Abstract
Purpose
To investigate the preventive effect of aerobic exercise on renal damage caused by obesity.
Methods
The mice in the Control (Con) and Control + Exercise (Con + Ex) groups received a standard chow diet for the 21-week duration of the study, while the High-fat diet (HFD) group and High-fat diet + Exercise (HFD + Ex) group were fed an HFD. Mice were acclimated to the laboratory for 1 week, given 12 weeks of being on their respective diets, and then the Con + Ex and HFD + Ex groups were subjected to moderate intensity aerobic treadmill running 45 min/day, 5 days/week for 8 weeks.
Results
We found that HFD-induced obesity mainly impacts kidney glycerin phospholipids, glycerides, and fatty acyls, and aerobic exercise mainly impacts kidney glycerides, amino acids and organic acids as well as their derivatives. We identified 18 metabolites with significantly altered levels that appear to be involved in aerobic exercise mediated prevention of HFD-induced obesity and renal damage, half of which were amino acids and organic acids and their derivatives.
Conclusion
Aerobic exercise rewires kidney metabolites to reduce high-fat diet-induced obesity and renal injury.
1. Introduction
High-fat diet-induced obesity is typically accompanied by complicated metabolic abnormalities and can be coincident with multiple chronic diseases, including renal dysfunction and kidney diseases [Citation1]. Despite the prevalence of kidney diseases across the globe, the lack of awareness, limited access to treatment, and high medical cost seriously threaten the health and lives of many people [Citation2]. Concurrently, obesity is a global epidemic phenomenon growing at an increased rate every year, which accelerates the morbidity of kidney diseases [Citation3]. Aerobic exercise has been shown to enhance metabolism, control body weight, and improve chronic kidney disease, diabetes, and hypertension-related kidney disease [Citation4–6]. However, there has been few researches on the effects of aerobic exercise in preventing obesity-related renal dysfunction or injury. Additionally, few studies have been conducted on the detection and identification of metabolites in kidney tissues because most studies have analyzed urine and blood or focused on specific regulatory pathways.
This study aims to use metabolomics analysis, specifically liquid chromatography electrospray ionization-tandem mass spectrometry (LC–ESI–MS/MS), to determine how aerobic exercise affects kidney metabolites in obese mice. We hypothesized that aerobic exercise could be a prevention strategy for obesity-related renal injury and attempted to identify several important metabolites that contribute to its effectiveness. This is a necessary first step in trying to elucidate the mechanism of how complicated metabolic pathways interact in obesity and renal function. Ultimately, our work will provide useful data for the future prevention and treatment of kidney diseases.
2. Materials and methods
2.1. Animals and exercise arrangement
Wild-type C57BL/6 male mice (n = 28, 3 weeks old) were purchased and raised in the Laboratory Animal Research Center at Tsinghua University (Beijing, China). This study was conducted under oversight and with the approval of the Institutional Animal Care and Use Committee of Tsinghua University (F16-00228 (A5916-01)). Mice were randomly divided into four groups after adapting to the laboratory environment for 1 week while being and were given ad libitum access to water and food. Then, the high fat diet (HFD) group was given a diet consisting of 45–60% fat for 12 weeks, which has been previously established as an HFD-induced model of obesity [Citation7, Citation8]. In this study, the Control (Con) group and Control + Exercise (Con + Ex) group were fed a standard chow diet (CD) with 12% kcal fat. The HFD group and HFD + Exercise (HFD + Ex) group were fed a diet with 60% kcal fat (n = 7 mice/group). The Con + Ex and HFD + Ex groups were familiarized with treadmill running for 1 week without training and then were subjected to 8 weeks of aerobic exercise (). Adaptive training was 5 m/min and 0° incline, 10–15 min/day. Aerobic exercise was 14 m/min in the first week, increased by 0.5 m/min each week, reaching 17.5 m/min in the eighth week, and the exercise intensity was maintained within 50%–60% of VO2max intensity [Citation9]. The aerobic training schedule was 45 min/day, 5 days/week after warming up at 5 m/min for 10 min/day. All mice were sacrificed and samples were harvested at the end of the 21-week duration.
2.2. Biological parameter measurements
The body weights of the mice were recorded every week. At the end of 8 weeks of aerobic exercise, the mice were anesthetized after fasting for 12 h. Blood samples were obtained through cardiac puncture until the mice died. Kidneys were harvested at the same time. Left kidney samples were fixed in 4% paraformaldehyde and prepared for periodic acid-Schiff (PAS) staining, and three sections were observed for each sample at 400x. The PAS-stained sections were visualized by Pannoramic DESK (3D Histech, Hungary) and analyzed by Image-Pro Plus 6.0 software. Right kidney samples were stored at −80 °C. Serum total cholesterol (TC), triglyceride (TG), high density lipoprotein cholesterol (HDL), and low-density lipoprotein cholesterol (LDL) were measured by an automated chemistry analyzer (Kehua ZY KHB1280, China). Serum cystatin-C (Cys-C) and neutrophil gelatinase-associated lipocalin (NGAL) were analyzed using ELISA kits (MEIMIAN, China).
2.3. Kidney sample preparation and extraction
From the frozen right kidneys, for hydrophilic compounds, a 50 mg sample was whirled for 5 min with 1 mL 70% methanol and then centrifuged at 12000 rpm for 10 min at 4 °C. After centrifugation, 400 µL of supernatant was stored at −20 °C overnight. Then, the samples were centrifuged at 12000 rpm for 3 min at 4 °C, and 200 µL of supernatant was used for onboard analysis. For hydrophobic compounds, a 20 mg sample was whirled for 15 min with a 1 mL solution of methyl-tert-butyl ether (MTBE), methanol, and an internal standard mixture. After adding 200 µL water, the samples were whirled for 1 min, and then centrifuged at 12000 rpm for 10 min at 4 °C. Next, 300 µL of supernatant was extracted and concentrated. Finally, the powder was dissolved 200 µL of mobile phase B and stored at −80 °C. The dissolving solution was taken into the sample bottle for LC–MS/MS analysis.
2.4. LC–ESI–MS/MS conditions
LC–ESI–MS/MS system (ExionLC AD UPLC-QTRAP, SCIEX, USA) and Analyst 1.6.3 software (AB SCIEX, USA) were used to analyze kidney sample extracts. Hydrophilic compounds were subject to a UPLC column temperature of 40 °C, 0.4 mL/min flow rate, T3 UPLC C18 column and Amide UPLC Amide column. Hydrophobic compounds were subject to a UPLC column of temperature 45 °C, 0.35 mL/min flow rate, C30 column.
2.5. Statistical analysis
Experimental data are presented as the mean ± SEM and were analyzed using GraphPad Prism 8.0 software. Metabolite analyses used the multivariate statistical method orthogonal projections to latent structures discriminant analysis (OPLS-DA) based on values of fold-change (FC) and variable importance in projection (VIP). We screened the metabolites using screening criteria FC ≥ 2 or ≤ 0.5 and VIP > 1. The metabolite analysis diagrams were generated using R software. Comparisons between groups were performed using one-way ANOVA followed by Tukey’s multiple comparison tests or Student’s t test, and p < 0.05 was considered statistically significant.
3. Results
3.1. Aerobic exercise prevented HFD-induced obesity
The body weights of HFD-fed mice have been reported to increase from the second week, while metabolic and phenotypic characteristics of obesity develop over a period of 10 to 12 weeks with HFD [Citation10, Citation11]. In our study, mice fed an HFD exhibited a significantly greater body weight than CD-fed mice at the end of 12 weeks (). The HFD + Ex and Con + Ex groups both showed a significant reduction in body weight compared to the HFD and Con groups, respectively. These data indicate that aerobic exercise can effectively regulate body weight in HFD and CD mice.
Serum TC, TG, HDL, and LDL have been reported to be higher in obese mice [Citation12]. To test the effects of aerobic exercise on obesity, we measured these serum parameters at the conclusion of our 21-week regimen. Serum TC, HDL, and LDL levels were significantly higher in the HFD group than in the Con group and had a decreasing trend in the HFD + Ex group compared to the HFD group (). However, there was no significant change in serum TG levels as a result of diet or exercise (). These data indicate that 8 weeks of aerobic exercise has the potential to reduce serum cholesterol in obese mice.
3.2. Aerobic exercise prevented HFD-induced renal injury
We then examined the impact of aerobic exercise on kidneys under normal and obesity-inducing conditions. Glomerular hypertrophy is one of the pathological changes in obesity-related glomerulopathy [Citation13]. Therefore, we used PAS staining to visualize the glomerulus. Histological results showed that glomerular areas and circumferences increased after HFD feeding, suggesting that the glomerulus was enlarged in these mice (). This obesity-related glomerular hypertrophy was prevented by aerobic exercise ().
Figure 2. Impact of aerobic exercise on the kidney. (A) PAS staining of glomerulus, (B) glomerular area, (C) glomerular circumference, (D) serum Cyc-C level, (E) serum NGAL level. [mean ± SEM, ** p < 0.01].
![Figure 2. Impact of aerobic exercise on the kidney. (A) PAS staining of glomerulus, (B) glomerular area, (C) glomerular circumference, (D) serum Cyc-C level, (E) serum NGAL level. [mean ± SEM, ** p < 0.01].](/cms/asset/a6c057b7-114e-4395-8bed-de1ac29f5fa2/irnf_a_2286330_f0002_c.jpg)
Serum Cyc-C and NGAL are related to renal injury [Citation14, Citation15], and our data show that obese mice demonstrated significantly increased levels in comparison to CD-fed mice. Notably, aerobic exercise resulted in a reduction in serum Cyc-C and NGAL levels in obese mice (). Collectively, these results demonstrate that HFD-induced obesity is related to renal injury, which can be averted by aerobic exercise.
3.3. Effects of HFD and aerobic exercise on kidney metabolites
To investigate the link between obesity-associated early kidney injury and aerobic exercise, we performed a metabolite analysis of kidney tissues using LC–ESI–MS/MS. We then compared the data between the Con and HFD groups () as well as between the HFD and HFD + Ex groups (). The OPLS-DA model clearly separated the Con group from the HFD group () and the HFD group from the HFD + Ex group (). We confirmed that these were effective models based on Q2 > 0.5 from 200 permutation tests (). For the Con vs. HFD group, our screening process identified 272 differentially abundant metabolites, including 136 that were increased and 136 that were decreased (). For the HFD vs. HFD + Ex group, we found 64 differentially abundant metabolites, with 53 being increased and 11 being decreased (). To identify the pathways that could be involved in these changes, we performed Kyoto Encyclopedia of Genes and Genomics (KEGG) analysis. For HFD-induced obesity, we found that glycerophospholipid metabolism, autophagy, ovarian steroidogenesis, fatty acid biosynthesis, and steroid biosynthesis pathways were mainly involved (). For aerobic exercise, we identified fat digestion and absorption, cholesterol metabolism, vitamin digestion and absorption, thermogenesis, insulin resistance, regulation of lipolysis in adipocytes, glycerolipid metabolism, retinol metabolism, and steroid biosynthesis as involved pathways ().
Figure 3. Metabolite analysis of the Con group compared to the HFD group. (A) OPLA-DA score chart for the Con (orange) and HFD (purple) groups, (B) OPLS-DA model validation plot (R2X = 0.472, R2Y = 0.997, Q2 = 0.959 and p < 0.005), (C) volcano plot of differentially abundant metabolites, (D) KEGG pathway enrichment analysis.
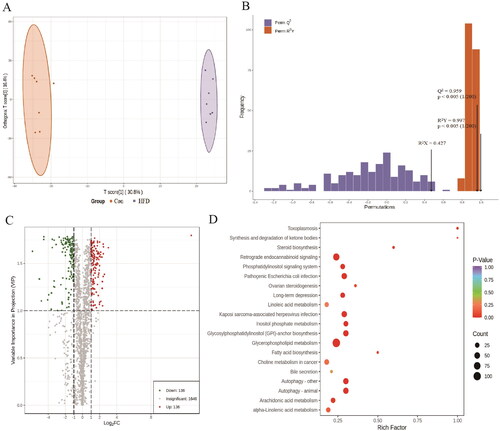
Figure 4. Metabolite analysis of the HFD group compared to the HFD + ex group. (A) OPLA-DA score chart for the HFD (purple) and HFD + ex (green) groups, (B) OPLS-DA model validation plot (R2X = 0.365, R2Y = 0.975, Q2 = 0.767 and p < 0.005), (C) volcano plot of differentially abundant metabolites, (D) KEGG pathway enrichment analysis.
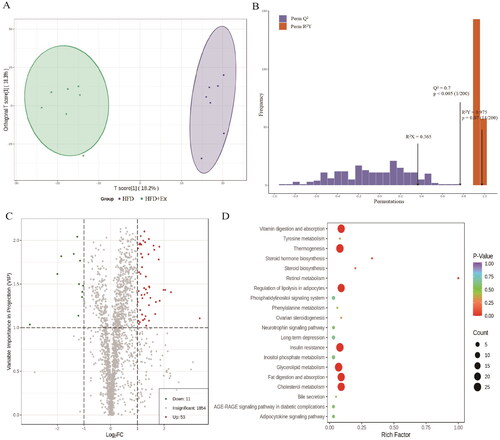
3.4. Aerobic exercise reconstructed kidney metabolites in obese mice
Finally, we analyzed differentially abundant metabolites in the Con group compared to the HFD group and the HFD group compared to the HFD + Ex group, and found that 24 codifferential metabolites (). Hierarchical clustering analysis based on these 24 codifferential metabolites revealed that Con, HFD, and HFD + Ex were distinguishable groups, and HFD + Ex and Con shared similar metabolomic profiles (). The metabolomic profiling data showed that HFD-induced obesity primarily affected glycerin phospholipids, followed by glycerides and fatty acyls; aerobic exercise primarily influenced glycerides, followed by amino acids and its derivatives, and organic acids and its derivatives (). We found that six metabolites were either increased or decreased by HFD and then showed a further change in the same direction when challenged with exercise (). The remaining 18 metabolites are primarily affected amino acids and its derivatives, and organic acids and its derivatives, which could be involved in preventing obesity-associated renal injury (, top 18). Collectively, our findings suggest that aerobic exercise restored the metabolomic profile of the kidney in obese mice to a normal state.
Figure 5. Correlation analysis in the Con, HFD, and HFD + ex groups. (A) Venn diagram of differentially abundant metabolites between Con compared to HFD and HFD compared to HFD + ex, (B) Clustering and heatmap of 24 codifferential metabolites in each sample.
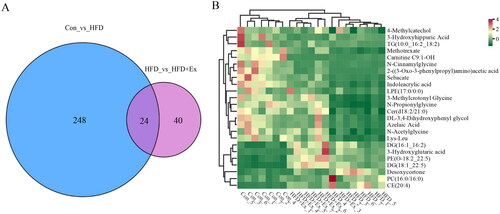
Table 1. Overview of annotated metabolites.
Table 2. Overview of 24 codifferential metabolites.
4. Discussion
This study investigated the effects of aerobic exercise on kidney metabolites in obese mice using LC–ESI–MS/MS and we found that aerobic exercise could prevent and ameliorate high-fat diet–induced renal injury through kidney metabolite modulation in mice.
HFD and aerobic exercise had no effect on TG in this study (), which is supported by a previous study that used an automated chemistry analyzer [Citation16]. However, another study reported that increased TG levels were detected by assay kits in obese mice [Citation17]. It is difficult to reconcile this discrepancy, but it could be due to assay method or experimental conditions. Although HDL levels are generally thought to be reduced with obesity, we and others reported that HDL levels appear to be increased () [Citation12, Citation16]. One possible explanation for this is that the HFD induces an increase in the total concentration of lipoprotein to transport lipids in serum, including both LDL and HDL.
Five of the 18 major kidney metabolites that we identified have previously been reported to be correlated with obesity-related metabolic dysregulation through previous studies [Citation18–24]: indoleacrylic acid, azelaic acid, N-acetylglycine, 4-methylcatechol, and desoxycortone (, top 5). The first four of these were increased in the HFD-Ex group compared to the HFD group. Indoleacrylic acid is reported to lower LDL levels and improve immune-inflammatory responses, and it has been reported to be increased during the recovery of kidney diseases [Citation18]. Patients with renal function decline show a decrease in azelaic acid [Citation18, Citation19]. Azelaic acid has been shown to positively rebalance plasma glucose, triglycerides, insulin, and markers for liver and kidney function to near normal levels as well as decrease the levels of lipid peroxidation markers in mice fed an HFD [Citation20]. Evidence shows that N-acetylglycine can serve as a potential biomarker in regulating body weight and may have an association with adipose-tissue immunity and lipid metabolism [Citation21]. The level of N-acetylglycine increases during weight loss, which is consistent with our findings that aerobic exercise may increase its levels. Moreover, the function of N-acetylglycine in the kidney has rarely been reported. 4-Methylcatechol could respond to exercise to reduce depression [Citation22]. In the kidney, 4-methylcatechol promotes renal function through modulating NGF/TrkA and ROS-related Akt/GSK3β/β-catenin pathways and could reduce depression when exercising [Citation23], which could be involved in the mechanism through which exercise improves kidney metabolism. Desoxycortone, was reduced in the HFD + Ex group compared to the HFD group. It has been used to induce hypertension in animal models associated with oxidative stress and inflammation, but could also promote renal inflammation and injury [Citation24]. An examination of the causal relationship between these five key metabolites and the preventive effects of aerobic exercise on obesity-related renal injury is warranted in the future. In addition, 3-hydroxyglutaric acid is a known metabolite of the kidney [Citation25, Citation26] (, metabolites 22–24), which we found to be affected by HFD and further changed in the same direction by exercise. We speculated that these may be metabolites of a stress response to obesity and aerobic challenge and should be further investigated. Together, our KEGG analysis and data from previous studies suggest that exercise-induced improvements in the kidney based on these metabolites could be involved in insulin resistance, regulation of lipolysis in adipocytes, glycerolipid metabolism, and inflammatory responses.
In this study, we focused on the metabolomic profiling of mouse kidneys under HFD-induced metabolic stress and aerobic exercise training, but we note that there are clear limitations in our work. First, our study lacked urinalysis, such as urine albumin and urine protein to creatinine ratio, to assess renal function, which was an enormous regret. While serum NGAL has been suggested to be correlated with renal injury, it is a primary marker of metaflammation or metabolic endotoxaemia due to factors such as leaky gut, which may be present in our model due to HFD. Urinalysis could provide an idea of tubular injury because it is considerably produced by injured renal tubules. Second, we found that exercise maintains body weight rather than inducing weight loss (), which may be due to the mice having ad libitum access to food, and future studies should investigate the effects of limiting caloric intake. Third, we were not able to identify previously reported relationships among metabolites 6–22 in related to kidney function and exercise, and future studies should investigate the roles of these metabolites in obesity-related kidney diseases and the effects of aerobic exercise, which may provide novel insights into the pathogenesis of obesity-related kidney diseases.
All in all, our results are summarized below: (1) HFD-induced obesity might associated with renal injury, and aerobic exercise could effectively prevent HFD-induced obesity. (2) HFD-induced obesity mainly impacts kidney glycerin phospholipids, glycerides, and fatty acyls; aerobic exercise mainly impacts kidney glycerides, amino acids and its derivatives, and organic acids and its derivatives. (3) Aerobic exercise mitigates the onset of obesity-related kidney diseases through reshaping kidney metabolism.
Disclosure statement
No potential conflict of interest was reported by the authors.
Additional information
Funding
References
- Neuen BL, Chadban SJ, Demaio AR, et al. Chronic kidney disease and the global ncds agenda[J]. BMJ Glob Health. 2017;2(2):1. doi:10.1136/bmjgh-2017-000380.
- Collaboration G B D C K D. Global, regional, and national burden of chronic kidney disease, 1990-2017: a systematic analysis for the global burden of disease study 2017[J]. Lancet. 2020;395(10225):709–9.
- Misra A, Tandon N, Ebrahim S, et al. Diabetes, cardiovascular disease, and chronic kidney disease in South asia: current status and future directions[J]. BMJ. 2017;357:j1420. doi:10.1136/bmj.j1420.
- Johansen KL, Painter P. Exercise in individuals with ckd[J]. Am J Kidney Dis. 2012;59(1):126–134. doi:10.1053/j.ajkd.2011.10.008.
- Duan YC, Shi L, Jin Z, et al. Swimming exercise ameliorates hypertension-induced kidney dysfunction via alleviating renal interstitial fibrosis and apoptosis. [JKidney Blood Press Res. 2021;46(2):219–228. doi:10.1159/000514680.
- Amaral LSB, Souza CS, Lima HN, et al. Influence of exercise training on diabetic kidney disease: a brief physiological approach[J]. Exp Biol Med (Maywood). 2020;245(13):1142–1154. doi:10.1177/1535370220928986.
- Li Y, Cheng Y, Zhou Y, et al. High fat diet-induced obesity leads to depressive and Anxiety-Like behaviors in mice via ampk/Mtor-Mediated autophagy[J]. Exp Neurol. 2022;348:113949. doi:10.1016/j.expneurol.2021.113949.
- Yeu J, Ko HJ, Kim D, et al. Evaluation of insight vet dxa (Dual-Energy X-Ray absorptiometry) for assessing body composition in obese rats fed with high fat diet: a follow-up study of diet induced obesity model for 8 weeks[J]. Lab Anim Res. 2019;35(1):2. doi:10.1186/s42826-019-0004-2.
- Xiong Y, Chen Y, Liu Y, et al. Moderate-Intensity continuous training improves Fgf21 and klb expression in obese mice[J]. Biochemistry (Mosc). 2020;85(8):938–946. doi:10.1134/S000629792008009X.
- da Silva Xavier G, Hodson DJ. Mouse models of peripheral metabolic disease[J]. Best Pract Res Clin Endocrinol Metab. 2018;32(3):299–315. doi:10.1016/j.beem.2018.03.009.
- Savetsky IL, Albano NJ, Cuzzone DA, et al. Lymphatic function regulates contact hypersensitivity dermatitis in obesity[J]. J Invest Dermatol. 2015;135(11):2742–2752. doi:10.1038/jid.2015.283.
- Lee HS, Nam Y, Chung YH, et al. Beneficial effects of phosphatidylcholine on high-fat diet-induced obesity, hyperlipidemia and fatty liver in mice[J]. Life Sci. 2014;118(1):7–14. doi:10.1016/j.lfs.2014.09.027.
- D’Agati VD, Chagnac A, de Vries AP, et al. Obesity-Related glomerulopathy: clinical and pathologic characteristics and pathogenesis[J]. Nat Rev Nephrol. 2016;12(8):453–471. doi:10.1038/nrneph.2016.75.
- Marakala V. Neutrophil Gelatinase-Associated lipocalin (ngal) in kidney injury - a systematic review[J]. Clin Chim Acta. 2022;536:135–141. doi:10.1016/j.cca.2022.08.029.
- Shlipak MG, Inker LA, Coresh J. Serum cystatin C for estimation of gfr[J]. JAMA. 2022;328(9):883–884. doi:10.1001/jama.2022.12407.
- Ivanovic N, Minic R, Dimitrijevic L, et al. Lactobacillus Rhamnosus La68 and Lactobacillus Plantarum Wcfs1 differently influence metabolic and immunological parameters in high fat diet-induced hypercholesterolemia and hepatic steatosis[J]. Food Funct. 2015;6(2):558–565. doi:10.1039/c4fo00843j.
- Liu R, Zheng Y, Cai Z, et al. Saponins and flavonoids from adzuki bean (vigna angularis L.) ameliorate high-fat diet-induced obesity in icr mice[J]. Front Pharmacol. 2017;8:687. doi:10.3389/fphar.2017.00687.
- Wang J, Yan W, Zhou X, et al. Metabolomics window into the role of acute kidney injury after coronary artery bypass grafting in diabetic nephropathy progression[J]. PeerJ. 2020;8:e9111. doi:10.7717/peerj.9111.
- Tang X, You J, Liu D, et al. 5-Hydroxyhexanoic acid predicts early renal functional decline in type 2 diabetes patients with microalbuminuria[J]. Kidney Blood Press Res. 2019;44(2):245–263. doi:10.1159/000498962.
- Muthulakshmi S, Saravanan R. Protective effects of azelaic acid against high-fat diet-induced oxidative stress in liver, kidney and heart of C57bl/6j mice[J]. Mol Cell Biochem. 2013;377(1-2):23–33. doi:10.1007/s11010-013-1566-1.
- Fluhr L, Mor U, Kolodziejczyk AA, et al. Gut microbiota modulates weight gain in mice after discontinued smoke exposure[J]. Nature. 2021;600(7890):713–719. doi:10.1038/s41586-021-04194-8.
- Xie Y, Wu Z, Zhou L, et al. Swimming exercise modulates gut microbiota in Cums-Induced depressed mice[J]. Neuropsychiatr Dis Treat. 2022;18:749–760. doi:10.2147/NDT.S355723.
- Selda GO, Ediz C, Merve E, et al. 4-Methylcatechol prevents Streptozotocin-Induced acute kidney injury through modulating ngf/trka and Ros-Related akt/Gsk3β/B-Catenin pathways[J]. Int Immunopharmacol. 2018;64:52–59.
- Thomas JM, Ling YH, Huuskes B, et al. Il-18 (interleukin-18) produced by renal tubular epithelial cells promotes renal inflammation and injury during deoxycorticosterone/Salt-Induced hypertension in mice[J]. Hypertension. 2021;78(5):1296–1309. doi:10.1161/HYPERTENSIONAHA.120.16437.
- Nowak PJ, Wilk R, Prymont-Przyminska A, et al. Hemodialysis decreases the concentration of accumulated plant phenols in the plasma of patients on maintenance dialysis: influence of residual renal function[J]. Ther Apher Dial. 2017;21(6):572–585. doi:10.1111/1744-9987.12586.
- Bazargani B, Mojtahedi SY, Fahimi D, et al. Evaluation of the relationship between serum carnitine levels and intradialytic complications in children with kidney failure[J]. Pediatr Nephrol. 2022;37(9):2179–2183. doi:10.1007/s00467-022-05449-w.