Abstract
The incidence and mortality of chronic kidney disease (CKD) are increasing globally. Studies have demonstrated the significance of genetic risk factors in the progression of CKD. Telomerase reverse transcriptase (TERT) may be implicated in the development of CKD. This study aimed to investigate the correlation between TERT gene variants and susceptibility to CKD in the Chinese population. A total of 507 patients with CKD and 510 healthy controls were recruited for this case-control study. Four candidate loci were identified using the MassARRAY platform. Logistic regression analysis was employed to assess the association between TERT gene variants and the risk of CKD. The false positive reporting probability (FPRP) method was utilized to evaluate the validity of statistically significant associations. The multifactorial dimensionality reduction (MDR) method was used to evaluate the interaction between SNPs and the risk of CKD. Furthermore, discrepancies in the clinical features of subjects with diverse genotypes were evaluated using one-way analysis of variance (ANOVA). Our findings revealed a correlation between rs2735940 and rs4635969 and an increased risk of CKD. Stratification analysis indicated that rs4635969 was related to an increased risk of CKD in different subgroups (age ≤ 50 years and male). MDR analysis indicated that the two-site model (rs2735940 and rs4635969) was the best prediction model. Furthermore, the rs2735940 GG genotype was found to be linked to an increased level of microalbuminuria (MAU) in patients with CKD. Our study is the first to reveal a connection between TERT gene variants and susceptibility to CKD, providing new insights into the field of nephrology.
Introduction
The kidney is a prominent excretory and endocrine organ that plays a vital role in maintaining body homeostasis by regulating fluid and electrolyte balance, blood pressure, and the composition and volume of blood [Citation1]. Chronic kidney disease (CKD) is a long condition characterized by the gradual of kidney function over time. CKD is defined as the presence of an abnormality in kidney structure or function persisting for more than 3 months [Citation2]. It is typically a progressive disease that can lead to kidney failure if left untreated. CKD affects about 10% of adults in many countries and is the common result of the continuous progression of various types of nephropathy [Citation3]. CKD is expected to become the fifth leading cause of death by 2040 [Citation4], which will impose a significant health and economic burden on the world. The study has shown that individuals with impaired renal function have an increased risk of progressing to ESRD [Citation5], as well as an increased risk of morbidity and mortality from cardiovascular disease [Citation6]. Simultaneously, hyperglycemia, hyperlipidemia, and hypertension are considered major risk factors that contribute to the progression of CKD [Citation7]. Furthermore, studies on familial aggregation have shown that ESRD and early CKD tend to occur within families [Citation8]. Studies have found the importance of other factors, including genetic risk factors, in the progression of nephropathy [Citation9]. Therefore, studies based on susceptibility loci to unravel the genetic basis of nephropathy may lead to the discovery of new disease mechanisms.
Telomerase reverse transcriptase (TERT) serves as the catalytic subunit of telomerase. Its structure is highly conserved and plays a vital role in maintaining telomere and chromosome stability, as well as preventing malignant tumors [Citation10,Citation11]. It has been reported that abnormal telomerase activity is associated with the occurrence and development of cancer, and telomere length is negatively correlated with the incidence and mortality of cancer [Citation12]. Some studies have found that carriers of short leukocyte telomere length exhibit a significant reduction in the GFR and an increase in the urinary albumin-to-creatinine ratio (ACR) [Citation13,Citation14]. TERT also plays a crucial role in the development and progression of kidney diseases. Several studies have provided evidence supporting the significance of TERT in renal pathologies. For example, a study Long et al. demonstrated that the upregulation of TERT promotes the growth of renal cell carcinoma (RCC) [Citation15]. In addition, the TERT locus genotypes of rs2736100-CC/CA and rs2736098-AA have been associated with shorter survival in patients with RCC, as reported by Ma et al. [Citation16]. Furthermore, the study by Casuscelli et al. focused on TERT promoter region mutations in RCC, and their findings suggest that TERT promoter mutations may serve as biomarkers for predicting the prognosis of RCC patients [Citation17]. Moreover, there is a published literature report on epigenome-wide association study (EGWAS) for IgA nephropathy, and they found that three methylation CpGs corresponding to TERT were significantly associated with IgA nephropathy [Citation18]. These findings indicate that TERT may play an important role in the pathogenesis of CKD. Single nucleotide polymorphisms (SNPs), the most common type of human heritable variants, are likely to be biomarkers for human disease, including nephropathy [Citation19]. Previous study has showed that rs2736100 of TERT was associated with the risk of glomerulonephritis (GN), CDK, and ESRD [Citation20]. However, the association between TERT gene variants and CKD has not been determined.
Therefore, this research aimed to investigate the association of TERT gene variants with CKD susceptibility in the Chinese population. Our study will help to elucidate the mechanism of TERT gene variants in the occurrence and progression of CKD, and provides new targets and strategies for the early diagnosis, prevention, and individualized treatment for CKD.
Materials and methods
Study subjects
The sample size was calculated using G* Power version 3.1.9.7 (Kiel, Germany) software prior to the study. Initially, the statistical method (t-test) was chosen, followed by the selection of classification (difference between two independent means (two groups)). The parameters were then set as follows: tail = 2, effective size = 0.2, α = 0.05, power = 0.885, and allocation ratio = 1. Finally, the sample size for both the case group and the control group was calculated to be 501 cases each. In this study, a total of 1017 unrelated Chinese Han participants, including 507 CKD patients and 510 healthy subjects, were randomly selected from the First Affiliated Hospital of Xi’an Jiaotong University between April 2018 and December 2021. This article fully complies with the principles of the Declaration of Helsinki and has been approved by the Ethics Committee of the First Affiliated Hospital of Hainan Medical College. Informed consent was obtained from all participants prior to the start of the study. All patients were initially diagnosed with CKD and confirmed by two experienced nephrologists through the standard clinical diagnosis, laboratory tests, imaging, or histopathological evaluation. The inclusion criteria for the patients are as follows: (1) age ≥ 18 years old; (2) patients were confirmed CKD by the diagnostic criteria for CKD; (3) patients were Chinese Han population. The criteria for CKD diagnosis are as follows: 1) a glomerular filtration rate (GFR) of less than 60 mL/min/1.73 m2, albuminuria of at least 30 mg per 24 h, or urine ACR ≥ 30 mg/g), or 2) markers of kidney damage (e.g., hematuria or structural abnormalities, such as polycystic or dysplastic kidneys) persisting for more than 3 months, or 3) renal tubular disorders [Citation21]. Staging of GFR is classified as G1 (GFR ≥ 90 mL/min/1.73 m2), G2 (GFR 60–89 mL/min/1.73 m2), G3 (30–59 mL/min/1.73 m2), G4 (15–29 mL/min/1.73 m2), and G5 (<15 mL/min/1.73 m2). The exclusion criteria for the patients were as follows: (1) patients with genetic diseases; (2) patients with any medical or family history of kidney damage or CKD (3) patients who had previously received renal replacement therapy patients with established nephropathy; (4) patients with other major diseases or comorbidities, such as malignant tumors or heart diseases (including arterial hypertension, left ventricular hypertrophy/diastolic dysfunction, and coronary artery disease); and (5) patients for whom complete clinical data were not available. The inclusion criteria for the control group were as follows: (1) healthy individuals with normal renal function who underwent a physical examination at the same hospital as the cases during the corresponding period; (2) age ≥ 18 years old; and (3) gender and other characteristics matched to the case group. The exclusion criteria for the control group were as follows: (1) a history of nephropathy or any other serious illness; (2) patients for whom complete clinical data were not available; and (3) the presence of a familial relationship with the case group. Moreover, the basic features of the subjects, such as age, gender, and CKD stage, were assessed through questionnaires or by professional physicians.
SNP selection and genotyping
The SNPs of TERT were chosen from the 1000 Genomes Project based on the following criteria: minor allele frequency (MAF) > 0.05, Hardy–Weinberg Equilibrium (HWE)-p > 0.05, and Tagger r2 < 0.8. Rs2736100 C/A, rs2853677 G/A, rs2735940 A/G, and rs4635969 A/G were selected for follow-up research. Genomic DNA was extracted using a DNA extraction kit following the manufacturer’s instructions (GoldMag Co. Ltd., Xi’an, China). The extracted DNA was then stored at −80 °C in an ultra-low temperature freezer. The primers were designed using MassARRAY Assay Design software. SNPs were genotyped using the MassARRAY system (Agena, San Diego, CA). SNP genotypes were identified using iPLEX chemistry. MALDI-TOF was used to obtain mass peak profiles for various reactions, and genotyping was subsequently successfully completed.
Statistical analysis
Baseline features of the subjects were analyzed using SPSS version 21.0 (SPSS, Chicago, IL). Among them, age and gender were analyzed by t-test and χ2 test, respectively. The genotype frequencies of all SNPs were obtained using a Chi-square test to determine if they satisfied HWE. Odds ratios (ORs) and 95% confidence intervals (95% CIs) were calculated using logistic regression analysis, considering different genetic models, to evaluate the association between all SNPs and the risk of CKD. Multiple genetic models were evaluated using PLINK version 1.9 (Massachusetts, USA), with wild-type alleles serving as the reference. Moreover, the results were analyzed using the false positive reporting probability (FPRP) test to determine if they warranted attention. The multifactorial dimensionality reduction (MDR) method was used to assess the interaction of four SNPs with the risk of CKD. Discrepancies in clinical features among subjects with diverse genotypes were compared using one-way analysis of variance (ANOVA).
Results
The basic characteristics of study participants
Baseline features of the control and case groups are presented in . There were 1017 unrelated subjects in our research, including 507 CKD patients with a mean age of 49.40 ± 16.76 years and 510 healthy individuals with a mean age of 49.40 ± 16.09 years. The proportion of females (38%) was equal in both groups. The mean age (p = 0.999) and gender distribution (p = 0.941) of the two groups were exactly matched. Moreover, the levels of microalbuminuria (MAU), uric acid (UA), creatinine (Cr), and urea were significantly higher in patients with CKD compared to the control group.
Table 1. Characteristics of patients with chronic kidney disease and healthy individuals.
Genotyping information
Detailed genotyping information for all SNPs is provided in . The genotypes of four SNPs were in accordance with HWE (p > 0.05), and MAF was greater than 5% in the study population. Four SNPs were predicted by HaploReg to be regulated by Motifs changed, NHGRI/EBI GWAS hit, Promoter histone marks, Enhancer histone marks, and GRASP QTL hits. Moreover, we discovered discrepancies in the genotype frequency and allele frequency of rs2735940 (p = 0.008 and p = 0.002, respectively), as well as the allele frequency of rs4635969 (p = 0.005), between CKD patients and healthy controls ().
Figure 1. Comparisons of the genotype frequency and allele frequency of SNPs in the TERT gene between the CKD case and healthy control groups. (a–d) Comparisons of the genotype frequencies of rs2736100, rs2853677, rs2735940, and rs4635969. (e–h) Comparisons of the allele frequencies of rs2736100, rs2853677, rs2735940, and rs4635969. ‘*’ indicates statistical significance at the 0.05 level.
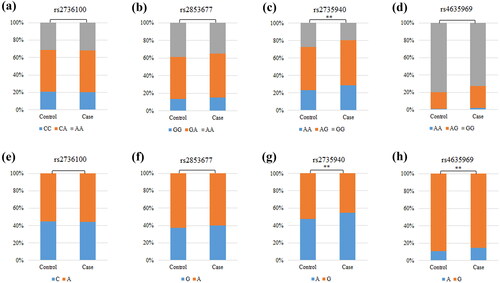
Table 2. The basic information and HWE about the selected SNPs.
Correlation between TERT gene variants and susceptibility to CKD
The correlation between four SNPs and susceptibility to CKD is presented in . The results indicated that rs2735940 and rs4635969 were significantly associated with susceptibility to CKD. Specifically, rs2735940 was significantly associated with an increased risk of CKD under the following models: allele (A vs. G: OR = 1.31, 95% CI 1.10–1.56, p = 0.002), heterozygote (AG vs. GG: OR = 1.44, 95% CI 1.06–1.97, p = 0.021), homozygote (AA vs. GG: OR = 1.74, 95% CI 1.22–2.48, p = 0.002), dominant (AG-AA vs. GG: OR = 1.54, 95% CI 1.15–2.06, p = 0.004), recessive (AA vs. GG-AG: OR = 1.35, 95% CI 1.02–1.80, p = 0.036), and log-additive (OR = 1.32, 95% CI 1.10–1.57, p = 0.002) models. Similarly, rs4635969 was notably linked to an increased risk of CKD under the allele (A vs. G: OR = 1.46, 95% CI 1.12–1.90, p = 0.005), codominant (AG vs. GG: OR = 1.40, 95% CI 1.04–1.88, p = 0.028), dominant (AG-AA vs. GG: OR = 1.46, 95% CI 1.09–1.95, p = 0.011), and log-additive (OR = 1.46, 95% CI 1.12–1.91, p = 0.005) models.
Correlation between TERT gene variants and the risk of CKD in different stratifications
The correlation between four SNPs and CKD risk stratified by age and gender is listed in . We discovered that rs2735940 is linked to an increased risk of CKD in both subjects aged ≤ 50 years (allele: OR = 1.29, 95% CI 1.00–1.67, p = 0.046; codominant: OR = 1.68, 95% CI 1.00–2.81, p = 0.048; and log-additive: OR = 1.30, 95% CI 1.00–1.68, p = 0.048) and subjects aged > 50 years (allele: OR = 1.32, 95% CI 1.04–1.69, p = 0.023; codominant: OR = 1.82, 95% CI 1.11–2.99, p = 0.018; dominant: OR = 1.63, 95% CI 1.07–2.47, p = 0.023; and log-additive: OR = 1.34, 95% CI 1.05–1.71, p = 0.020). And it was also linked to an increased risk of CKD in both male participants (allele: OR = 1.27, 95% CI 1.02–1.59, p = 0.035; heterozygote: OR = 1.57, 95% CI 1.05–2.33, p = 0.027; homozygote: OR = 1.64, 95% CI 1.05–2.56, p = 0.031; dominant: OR = 1.59, 95% CI 1.09–2.32, p = 0.015; and log-additive: OR = 1.27, 95% CI 1.02–1.59, p = 0.036) and female participants (allele: OR = 1.38, 95% CI 1.04–1.82, p = 0.026; codominant: OR = 1.98, 95% CI 1.10–3.56, p = 0.023; recessive: OR = 1.67, 95% CI 1.04–2.68, p = 0.033; and log-additive: OR = 1.41, 95% CI 1.05–1.89, p = 0.023). Furthermore, rs4635969 was tied to an increased risk of CKD in individuals aged ≤ 50 years under a variety of genetic models (allele: OR = 1.62, 95% CI 1.09–2.40, p = 0.016; dominant: OR = 1.59, 95% CI 1.03–2.46, p = 0.036; and log-additive: OR = 1.59, 95% CI 1.08–2.36, p = 0.020). And it was also a risk factor in male participants (allele: OR = 1.61, 95% CI 1.14–2.28, p = 0.006; codominant: OR = 1.55, 95% CI 1.05–2.27, p = 0.027; dominant: OR = 1.63, 95% CI 1.12–2.37, p = 0.012; and log-additive: OR = 1.62, 95% CI 1.15–2.30, p = 0.006). When stratified by CKD stage, it was observed that there was no significant correlation between TERT gene variants and the susceptibility to CKD (Table S1).
Table 3. The SNPs associated with susceptibility to chronic kidney disease in the subgroup tests (age and gender).
FPRP results
The results of FPRP analysis can be found in Table S2. The FPRP values were all less than 0.2 at a prior probability level of 0.25, indicating that the positive results were noteworthy. The overall analysis findings were entirely noteworthy and the stratification analysis findings were almost noteworthy at a prior probability level of 0.25 and an FPRP value of 0.2.
MDR results
The results of MDR analysis were carried out to evaluate SNP-SNP interactions (). The blue lines in the dendrogram indicate a strong redundant effect of rs4635969, rs2853677, and rs2735940 on the risk of CKD. Details of SNP-SNP interactions are listed in . We discovered that the best prediction model was the two-site model: rs2735940 and rs4635969 (the largest CVC: 10/10, with the largest testing balanced accuracy of 0.559 (p < 0.0001).
Figure 3. Dendrogram of SNP-SNP interactions. The colors in the tree diagram represent either synergy or redundancy.
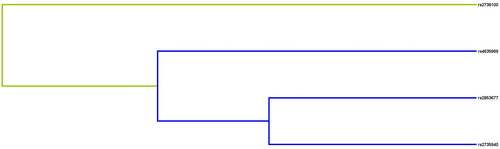
Table 4. SNP–SNP interaction models analyzed by the MDR method.
The correlation of TERT gene variants with clinical indicators
The correlation between TERT gene variants and clinical indicators of CKD patients was conducted, as presented in . Compared to the GA genotype, individuals with the rs2735940 AA/GG genotype were found to have a higher level of MAU (p = 0.023). There was no relevance between the remaining three variants and the levels of FPG, MAU, LDL, UA, Cr, and urea (p > 0.05).
Table 5. Clinical characteristics of patients (N = 507) based on the genotypes of selected SNPs.
Discussion
In this study, we examined the association between four SNPs in the TERT gene and the risk of CKD in a Chinese population. The results showed that rs2735940 and rs4635969 were associated with an increased risk of CKD. This study is the first and largest case-control study to date on four SNPs of TERT and their association with the risk of developing CKD.
Increasing evidence has shown that TERT gene variants are associated with the risk of developing, surviving, and prognosing various cancers [Citation22–24]. However, there are relatively few studies on the effect of TERT gene variants on the risk of CKD. Although this association signal was reported in a recent association study, it included only one SNP of TERT, rs2736100 [Citation20]. Interestingly, in this study, we did not identify this signal. The variation in the results of the aforementioned studies could be attributed to several factors, including the limited impact of this genetic variants on CKD, the relatively small sample size in each study, and the heterogeneity of the population [Citation25]. The role of TERT variants in CKD is not yet well understood. Therefore, the aim of this study was to investigate the association between TERT gene polymorphisms and susceptibility to CKD. Our study demonstrated that rs2735940 and rs4635969 were associated with an increased risk of CKD. Our study is the first to reveal a connection between TERT polymorphisms and susceptibility to CKD, providing new insights into the field of nephrology.
There have been numerous studies conducted on rs2735940, with the majority of them concentrating on the correlation between rs2735940 and the susceptibility to different types of cancer. To the best of our knowledge, no studies have reported genetic association studies between the SNP rs2735940 and CKD. However, a study has found that the TERT rs2735940 affects early renal function after transplantation [Citation26]. The study by Sheng et al. has shown that the TERT rs2735940 is a functional SNP both in vitro and in vivo, which may affect TERT mRNA expression by influencing transcriptional activity [Citation27]. Thus, this polymorphism may lead to a reduced expression of TERT. Lower TERT levels could result in shorter telomeres and accelerated cellular aging, making the kidneys more vulnerable to damage and CKD. Thus, the potential influence of rs2735940 on the development of CKD may be attributed to impact on the expression of TERT. The results of our study indicate that individuals with the A, AG, and AA genotypes had a higher risk of developing CKD compared to those with other genotypes. Furthermore, our study discovered that individuals with the A and AG genotype of rs4635969 in TERT are at a heightened risk of developing CKD in comparison to individuals with other genotypes. These findings potentially have implications for early CKD diagnosis, personalized treatment approaches, and preventive measures. Analyzing an individual’s genotype can enable us to predict their likelihood of developing CKD and implement appropriate preventive measures or early treatments. However, it is important to note that these results are preliminary, and further research, including larger cohorts and functional studies, is needed to validate the clinical relevance and applicability of these SNPs in CKD diagnosis. Although there is currently no report on the biological function of rs4635969, we used Haploreg4.2 online software to predict its potential functions and found that rs4635969 could influence the regulation of enhancer histone marks and Motifs changed. Taken above, we speculated that TERT SNPs, especially rs2735940 and rs4635969 may affect gene expression and function, and then contribute to CKD development, which needs further study and confirmation. Moreover, the identification of genetic markers like rs2735940 and rs4635969 in understanding the underlying genetic predispositions to these conditions. This understanding can lead to better risk assessment, early detection, and potentially more targeted therapeutic strategies.
Age could contribute to the progression of CKD [Citation28]. Meanwhile, gender has historically been regarded as an important factor affecting renal function and the progression of CKD [Citation29]. Therefore, we stratified by age and gender to investigate the relationship between TERT variants and susceptibility to CKD. In our study, we found that rs4635969 is associated with an increased risk of CKD in individuals aged ≤ 50 years. The results of Kobayashi et al. have demonstrated that genetic variants are associated with renal function in an elderly Japanese population (mean age 73 years) [Citation30], which is inconsistent with our findings. The evidence above suggests that genetic variants and susceptibility to CKD may be influenced by age. Our study found that rs4635969 was associated with an increased risk of CKD in males. Cumulative evidence from epidemiological and clinical studies has suggested that there may be gender-related differences in the prevalence, course, and severity of CKD [Citation31]. Consistent with our findings, the study by Chung et al. found that genetic variants were associated with the progression of nephropathy in Taiwanese males [Citation32]. A case-control study by Niu et al. showed that genetic variants were associated with an increased risk of nephropathy in males [Citation33]. In contrast to our findings, Sun et al. have reported that variants in the telomerase RNA component (TERC) gene were associated with GN, CKD, and ESRD risk only in females [Citation20]. A plausible explanation for this phenomenon is that estrogen and progesterone can activate the transcription of TERT and TERC genes [Citation34,Citation35], and it is speculated that female sex hormones play a significant role in this process. Therefore, there are gender differences in genetic variants and susceptibility to CKD.
Additionally, studies have shown that MAU is an indicator of kidney damage and a risk factor for the progression of nephropathy, mainly reflects glomerular damage and glomerular permeability to macromolecules [Citation36]. The presence of MAU is indicative of early nephropathy, and both the absolute level and rate of change of MAU are associated with the development and progression of diabetic kidney disease [Citation37]. Our study found that that individuals with the rs2735940 GG genotype was linked to an elevated level of MAU in patients with CKD. Therefore, rs2735940 may be a risk factor for CKD.
However, it is important to note that our study was limited to a Chinese population. The study has found that the TERT variant has a different genotype distribution in Swedish and Chinese populations [Citation38]. The prevalence of primary GN, CKD, and ESRD in China is significantly higher than in European and American countries [Citation39]. Therefore, different genotype distributions of TERT variants may lead to varying susceptibility to CKD. Further studies will be conducted to investigate the association between TERT variants and susceptibility to CKD in different populations, aiming to provide a more precise understanding of this possibility. Despite the above limitation, our study is the first to reveal a connection between TERT variants and susceptibility to CKD, providing new insights into the field of nephrology.
Conclusion
In summary, this study demonstrated that rs2735940 and rs4635969 in the TERT gene variants were significantly associated with an increased susceptibility to CKD in the Chinese population. Our findings could potentially change nephrologists’ understanding of disease susceptibility and offer new strategies for potential risk assessment, prevention, and early intervention.
Acknowledgments
We are grateful to the individuals for their participation in this study. We also thank the First Affiliated Hospital of Xi’an Jiaotong University to provide samples in this study.
Ethical approval and consent to participation
This study fully followed the principles of the Declaration of Helsinki and was approved by the Ethics Committee of the First Affiliated Hospital of Hainan Medical College. Informed consent from all participants was obtained.
Authorship contribution statement
Yan Su, Yuan Feng, and Xinran Lin wrote the article; Yan Su, Yuan Feng, and Xinran Lin analyzed and interpreted the data; Chunyang Ma and Jiali Wei conceived and designed the experiments; Yan Su, Yuan Feng, and Xinran Lin performed the experiments. All authors reviewed the manuscript.
Supplemental Material
Download PDF (190.6 KB)Disclosure statement
The authors report there are no competing interests to declare.
Data availability statement
The original contributions or analyzed during this study are available from the article. Further inquiries can be made to the corresponding author.
Additional information
Funding
References
- Luan ZL, Zhang C, Ming WH, et al. Nuclear receptors in renal health and disease. EBioMedicine. 2022;76:1. doi:10.1016/j.ebiom.2022.103855.
- Levey AS, Becker C, Inker LA. Glomerular filtration rate and albuminuria for detection and staging of acute and chronic kidney disease in adults: a systematic review. JAMA. 2015;313(8):837–10. doi:10.1001/jama.2015.0602.
- Zhang QL, Rothenbacher D. Prevalence of chronic kidney disease in population-based studies: systematic review. BMC Public Health. 2008;8(1):117. doi:10.1186/1471-2458-8-117.
- Foreman KJ, Marquez N, Dolgert A, et al. Forecasting life expectancy, years of life lost, and all-cause and cause-specific mortality for 250 causes of death: reference and alternative scenarios for 2016-40 for 195 countries and territories. Lancet. 2018;392(10159):2052–2090. doi:10.1016/S0140-6736(18)31694-5.
- Bash LD, Astor BC, Coresh J. Risk of incident ESRD: a comprehensive look at cardiovascular risk factors and 17 years of follow-up in the atherosclerosis risk in communities (ARIC) study. Am J Kidney Dis. 2010;55(1):31–41. doi:10.1053/j.ajkd.2009.09.006.
- Go AS, Chertow GM, Fan D, et al. Chronic kidney disease and the risks of death, cardiovascular events, and hospitalization. N Engl J Med. 2004;351(13):1296–1305. doi:10.1056/NEJMoa041031.
- Balakumar P, Chakkarwar VA, Kumar V, et al. Experimental models for nephropathy. J Renin Angiotensin Aldosterone Syst. 2008;9(4):189–195. doi:10.1177/1470320308098343.
- Kastarinen M, Juutilainen A, Kastarinen H, et al. Risk factors for end-stage renal disease in a community-based population: 26-year follow-up of 25,821 men and women in Eastern Finland. J Intern Med. 2010;267(6):612–620. doi:10.1111/j.1365-2796.2009.02197.x.
- Köttgen A. Genome-wide association studies in nephrology research. Am J Kidney Dis. 2010;56(4):743–758. doi:10.1053/j.ajkd.2010.05.018.
- Ding D, Zhou J, Wang M, et al. Implications of telomere-independent activities of telomerase reverse transcriptase in human cancer. FEBS J. 2013;280(14):3205–3211. doi:10.1111/febs.12258.
- Blackburn EH. Telomeres and telomerase: the means to the end (nobel lecture). Angew Chem Int Ed Engl. 2010;49(41):7405–7421. doi:10.1002/anie.201002387.
- Willeit P, Willeit J, Mayr A, et al. Telomere length and risk of incident cancer and cancer mortality. Jama. 2010;304(1):69–75. doi:10.1001/jama.2010.897.
- Mazidi M, Rezaie P, Covic A, et al. Telomere attrition, kidney function, and prevalent chronic kidney disease in the United States. Oncotarget. 2017;8(46):80175–80181. doi:10.18632/oncotarget.20706.
- Ameh OI, Okpechi IG, Dandara C, et al. Association between telomere length, chronic kidney disease, and renal traits: a systematic review. OMICS. 2017;21(3):143–155. doi:10.1089/omi.2016.0180.
- Long Q, Hua Y, He L, et al. Poly(U) binding splicing factor 60 promotes renal cell carcinoma growth by transcriptionally upregulating telomerase reverse transcriptase. Int J Biol Sci. 2020;16(15):3002–3017. doi:10.7150/ijbs.45115.
- Ma R, Liu C, Lu M, et al. The TERT locus genotypes of rs2736100-CC/CA and rs2736098-AA predict shorter survival in renal cell carcinoma. Urol Oncol. 2019;37(5):301.e1–301.e10. doi:10.1016/j.urolonc.2019.01.014.
- Casuscelli J, Becerra MF, Manley BJ, et al. Characterization and impact of TERT promoter region mutations on clinical outcome in renal cell carcinoma. Eur Urol Focus. 2019;5(4):642–649. doi:10.1016/j.euf.2017.09.008.
- Lin Y, Yin P, Zhu Z, et al. Epigenome-wide association study and network analysis for IgA nephropathy from CD19(+) B-cell in chinese population. Epigenetics. 2021;16(12):1283–1294. doi:10.1080/15592294.2020.1861171.
- Han Q, Geng W, Zhang D, et al. ADIPOQ rs2241766 gene polymorphism and predisposition to diabetic kidney disease. J Diabetes Res. 2020;2020:5158497–5158498. doi:10.1155/2020/5158497.
- Sun Q, Liu J, Cheng G, et al. The telomerase gene polymorphisms, but not telomere length, increase susceptibility to primary glomerulonephritis/end stage renal diseases in females. J Transl Med. 2020;18(1):184. doi:10.1186/s12967-020-02347-3.
- Tomson CRV, Cheung AK, Mann JFE, et al. Management of blood pressure in patients with chronic kidney disease not receiving dialysis: synopsis of the 2021 KDIGO clinical practice guideline. Ann Intern Med. 2021;174(9):1270–1281. doi:10.7326/M21-0834.
- de Martino M, Taus C, Lucca I, et al. Association of human telomerase reverse transcriptase gene polymorphisms, serum levels, and telomere length with renal cell carcinoma risk and pathology. Mol Carcinog. 2016;55(10):1458–1466. doi:10.1002/mc.22388.
- Singh V, Jaiswal PK, Mittal RD. Replicative study of GWAS TP63C/T, TERTC/T, and SLC14A1C/T with susceptibility to bladder cancer in North indians. Urol Oncol. 2014;32(8):1209–1214. doi:10.1016/j.urolonc.2014.05.013.
- Dratwa M, Wysoczańska B, Łacina P, et al. TERT-Regulation and roles in cancer formation. Front Immunol. 2020;11:589929. doi:10.3389/fimmu.2020.589929.
- Chang WW, Su H, He L, et al. Association between transforming growth factor-beta1 T869C polymorphism and rheumatoid arthritis: a meta-analysis. Rheumatology (Oxford). 2010;49(4):652–656. doi:10.1093/rheumatology/kep417.
- Kłoda K, Domanski L, Kwiatkowska E, et al. hTERT, BICD1 and chromosome 18 polymorphisms associated with telomere length affect kidney allograft function after transplantation. Kidney Blood Press Res. 2015;40(2):111–120. doi:10.1159/000368487.
- Sheng X, Tong N, Tao G, et al. TERT polymorphisms modify the risk of acute lymphoblastic leukemia in chinese children. Carcinogenesis. 2013;34(1):228–235. doi:10.1093/carcin/bgs325.
- Gnanasambandan R, Elumalai R, Soundararajan P, et al. Angiotensinogen gene polymorphisms and progression of chronic kidney disease in ADPKD patients. Clin Exp Nephrol. 2016;20(4):561–568. doi:10.1007/s10157-015-1183-2.
- Cobo G, Hecking M, Port FK, et al. Sex and gender differences in chronic kidney disease: progression to end-stage renal disease and haemodialysis. Clin Sci (Lond). 2016;130(14):1147–1163. doi:10.1042/CS20160047.
- Kobayashi H, Otsuka H, Yanai M, et al. Adiponectin receptor gene polymorphisms are associated with kidney function in elderly Japanese populations. J Atheroscler Thromb. 2019;26(4):328–339. doi:10.5551/jat.45609.
- Mills KT, Xu Y, Zhang W, et al. A systematic analysis of worldwide population-based data on the global burden of chronic kidney disease in 2010. Kidney Int. 2015;88(5):950–957. doi:10.1038/ki.2015.230.
- Chung HF, Long KZ, Hsu CC, et al. Adiponectin gene (ADIPOQ) polymorphisms correlate with the progression of nephropathy in Taiwanese male patients with type 2 diabetes. Diabetes Res Clin Pract. 2014;105(2):261–270. doi:10.1016/j.diabres.2014.04.015.
- Niu D, Gao Y, Xie L, et al. Genetic polymorphisms in TNFSF13 and FDX1 are associated with IgA nephropathy in the han Chinese population. Hum Immunol. 2015;76(11):831–835. doi:10.1016/j.humimm.2015.09.044.
- Misiti S, Nanni S, Fontemaggi G, et al. Induction of hTERT expression and telomerase activity by estrogens in human ovary epithelium cells. Mol Cell Biol. 2000;20(11):3764–3771. doi:10.1128/MCB.20.11.3764-3771.2000.
- Vidal JD, Register TC, Gupta M, et al. Estrogen replacement therapy induces telomerase RNA expression in the macaque endometrium. Fertil Steril. 2002;77(3):601–608. doi:10.1016/s0015-0282(01)03227-7.
- Liu R, Li G, Cui XF, et al. Methodological evaluation and comparison of five urinary albumin measurements. J Clin Lab Anal. 2011;25(5):324–329. doi:10.1002/jcla.20477.
- MacIsaac RJ, Ekinci EI, Jerums G. Progressive diabetic nephropathy. How useful is microalbuminuria: contra. Kidney Int. 2014;86(1):50–57. doi:10.1038/ki.2014.98.
- Dahlström J, Liu T, Yuan X, et al. TERT rs2736100 genotypes are associated with differential risk of myeloproliferative neoplasms in Swedish and Chinese male patient populations. Ann Hematol. 2016;95(11):1825–1832. doi:10.1007/s00277-016-2787-7.
- Liu ZH. Nephrology in China. Nat Rev Nephrol. 2013;9(9):523–528. doi:10.1038/nrneph.2013.146.