, Pune, India, for providing necessary facilities to carry out the study. The authors also acknowledge Invaluesys Research Group to carry out statistical analysis of the study.
Disclosure statement
The Authors declare that they have no conflicts of interest to disclose.
References
- Evans JL, Goldfine ID, Maddux BA, Grodsky GM. Oxidative stress and stress-activated signaling pathways: A unifying hypothesis of type 2 diabetes. Endocr Rev. 2002;23:599–622.
- Yorek MA. The role of oxidative stress in diabetic vascular and neural disease. Free Radic. Res. 2003;37:471–480.
- Atkins RC. The epidemiology of chronic kidney disease. Kidney Int. 2005;67:S14–S18.
- Tarchini R, Bottini E, Botti P, et al. [Type 2 diabetic nephropathy: clinical course and prevention proposals 2004]. Giornale Italiano Di Nefrologia: Organo Ufficiale Della Societa Italiana Di Nefrologia. 2004;22:S15–S19.
- Leehey DJ, Singh AK, Alavi N, Singh R. Role of angiotensin II in diabetic nephropathy. Kidney Int. 2000;58:S93–S98.
- Rahimi R, Nikfar S, Larijani B, Abdollahi M. A review on the role of antioxidants in the management of diabetes and its complications. Biomed Pharmacother. 2005;59:365–373.
- Ruiz-Munoz L, Vidal-Vanaclocha F, Lampreabe I. Enalaprilat inhibits hydrogen peroxide production by murine mesangial cells exposed to high glucose concentrations. Nephrol Dial Transplant. 1997;12:456–464.
- Hunt JV, Bottoms MA, Mitchinson MJ. Oxidative alterations in the experimental glycation model of diabetes mellitus are due to protein-glucose adduct oxidation. Some fundamental differences in proposed mechanisms of glucose oxidation and oxidant production. Biochem J. 1993;291:529–535.
- Bierhaus A, Hofmann MA, Ziegler R, Nawroth PP. AGEs and their interaction with AGE-receptors in vascular disease and diabetes mellitus. I. The AGE concept. Cardiovasc Res. 1998;37:586–600.
- Sourris K, Morley A, Koitka A, et al. Receptor for AGEs (RAGE) blockade may exert its renoprotective effects in patients with diabetic nephropathy via induction of the angiotensin II type 2 (AT2) receptor. Diabetologia. 2010;53:2442–2451.
- Yamagishi S-i, Matsui T. Advanced glycation end products, oxidative stress and diabetic nephropathy. Oxid Med Cell Longev. 2010;3:101–108.
- Gross M, Ritz E, Schoof A, et al. Comparison of renal morphology in the Streptozotocin and the SHR/N-cp models of diabetes. Lab Invest. 2004;84:452–464.
- Lee SE, Ma W, Rattigan EM, et al. Ultrastructural features of retinal capillary basement membrane thickening in diabetic swine. Ultrastruct Pathol. 2010;34:35–41.
- Rohrbach D, Wagner C, Star V, Martin G, Brown K, Yoon J-W. Reduced synthesis of basement membrane heparan sulfate proteoglycan in streptozotocin-induced diabetic mice. J Biol Chem. 1983;258:11672–11677.
- Ihm C-G, Lee GS, Nast CC, et al. Early increased renal procollagen α1 (IV) mRNA levels in streptozotocin induced diabetes. Kidney Int. 1992;41:768–777.
- Carpenter L, Cordery D, Biden TJ. Inhibition of protein kinase C δ protects rat INS-1 cells against interleukin-1β and streptozotocin-induced apoptosis. Diabetes. 2002;51:317–324.
- Bendayan M. Immunocytochemical detection of advanced glycated end products in rat renal tissue as a function of age and diabetes. Kidney Int. 1998;54:438–447.
- Chai J, Sheng Z. [A brief account of prevention and treatment of infection in burn patients]. Zhonghua Shao Shang Za Zhi = Zhonghua Shaoshang Zazhi = Chinese Journal of Burns. 2008;24:84–86.
- Gismondo M, Drago L, Fassina M, Vaghi I, Abbiati R, Grossi E. Immunostimulating effect of oral glutamine. Dig Dis Sci. 1998;43:1752–1754.
- Kandhare AD, Bodhankar SL, Singh V, Mohan V, Thakurdesai PA. Anti-asthmatic effects of type-A procyanidine polyphenols from cinnamon bark in ovalbumin-induced airway hyperresponsiveness in laboratory animals. Biomed Aging Pathol. 2013;3:23–30.
- Williams MH. Dietary supplements and sports performance: minerals. J Int Soc Sports Nutr. 2005;2:43–49.
- Garrett AR, Weagel EG, Martinez AD, Heaton M, Robison RA, O’Neill KL. A novel method for predicting antioxidant activity based on amino acid structure. Food Chem. 2014;158:490–496.
- Trachtman H, Futterweit S, Maesaka J, et al. Taurine ameliorates chronic streptozocin-induced diabetic nephropathy in rats. Am J Physiol Renal Physiol. 1995;269:F429–F438.
- Winiarska K, Szymanski K, Gorniak P, Dudziak M, Bryla J. Hypoglycaemic, antioxidative and nephroprotective effects of taurine in alloxan diabetic rabbits. Biochimie. 2009;91:261–270.
- Manna P, Das J, C Sil P. Role of sulfur containing amino acids as an adjuvant therapy in the prevention of diabetes and its associated complications. Curr Diabetes Rev. 2013;9:237–248.
- Todorova VK, Kaufmann Y, Hennings L, Klimberg VS. Oral glutamine protects against acute doxorubicin-induced cardiotoxicity of tumor-bearing rats. J Nutr. 2010;140:44–48.
- Furukawa S, Saito H, Fukatsu K, et al. Glutamine-enhanced bacterial killing by neutrophils from postoperative patients. Nutrition. 1997;13:863–869.
- Todorova V, Kaufmann Y, Luo S, Harms S, Babb K, Klimberg V. Glutamine supplementation reduces glutathione levels and stimulates apoptosis in DMBA-induced mammary tumors. J Surg Res. 2003;114:276.
- Masuko Y. [Impact of stress response genes induced by L-glutamine on warm ischemia and reperfusion injury in the rat small intestine]. [Hokkaido Igaku Zasshi] Hokkaido J Med Sci. 2002;77:169–183.
- de Oliveira Mora L, Antunes LMG, Bianchi MLP. The effects of oral glutamine on cisplatin-induced nephrotoxicity in rats. Pharmacol Res. 2003;47:517–522.
- Bhatt L, Veeranjaneyulu A. Minocycline with aspirin: A therapeutic approach in the treatment of diabetic neuropathy. Neurol Sci. 2010;31:705–716.
- Goswami S, Kandhare A, Zanwar AA, et al. Oral l-glutamine administration attenuated cutaneous wound healing in Wistar rats. Int Wound J. 2016;13:116–124.
- Vaghasiya J, Sheth N, Bhalodia Y, Manek R. Sitagliptin protects renal ischemia reperfusion induced renal damage in diabetes. Regul Pept. 2011;166:48–54.
- Kandhare AD, Shivakumar V, Rajmane A, Ghosh P, Bodhankar SL. Evaluation of the neuroprotective effect of chrysin via modulation of endogenous biomarkers in a rat model of spinal cord injury. J Nat Med. 2014;68:586–603.
- Adil M, Visnagri A, Kumar VS, Kandhare AD, Ghosh P, Bodhankar SL. Protective effect of naringin on sodium arsenite induced testicular toxicity via modulation of biochernical perturbations in rxperiinental rats. Pharmacologia. 2014;5:222–234.
- Badole SL, Chaudhari SM, Jangam GB, Kandhare AD, Bodhankar SL. Cardioprotective activity of Pongamia pinnata in Streptozotocin-Nicotinamide Induced Diabetic Rats. Biomed Res Int. 2015;2015:403291.
- Ghule AE, Kandhare AD, Jadhav SS, Zanwar AA, Bodhankar SL. Omega-3-fatty acid adds to the protective effect of flax lignan concentrate in pressure overload-induced myocardial hypertrophy in rats via modulation of oxidative stress and apoptosis. Int Immunopharmacol. 2015;28:751–763.
- Honmore V, Kandhare A, Zanwar AA, Rojatkar S, Bodhankar S, Natu A. Artemisia pallens alleviates acetaminophen induced toxicity via modulation of endogenous biomarkers. Pharm Biol. 2015;53:571–581.
- Kamble H, Kandhare AD, Bodhankar SL, Mohan V, Thakurdesai PA. Effect of low molecular weight galactomannans from fenugreek seeds on animal models of diabetes mellitus. Biomed Aging Pathol. 2013;3:145–151.
- Kandhare AD, Alam J, Patil MV, Sinha A, Bodhankar SL. Wound healing potential of naringin ointment formulation via regulating the expression of inflammatory, apoptotic and growth mediators in experimental rats. Pharm Biol. 2016;54:419–432.
- Adil M, Kandhare AD, Visnagri A, Bodhankar SL. Naringin ameliorates sodium arsenite-induced renal and hepatic toxicity in rats: decisive role of KIM-1, Caspase-3, TGF-β, and TNF-α. Ren Fail. 2015;37:1396–1407.
- Kandhare AD, Bodhankar SL, Mohan V, Thakurdesai PA. Prophylactic efficacy and possible mechanisms of oligosaccharides based standardized fenugreek seed extract on high-fat diet-induced insulin resistance in C57BL/6 mice. J App Pharma Sci. 2015;5:035–045.
- Kandhare AD, Ghosh P, Ghule AE, Bodhankar SL. Elucidation of molecular mechanism involved in neuroprotective effect of Coenzyme Q10 in alcohol-induced neuropathic pain. Fundam Clin Pharmacol. 2013;27:603–622.
- Visnagri A, Kandhare AD, Ghosh P, Bodhankar SL. Endothelin receptor blocker bosentan inhibits hypertensive cardiac fibrosis in pressure overload-induced cardiac hypertrophy in rats. Cardiovasc Endocrinol. 2013;2:85–97.
- Visnagri A, Kandhare AD, Bodhankar SL. Renoprotective effect of berberine via intonation on apoptosis and mitochondrial-dependent pathway in renal ischemia reperfusion-induced mutilation. Ren Fail. 2015;37:482–493.
- Kandhare AD, Patil MV, Bodhankar SLL. Arginine attenuates the ethylene glycol induced urolithiasis in ininephrectomized hypertensive rats: role of KIM-1, NGAL, and NOs. Ren Fail. 2015;37:709–721.
- Collart F. [Kidney failure, proteinuria, and diabetic nephropathy]. Rev Med Brux. 2003;24:A257–A262.
- Kandhare AD, Raygude KS, Ghosh P, Ghule AE, Bodhankar SL. Neuroprotective effect of naringin by modulation of endogenous biomarkers in streptozotocin induced painful diabetic neuropathy. Fitoterapia. 2012;83:650–659.
- Kandhare AD, Ghosh P, Bodhankar SL. Naringin, a flavanone glycoside, promotes angiogenesis and inhibits endothelial apoptosis through modulation of inflammatory and growth factor expression in diabetic foot ulcer in rats. Chem Biol Interact. 2014;219:101–112.
- de Oliveira Mora L, Antunes LMG, Francescato HDC, Bianchi MLP. The effects of oral glutamine on cisplatin-induced genotoxicity in Wistar rat bone marrow cells. Mutat Res Gen Toxicol Environ Mutagen. 2002;518:65–70.
- Devkar S, Kandhare A, Zanwar A, et al. Hepatoprotective effect of withanolide rich fraction in acetaminophen intoxicated rat: Decisive role of TNF-α, IL-1β, COX-II, and iNOS. Pharm. Biol. 2016. [Epub ahead of print]. DOI: 10.3109/13880209.2016.1157193.
- Budhiraja S, Singh J, Lal H, Arora BR. Renoprotection by telmisartan versus benazepril in streptozotocin induced diabetic nephropathy. Iranian J Pharmacol Ther. 2006;5:135–139.
- Baynes JW. Role of oxidative stress in development of complications in diabetes. Diabetes. 1991;40:405–412.
- Ha H, Kim KH. Role of oxidative stress in the development of diabetic nephropathy. Kidney Int. Suppl. 1995;51:S18–S21.
- Paolisso G, D'Amore A, Galzerano D, et al. Daily vitamin E supplements improve metabolic control but not insulin secretion in elderly type II diabetic patients. Diabetes Care 1993;16:1433–1437.
- Ruiz C, Alegria A, Barbera R, Farre R, Lagarda M. Lipid peroxidation and antioxidant enzyme activities in patients with type 1 diabetes mellitus. Scand J Clin Lab Invest. 1999;59:99–105.
- Kandhare AD, Raygude KS, Ghosh P, Ghule AE, Bodhankar SL. Therapeutic role of curcumin in prevention of biochemical and behavioral aberration induced by alcoholic neuropathy in laboratory animals. Neurosci Lett. 2012;511:18–22.
- Uchiyama M, Mihara M. Determination of malonaldehyde precursor in tissues by thiobarbituric acid test. Anal Biochem. 1978;86:271–278.
- Kandhare AD, Kumar VS, Adil M, Rajmane AR, Ghosh P, Bodhankar SL. Investigation of gastro protective activity of Xanthium strumarium L. by modulation of cellular and biochemical marker. Orient Pharm Exper Med. 2012;12:287–299.
- Kandhare AD, Raygude KS, Shiva Kumar V, et al. Ameliorative effects quercetin against impaired motor nerve function, inflammatory mediators and apoptosis in neonatal streptozotocin-induced diabetic neuropathy in rats. Biomed Aging Pathol. 2012;2:173–186.
- Welbourne T. Ammonia production and glutamine incorporation into glutathione in the functioning rat kidney. Can J Biochem. 1979;57:233–237.
- Hall J, Heel K, McCauley R. Glutamine. Br J Surg. 1996;83:305–312.
- Chan GC, Tang SC. Diabetic nephropathy: Landmark clinical trials and tribulations. Nephrol Dial Transplant. 2016;31:359–368.
- Donate-Correa J, Martín-Núñez E, Muros-de-Fuentes M, Mora-Fernández C, Navarro-González JF. Inflammatory cytokines in diabetic nephropathy. J Diabet Res. 2015;2015:948417.
- O’Connor AS, Schelling JR. Diabetes and the kidney. Am J Kidney Dis. 2005;46:766–773.
- Makino H, Miyamoto Y, Sawai K, et al. Altered gene expression related to glomerulogenesis and podocyte structure in early diabetic nephropathy of db/db mice and its restoration by pioglitazone. Diabetes. 2006;55:2747–2756.
- Lan HY. Transforming growth factor-β/Smad signalling in diabetic nephropathy. Clin Exp Pharmacol Physiol.2012;39:731–738.
- Li L, Yin Q, Tang X, et al. C3a receptor antagonist ameliorates inflammatory and fibrotic signals in type 2 diabetic nephropathy by suppressing the activation of TGF-β/smad3 and IKBα pathway. PLoS One. 2014;9:e113639.
- Shaker O, Sadik N. Transforming growth factor beta 1 and monocyte chemoattractant protein-1 as prognostic markers of diabetic nephropathy. Human Exp Toxicol. 2013;32:1089–1096.
- Yagi K, Kim S, Wanibuchi H, Yamashita T, Yamamura Y, Iwao H. Characteristics of diabetes, blood pressure, and cardiac and renal complications in Otsuka Long-Evans Tokushima Fatty rats. Hypertension. 1997;29:728–735.
- Hellmich B, Schellner M, Schatz H, Pfeiffer A. Activation of transforming growth factor-β 1 in diabetic kidney disease. Metabolism. 2000;49:353–359.
- Kanter M, Sen S, Donmez S, Aktas C, Ustundag S, Erboga M. Protective effects of irbesartan and alpha lipoic acid in STZ-induced diabetic nephropathy in rats. Ren Fail. 2010;32:498–505.
- Zhang Q, Ji Y, Lv W, He T, Wang J. Protective effects of leflunomide on renal lesions in a rat model if diabetic nephropathy. Ren Fail. 2016;38:124–130.
- Yagmurca M, Erdogan H, Iraz M, Songur A, Ucar M, Fadillioglu E. Caffeic acid phenethyl ester as a protective agent against doxorubicin nephrotoxicity in rats. Clin Chim Acta 2004;348:27–34.
- Sudnikovich EJ, Maksimchik YZ, Zabrodskaya SV, et al. Melatonin attenuates metabolic disorders due to streptozotocin-induced diabetes in rats. Eur J Pharmacol. 2007;569:180–187.
- Madar Z, Kalet-Litman S, Stark AH. Inducible nitric oxide synthase activity and expression in liver and hepatocytes of diabetic rats. Pharmacology. 2005;73:106–112.
- Nielsen S, Schjoedt K, Astrup A, et al. Neutrophil Gelatinase‐Associated Lipocalin (NGAL) and Kidney Injury Molecule 1 (KIM1) in patients with diabetic nephropathy: a cross‐sectional study and the effects of lisinopril. Diabetic Med. 2010;27:1144–1150.
- Kjeldsen L, Johnsen AH, Sengeløv H, Borregaard N. Isolation and primary structure of NGAL, a novel protein associated with human neutrophil gelatinase. J Biol Chem 1993;268:10425–10432.
- Mishra J, Mori K, Ma Q, et al. Amelioration of ischemic acute renal injury by neutrophil gelatinase-associated lipocalin. J Am Soc Nephrol. 2004;15:3073–3082.
- Ahmed SA, Hamed MA. Kidney injury molecule-1 as a predicting factor for inflamed kidney, diabetic and diabetic nephropathy Egyptian patients. J Diabetes Metab Disord. 2015;14:1.
- Alter ML, Kretschmer A, Von Websky K, et al. Early urinary and plasma biomarkers for experimental diabetic nephropathy. Clin Lab. 2012;58:659.
- Attisano L, Wrana JL. Signal transduction by members of the transforming growth factor-beta superfamily. Cytokine Growth Factor Rev.1996;7:327–339.
- August P, Suthanthiran M. Transforming growth factor beta signaling, vascular remodeling, and hypertension. N Engl J Med. 2006;354:2721–2723.
- Nakamura T, Fukui M, Ebihara I, et al. mRNA expression of growth factors in glomeruli from diabetic rats. Diabetes. 1993;42:450–456.
- MacKay K, Striker LJ, Stauffer JW, Doi T, Agodoa LY, Striker GE. Transforming growth factor-beta. Murine glomerular receptors and responses of isolated glomerular cells. J Clin Invest. 1989;83:1160
- Yamamoto T, Nakamura T, Noble NA, Ruoslahti E, Border WA. Expression of transforming growth factor beta is elevated in human and experimental diabetic nephropathy. Proc Natl Acad Sci. 1993;90:1814–1818.
- Ellis D, Forrest KY, Erbey J, Orchard TJ. Urinary measurement of transforming growth factor-beta and type IV collagen as new markers of renal injury: Application in diabetic nephropathy. Clin Chem. 1998;44:950–956.
- Bertoluci MC, Uebel D, Schmidt A, Thomazelli FCS, Oliveira FR, Schmid H. Urinary TGF-β1 reduction related to a decrease of systolic blood pressure in patients with type 2 diabetes and clinical diabetic nephropathy. Diabetes Res Clin Prac. 2006;72:258–264.
- Kreisberg JI, Ayo SH. The glomerular mesangium in diabetes mellitus. Kidney Int. 1993;43:109–113.
- Wang W-S, Lin J-K, Lin T-C, et al. Oral glutamine is effective for preventing oxaliplatin-induced neuropathy in colorectal cancer patients. Oncologist. 2007;12:312–319.