Abstract
Objective
Chronic kidney disease (CKD) is a condition influenced by both genetic and environmental factors and has been a focus of extensive research. Utilizing Mendelian randomization, researchers have begun to untangle the complex causal relationships underlying CKD. This review delves into the advances and challenges in the application of MR in the field of nephrology, shifting from a mere summary of its principles and limitations to a more nuanced exploration of its contributions to our understanding of CKD.
Methods
Key findings from recent studies have been pivotal in reshaping our comprehension of CKD. Notably, evidence indicates that elevated testosterone levels may impair renal function, while higher sex hormone-binding globulin (SHBG) levels appear to be protective, predominantly in men. Surprisingly, variations in plasma glucose and glycated hemoglobin levels seem unaffected by genetically induced changes in the estimated glomerular filtration rate (eGFR), suggesting an independent pathway for renal function impairment.
Results
Furthermore, lifestyle factors such as physical activity and socioeconomic status emerge as significant influencers of CKD risk and kidney health. The relationship between sleep duration and CKD is nuanced; short sleep duration is linked to increased risk, while long sleep duration does not exhibit a clear causal effect. Additionally, lifestyle factors, including diet, exercise, and mental wellness activities, play a crucial role in kidney health. New insights also reveal a substantial causal connection between both central and general obesity and CKD onset, while no significant links were found between genetically modified LDL cholesterol or triglyceride levels and kidney function.
Conclusion
This review not only presents the recent achievements of MR in CKD research but also illuminates the path forwards, underscoring critical unanswered questions and proposing future research directions in this dynamic field.
Key analysis methods
Observational studies have been valuable in advancing our understanding of the causes of diseases. However, these studies have limitations in regard to drawing causal inferences. In some cases, the exposure that was thought to impact disease risk in observational studies was later found to be a risk marker rather than a cause, as later randomized controlled trials (RCTs) did not show any effect or even showed a reverse effect. This phenomenon is likely due to residual confounding, which occurs when relevant confounding factors, such as lifestyle factors, are not identified or measured comprehensively. Alternatively, findings can be attributed to reverse causation, which occurs when the subclinical presence of disease influences the exposure under investigation rather than the other way around. Mendelian randomization (MR) studies offer a solution by using the random distribution of genetic variants during meiosis, comparable to the random treatment assignment in clinical trials, to assess causal relationships using observational data [Citation1] ().
MR studies involve the utilization of genetic variants that occur during meiosis. Due to the unpredictable nature of the genetic recombination process, genetic variation is randomly distributed, which means that people are naturally randomized into various genetic groups. This genetic randomization approach enables researchers to use genetic variants as instruments to investigate the causality of a risk factor and an outcome of interest. As a result, similar to RCTs, MR studies offer the possibility to infer causality, but MR can be applied to observational data without the requirement for an actual intervention [Citation2].
Furthermore, MR studies have the advantage of reducing bias and offering more reliable results than observational studies. These studies overcome the limitations of residual confounding and reverse causation that undermine the validity of observational studies. MR studies have provided a more comprehensive understanding and accurate assessment of the causal relationships between risk factors and various diseases, including coronary artery disease, type 2 diabetes, and obesity.
In this review, we primarily focus on the MR method. The MR method is a genetic information-based tool that can be used to investigate the causal relationship between specific genetic variants and disease risk. The MR method relies on the fulfillment of three fundamental assumptions. (1) The relevance assumption is met if the selected genetic variants are significantly associated with the exposure variable under study, ensuring that they can reasonably represent the level of exposure. (2) The independence assumption is met if the genetic variants are unrelated to potential confounders of the disease, ensuring that the genetic instruments influence disease risk only through their effect on the exposure variable. (3) The exclusion restriction assumption is met if the impact of genetic variants on health outcomes are mediated entirely through the exposure variable and not through any separate independent pathway.
When interpreting the results of MR analysis, we also consider several potential limitations of the method that might affect our conclusions: (1) the difficulty of assumption validation, as it is sometimes challenging to ensure that all genetic variants strictly meet the necessary MR assumptions; (2) horizontal pleiotropy, where genetic variants may affect multiple biological pathways, thereby having a direct effect on the disease; (3) the strength of the instrumental variable, where weaker instruments could lead to excessive random error and biased estimates; (4) population-specific effects, as the same genetic variant may have different associations with exposure and outcomes in different populations; and (5) data quality and the choice of statistical methods also significantly affect the results of MR analysis.
Overall, the MR approach based on genetic randomization has the potential to become an effective and efficient way of identifying causal associations between risk factors and health outcomes, contributing to the development of preventive strategies and decision-making processes in clinical practice and public health policies.
Key methods to analyze pleiotropy and heterogeneity
The mainstream MR methods include the inverse-variance weighting method (IVW, fixed-effects or multiplicative random-effects model) [Citation3], MR–Egger [Citation4], median-based methods (weighted median, simple median or penalized weighted-median) [Citation5], and mode-based methods (simple mode or weighted mode) [Citation6]. Therefore, the IVW method assumes that all instruments are valid, while other methods can yield robust estimates even if some instruments are invalid. The MR–Egger approach is utilized to evaluate whether genetic variations have pleiotropic influences on the study outcome by comparing the regression intercept with zero [Citation4], while the weighted median method can give a true estimate even when up to 50% of the instruments are invalid [Citation5]. Horizontal pleiotropy is an important issue and an inborn weakness of MR, as the current genetic studies suggest that a gene usually affects more than 1 phenotype and that a complex phenotype is usually determined by multiple genetic factors. Currently, several methods have been proposed to address this issue. (1) MR-PRESSO (Pleiotropy RESidual Sum and Outlier) is an outlier detection method used to identify and remove horizontal pleiotropy [Citation7]. (2) MR-CAUSE (Causal Analysis Using Summary Effect estimates) is a method that accounts for both correlated and uncorrelated horizontal pleiotropy [Citation8]. In addition, the MR-BMA (Bayesian Model Averaging) method is suitable for dealing with multidimensional data in MR analysis [Citation8].
In this review, we explore the potential application of more complex MR methods, including multivariable, bidirectional, and nonlinear MR methods, in the field of CKD. Given the multifactorial nature of CKD, which involves intricate genetic and environmental interactions, these advanced MR methods offer unique perspectives for a deeper understanding of disease mechanisms and the development of new treatment strategies. Multivariate MR enables the assessment of the impact of multiple interrelated risk factors for CKD, such as hypertension, diabetes, and obesity, while accounting for their interactions. This approach helps in distinguishing which factors contribute most significantly to the progression of CKD, thus guiding the formulation of clinical prevention and treatment strategies. Bidirectional MR provides a tool for exploring the potential bidirectional causal relationships between CKD and its related complications. For instance, this method can investigate how CKD may affect the risk of cardiovascular diseases and how cardiovascular diseases, in turn, may accelerate the progression of CKD. Understanding this bidirectional causality is crucial for devising comprehensive treatment plans. Finally, nonlinear MR allows researchers to explore the nonlinear relationships between risk factors for CKD and disease progression. For example, it can help identify situations where the disease may suddenly worsen when certain biomarkers or clinical indicators reach specific thresholds. This method is particularly important in the formulation of individualized treatment strategies, helping to determine the optimal timing for therapeutic intervention [Citation9]. In summary, the application of these complex MR methods in CKD research not only provides deep insights into genetic risks and disease mechanisms but also offers valuable information for the development of new therapeutic drugs and intervention measures. However, the application of these methods requires consideration of the corresponding data requirements, statistical expertise, potential limitations and validity of the assumptions. In the context of discussing CKD, the assessment of kidney function is a critical component, typically evaluated by metrics such as the eGFR, urinary protein levels, and other relevant indicators. Recent research has identified rs77924615 as a potential effector gene variant for urinary protein, suggesting that this gene variant is a promising target for further investigation within the field [Citation10].
Overall, the methodology of MR design is still under rapid development, and numerous methods have been proposed to address core questions. However, under these circumstances, we should bear in mind that the basic method, IVW, should be the core method, and other methods can be used as supplementary and sensitivity analyses. Only the results that are confirmed by different methods that rely on different assumptions can be deemed valid and scientific.
Associations of biochemical parameters with renal function
Diabetes and its associated indicators are frequently associated with renal function and CKD, and the kidney damage caused by diabetes is called diabetic nephropathy [Citation11]. A higher plasma blood glucose concentration can induce oxidative stress and promote inflammation, damaging renal tubules. A Danish population-based MR study confirmed that greater plasma glucose concentrations should increase the risk of diabetic nephropathy even when they are in the normoglycaemic range [Citation12]. Another MR study revealed that genetically predicted elevated fasting insulin increases the risk of CKD, and such causal effects might be sex specific, as the result was significant for men but not for women [Citation13]. Additionally, Min et al. reported that genetic predisposition to insulin resistance and type 2 diabetes (T2D) could also lead to decreased renal function in the Chinese population [Citation14]. Pooling all the current evidence together, it is clear that genetic factors related to higher plasma glucose levels, insulin resistance, and T2D status could compromise renal function and result in CKD. Moreover, such results might be applied to both European and East Asian populations. However, the plasma levels of glucose and glycated hemoglobin did not seem to change with a genetically predicted elevated eGFR, suggesting that compromised renal function might not change the plasma glucose level [Citation15]. Notably, genetically predicted fasting glucose was not causally associated with CKD development in the nondiabetic population according to a Korean population study [Citation16]. Additionally, T2D status should render the kidneys more vulnerable to harmful substances. For instance, exposure to lead might more easily induce renal dysfunction in individuals with T2D [Citation17]. In general, it is well recognized that increased plasma glucose levels, insulin resistance, and the presence of T2D can weaken the kidneys and impair renal function, rather than the other way around.
Many trace metal elements can also affect renal function. Genetically predicted elevated circulating copper levels may be a causal risk factor for CKD and possibly reduce the eGFR and induce a rapid decline in kidney function [Citation18]. In addition to trace metal elements, calcium and selenium can also impact renal function. A higher serum calcium concentration in the normal range may reflect increased tissue calcification and altered immune function, causing renal colic [Citation19]. Additionally, genetically predicted elevated selenium levels may be a causal risk factor for a decreased eGFR and increased BUN [Citation20].
In the realm of CKD, the influence of sex hormones is notable. Research indicates that in males, a genetic predisposition for increased testosterone is associated with a greater risk of developing CKD and deteriorating renal function; this factor could explain the sex-based differences observed in CKD prevalence [Citation21]. Interestingly, the serum testosterone concentration appears to be independent of kidney functionality [Citation21]. Moreover, a genetic predisposition to elevated levels of sex hormone-binding globulin (SHBG) was linked to reduced CKD risk and improved kidney health in men, a relationship not mirrored in women. This finding suggested a potential sex-specific role of SHBG in the context of CKD [Citation22]. The current evidence suggests that genetically predicted elevated testosterone could compromise renal function, while higher SHBG might protect against CKD, and such causal effects should be greater in men than in women. Notably, sex disparities, which have been studied in digestive diseases, are important factors that should be investigated further [Citation23].
In addition, other metabolic products play important roles. In the context of renal health, the role of homocysteine levels should be considered. Elevated levels of total homocysteine (tHcy) have been linked to impaired kidney function and the progression of CKD. Accordingly, interventions aimed at reducing tHcy levels could mitigate these adverse effects [Citation24]. Additionally, there appears to be a positive correlation between genetically higher concentrations of serum bilirubin and improved renal function.
In essence, the evidence collectively points to certain genetic factors that may defend against kidney disease: higher levels of SHBG and bilirubin are potentially linked to better kidney health and a reduced risk of CKD. Conversely, risk factors, including elevated fasting glucose levels, insulin resistance, type 2 diabetes, increased serum testosterone, homocysteine, and certain metals, are correlated with a greater likelihood of kidney impairment and CKD development. Importantly, the influence of testosterone and SHBG on kidney disease appears to be primarily relevant in males, underscoring the importance of sex-specific considerations in CKD research ().
Table 1. Blood biochemistry factors and renal function.
Cardiovascular disease and CKD
Hypertension and CKD are two closely interlinked pathophysiological states in which continuous hypertension can lead to worse kidney function and progressive decline in kidney function can conversely lead to worse blood pressure (BP) control. Multiple MR studies (assuming linear relationships) did not find an association between blood pressure and renal function, but the use of a nonlinear approach showed that higher genetically predicted blood pressure was associated with decreased renal function. However, nonlinear MR analysis requires individual-level data, which may not be easily accessible [Citation25]. Hypertension that is not adequately managed can result in severe cardiovascular complications and death and can hasten the development of end-stage renal disease [Citation26]. In a study conducted by Hui-Min and colleagues, the team utilized genetic methodologies to explore the connection between certain health risks and CKD. The study concluded that diastolic blood pressure (DBP) had a direct causal relationship with CKD risk. The authors also found indications that systolic blood pressure (SBP) might have a similar causal relationship with CKD, while elevated levels of HDL cholesterol appeared to be protectively associated with a lower incidence of CKD [Citation27]. In addition, a cross-population analysis was used to highlight potential variation in the association between hypertension and CKD among different ethnic groups. Research has shown that hypertension significantly influences CKD development in the European population, but this correlation was not apparent in the East Asian population [Citation28]. These findings imply that genetic ancestry might influence how BP affects CKD development [Citation26]. Multiple MR studies (assuming linear relationships) did not find an associations between blood pressure variance and renal function, but the use of a nonlinear approach showed that higher genetically predicted blood pressure was associated with reduced renal function. A plausible interpretation here is that linear models consider average effects, and if the relationship between blood pressure and renal function is indeed nonlinear, then the impact of blood pressure on renal function could be positive in some ranges and negative in others, thereby neutralizing each other. This could lead to nonsignificant results under a linear assumption.
Cardiovascular disease (CVD), which is globally recognized as the principal cause of mortality, often arises from cardiometabolic risks and CKD. CVD and CKD share a complex bilateral relationship in which each can exacerbate the other, and the presence of CKD, particularly end-stage renal disease, considerably increases the risk of death from CVD [Citation29]. Furthermore, CKD is associated with various other health complications, including anemia, bone disorders, cancer, and, specifically, cardiovascular conditions such as atrial fibrillation (AF), which is the most prevalent form of irregular heartbeat. The intricate link between CKD and AF has been substantiated through multiple clinical observational studies. Patients with CKD often exhibit a heightened risk of cardiovascular diseases, including AF, which, in turn, can exacerbate renal impairment. The bidirectional association highlights a potentially vicious cycle where each condition can precipitate and perpetuate the other. Hence, a holistic approach to the study of CKD should integrate an assessment of AF, considering its implications for the pathophysiology of kidney dysfunction, as well as its impact on the management and clinical outcomes of CKD patients.
In particular, the mechanisms underlying this bidirectional relationship may include shared risk factors such as hypertension, diabetes, and systemic inflammation, as well as the direct effects of AF-related haemodynamic changes on renal function. Therefore, the discussion of AF within CKD research is not merely additive but pivotal for understanding the full scope of renal disease progression, patient prognosis, and the selection of appropriate therapeutic interventions. Nevertheless, the probability that a reduction in the eGFR could independently increase the risk of developing AF or atrial fibrillation/flutter (AF/F) is considered unlikely based on the current evidence. Taken together, these results reveal the intricate interplay among hypertension, lipid concentrations, genetic heritage, CKD, and cardiovascular disease. This underscores the importance of a thorough and nuanced methodology for evaluating the risk determinants and concurrent disorders linked with chronic kidney conditions. A recent MR analysis conducted by Sven and his team revealed a reciprocal causative link between renal function and AF [Citation30]. Moreover, a separate MR investigation encompassing a cohort of 567,460 individuals of European ancestry revealed the directional influence of AF and atrial fibrillation/flutter on the estimated glomerular filtration rate (eGFR). This study also revealed the direct impact of these cardiac conditions on the likelihood of developing CKD [Citation31]. In a recent study involving approximately 640,000 participants, in which genetic, biomarker, and clinical data were integrated, individuals without obvious cardiovascular diseases (CVDs) or diabetes were shown to experience an increased risk of CVD even when kidney function was mildly impaired. The findings of that study indicate that the detrimental effects of decreased kidney function on CVD incidence occur independently of traditional cardiovascular risk factors. This emphasizes the critical need for direct preservation of renal function, in addition to the management of known risk factors, as a strategy for preventing cardiovascular diseases [Citation32].
A significant risk factor for coronary artery disease is CKD. People with CKD are exposed to nontraditional cardiovascular disease risk factors such as inflammation, oxidative stress, and abnormal calcium-phosphorus metabolism in addition to a high prevalence of traditional CAD risk factors such as diabetes and hypertension [Citation33]. The analysis spearheaded by Jie’s team highlights a distinct discrepancy in how certain metabolic and cardiovascular risk indicators affect CKD prevalence between European and East Asian cohorts. Eight risk factors were confirmed to influence CKD causally in European subjects; conversely, only three of these risk factors mirrored this causality in East Asians. These included adiposity measurements such as BMI; metabolic disorders such as type 2 diabetes; and conditions contributing to urinary stone formation. This investigative outcome underscores the strong synergy between renal health impairment and disorders of metabolic and cardiovascular origin. Moreover, ethnicity may modulate the manifestation and complexity of these risk factors, emphasizing the importance of contextual and population-specific health strategies in addressing CKD prevention and management [Citation28].
Taken together, these new MR study results suggest that CKD and CVD are closely related. Current research underscores the pronounced bidirectional relationship between cardiovascular disease (CVD) and chronic kidney disease (CKD). Common risk factors for CVD, such as hypertension and diabetes, not only directly compromise cardiac health but also significantly elevate the risk of CKD. On the other hand, CKD itself, particularly end-stage renal disease, substantially increases the risk of developing cardiovascular diseases, including coronary artery disease and atrial fibrillation. Additionally, the association between CKD and cardiovascular diseases may be influenced by genetic background and ethnic variation. Thus, it is crucial in the treatment and management of CKD to consider cardiovascular health factors comprehensively, and in the prevention of cardiovascular diseases, the preservation of renal function should not be overlooked. This bidirectional relationship suggests a potentially vicious cycle where each condition may exacerbate and perpetuate the other, highlighting the necessity of adopting a holistic and integrated treatment strategy to concurrently address these two interrelated chronic conditions ().
Table 2. Chronic heart disease and CKD.
Lifestyle factors and renal function
Sehoon et al.'s research indicated that physical activity is associated with a lower risk of CKD and a higher eGFR, while sedentary behavior, particularly watching television, is linked to worse kidney function. These findings were supported by MR analysis, which suggested causal relationships between physical activity, television watching, and kidney function [Citation34]. Another study by Sehoon et al. revealed that education attainment has a causal effect on CKD development, with higher education attainment being associated with a reduced risk of CKD [Citation35]. Additionally, short sleep duration was associated with CKD, while long sleep duration did not have significant causal effects [Citation36]. Symptoms of depression were identified as a substantial contributing element to the deterioration of renal function, whereas a genetic inclination toward a positive mood was linked with enhanced renal health [Citation37]. Overall, a sense of psychological well-being is causally linked to kidney function. These findings have important implications for public interventions aimed at reducing the burden of CKD ().
Figure 2. Mendelian randomization-confirmed factors affecting kidney function. Red lines indicate factors detrimental to kidney function; blue lines indicate factors beneficial to kidney function.
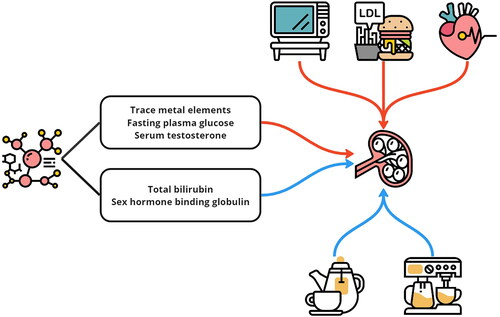
In the research conducted by Mohsen and colleagues [Citation38], there was no notable correlation between the consumption of monounsaturated fatty acids (MUFAs) and the occurrence of CKD. On the other hand, the study revealed reverse associations of the intake of polyunsaturated fatty acids (PUFAs) with renal health indicators and CKD prevalence. Furthermore, MR studies did not corroborate any causal relationship between the levels of either MUFAs or PUFAs and the development of CKD. An investigation led by Sehoon et al. revealed a significant link between the type of macronutrients consumed and the likelihood of developing CKD, independent of overall caloric consumption. They identified a positive correlation between kidney health and diets that emphasize greater protein intake as opposed to greater fat consumption. This implies that for individuals who typically follow a diet rich in fats and poor in proteins, adjusting their macronutrient ratio could be beneficial for their renal health. Consequently, this highlights the importance for medical practitioners to evaluate and advise on the composition of macronutrients in a patient’s diet, as these nutrients play a critical role in influencing kidney function [Citation39] ().
An MR investigation led by Oliver J and team [Citation40] indicated the potential protective impact of routine coffee consumption on kidney health. Concurrently, the study by Yangchang and collaborators [Citation41] revealed a causal link between an additional daily cup of tea and a decreased likelihood of CKD. In contrast, research conducted by Mohsen et al. [Citation42], employing three various methodologies, assessed the relationship between caffeine consumption and the prevalence of CKD. Their findings did not establish a substantial link between the intake of caffeine and either the risk of CKD or the outcomes of kidney function tests. Additionally, their MR analysis did not reveal a causal connection between coffee consumption and changes in the estimated glomerular filtration rate (eGFR) or CKD incidence. This finding suggested that caffeine might not be a key risk factor for the development of CKD. Furthermore, the study by Yangchang et al. [Citation41] suggested that tea consumption might offer protection against CKD, positively influencing the eGFR and reducing albuminuria. Their MR findings indicate that increasing tea intake could be advantageous for kidney health. According to the study by Sehoon and associates [Citation43], elevated alcohol consumption may heighten the risk of end-stage kidney disease (ESKD) and overall mortality. Another investigation by Sehoon et al. [Citation44] revealed that higher consumption of vegetables might be beneficial for kidney health, particularly in maintaining an optimal eGFR.
Generally, social and economic status are factors that can contribute to maintaining healthy kidney function. Along with a well-balanced diet, adopting healthy daily habits and maintaining a positive mindset can also have a beneficial impact on preserving kidney function ().
Table 3. Lifestyle factor and renal function data.
Obesity factors and renal function
Recent studies have provided new insights into the complex relationships between various factors and CKD. Obesity is a well-known risk factor for several subclinical vascular diseases, including CKD and arterial stiffness. Chaojie et al.'s study further provided novel insights into the causal relationships of obesity with CKD and arterial stiffness, highlighting the importance of weight management in the primary prevention and control of subclinical vascular diseases [Citation45]. Matthew B. et al. reported no significant associations between genetically altered LDL cholesterol or triglyceride concentrations and kidney function. However, there is a need for a more detailed analysis of CKD outcomes in trials that involve interventions targeting HDL cholesterol. Notably, recent MR studies have revealed a modest yet significant causal relationship between higher HDL cholesterol levels and an increased glomerular filtration rate (GFR). In contrast, no such causal link has been established for LDL cholesterol. Furthermore, the findings of that study suggest that increasing HDL cholesterol concentrations may prevent CKD progression. The observed causal association also raises the hypothesis that HDL cholesterol may have separate causal pathways on glomerular microcirculation from those involved in coronary atherosclerotic disease [Citation46]. Adiponectin is another factor that has been linked to CKD risk. Using genetic data, Ruicheng et al.'s study provides robust causal evidence that adiponectin can protect kidney function and reduce the risk of CKD, not vice versa. The observed increase in adiponectin may be the result of kidney damage rather than the cause of kidney function injury. Nonetheless, adiponectin has been shown to improve insulin sensitivity and maintain homeostasis, which may be a mechanism underlying its protective effect on kidney function [Citation47]. Moreover, low birth weight has been associated with an increased risk of CKD. Research by Xinghao’s team has provided significant insights into how early life factors, specifically birth weight, influence the risk of developing CKD later in life. Their study corroborates prior observations that a lower birth weight is a risk factor for CKD. Moreover, unraveling the genetic interplay highlights that the roots of this correlation lie in both the direct impact of fetal development conditions and the indirect influence of genetic factors from the mother. These dual pathways are instrumental in determining the inverse relationship between an individual’s birth weight and their susceptibility to CKD [Citation48]. Overall, these recent studies have provided essential insights into the complex relationships between obesity and CKD risk. These findings highlight the importance of weight management in the primary prevention and control of subclinical vascular diseases and suggest potential interventions to mitigate CKD progression.
In their research, Pengfei and colleagues presented evidence suggesting a significant causal link between both central and general obesity and the development of CKD. That study revealed that when adjustments were made for factors such as diabetes status and BP measurements, the statistical significance of the relationships of the waist-to-hip ratio and body mass index (BMI) with CKD decreased considerably, with a 56% reduction in chi-square values. Mendelian randomization is useful for elucidating the mediating effects of relevant risk factors, and those effects are often underestimated in conventional observational studies. Moreover, when a multifaceted MR approach that included adjustments for potential mediators was applied, that study showed an even more pronounced decrease in the chi-square values for models based on the genetically predicted waist-to-hip ratio and BMI, by 83% and 69%, respectively. These findings from genetic analyses strongly support the hypothesis of a causal association between obesity, both in terms of central fat distribution and overall body mass, and the incidence of CKD [Citation49].
In summary, these studies offer significant insights into the causal associations of obesity factors with cardiovascular and kidney diseases. These insights may guide the development of new preventive and therapeutic strategies to mitigate the effects of these diseases ().
Table 4. Obesity factors and renal function.
Future CKD and MR research
Mendelian randomization is a statistical method that uses genetic variation to determine the causal relationship between an exposure and an outcome. Regarding CKD, MR has the potential to be used to identify modifiable risk factors that can be targeted for intervention to prevent or treat CKD. As mentioned above, we summarized the protective and risk factors for CKD separately. Recent MR studies have confirmed causal links between CKD or CVD and high BP. Focusing on the genetic architecture linking AF and kidney disorders may help to treat both problems more effectively. While there may be minor racial differences, factors that impact CVD incidence could also affect CKD incidence. Maintaining a healthy lifestyle, including a balanced diet and healthy daily habits, and having adequate social and economic status could contribute to preserving kidney function. Proper management of obesity may have favorable impacts on CKD, as the causal relationship between obesity, cardiovascular disease, and kidney disease has been further confirmed through new MR studies. These insights could lead to the development of potential therapeutic approaches to mitigate the effects of these diseases.
The future applications of MR in CKD research can be generally classified into two categories: (1) biomarkers for early detection and monitoring and (2) therapeutic targets for drug research and development. On the one hand, MR studies may also help to identify novel biomarkers for CKD. By identifying genetic variants that are associated with CKD outcomes, researchers may be able to develop new biomarkers for CKD that can be used for early detection and monitoring of this disease. On the other hand, the application of MR in CKD may help identify potential therapeutic targets by testing the causal relationship between genetic variants that are associated with molecular exposure (serum mRNA levels, protein levels, methylation levels, and so on) and CKD outcomes. For example, proteome-wide MR studies have identified potential targets for heart failure [Citation50] and inflammatory bowel disease. However, there is a paucity of related studies on CKD, and one study alone has identified 57 proteins as candidate targets for CKD treatment [Citation51]. By understanding the causal relationship between these molecular exposures and CKD, researchers may be able to develop targeted interventions to reduce the risk of CKD.
The application of MR to nephrology research is revolutionizing our understanding of CKD, yet several evolving challenges remain. As the field progresses, a key focus is the refinement of electronic phenotyping from electronic health records to ensure precise disease classification, which is foundational for accurate genetic association studies. Furthermore, interpreting the clinical significance of genetic variants remains complex; a deeper integration of functional genomics is necessary to unravel the implications of these genetic insights for CKD. Ensuring global representation in genetic studies is another crucial effort, as current databases heavily feature populations of European descent. This underrepresentation limits the applicability of MR findings and necessitates a concerted effort toward inclusive data sharing and international collaboration. Likewise, the development of polygenic risk scores demands larger and more diverse datasets to provide a fuller genetic risk profile for CKD. The journey from genetic associations to clinical applications involves translating epidemiological findings into actionable interventions, a challenge that calls for multidisciplinary collaboration. Moreover, the implementation of MR in clinical settings is hindered by technical complexity and cost, prompting a need for research into scalable, cost-effective MR applications that could improve CKD prognosis and personalize treatment protocols. Advancing the use of MR in nephrology research requires a holistic approach that addresses these challenges to maximize its potential and foster innovative strides in preventing, diagnosing, and managing CKD.
Conclusion
In summary, our review of the latest findings from Mendelian randomization (MR) research confirms the intricate connections between specific genetic markers and the risk of developing chronic kidney disease (CKD). This body of research underscores the innovative potential of MR in initializing preventative and therapeutic interventions in nephrology. The evidence suggests that certain genetic predispositions, such as elevated levels of sex hormone-binding globulin (SHBG) and bilirubin, are associated with a reduced risk of CKD. Conversely, factors such as high fasting glucose levels, insulin resistance, type 2 diabetes, and increased serum testosterone and homocysteine levels are associated with a greater risk of kidney dysfunction. Furthermore, a bidirectional link between CKD and cardiovascular diseases (CVDs) complicates the disease landscape, necessitating an integrative management approach. Lifestyle factors, in addition to socioeconomic conditions, also emerge as significant influencers of renal health, accentuating the need for a holistic prevention strategy. However, challenges remain in refining MR techniques, particularly in terms of precise disease classification, interpreting genetic variant impacts, and obtaining a globally representative genetic database. Addressing these issues requires a collaborative and international effort to harness the full potential of MR in combating CKD. It is worth noting that some of the health conditions that we have considered to be the cause of chronic kidney disease are risk factors. In this way, the paradigm of prevention and treatment will change, not only for chronic kidney disease but also for other diseases.
In conclusion, ongoing MR research highlights vital genetic relationships with CKD, offering a promising avenue for enhancing diagnosis, prognosis, and treatment strategies. Embracing a comprehensive and collaborative approach to resolving existing challenges will be crucial in diminishing the global impact of CKD.
Authors contributions
Chaofan Wen1 was responsible for guiding the main writing of the article. Lanlan Chen2 refined and revised the manuscript. Dan Jia3 was responsible for proofreading the article. Ziqi Liu4 was responsible for the data collection section of the article. Yidan Lin5 was responsible for the drafting of the article. GuanLiu6: Upon reviewing and editing the manuscript, we identified sections with a high similarity index when compared against databases. To address this issue, we diligently revised these passages to reduce the level of duplication. ShuoZhang7: Upon reviewing and editing the manuscript, we identified sections with a high similarity index when compared against databases. To address this issue, we diligently revised these passages to reduce the level of duplication. This step was essential for ensuring the originality of our work and adhering to the standards of academic integrity. Baoshan Gao1*: Responsible for guiding the overall planning of the article.
Consent form
All contributing authors consent to the publication of this study.
Ethical approval
This was a systematic review and did not require ethical review.
Acknowledgments
Science and Technology Plan Project of Jilin Province (20210101440JC).
Disclosure statement
No potential conflict of interest was reported by the author(s).
Additional information
Funding
References
- Davey Smith G, Hemani G. Mendelian randomization: genetic anchors for causal inference in epidemiological studies. Hum Mol Genet. 2014;23(R1):1–11. doi:10.1093/hmg/ddu328.
- Tin A, Köttgen A. Mendelian randomization analysis as a tool to gain insights into causes of diseases: a primer. J Am Soc Nephrol. 2021;32(10):2400–2407. doi:10.1681/ASN.2020121760.
- Burgess S, Dudbridge F, Thompson SG. Combining information on multiple instrumental variables in mendelian randomization: comparison of allele score and summarized data methods. Stat Med. 2016;35(11):1880–1906. doi:10.1002/sim.6835.
- Burgess S, Thompson SG. Interpreting findings from Mendelian randomization using the MR-Egger method. Eur J Epidemiol. 2017;32(5):377–389. doi:10.1007/s10654-017-0255-x.
- Bowden J, Davey Smith G, Haycock PC, et al. Consistent estimation in Mendelian randomization with some invalid instruments using a weighted median estimator. Genet Epidemiol. 2016;40(4):304–314. doi:10.1002/gepi.21965.
- Hartwig FP, Davey Smith G, Bowden J. Robust inference in summary data Mendelian randomization via the zero modal pleiotropy assumption. Int J Epidemiol. 2017;46(6):1985–1998. doi:10.1093/ije/dyx102.
- Verbanck M, Chen CY, Neale B, et al. Detection of widespread horizontal pleiotropy in causal relationships inferred from Mendelian randomization between complex traits and diseases. Nat Genet. 2018;50(5):693–698. doi:10.1038/s41588-018-0099-7.
- Morrison J, Knoblauch N, Marcus JH, et al. Mendelian randomization accounting for correlated and uncorrelated pleiotropic effects using genome-wide summary statistics. Nat Genet. 2020;52(7):740–747. doi:10.1038/s41588-020-0631-4.
- Chen L, Burgess S, Luo S, et al. First release of Mendelian randomisation book in Chinese. eGastroenterology. 2023;1(2):e100043. doi:10.1136/egastro-2023-100043.
- Wuttke M, Li Y, Li M, et al. A catalog of genetic loci associated with kidney function from analyses of a million individuals. Nat Genet. 2019;51(6):957–972. doi:10.1038/s41588-019-0407-x.
- Thomas MC, Cooper ME, Zimmet P. Changing epidemiology of type 2 diabetes mellitus and associated chronic kidney disease. Nat Rev Nephrol. 2016;12(2):73–81. doi:10.1038/nrneph.2015.173.
- Emanuelsson F, Marott S, Tybjærg-Hansen A, et al. Impact of glucose level on micro- and macrovascular disease in the general population: a Mendelian randomization study. Diabetes Care. 2020;43(4):894–902. doi:10.2337/dc19-1850.
- Zhao JV, Schooling CM. Sex-specific associations of insulin resistance with chronic kidney disease and kidney function: a bi-directional Mendelian randomization study. Diabetologia. 2020;63(8):1554–1563. doi:10.1007/s00125-020-05163-y.
- Xu M, Bi Y, Huang Y, et al. Type 2 diabetes, diabetes genetic score and risk of decreased renal function and albuminuria: a Mendelian randomization study. EBioMedicine. 2016;6:162–170. doi:10.1016/j.ebiom.2016.02.032.
- Park S, Lee S, Kim Y, et al. Mendelian randomization reveals causal effects of kidney function on various biochemical parameters. Commun Biol. 2022;5(1):713. doi:10.1038/s42003-022-03659-4.
- Kim H, Park S, Kwon SH, et al. Impaired fasting glucose and development of chronic kidney disease in non-diabetic population: a Mendelian randomization study. BMJ Open Diabetes Res Care. 2020;8(1):e001395. doi:10.1136/bmjdrc-2020-001395.
- Mazidi M, Kirwan R, Davies IG. Genetically determined blood lead is associated with reduced renal function amongst individuals with type 2 diabetes mellitus: insight from Mendelian randomisation. J Mol Med. 2022;100(1):125–134. doi:10.1007/s00109-021-02152-5.
- Ahmad S, Ärnlöv J, Larsson SC. Genetically predicted circulating copper and risk of chronic kidney disease: a Mendelian randomization study. Nutrients. 2022;14(3):509. doi:10.3390/nu14030509.
- Zhou A, Morris HA, Hyppönen E. Health effects associated with serum calcium concentrations: evidence from MR-PheWAS analysis in UK biobank. Osteoporos Int. 2019;30(11):2343–2348. doi:10.1007/s00198-019-05118-z.
- Fu S, Zhang L, Ma F, et al. Effects of selenium on chronic kidney disease: a Mendelian randomization study. Nutrients. 2022;14(21):4458. doi:10.3390/nu14214458.
- Zhao JV, Schooling CM. The role of testosterone in chronic kidney disease and kidney function in men and women: a bi-directional Mendelian randomization study in the UK biobank. BMC Med. 2020;18(1):122. doi:10.1186/s12916-020-01594-x.
- Zhao JV, Schooling CM. Sex-specific associations of sex hormone binding globulin with CKD and kidney function: a univariable and multivariable Mendelian randomization study in the UK biobank. J Am Soc Nephrol. 2021;32(3):686–694. doi:10.1681/ASN.2020050659.
- Chen L, Lv G. Horizons of human genetics in digestive disease gastroenterology. egastro. 2023;1(2):e100029. doi:10.1136/egastro-2023-100029.
- Xiong Y, Zhang Y, Zhang F, et al. Genetic evidence supporting the causal role of homocysteine in chronic kidney disease: a Mendelian randomization study. Front Nutr. 2022;9:843534. doi:10.3389/fnut.2022.843534.
- Staplin N, Herrington WG, Murgia F, et al. Determining the relationship between blood pressure, kidney function, and chronic kidney disease: insights from genetic epidemiology. Hypertension. 2022;79(12):2671–2681. doi:10.1161/HYPERTENSIONAHA.122.19354.
- Ku E, Lee BJ, Wei J, et al. Hypertension in CKD: core curriculum 2019. Am J Kidney Dis. 2019;74(1):120–131. doi:10.1053/j.ajkd.2018.12.044.
- Liu HM, Hu Q, Zhang Q, et al. Causal effects of genetically predicted cardiovascular risk factors on chronic kidney disease: a two-sample Mendelian randomization study. Front Genet. 2019;10:415. doi:10.3389/fgene.2019.00415.
- Zheng J, Zhang Y, Rasheed H, et al. Trans-ethnic Mendelian-randomization study reveals causal relationships between cardiometabolic factors and chronic kidney disease. Int J Epidemiol. 2022;50(6):1995–2010. doi:10.1093/ije/dyab203.
- Liu M, Li XC, Lu L, et al. Cardiovascular disease and its relationship with chronic kidney disease. Eur Rev Med Pharmacol Sci. 2014;18(19):2918–2926.
- Geurts S, van der Burgh AC, Bos MM, et al. Disentangling the association between kidney function and atrial fibrillation: a bidirectional Mendelian randomization study. Int J Cardiol. 2022;355:15–22. doi:10.1016/j.ijcard.2022.03.004.
- Yoshikawa M, Asaba K, Nakayama T. Causal effect of atrial fibrillation/flutter on chronic kidney disease: a bidirectional two-sample Mendelian randomization study. PLOS One. 2021;16(12):e0261020. doi:10.1371/journal.pone.0261020.
- Gaziano L, Sun L, Arnold M, et al. Mild-to-moderate kidney dysfunction and cardiovascular disease: observational and Mendelian randomization analyses. Circulation. 2022;146(20):1507–1517. doi:10.1161/CIRCULATIONAHA.122.060700.
- Sarnak MJ, Amann K, Bangalore S, et al. Chronic kidney disease and coronary artery disease: JACC state-of-the-Art review. J Am Coll Cardiol. 2019;74(14):1823–1838. doi:10.1016/j.jacc.2019.08.1017.
- Park S, Lee S, Kim Y, et al. Causal effects of physical activity or sedentary behaviors on kidney function: an integrated population-scale observational analysis and Mendelian randomization study. Nephrol Dial Transplant. 2022;37(6):1059–1068. doi:10.1093/ndt/gfab153.
- Park S, Lee S, Kim Y, et al. Causal effects of education on chronic kidney disease: a Mendelian randomization study. Clin Kidney J. 2020;14(8):1932–1938. doi:10.1093/ckj/sfaa240.
- Park S, Lee S, Kim Y, et al. Short or long sleep duration and CKD: a Mendelian randomization study. J Am Soc Nephrol. 2020;31(12):2937–2947. doi:10.1681/ASN.2020050666.
- Park S, Lee S, Kim Y, et al. Causal effects of positive affect, life satisfaction, depressive symptoms, and neuroticism on kidney function: a Mendelian randomization study. J Am Soc Nephrol. 2021;32(6):1484–1496. doi:10.1681/ASN.2020071086.
- Mazidi M, Kengne AP, Siervo M, et al. Association of dietary intakes and genetically determined serum concentrations of Mono and poly unsaturated fatty acids on chronic kidney disease: insights from dietary analysis and Mendelian randomization. Nutrients. 2022;14(6):1231. doi:10.3390/nu14061231.
- Park S, Lee S, Kim Y, et al. Causal effects of relative fat, protein, and carbohydrate intake on chronic kidney disease: a Mendelian randomization study. Am J Clin Nutr. 2021;113(4):1023–1031. doi:10.1093/ajcn/nqaa379.
- Kennedy OJ, Pirastu N, Poole R, et al. Coffee consumption and kidney function: a Mendelian randomization study. Am J Kidney Dis. 2020;75(5):753–761. doi:10.1053/j.ajkd.2019.08.025.
- Zhang Y, Xiong Y, Shen S, et al. Causal association between tea consumption and kidney function: a Mendelian randomization study. Front Nutr. 2022;9:801591. doi:10.3389/fnut.2022.801591.
- Mazidi M, Mikhailidis DP, Dehghan A, et al. The association between coffee and caffeine consumption and renal function: insight from individual-level data, Mendelian randomization, and meta-analysis. Arch Med Sci. 2022; 18(4):900–911. doi:10.5114/aoms/144905.
- Park S, Lee S, Kim Y, et al. Causal effect of alcohol use on the risk of end-stage kidney disease and related comorbidities: a Mendelian randomization study. Kidney Res Clin Pract. 2021;40(2):282–293. doi:10.23876/j.krcp.20.186.
- Park S, Lee S, Kim Y, et al. Observational or genetically predicted higher vegetable intake and kidney function impairment: an integrated population-scale cross-sectional analysis and Mendelian randomization study. J Nutr. 2021;151(5):1167–1174. doi:10.1093/jn/nxaa452.
- Ye C, Kong L, Zhao Z, et al. Causal associations of obesity with chronic kidney disease and arterial stiffness: a Mendelian randomization study. J Clin Endocrinol Metab. 2022;107(2):e825–e835. doi:10.1210/clinem/dgab633.
- Lanktree MB, Thériault S, Walsh M, et al. HDL cholesterol, LDL cholesterol, and triglycerides as risk factors for CKD: a Mendelian randomization study. Am J Kidney Dis. 2018;71(2):166–172. doi:10.1053/j.ajkd.2017.06.011.
- Wu R, Luo P, Luo M, et al. Genetically predicted adiponectin causally reduces the risk of chronic kidney disease, a bilateral and multivariable Mendelian randomization study. Front Genet. 2022;13:920510. doi:10.3389/fgene.2022.920510.
- Yu X, Yuan Z, Lu H, et al. Relationship between birth weight and chronic kidney disease: evidence from systematic review and two-sample Mendelian randomization analysis. Hum Mol Genet. 2020;29(13):2261–2274. doi:10.1093/hmg/ddaa074.
- Zhu P, Herrington WG, Haynes R, et al. Conventional and genetic evidence on the association between adiposity and CKD. J Am Soc Nephrol. 2021;32(1):127–137. doi:10.1681/ASN.2020050679.
- Henry A, Gordillo-Marañón M, Finan C, et al. Therapeutic targets for heart failure identified using proteomics and mendelian randomization. Circulation. 2022;145(16):1205–1217. doi:10.1161/CIRCULATIONAHA.121.056663.
- Matías-García PR, Wilson R, Guo Q, et al. Plasma proteomics of renal function: a transethnic meta-analysis and Mendelian randomization study. J Am Soc Nephrol. 2021;32(7):1747–1763. doi:10.1681/ASN.2020071070.