Abstract
Nanostructures composed of liposomes and polydopamine (PDA) have demonstrated efficacy as carriers for delivering plasmids, effectively alleviating renal cell carcinoma. However, their role in acute kidney injury (AKI) remains unclear. This study aimed to investigate the effects of the plasmid-encoded lncRNA-OIP5-AS1@PDA nanoparticles (POP-NPs) on renal ischemia/reperfusion (RI/R) injury and explore the underlying mechanisms. RI/R or OGD/R models were established in mice and HK-2 cells, respectively. In vivo, vector or POP-NPs were administered (10 nmol, IV) 48 h after RI/R treatment. In the RI/R mouse model, the OIP5-AS1 and Nrf2/HO-1 expressions were down-regulated, while miR-410-3p expression was upregulated. POP-NPs treatment effectively reversed RI/R-induced renal tissue injury, restoring altered levels of blood urea nitrogen, creatinine, malondialdehyde, inflammatory factors (IL-8, IL-6, TNF-α), ROS, apoptosis, miR-410-3p, as well as the suppressed expression of SOD and Nrf2/HO-1 in the model mice. Similar results were obtained in cell models treated with POP-NPs. Additionally, miR-410-3p mimics could reverse the effects of POP-NPs on cellular models, partially counteracted by Nrf2 agonists. The binding relationship between OIP5-AS1 and miR-410-3p, alongside miR-410-3p and Nrf2, has been substantiated by dual-luciferase reporter and RNA pull-down assays. The study revealed that POP-NPs can attenuate RI/R-induced injury through miR-410-3p/Nrf2 axis. These findings lay the groundwork for future targeted therapeutic approaches utilizing nanoparticles for RI/R-induced AKI.
Introduction
Acute kidney injury (AKI) is a severe clinical syndrome that develops in approximately 60% of critically ill patients [Citation1,Citation2]. It is characterized by a rapid progression and has a high mortality rate. In recent years, there has been a rise in the incidence of AKI [Citation3]. One of the major causes of AKI is renal ischemia/reperfusion (RI/R) injury, which occurs due to renal vascular occlusion and renal transplantation [Citation4,Citation5]. The progression of AKI can be accelerated by abnormal apoptosis and oxidative stress [Citation6]. Inhibition of PGC-1α/Nuclear factor erythroid2-related factor 2 (Nrf2)/heme oxygenase-1 (HO-1) expression exacerbates renal tubular cell apoptosis and thus RI/R [Citation7]. Although, in recent years, some progress has been made in the study of AKI. For example, dihydromyricetin attenuated cisplatin-induced AKI by reducing oxidative stress and inflammation [Citation8]. The pathophysiologic mechanisms of RI/R injury have not been fully elucidated. Therefore, identifying molecular markers and targets for the early diagnosis of RI/R injury holds great significance in its prevention and early detection.
Nrf2 is widely recognized as a crucial endogenous regulator of antioxidative stress [Citation9]. Nrf2 activates the expression of HO-1, which helps alleviate oxidative stress and inflammation [Citation10]. Recent studies have demonstrated that the Nrf2 pathway plays a significant protective role in I/R injury of the heart, brain, and intestines [Citation11–13]. Similarly, activation of NRF2 can alleviate RI/R injury through HO-1 [Citation14]. MicroRNAs (miRNAs) can directly regulate the transcriptional expression of Nrf2 [Citation15]. A recent study showed that miR-200a attenuates sodium dextran sulfate-induced colitis by activating Nrf2 [Citation16]. Nevertheless, further investigation is needed to identify the specific miRNA that regulates Nrf2/HO-1 in RI/R.
Non-coding RNAs (ncRNA), mainly including Long ncRNA (lncRNA) and miRNA, may be related to the pathogenesis of AKI [Citation17]. Recent studies have shown that certain miRNAs can target both coding and ncRNA molecules, depending on the cellular environment. These ncRNAs are also referred to as competitive endogenous RNAs (ceRNAs) [Citation18,Citation19]. In the context of AKI following kidney transplantation, lncRNAs can act as ceRNAs to regulate the expression of specific proteins [Citation20]. However, the exploration of lncRNAs with potential protective roles in AKI is currently limited due to challenges associated with their synthesis, such as their lengthy nature and poor stability.
The recent approval of lipid nanoparticle delivery for RNA has established it as a potent therapeutic strategy, applicable to a broad spectrum of clinical uses [Citation21,Citation22]. Polydopamine (PDA), a derivative of dopamine that can polymerize itself, has gained momentum in biomedical applications [Citation23]. Mao et al. demonstrated that nanostructures combining liposomes and PDA as carriers can effectively deliver plasmids to alleviate renal cell carcinoma [Citation21]. Zheng et al. revealed that PDA@MF NPs (manganese ferrite nanoparticles wrapped with PDA) effectively reduced oxidative stress in vivo, mitigated renal inflammatory events, and enhanced renal function [Citation23]. Building on these findings, this study explored the effects of the plasmid-encoded lncRNA-OIP5-AS1@PDA nanoparticles (POP-NPs) mediated ceRNA network in RI/R injury. Our study lays the theory for developing new nanoparticle-related targeted therapeutic approaches for RI/R-induced AKI.
Methods
Construction of animal models
All the C57BL/6 mice (adult male, weight 25–35 g, 8–10 weeks) were provided by Hunan SJA Laboratory Animal Co., Ltd. They were placed in an air filter unit with constant temperature and humidity for a 12 h light/dark cycle and fed standard mouse food and water. Mice were divided into two groups: a RI/R group (n = 8) and a control group (Ctrl, n = 8). For the RI/R mouse model construction, sodium pentobarbital (50 mg/kg) was injected intraperitoneally for anesthesia. The RI/R mouse model was constructed as described in our previous literature report [Citation24]. Mice were sacrificed at reperfusion for 48 h by intraperitoneal injection of sodium pentobarbital (150 mg/kg). Kidney tissues and blood was collected, and its recovery was observed. The animal/cage stayed in the same location throughout the experiment. During the intraoperative and postoperative periods of the experiments on the animals, there were no dead mice in the control group. There were 8 mice in the experimental group, of which 1 died.
Cell culture and transfection
Human kidney-2 (HK-2) cells (AW-CNH063, Abiowell, Changsha, Hunan, China) were incubated in DMEM/F12 (D8437, Sigma) cell culture medium added with 10% fetal bovine serum (FBS, C11108862, Gibco) in a 37 °C incubator (5% CO2). The cells were transfected with miR-410-3p inhibitors (80 nM and 160 nM), miR-410-3p mimics (40 nM and 80 nM), OIP5-AS1 plasmid (pcDNA-OIP5-AS1, 0.1, 0.2, 0.5 μg), silencing OIP5-AS1 plasmid (si-OIP5-AS1, 40, 80, and 160 nM), silencing Nrf2 plasmid (si-Nrf2), and their negative control (NC, NC-1, pc-NC, si-NC, or siRNA-NC) via lipofectamine 2000 (1668030, ThermoFisher) for 48 h following the instructions. The cells were validated by STR profiling and tested negative for mycoplasma.
Oxygen-glucose deprivation and reoxygenation (OGD/R) model
As described previously, the OGD/R model was constructed in vitro [Citation25]. First, HK-2 cells were incubated in a serum-free medium for 12 h to synchronize cell growth. Next, after being washed twice, the cells were placed into a hypoxic incubator (37 °C, 1% O2, and 5% CO2, Thermo Fisher) and incubated in d-glucose and serum deprivation medium for 6 h. Subsequently, the HK-2 cells were transferred to an incubator set at a constant temperature. They were cultured for 12 h in a medium enriched with 10% FBS, all whilst stabilizing conditions were maintained at 37 °C, an oxygen concentration of 21%, and a CO2 concentration of 5%.
Hematoxylin & eosin (H&E) staining
As mentioned in our previous article [Citation24], the kidney tissue was initially fixed. Sections were stained with H&E. The cytoplasm was stained red in contrast to the blue nucleus. Sections were observed under a light microscope (BA210T, Motic).
Biochemical kit testing
The biochemical kits, including blood urea nitrogen (BUN, C013-2-1), serum creatinine (Cre, C011-2-1), superoxide dismutase (SOD, A001-3-2), malondialdehyde (MDA, A003-1-2), alanine aminotransferase (ALT, C009-2-1), and aspartate aminotransferase (AST, C010-2-1) were used to assay BUN, Cre, SOD, MDA, ALT, and AST levels as per the instructions. These kits were purchased from Nanjing Jiancheng Technology Co., Ltd (China).
The levels of the mouse whole blood hematological indices, including red blood cell count (RBC), hemoglobin (Hb), white blood cells (WBC), platelets (PLT), and the percentage of neutrophils (Gran%), were examined utilizing a fully automated blood cell analyzer (Mindray BC-5000, China).
Reverse transcription-quantitative polymerase chain reaction (RT-qPCR)
Total RNA was extracted with TRIzol reagent (15596026, ThermoFisher) and reversely transcribed into cDNA by PCR Amplifier (Bio-Rad). An RT-qPCR experiment was conducted using the ABI7500 quantitative PCR instrument (ABI7500, ThermoFisher). β-actin and U6 were used as the internal references. The relative transcriptional levels were calculated by 2−ΔΔCT. The primer sequences are shown in Table S1.
ROS and apoptosis detection
Tissues were first sheared and digested into cell suspension. DCFH-DA (ROS kit, S0033S, Biotronik) was diluted to 10 uM with serum-free culture medium and then co-incubated with the collected cells for 20 min in an incubator at 37 °C. Cells were added 5 uL Annexin V-APC and 5 uL Propidium Iodide (apoptosis detection kit, KGA1030, KeyGEN BioTECH) and mixed well. Afterward, the cells were incubated at room temperature and shielded from light for 10 min to assess the level of apoptosis. Flow cytometry was used to detect ROS and apoptosis levels.
TdT-mediated dUTP nick end labeling (TUNEL) staining for apoptosis
The tissues were fixed for 24 h, subsequently embedded in paraffin, and then cut into sections (4–5 μm). Following the guidelines provided by the manufacturer, the staining procedure was performed using a TUNEL assay kit (40306ES50, Shanghai Yisheng Biotechnology). Pictures of tissues were observed with a fluorescence microscope (BA410T, Motic). The apoptosis rate was expressed by comparing the number of TUNEL-positive nuclei with the number of DAPI-stained nuclei.
Western blot
The cells or tissues were collected, and the protein concentration was determined. The protein was transferred to the PVDF membrane and blocked with 5% skim milk-TBST. Then, they were cultured with the following primary antibodies at 4 °C overnight, including anti-Nrf2 (1:5000, 16396-1-AP, Proteintech, USA), anti-HO-1 (1:3000, 10701-1-AP, Proteintech, USA), and β-actin (1:5000, 66009-1-Ig, Proteintech, USA). Subsequently, the membrane was incubated with goat anti-rabbit/mouse IgG (1:5000, SA00001-2/SA00001-1, Proteintech, USA). Subsequently, the membrane was conducted using enhanced chemiluminescence (180-506, Tanon™ Femto-sig ECL). The protein level was defined by the gray value rate of target bands to β-actin.
Immunohistochemistry (IHC)
Tissue sections (5 μm) were dewaxed in xylene. Slides were cultured overnight at 4 °C with the primary antibody Nrf2 and HO-1. The percentage of HO-1 and Nrf2 positive areas was measured on the whole cortical surface, and the perivascular areas of large vessels and medulla were excluded. All measurements and quantifications were meticulously performed in a randomized and blinded fashion, leading to the subsequent attainment of the integrated optical density (IOD).
Bioinformatics analysis
We used the GEO database GSE53771 for screening. The GSE53771 database included two miRNA data, which were 18 zero-hour (zero) and 18 selected post-transplant (post) samples from 18 kidney allografts. The R language Limma package was used for miRNA differential analysis in the AKI vs. Control groups. Cutoff was |logFC|>log2 (1.5) & p < .05. The R package ClusterP was utilized to generate heat maps, Venn diagrams, bar diagrams, and co-expression network diagrams alongside KEGG and Gene Ontology (GO) annotation diagrams.
Dual-luciferase reporter assay
The OIP5-AS1 fragment, which contained the predicted miR-410-3p binding site, was embedded into the pmirG10 dual-luciferase miRNA target expression vector (Promega, Madison, Wisconsin) to establish a wild-type reporter gene vector (WT) and mutant (MUT) OIP5-AS1. Following that, cells were cultured into a 96-well plate for 24 h. Once the cells reached 50% to 70% confluence, they were co-transfected with OIP5-AS1-MUT or OIP5-AS1-WT plasmid and pre-miR-ctrl or premiR-410-3p by Lipofectamine 2000 (1668030, ThermoFisher). Dual-luciferase reporter assays (Promega) were used 48 h following transfection to evaluate luciferase activity. The targeting validation of miR-410-5p and Nrf2 was consistent with the above method.
RNA pull-down assay
The biotinylated miR-410-3p probe and the corresponding control (oligo probe) were pre-incubated with Streptavidin-Dyna beads M-280 (Invitrogen) for 2 h at 25 °C. Then, the cell lysate was incubated with the beads at 4 °C overnight. After washing the beads several times with washing buffer, RNA complexes bound to the beads were eluted and extracted with RNAiso Plus (TaKaRa), followed by RT-qPCR.
Synthesis and characterization of plasmid-OIP5-AS1@PDA nanoparticles (POP-NPs)
POP-NPs were synthesized as described previously [Citation21]. In brief, we synthesized plasmid-OIP5-AS1@liposomes by amalgamating plasmid OIP5-AS1 (50 nM) with liposomes (50 ng/L, Yessen, China). The concoction was mixed for 30 s and then vortexed for another 30 s for optimal integration. Plasmid-OIP5-AS1@liposomes were dispersed in Tris-HCl (pH 8.8, 10 mM, 5 mL) solution, and then dopamine hydrochloride (5 mg, Adamas-beta Inc. China) was added and stirred for 3 h to form the PDA. Then, POP-NPs were collected by centrifugation (8000 rpm) for 10 min. Finally, after washing, the POP-NPs were stored at 4 °C. Transmission electron microscopy (TEM, JEM1400, JEOL) characterized the PSPM-NPs. A nanoparticle size analyzer (ZS-90, Malvern Instruments) was used to examine the diameters of the samples.
Processing of POP-NPs on animals
The effects of POP-NPs on the RI/R animal model were further explored. C57BL/6 mice were randomly divided into 4 groups: the Ctrl group (n = 8), the RI/R group (n = 8), the RI/R + Vector group (n = 8), and the RI/R + POP-NPs group (n = 8). After RI/R, Vector or POP-NPs were injected into mice (10 nmol, IV). Mice were executed 48 h after tail vein injection [Citation21,Citation26]. Kidney tissues and blood was collected, and its recovery was observed. During the intraoperative and postoperative periods of the experiments on the animals, there were no dead mice in the control group. There were 24 mice in the experimental group, of which 2 died. No mice succumbed after the administration of the drug.
Biocompatibility experiments of POP-NPs
C57BL/6 normal mice were injected with 200 μl of PBS or PSPM-NPs (10 nmol), respectively. Four days subsequent, the mice were humanely euthanized, following which their hearts, livers, spleens, lungs, and kidneys were carefully extracted for H&E staining.
Cell counting kit-8 (CCK-8) assay
The viability of the cells was determined by the CCK-8 kit (NU679, Dojindo Laboratories). Cells were incubated with 10% CCK-8 at 37 °C for 2 h before being placed in a microplate reader (MB-530, Heales) to obtain optical density (OD450 nm).
Flow Cytometer
The above cells were collected and then double-stained with 5 μL fluorescein isothiocyanate (FITC)-Annexin V and 5 μL propidium iodide by the FITC-Annexin V apoptosis detection kit (BD Biosciences, 556547) for 10 min. Intracellular ROS were detected using a ROS test kit (Merck, MAK143-1KT). Afterward, cells were detected by Flow Cytometer (BD Biosciences), and ROS and apoptosis levels were obtained.
Enzyme-linked immunosorbent assay (ELISA)
The supernatant of the treated macrophage culture medium was collected and centrifuged. Subsequently, the levels of IL-18, IL-6, and TNF-α present in the supernatant were quantified in accordance with the guidelines provided by the ELISA detection kit (Cusabio, CSB-E04638h, CSB-E07450h, and CSB-E04740h).
Statistics analysis
All data represented at least biological replicate experiments and were expressed as the mean ± Standard Deviation (SD). First of all, normality and homogeneity of variance tests were carried out. The test conformed to normal distribution and homogeneity of variance. The data were further evaluated via an unpaired t-test or one-way analysis of variance (ANOVA). Next, post-hoc Tukey’s test was performed. Data was analyzed using GraphPad Prism 8.0 (GraphPad Software, USA). Statistical significance was confirmed when p < .05.
Results
The Nrf2/HO-1 expressions decrease after RI/R
First, the mouse RI/R model was constructed. After RI/R in mice, extensive congestion of kidney tissue was observed (). H&E staining showed vacuolar degeneration and nuclear condensation in the renal tubular epithelial cells, indicating severe renal tubular injury in the RI/R group (). Following RI/R, there was a significant increase in the levels of BUN, serum Cre, MDA, inflammatory factors (IL-8, IL-6, TNF-α), ROS, and tissue apoptosis, whereas the level of SOD in the serum noticeably decreased (). The Nrf2 pathway plays a pivotal role in safeguarding against small intestine and cerebral I/R damage and RI/R injury [Citation27–29]. The RI/R group showed lower Nrf2/HO-1 expression levels than the Ctrl group (). The above results demonstrate the successful construction of the RI/R model, with a significant downregulation of Nrf2/HO-1 expression levels observed in the RI/R group.
Figure 1. The Nrf2/HO-1 expressions decrease after RI/R. (A) Representative images of mouse kidney tissue. (B) Representative images of H&E staining. (C,D) Representative bar graphs of BUN and Cre levels detected through ELISA. (E) Representative bar graphs of SOD and MDA levels in serum detected using biochemical assay kits. (F) The representative bar chart of the concentrations of inflammatory factors IL-8, IL-6, and TNF-α was detected by RT-qPCR. (G) The ROS levels were detected using a ROS assay kit. (H) The representative image and quantification analysis of renal tissue apoptosis levels. (I,J) Representative images of the Nrf2/HO-1 gene and protein expression were determined by RT-qPCR and western blot, respectively. (K,L) The representative images showcasing the expressions of Nrf2/HO-1 and their quantitative statistics were gathered through IHC. n = 7-8 mice/group, Student’s t test, *p < 0.05, vs the Ctrl.
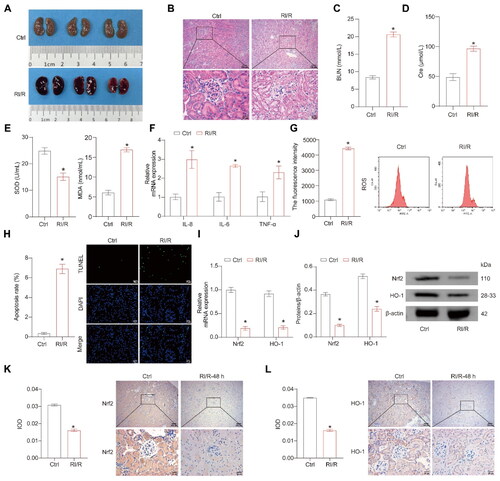
miR-410-3p may regulate Nrf2 through bioinformatics analysis
We used bioinformatics analysis to determine the miRNA that plays a regulatory role in Nrf2/HO-1. The volcano map showed all upregulated and downregulated miRNAs in both data sets (). There were 22 miRNAs upregulated in post-TX biopsies and only two in zero-hour biopsies. Only miR-410-3p was in the co-upregulated miRNAs in the two data (). miR-410-3p was notably overexpressed in both databases, as depicted in . showed miRNAs that might target Nrf2, including miR-410-3p. miR-410-3p might be associated with signaling pathways regulating pluripotency of stem cells, PI3K-Akt signaling pathway, proximal promoter sequence-specific DNA binding, RNA polymerase II proximal promoter sequence-specific DNA binding pathways, etc. ().
Figure 2. miR-410-3p may regulate Nrf2 through biological analysis. (A and B) Volcanic map with differential expression of miRNAs. (C) Venn diagram of highly differentially expressed miRNA. (D and E) Box diagram of miR-410-3p expressed. (F) Co-expression network diagram of Nrf2 and miRNAs. (G) Enrichment analysis of Nrf2 pathway through GO and KEGG. Student’s t test, *p < .05.
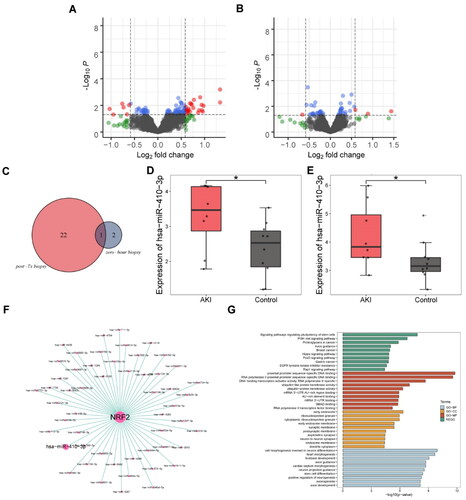
miR-410-3p directly targets Nrf2 mRNA and inhibits Nrf2/HO-1 expression
Next, an in vitro cell injury model induced by OGD/R was constructed. After OGD/R, the levels of apoptosis rate, ROS, inflammatory factors, and MDA in the cells significantly increased, while the level of SOD significantly decreased (). The expression of miR-410-3p was significantly upregulated in the OGD/R group (). Next, we tested the transfection effects of miR-410-3p inhibitors and mimics at different concentrations. The expression of miR-410-3p decreased gradually with the dose of inhibitor but increased gradually with the concentration of mimics (). High-concentration inhibitors and mimics with better transfection efficiency were selected for the following experiments. We predicted the binding sites for miR-410-3p and Nrf2 mRNA to determine whether miR-410-3p could directly inhibit Nrf2. showed that miR-410-3p might bind directly to the 3'UTR region of Nrf2. Meanwhile, luciferase activity decreased when miR-410-3p and Nrf2 WT were co-transfected. In contrast, the transfection of miR-410-3p and Nrf2-MUT did not affect luciferase activity (), indicating that Nrf2 could bind to miR-410-3p directly. RNA pull-down assay further confirmed that Nrf2 could bind to miR-410-3p (). The inhibitor of miR-410-3p upregulated Nrf2/HO-1 expression levels, while Nrf2/HO-1 expression levels were down-regulated when overexpressing miR-410-3p (). The results reveal that miR-410-3p can inhibit Nrf2/HO-1 expressions by targeting Nrf2.
Figure 3. miR-410-3p targets Nrf2 mRNA and inhibits Nrf2/HO-1 expressions. Cells were treated with OGD/R or transfected with inhibitors, mimics, and their empty vector (NC or NC-1). (A and B) Representative images of cell apoptosis and ROS levels. (C) The concentration of inflammatory factors in the cell supernatant was detected by ELISA. (D) The levels of SOD and MDA in the cells were measured using biochemical assay kits. (E) RT-qPCR analysis of miR-410-3p expression level. (F) Different miR-410-3p inhibitors or mimics were transfected into HK-2 cells, and Nrf2/HO-1 expressions were measured via RT-qPCR. (G) Prediction of Nrf2 mRNA and miR-410-3p binding site. (H) Fluorescence activity was detected after co-transfection of Nrf2-WT or Nrf2-MUT and miR-410-3p or NC. (I) RNA pull-down assay. (J) Inhibitors or mimics of miR-410-3p were transfected into HK-2 cells, and Nrf2/HO-1 expression was measured using RT-qPCR or western blot. Student’s t test or ANOVA, *p < .05, vs. the Ctrl or NC #p < .05, vs. the NC-1.
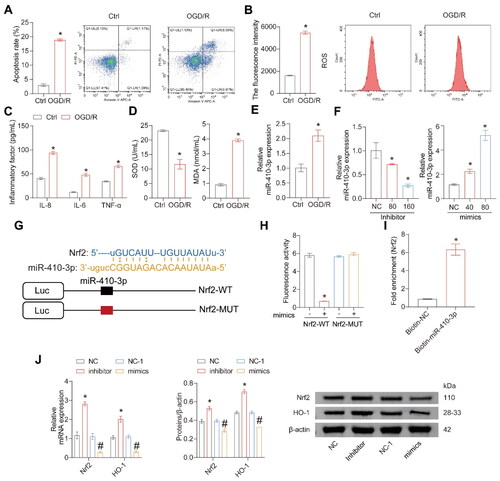
miR-410-3p inhibitor alleviates OGD/R-induced oxidative stress damage through the Nrf2/HO-1 axis
Next, we investigated the effects of miR-410-3p and the Nrf2/HO-1 axis on OGD/R-induced oxidative stress damage. Cells were transfected with si-Nrf2, resulting in a significant decrease in the expression of Nrf2 (). Compared to the OGD/R + NC + siRNA-NC group, the levels of apoptosis rate, ROS, inflammatory factors, and MDA significantly decreased, while the level of SOD significantly increased in the OGD/R + inhibitor + siRNA-NC group. The trend of the above indicators in the OGD/R group cells transfected with si-Nrf2 and inhibitor is the opposite. Moreover, the above indicators of the cells in the OGD/R group transfected with si-Nrf2 and inhibitor simultaneously reversed the changes observed in OGD/R + inhibitor + siRNA-NC group cells (). The expression of miR-410-3p was lower in the OGD/R + inhibitor + siRNA-NC and OGD/R + inhibitor + si-Nrf2 groups compared to the other two groups. After transfection with the inhibitor, the Nrf2/HO-1 pathway was activated in the OGD/R group (). The above results suggest that miR-410-3p inhibitor can alleviate OGD/R-induced oxidative stress damage through the Nrf2/HO-1 axis.
Figure 4. miR-410-3p inhibitor alleviates OGD/R-induced oxidative stress damage through the Nrf2/HO-1 axis. The cells were transfected with inhibitor and si-Nrf2 empty vector (NC and siRNA-NC), inhibitor and siRNA-NC, NC and si-Nrf2, or inhibitor and si-Nrf2, and then subjected to OGD/R treatment. (A) Nrf2 expression was detected using a western blot. (B and C) Representative images of cell apoptosis and ROS levels. (D) The concentration of inflammatory factors in the cell supernatant was detected using ELISA. (E) The levels of SOD and MDA in the cells were detected using biochemical assay kits. (F) RT-qPCR analysis of miR-410-3p, Nrf2, and HO-1 expression levels. (G) Western blot analysis of Nrf2 and HO-1 expression levels. ANOVA, *p < .05, vs. the OGD/R + NC + siRNA-NC, #p < .05, vs. the OGD/R + NC + si-Nrf2.
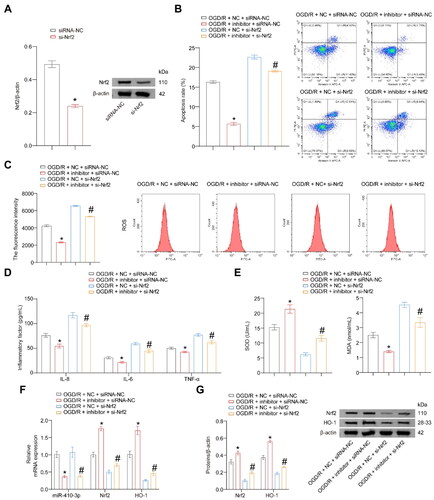
OIP5-AS1 binds to miR-410-3p directly
OIP5-AS1 can alleviate myocardial I/R and cerebral hypoxic-ischemia-induced injury [Citation30,Citation31]. The expression level of OIP5-AS1 was also significantly down-regulated after OGD/R (). OIP5-AS1 affects glioma progression by targeting miR-410-3p [Citation32]. However, it has not been reported in RI/R. We hypothesized if OIP5-AS1 could mediate the ceRNA regulatory mechanism, thus affecting the expressions of Nrf2/HO-1. We predicted the binding sites of OIP5-AS1 and miR-410-3p in database 3.0. The binding sequence of OIP5-AS1 and miR-410-3p are shown in . The luciferase activity decreased when OIP5-AS1-WT and miR-410-3p were co-transfected. In contrast, the transfection of OIP5-AS1-MUT and miR-410-3p did not affect luciferase activity, implying that OIP5-AS1 could bind to miR-410-3p directly (). A transfection experiment was conducted at various concentrations with OIP5-AS1 overexpression and silence. The expression of OIP5-AS1 was increased after transfection of the overexpressed plasmid but was decreased after transfection of the silencing plasmid (). RNA pull-down assay further confirmed that OIP5-AS1 could bind to miR-410-3p (). The expression of miR-410-3p declined when OIP5-AS1 was overexpressed, but the miR-410-3p expression was raised when OIP5-AS1 was knocked down (). The above results illustrate that OIP5-AS1 can directly bind to miR-410-3p. miR-410-3p and OIP5-AS1 were overexpressed to further prove whether OIP5-AS1 could regulate Nrf2/HO-1 expression levels through miR-410-3p. Transfection of mimics reversed the attenuation of Nrf2/HO-1 expression level induced by overexpression of OIP5-AS1 (). miR-410-3p can directly target Nrf2 mRNA and suppress Nrf2 expression, and the process may depend on the ceRNA pathway mediated by OIP5-AS1.
Figure 5. OIP5-AS1 binds to miR-410-3p directly. The cells were treated with OGD/R or transfected with pcDNA-OIP5-AS1, si-OIP5-AS1, pcDNA-OIP5-AS1 combined with mimics empty vector (NC-1 and pc-NC), mimics combined with pc-NC, pcDNA-OIP5-AS1 combined with NC-1, and pcDNA-OIP5-AS1 combined with mimics, respectively. (A) RT-qPCR analysis of miR-410-3p expression level. (B) Predicted binding sequences and alignment of OIP5-AS1 and miR-410-3p. (C) Fluorescence activity was measured after co-transfection of OIP5-AS1-WT or OIP5-AS1-MUT and miR-410-3p or NC-1. (D) Different amounts of OIP5-AS1 or siRNA were transfected into HK-2 cells, and OIP5-AS1 expression was measured via RT-qPCR. (E) RNA pull-down assay. (F–H) miR-410-3p mimics/NC and OIP5-AS1 were co-transfected in HK-2 cells, and Nrf2/HO-1 levels were measured using RT-qPCR and western blot, respectively. ANOVA, *p < .05, vs. the Ctrl, lnc-WT, or NC + pc-NC, #p < .05, vs. the NC-1 + pcDNA-OIP5-AS1.
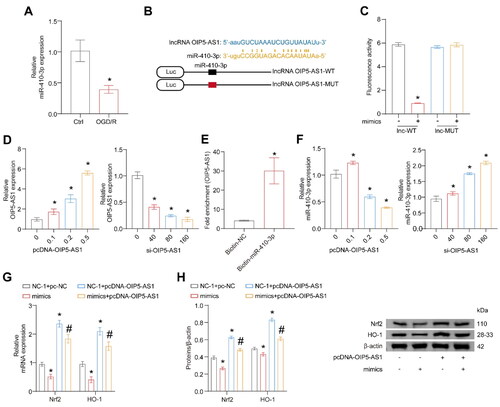
POP-NPs suppress oxidative stress injury in the OGD/R group through the miR-410-3p/Nrf2/HO-1 axis
Gene transfer using NPs as carriers is a promising direction for the future treatment of AKI [Citation23,Citation33]. Mao et al. demonstrated that nanostructures incorporating liposomes and PDA as carriers can effectively deliver plasmids to alleviate renal cell carcinoma [Citation21]. However, NPs with liposomes and PDA as carriers have never been explored in RI/R-induced AKI. The effects of POP-NPs on the modeling of AKI were explored in this study. First, POP-NPs were constructed. The strategy for preparing POPs is shown schematically in Figure S1A. TEM imaging showed that the synthesized POP-NPs were close to spherical (Figure S1B). The average diameter of POP-NPs was approximately 130 nm (Figure S1C). Next, cells were transfected with Plasmid-OIP5-AS1, POP-NPs, and their empty carriers. The results showed that both POP-NPs and Plasmid-OIP5-AS1 significantly increased the expression of OIP5-AS1 compared to their empty carrier groups (Figure S1D). The aforementioned treatments did not significantly impact cell viability, as demonstrated in Figure S1E. POP-NPs could inhibit OGD/R-induced cell apoptosis, ROS, inflammatory factors, and the increase in MDA levels, as well as the decrease in SOD levels. Treatment with mimics alone in the OGD/R group cells enhanced the earlier stated indexes induced by OGD/R. Co-treatment of OGD/R group cells with POP-NPs and mimics could reverse the changes in the indicators mentioned above seen in the OGD/R + POP-NPs + NC-1 group. The apoptosis, ROS, inflammatory factors, and MDA levels in the OGD/R + POP-NPs + mimics + Bardoxolone group were lower compared to the OGD/R + POP-NPs + mimics group, while the SOD levels were higher (). The expression of OIP5-AS1 in the OGD/R + Vector + NC-1 and OGD/R + Vector + mimics groups was lower compared to the other groups. POP-NPs inhibited miR-410-3p and promoted the expression of Nrf2/HO-1. After transfection with mimics, the Nrf2/HO-1 pathway was inhibited (). The above results reveal that POP-NPs can alleviate OGD/R-induced cellular oxidative stress damage through the miR-410-3p/Nrf2/HO-1 axis.
Figure 6. POP-NPs suppress oxidative stress injury in the OGD/R group through the miR-410-3p/Nrf2/HO-1 axis. Cells were transfected with POP-NPs and mimics negative control (Vector and NC-1), POP-NPs and NC-1, Vector and mimics, as well as POP-NPs and mimics. Afterward, they were treated with OGD/R in the presence or absence of Nrf2 agonists (Bardoxolone, 0.3 μM, HY-14909, MCE). (A) The apoptosis was detected using flow cytometry. (B) The ROS level of HK-2 cells was measured using DCFH-DA fluorescence. (C) The level of inflammatory factors was measured via ELISA. (D) SOD and MDA levels of the supernatant were measured using a spectrophotometer. (E and F) OIP5-AS1, miR-410-3p, and Nrf2/HO-1 expressions were measured via RT-qPCR and western blot. ANOVA, *p < .05, vs. the OGD/R + Vector + NC-1, #p < .05, vs. the OGD/R + POP-NPs + NC-1, &p < .05, vs. the OGD/R + POP-NPs + mimics.
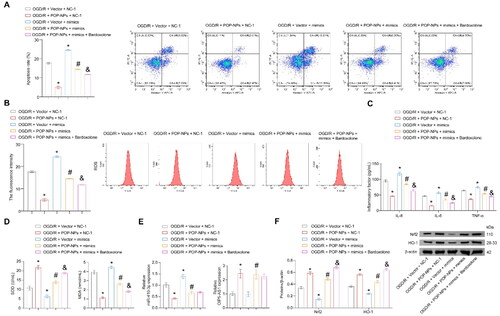
In vivo assessment of the toxicity of POP-NPs
Upon administering POP-NPs, there was no observable significant damage to the vital organs, including the heart, liver, lungs, and spleen, in the healthy mice (Figure S2A). There were no significant differences in the levels of the hematological and hepatocyte injury-related indices, including RBC, Hb, WBC, PLT, Gran%, ALT, AST, CREA, and BUN in POP-NPs-treated mice as compared to mice in the normal group (Figure S2B-S2C). The results suggest that POP-NPs did not cause unfavorable effects on healthy mice and demonstrated remarkable biocompatibility in vivo.
The influence of POP-NPs on the RI/R and miR-410-3p/Nrf2/HO-1 pathway in vivo
We further validated the influence of POP-NPs on the RI/R and miR-410-3p/Nrf2/HO-1 pathway in vivo. First, the expression of OIP5-AS1 significantly increased in normal mice after the injection of POP-NPs (). POP-NPs reversed the increase in kidney tissue damage, BUN, Cre, MDA, inflammatory factors, ROS, and apoptosis levels induced by RI/R, as well as the decrease in SOD levels (). POP-NPs also reversed the upregulation of miR-410-3p expression and the downregulation of OIP5-AS1 and Nrf2/HO-1 expressions induced by RI/R (). In conclusion, POP-NPs alleviate RI/R, suppress the expression of miR-410-3p, and activate the Nrf2/HO-1 pathway.
Figure 7. The influence of POP-NPs on the RI/R and miR-410-3p/Nrf2/HO-1 pathway in vivo. (A) Detection of OIP5-AS1 expression in the tissue. Normal mice were injected with either Vector or POP-NPs. (B) Representative images of mouse kidney tissue. (C) Representative images of H&E staining. (D and E) Representative bar graphs of BUN and Cre levels detected through ELISA. (F) Representative bar graphs of SOD and MDA levels in serum detected using biochemical assay kits. (G) The representative bar chart of the concentrations of inflammatory factors IL-8, IL-6, and TNF-α was detected by RT-qPCR. (H) The ROS levels were detected using a ROS assay kit. (I) The representative image and quantification analysis of renal tissue apoptosis levels. (J) Representative images of the OIP5-AS1, miR-410-3p, and Nrf2/HO-1 gene expressions were determined by RT-qPCR. (K) Representative images of the Nrf2/HO-1 protein expressions were determined by western blot. (L) The representative images showcasing the expressions of Nrf2/HO-1 and their quantitative statistics were gathered through IHC. n = 6–8 mice/group, ANOVA, *p < .05, vs. the Ctrl, #p < .05 vs. the RI/R + Vector.
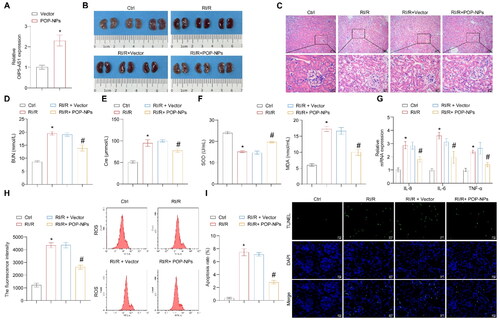
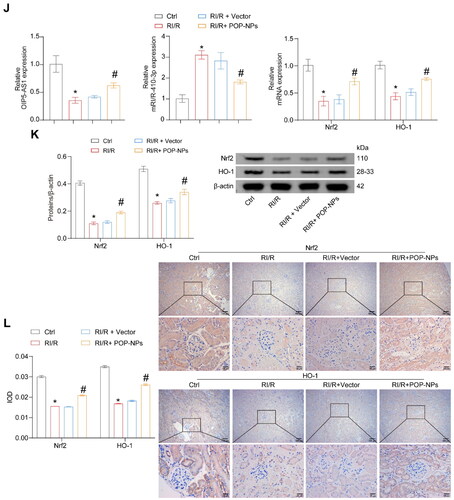
Discussion
Available data indicate that one-tenth of the world population lives under the shadow of kidney disease [Citation34,Citation35]. However, only renal replacement therapy can effectively treat kidney disease, requiring patients to bear high medical expenses. To develop innovative treatments for kidney disease, it becomes essential to illustrate the molecular pathology of the disease. Our study found that decreased expression of OIP5-AS1 weakened its ability to bind to and inhibit miR-410-3p competitively. Following that, Nrf2, the target gene of miR-410-3p, was inhibited by miR-410-3p, resulting in the suppression of the expression of HO-1. The results strongly hint that the Nrf2/HO-1 pathway might play a protective role in RI/R. Such a role was largely fulfilled by the regulatory network of OIP5-AS1 and miR-410-3p. This study further revealed the role and mechanism of Nrf2/HO-1 in and enriched the theoretical research in relevant areas.
PDA, a self-polymerizing derivative of dopamine, is a melanin-like structure that is integrated into the core because of its strong antioxidant capacity [Citation36]. PDA NPs are considered attractive alternatives to NPs in nanomedicine [Citation36,Citation37]. Ma et al. reported that HACE2-exosome-based PDA NPs have high capture, multiple antioxidant capacity and good biocompatibility [Citation38]. PDA and liposomes NPs have good biocompatibility and biodegradability [Citation39,Citation40]. Mao et al. demonstrated that nanostructures incorporating liposomes and PDA as carriers can efficiently deliver plasmids to alleviate renal cell carcinoma [Citation21]. Zheng et al. revealed that PDA@MF NPs (manganese ferrite nanoparticles wrapped with PDA) effectively attenuated oxidative stress in vivo, ameliorated renal inflammatory events, and improved renal function [Citation23]. Similar to the above study, our study found that POP-NPs alleviated RI/R-induced oxidative stress, inflammation and apoptosis in mice and HK-2 cells. Our study revealed the potential of POP-NPs as an effective treatment for RI/R-induced AKI.
The Nrf2/HO-1 pathway is associated with many diseases, such as Parkinson’s disease, liver injury, cancer, etc. [Citation41–43]. Recent research has revealed that the complex interplay within the renal vasculature often precipitates I/R injury, culminating in oxidative stress. In contrast, the accumulation of oxidative stress has been widely regarded as the main route contributing to various kidney diseases [Citation44,Citation45]. Activation of renal tubular antioxidant transcription factor Nrf2 in the RI/R injury mice can induce cytoprotection-related genes related to antioxidative stress and effectively alleviate renal tubular injury interstitial fibrosis [Citation45,Citation46]. The activation of the Nrf2/HO-1 pathway has been found to alleviate oxidative stress-induced pyroptosis in a mouse model of RI/R injury [Citation27]. The role of Nrf2/HO-1 pathway activation in countering acetaminophen-induced oxidative stress has been observed in HK-2 cells [Citation47]. This study found that in the RI/R mouse model, the expressions of Nrf2 and its target gene HO-1 in the kidney tissues were decreased. OIP5-AS1 could regulate Nrf2/HO-1 expressions.
LncRNA FTX regulates cardiomyocyte I/R by upregulating the relative pathway by sponge miR-410-3p [Citation48]. OIP5-AS1 can effectively alleviate myocardial I/R injury [Citation30]. OIP5-AS1 reduces renal epithelial cell apoptosis in cisplatin-induced AKI by regulating the miR-144-5p/PKM2 axis [Citation49]. Besides, some studies have also shown that miR-410-3p is regulated by OIP5-AS1 in glioma and multiple myeloma progression [Citation32,Citation50]. Here, we reported that OIP5-AS1 could physiologically function like a ceRNA in binding miR-410-3p and upregulating the expression levels of Nrf2/HO-1. Our study discovered the regulative relationship of OIP5-AS1/miR-410-3p/Nrf2 for the first time in HK-2 cells. Since the expression of miR-410-5p was greatly increased in the RI/R mouse models, it could be used as a potential marker of RI/R to provide more guidance for the AKI caused by RI/R in clinical kidney transplantation. Meanwhile, our research also provides new ideas on how to activate Nrf2 and apply it to the treatment of antioxidative damage.
It would be beneficial to the quality of the manuscript if a fluorescence imaging system could be utilized to observe and illustrate whether POP-NPs aggregate in the kidney. Unfortunately, our laboratory lacks the appropriate equipment, such as a fluorescence imaging system. Therefore, we cannot provide data on this part for the time being, which limits our study. Particle size is critical to its performance in biomedical applications. POP-NPs were prepared with reference to the pre-experiments and reference [Citation21]. The particle size of the plasmid prepared by Mao et al. using PDA and liposomes was about 140 nm [Citation21]. The particle size of SOD2 mRNA encapsulated by Hou et al. using liposomes was about 100 nm [Citation22]. Similar to previous studies, the average particle size of POP-NPs prepared in our study was about 100 nm according to the current method. A limitation of our study is that we have not delved further into how to control the synthesis procedure to adjust or further optimize the particle size. We plan to investigate further whether changes in some variables, such as processing time, can optimize the particle size. Due to the lack of relevant equipment, whether POP-NPs aggregate in the kidney has not been investigated for the time being, which is a limitation of our study. This study did not verify the expression of OIP5-AS1, miR-410-3p, and Nrf2/HO-1 pathway in clinical samples, which is another limitation of this study. In addition, further study of gene chips will help to discover more potential markers and targets.
In conclusion, POP-NPs could attenuate RI/R-induced injury through miR-410-3p/Nrf2 axis. Our study lays the theory for developing new nanoparticle-related targeted therapeutic approaches for RI/R-induced AKI.
Authors contributions
Rongjie Zhang, Xin Zhang and Xiuwu Han designed the research, Xuhui Zhu, Tao Li, and Yansheng Li performed the research, and Peng Zhang, Yuanhao Chen, and Gao Li analyzed the data. All authors contributed to the writing and revisions. All authors contributed to the article and approved the submitted version.
Ethics statement
All experimental procedures and animal handling were performed with the approval of the Animal Care and Use Committee of the Beijing Chao-Yang Hospital, in accordance with the National Institutes of Health Guide for the Care and Use of Laboratory Animals, and studies involving laboratory animals follows the ARRIVE guidelines.
Supplemental Material
Download PDF (88.8 KB)Disclosure statement
No potential conflict of interest was reported by the author(s).
Data availability statement
The data that support the findings of this study are available from the corresponding author upon reasonable request.
Additional information
Funding
References
- Rossiter A, La A, Koyner JL, et al. New biomarkers in acute kidney injury. Crit Rev Clin Lab Sci. 2024;61(1):23–44. doi: 10.1080/10408363.2023.2242481.
- Husain-Syed F, Takeuchi T, Neyra JA, et al. Acute kidney injury in neurocritical care. Crit Care. 2023;27(1):341. doi: 10.1186/s13054-023-04632-1.
- Pan M, Wang Z, Wang Y, et al. Celastrol alleviated acute kidney injury by inhibition of ferroptosis through Nrf2/GPX4 pathway. Biomed Pharmacother. 2023;166:115333. doi: 10.1016/j.biopha.2023.115333.
- Lau A, Rahn JJ, Chappellaz M, et al. Dipeptidase-1 governs renal inflammation during ischemia reperfusion injury. Sci Adv. 2022;8(5):eabm0142. doi: 10.1126/sciadv.abm0142.
- Su L, Zhang J, Wang J, et al. Pannexin 1 targets mitophagy to mediate renal ischemia/reperfusion injury. Commun Biol. 2023;6(1):889. doi: 10.1038/s42003-023-05226-x.
- González-Soria I, Soto-Valadez AD, Martínez-Rojas MA, et al. SerpinA3K deficiency reduces oxidative stress in acute kidney injury. Int J Mol Sci. 2023;24(9):7815. doi: 10.3390/ijms24097815.
- Li C, Han S, Zhu J, et al. MiR-132-3p activation aggravates renal ischemia-reperfusion injury by targeting Sirt1/PGC1alpha axis. Cell Signal. 2023;110:110801. doi: 10.1016/j.cellsig.2023.110801.
- Xu Z, Zhang M, Wang W, et al. Dihydromyricetin attenuates cisplatin-induced acute kidney injury by reducing oxidative stress, inflammation and ferroptosis. Toxicol Appl Pharmacol. 2023;473:116595. doi: 10.1016/j.taap.2023.116595.
- Xu L, Cai J, Li C, et al. 4-Octyl itaconate attenuates LPS-induced acute kidney injury by activating Nrf2 and inhibiting STAT3 signaling. Mol Med. 2023;29(1):58. doi: 10.1186/s10020-023-00631-8.
- Sun YY, Zhu HJ, Zhao RY, et al. Remote ischemic conditioning attenuates oxidative stress and inflammation via the Nrf2/HO-1 pathway in MCAO mice. Redox Biol. 2023;66:102852. doi: 10.1016/j.redox.2023.102852.
- Chen Y, He W, Wei H, et al. Srs11-92, a ferrostatin-1 analog, improves oxidative stress and neuroinflammation via Nrf2 signal following cerebral ischemia/reperfusion injury. CNS Neurosci Ther. 2023;29(6):1667–1677. doi: 10.1111/cns.14130.
- Wang L, Liu J, Wang Z, et al. Dexmedetomidine abates myocardial ischemia reperfusion injury through inhibition of pyroptosis via regulation of miR-665/MEF2D/Nrf2 axis. Biomed Pharmacother. 2023;165:115255. doi: 10.1016/j.biopha.2023.115255.
- Liu M, Wen H, Zuo L, et al. Bryostatin-1 attenuates intestinal ischemia/reperfusion-induced intestinal barrier dysfunction, inflammation, and oxidative stress via activation of Nrf2/HO-1 signaling. Faseb J. 2023;37(6):e22948. doi: 10.1096/fj.202201540R.
- Song J, Wang H, Sheng J, et al. Vitexin attenuates chronic kidney disease by inhibiting renal tubular epithelial cell ferroptosis via NRF2 activation. Mol Med. 2023;29(1):147. doi: 10.1186/s10020-023-00735-1.
- Shen K, Wang X, Wang Y, et al. miR-125b-5p in adipose derived stem cells exosome alleviates pulmonary microvascular endothelial cells ferroptosis via Keap1/Nrf2/GPX4 in sepsis lung injury. Redox Biol. 2023;62:102655. doi: 10.1016/j.redox.2023.102655.
- Peng S, Shen L, Yu X, et al. miR-200a attenuated oxidative stress, inflammation, and apoptosis in dextran sulfate sodium-induced colitis through activation of Nrf2. Front Immunol. 2023;14:1196065. doi: 10.3389/fimmu.2023.1196065.
- Xia H, Shanshan X, Sumeng L, et al. LncRNA RMRP aggravates LPS-induced HK-2 cell injury and AKI mice kidney injury by upregulating COX2 protein via targeting ELAVL1. Int Immunopharmacol. 2023;116:109676. doi: 10.1016/j.intimp.2022.109676.
- Wang J, Jiao P, Wei X, et al. Silencing long non-coding RNA Kcnq1ot1 limits acute kidney injury by promoting miR-204-5p and blocking the activation of NLRP3 inflammasome. Front Physiol. 2021;12:721524. doi: 10.3389/fphys.2021.721524.
- Ma SR, Ma Q, Ma YN, et al. Comprehensive analysis of ceRNA network composed of circRNA, miRNA, and mRNA in septic acute kidney injury patients based on RNA-seq. Front Genet. 2023;14:1209042. doi: 10.3389/fgene.2023.1209042.
- Yang N, Yan N, Bai Z, et al. FTO attenuates LPS-induced acute kidney injury by inhibiting autophagy via regulating SNHG14/miR-373-3p/ATG7 axis. Int Immunopharmacol. 2024;128:111483. doi: 10.1016/j.intimp.2023.111483.
- Mao W, Wang K, Zhang W, et al. Transfection with plasmid-Encoding lncRNA-SLERCC nanoparticle-mediated delivery suppressed tumor progression in renal cell carcinoma. J Exp Clin Cancer Res. 2022;41(1):252. doi: 10.1186/s13046-022-02467-2.
- Hou Y, Lin S, Xia J, et al. Alleviation of ischemia-reperfusion induced renal injury by chemically modified SOD2 mRNA delivered via lipid nanoparticles. Mol Ther Nucleic Acids. 2023;34:102067. doi: 10.1016/j.omtn.2023.102067.
- Zheng B, Deng G, Zheng J, et al. Self-polymerized polydopamine-based nanoparticles for acute kidney injury treatment through inhibiting oxidative damages and inflammatory. Int J Biochem Cell Biol. 2022;143:106141. doi: 10.1016/j.biocel.2021.106141.
- Zhang P, Han X, Zhang X, et al. Lactobacillus acidophilus ATCC 4356 alleviates renal ischemia-reperfusion injury through antioxidant stress and anti-inflammatory responses and improves intestinal microbial distribution. Front Nutr. 2021;8:667695. doi: 10.3389/fnut.2021.667695.
- Zhu DJ, Liao XH, Huang WQ, et al. Augmenter of liver regeneration protects renal tubular epithelial cells from ischemia-reperfusion injury by promoting PINK1/Parkin-Mediated mitophagy. Front Physiol. 2020;11:178. doi: 10.3389/fphys.2020.00178.
- Wei L, Zhang X, Ye Q, et al. The transfection of A20 gene prevents kidney from ischemia reperfusion injury in rats. Mol Med Rep. 2017;16(2):1486–1492. doi: 10.3892/mmr.2017.6725.
- Diao C, Chen Z, Qiu T, et al. Inhibition of PRMT5 attenuates oxidative stress-induced pyroptosis via activation of the Nrf2/HO-1 signal pathway in a mouse model of renal ischemia-reperfusion injury. Oxid Med Cell Longev. 2019;2019:2345658–2345618. doi: 10.1155/2019/2345658.
- Jin B, Li G, Zhou L, et al. Mechanism involved in acute liver injury induced by intestinal ischemia-reperfusion. Front Pharmacol. 2022;13:924695. doi: 10.3389/fphar.2022.924695.
- Xue Z, Zhao K, Sun Z, et al. Isorhapontigenin ameliorates cerebral ischemia/reperfusion injury via modulating kinase Cε/Nrf2/HO-1 signaling pathway. Brain Behav. 2021;11(7):e02143. doi: 10.1002/brb3.2143.
- Niu X, Pu S, Ling C, et al. lncRNA Oip5-as1 attenuates myocardial ischaemia/reperfusion injury by sponging miR-29a to activate the SIRT1/AMPK/PGC1α pathway. Cell Prolif. 2020;53(6):e12818. doi: 10.1111/cpr.12818.
- Chen Y, Liu W, Chen M, et al. Up-regulating lncRNA OIP5-AS1 protects neuron injury against cerebral hypoxia-ischemia induced inflammation and oxidative stress in microglia/macrophage through activating CTRP3 via sponging miR-186-5p. Int Immunopharmacol. 2021;92:107339. doi: 10.1016/j.intimp.2020.107339.
- Sun WL, Kang T, Wang YY, et al. Long noncoding RNA OIP5-AS1 targets wnt-7b to affect glioma progression via modulation of miR-410. Biosci Rep. 2019;39(1):BSR20180395. doi: 10.1042/bsr20180395.
- Tang W, Panja S, Jogdeo CM, et al. Modified chitosan for effective renal delivery of siRNA to treat acute kidney injury. Biomaterials. 2022;285:121562. doi: 10.1016/j.biomaterials.2022.121562.
- Sato Y, Yanagita M. Renal anemia: from incurable to curable. Am J Physiol Renal Physiol. 2013;305(9):F1239–1248. doi: 10.1152/ajprenal.00233.2013.
- Levey AS, Titan SM, Powe NR, et al. Kidney disease, race, and GFR estimation. Clin J Am Soc Nephrol. 2020;15(8):1203–1212. doi: 10.2215/cjn.12791019.
- Liu H, Yang Y, Liu Y, et al. Melanin-like nanomaterials for advanced biomedical applications: a versatile platform with extraordinary promise. Adv Sci. 2020;7(7):1903129. doi: 10.1002/advs.201903129.
- Gholami Derami H, Gupta P, Weng KC, et al. Reversible photothermal modulation of electrical activity of excitable cells using polydopamine nanoparticles. Adv Mater. 2021;33(32):e2008809. doi: 10.1002/adma.202008809.
- Ma X, Guo S, Ruan S, et al. HACE2-exosome-based nano-bait for concurrent SARS-CoV-2 trapping and antioxidant therapy. ACS Appl Mater Interfaces. 2022;14(4):4882–4891. doi: 10.1021/acsami.1c19541.
- Seth A, Gholami Derami H, Gupta P, et al. Polydopamine-mesoporous silica core-shell nanoparticles for combined photothermal immunotherapy. ACS Appl Mater Interfaces. 2020;12(38):42499–42510. doi: 10.1021/acsami.0c10781.
- Bi D, Zhao L, Li H, et al. A comparative study of polydopamine modified and conventional chemical synthesis method in doxorubicin liposomes form the aspect of tumor targeted therapy. Int J Pharm. 2019;559:76–85. doi: 10.1016/j.ijpharm.2019.01.033.
- Wang Y, Gao L, Chen J, et al. Pharmacological modulation of Nrf2/HO-1 signaling pathway as a therapeutic target of Parkinson’s disease. Front Pharmacol. 2021;12:757161. doi: 10.3389/fphar.2021.757161.
- Wang C, Liu T, Tong Y, et al. Ulinastatin protects against acetaminophen-induced liver injury by alleviating ferroptosis via the SIRT1/NRF2/HO-1 pathway. Am J Transl Res. 2021;13:6031–6042.
- Wei R, Zhao Y, Wang J, et al. Tagitinin C induces ferroptosis through PERK-Nrf2-HO-1 signaling pathway in colorectal cancer cells. Int J Biol Sci. 2021;17(11):2703–2717. doi: 10.7150/ijbs.59404.
- Tammaro A, Kers J, Scantlebery AML, et al. Metabolic flexibility and innate immunity in renal ischemia reperfusion injury: the fine balance between adaptive repair and tissue degeneration. Front Immunol. 2020;11:1346. doi: 10.3389/fimmu.2020.01346.
- Nezu M, Suzuki N. Roles of Nrf2 in protecting the kidney from oxidative damage. Int J Mol Sci. 2020;21(8):2951. doi: 10.3390/ijms21082951.
- Lu M, Wang P, Qiao Y, et al. GSK3β-mediated Keap1-independent regulation of Nrf2 antioxidant response: a molecular rheostat of acute kidney injury to chronic kidney disease transition. Redox Biol. 2019;26:101275. doi: 10.1016/j.redox.2019.101275.
- Ruan H, Wang L, Wang J, et al. Sika deer antler protein against acetaminophen-induced oxidative stress and apoptosis in HK-2 cells via activating Nrf2/keap1/HO-1 pathway. J Food Biochem. 2019;43(12):e13067. doi: 10.1111/jfbc.13067.
- Li L, Li L, Zhang YZ, et al. Long non-coding RNA FTX alleviates hypoxia/reoxygenation-induced cardiomyocyte injury via miR-410-3p/Fmr1 axis. Eur Rev Med Pharmacol Sci. 2020;24:396–408. doi: 10.26355/eurrev_202001_19938.
- Chang S, Chang M, Liu G, et al. LncRNA OIP5-AS1 reduces renal epithelial cell apoptosis in cisplatin-induced AKI by regulating the miR-144-5p/PKM2 axis. Biomed J. 2022;45(4):642–653. doi: 10.1016/j.bj.2021.07.005.
- Yang N, Chen J, Zhang H, et al. LncRNA OIP5-AS1 loss-induced microRNA-410 accumulation regulates cell proliferation and apoptosis by targeting KLF10 via activating PTEN/PI3K/AKT pathway in multiple myeloma. Cell Death Dis. 2017;8(8):e2975–e2975. doi: 10.1038/cddis.2017.358.