Abstract
Purpose
Heavy metal exposure can cause impaired or reduced pathology in the kidneys, lungs, liver, and other vital organs. However, the relationship between heavy metal exposure and kidney stones has not been determined. The goal of this research was to determine the association between heavy metal exposure and kidney stones in a population of American adults in general.
Materials and methods
We evaluated 29,201 individuals (≥20 years) from the National Health and Nutrition Examination Survey (NHANES). The association between heavy metal exposure and kidney stones was verified by multiple logistic regression and restricted cubic spline (RCS) regression. Dose–response curves were generated to analyze the relationship between heavy metal concentrations and the occurrence of kidney stones. Moreover, we used propensity score matching (PSM) to exclude the effect of confounding variables.
Results
After a rigorous enrollment screening process, we included 8518 participants. Logistic regression showed that urinary cadmium (U-Cd) and urinary cobalt (U-Co) concentrations were significantly different in the kidney stone group before PSM (p < 0.001). Dose–response curves revealed that the occurrence of kidney stones increased significantly with increasing U-Cd and U-Co concentrations. After adjustment for covariates, only biomarkers of U-Co were linked to the occurrence of kidney stones. When the lowest quartile was used as a reference, the 95% confidence intervals (95% CIs) for kidney stones across the other quartiles were 1.015 (0.767–1.344), 1.409 (1.059–1.875), and 2.013 (1.505–2.693) for U-Cos (p < 0.001).
Conclusion
In the U.S. population, high U-Co levels are positively correlated with the potential risk of kidney stones.
Introduction
The formation of kidney stones is the best-known disease of the urinary tract and constitutes an enormous health care burden among the working age population. The prevalence of kidney stones has risen over the past decades, possibly due to changes in nutritional and lifestyle factors [Citation1]. Moreover, the incidence of this disease appears to be increasing in almost all countries [Citation2]. In the United States, the prevalence of self-reported kidney stones nearly tripled, from 3.2% in 1976–1980 to 8.8% in 2007–2010 [Citation3]. Kidney stones can also lead to many serious complications, pain, hematuria, infection, and decreased kidney function. As a result, kidney stones are a considerable burden and a problem for the public health care system, with total health care expenditures for kidney stones exceeding $2 billion annually in the United States [Citation4].
Epidemiological investigations of kidney stones have shown that dietary and lifestyle habits may play important roles [Citation5]. The recognized risk factors for stone formation include obesity, diabetes, hypertension, and metabolic syndrome [Citation2]. Metals, especially heavy metals, are used in a wide range of industries, including the medical industry. The general routes of exposure to environmental metals include ingestion of contaminated food and water, inhalation of ambient air, and dermal contact [Citation6]. Low levels of metal exposure have been epidemiologically reported to potentially cause impairment of multiple organ functions [Citation7,Citation8]. Several well-known heavy metals, including arsenic (As), lead (Pb), and mercury (Hg), are associated with increased risk of cardiovascular disease, kidney disease, bone disease, and cancer.
The nephrotoxicity of metals has also been extensively studied. One of the mechanisms of heavy metal-induced nephrotoxicity is oxidative stress [Citation9]. Hypercalciuria, impaired renal function, and proteinuria were observed when urinary cadmium (U-Cd) concentration exceeded 2.0 μg/g creatinine, blood Pb levels were 5 μg/dL or greater, or urinary Hg exceeded 50 μg/g creatinine [Citation10,Citation11]. However, it is not known whether low exposure to metals in the general population enhances the potential hazard of nephrotoxicity [Citation12]. Investigations in normal populations have shown that higher blood Cd or Pb concentrations or elevated urinary chromium (Cr) or Pb concentrations [Citation13] are correlated with a lower estimated glomerular filtration rate (eGFR). However, other studies have also shown that elevated urinary Cd, Pb, and As levels are related to elevated eGFR. Kidney stones and atherosclerosis may be common risk factors [Citation9]. Research has demonstrated that the main mechanism of the potential atherogenic effect of multiple metals, such as Cd and cobalt (Co), is through the induction of oxidative stress [Citation14]. However, evidence linking Cd and Co exposure to the risk of kidney stones is scarce. Thus, we hypothesized that nephrotoxic Cd and Co exposure may be relevant to the incidence of kidney stones, and the purpose of this research was to investigate the exposure–response relationships between biomarkers of Cd and Co in blood and urine and the incidence of kidney stones in the National Health and Nutrition Examination Survey (NHANES) from 2007 to 2016.
Study design and participants
In this study, all the data were collected from the NHANES, which is a nationally representative survey program administered by the National Center for Health Statistics. The NHANES primarily reflects civilian non-institutionalized resident population information that utilizes a sophisticated stratified multistage sampling design. The interviews focused on diet and health-related issues. The study protocol for the project was endorsed by the NHANES Institutional Review Board. All participants provided informed consent while the survey was in progress.
This research consisted of 29,201 individuals (≥20 years) from the NHANES 2007–2008, 2009–2010, 2011–2012, 2013–2014, and 2015–2016 cycles. Patients were excluded if they had any of the following: (1) had no kidney stone information (n = 80); (2) had unknown heavy metal exposure (n = 19,955); (3) had unknown education, marital status, or body mass index (BMI) (n = 109); (4) had hypertension or diabetes (n = 11); or (5) had unknown blood creatinine, urine creatinine, and uric acid levels (n = 528). Finally, 8518 participants were included for analysis ().
Exposure and outcomes
Urinary Co (U-Co) and U-Cd were the major forecasters in this research. U-Co and U-Cd concentrations were measured via inductively coupled plasma dynamic reaction cell mass spectrometry.
The major outcome of the study was a history of kidney stones. Based on the answers to the question ‘Have you ever had kidney stones?’, the participants who answered ‘Yes’ to the question were divided into kidney stone groups; otherwise, they were divided into non-kidney stone groups.
Covariates determination
Based on previous studies [Citation15,Citation16], relevant covariates were identified. Continuous variables included age, BMI, blood urea nitrogen, creatinine, uric acid, and urine creatinine. eGFR and categorical variables included race (non-Hispanic White/non-Hispanic Black/Mexican American/other Hispanic/other), education level (less than high school/high school or equivalent/college or above), sex (male/female), marital status (married/unmarried), hypertension, diabetes, and vigorous recreational activities.
Statistical analysis
Continuous variables are presented as the mean ± standard deviation, and weighted t tests for continuous variables and weighted χ2 tests for categorical variables were applied to determine significant differences. Multivariate logistic regression models were applied to assess the corrected odds ratio (OR) and corresponding 95% confidence intervals (CIs) to determine the incidence of kidney stones associated with urinary metals. In the extended model, Model 1 involved univariate analysis without adjustment; Model 2 included sex, age, education level, race, and marital status; Model 3 included BMI, hypertension, diabetes status, vigorous recreational activities, and moderate recreational activities; and Model 4 included the same factors as Model 3 but included additional variables, such as blood urea nitrogen, creatinine, uric acid, eGFR, and urine creatinine.
The powerful tool restricted cubic spline (RCS) was applied to describe dose–response relationships between continuous variables and outcomes [Citation17] and was also utilized in our research to characterize the dose–response relationships among Cd concentrations and Co concentrations and kidney stone risk adjusted for model variables. All the statistical analyses were conducted using IBM SPSS version 20.0 software (IBM, Armonk, NY) and GraphPad Prism version 8 software (GraphPad Software, Inc., La Jolla, CA). p Values less than 0.05 were considered to indicate statistical significance.
Results
Patient characteristics
A total of 29,201 individuals (≥20 years) from the NHANES (2007–2016) were enrolled. After screening, 8518 participants met the inclusion criteria (). The basic demographic features are presented in . A total of 7699 (90.4%) participants were stratified into the non-kidney stone group, and 819 (9.6%) participants were distributed into the kidney stone group. Education level and moderate recreational activity did not significantly differ between the two groups (p > 0.05). The other variables, including urine creatinine concentration, eGFR, uric acid concentration, creatinine concentration, blood urea nitrogen concentration, vigorous recreational activities, diabetes status, hypertension status, BMI, marital status, race, age, and sex, were significantly different between the two groups (p < 0.05).
Table 1. Baseline characteristics of NHANES participants between 2007 and 2016 (n = 8518).Table Footnotea
Profiles of urinary heavy metals
First, we examined the distribution of urinary heavy metals in the study population with and without kidney stones in the NHANES 2007–2016 before propensity score matching (PSM; Table S1). We found that U-Cd and U-Co were significantly increased in the kidney stone group. Spearman’s rank correlation coefficients for urinary heavy metals, creatinine, and eGFR are shown in Figure S1.
U-Co and U-Cd and kidney stones
The dose–response relationships between U-Co concentrations and U-Cd concentrations and kidney stones are displayed in . The multivariate adjusted RCS plot revealed that there was a nonlinear association between log2-transformed U-Cd/U-Cr levels and log2-transformed U-Co/U-Cr levels and the incidence of kidney stones (). The occurrence of kidney stones increased with increasing U-Co and U-Cd concentrations. Each urinary metal concentration was divided into quartiles, with the lowest quartile (Q1) serving as the reference group. Multivariate logistic regression analysis of the correlations between adjusted U-Cd and U-Co concentrations and kidney stones was carried out with four models (, ). When Q1 was used as a reference in Model 4 (), the OR for Q2 was 1.483 (95% CI, 1.172–1.878; p < 0.001), the OR for Q3 was 1.216 (95% CI, 0.945–1.565; p < 0.001), and the OR for Q4 was 1.663 (95% CI, 1.277–2.167; p < 0.001); these values were consistent with those of the other three models. The characteristics of the study population by U-Cd level according to the NHANES (2007–2016) before PSM are shown in Table S2. We observed that the p values for all covariates were less than 0.05 in the four models.
Figure 2. Relative risk for kidney stones based on U-Cd and U-Co level before PSM. The shaded areas represent upper and lower 95% CIs. Adjustment factors are as same as which presented in extended model 4. Restricted cubic spline (RCS) plot of the association between log2-transformed U-Cd/U-Cr and U-Co/U-Cr levels and kidney stones. The solid and dashed lines represent the odds ratios and 95% confidence intervals. (A) U-Cd; (B) U-Co; (C) log2-transformed U-Cd/U-Cr; (D) log2-transformed U-Co/U-Cr.
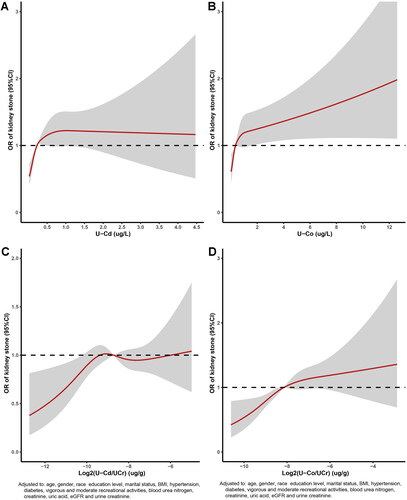
Figure 3. Adjusted odds ratios for associations between the U-Cd and U-Co and the presence of kidney stones in NHANES 2007–2016 before PSM. (A) U-Cd; (B) U-Co.
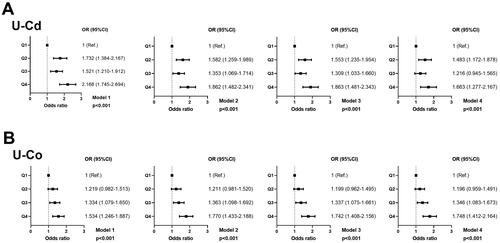
Table 2. Adjusted odds ratios for associations between the heavy metals and the presence of kidney stone in NHANES 2007–2016 before PSM.Table Footnotea
For U-Co (), the OR for Q2 was 1.196 (95% CI, 0.959–1.491; p < 0.001), the OR for Q3 was 1.346 (95% CI, 1.083–1.673; p < 0.001), and the OR for Q4 was 1.748 (95% CI, 1.412–2.164; p < 0.001); these values also remained consistent with those of the other three models. The demographic features according to U-Co level before PSM are presented in Table S3. Education level, vigorous recreational activities, moderate recreational activities, and blood urea nitrogen were similar among those models.
Association after PSM
Due to large differences between the non-kidney stone group and the kidney stone group, we performed PSM on the participants. The results after PSM are shown in and Figure S2. Moreover, we evaluated the distribution levels of urinary heavy metals in participants with and without kidney stones (Table S4). However, only U-Co exhibited a significant difference between the two groups (p < 0.001), and the p value of U-Cd was 0.786. The demographic features according to U-Cd and U-Co levels after PSM are presented in Tables S5 and S6, respectively.
Spearman’s rank correlation coefficients for urinary heavy metals, creatinine, and eGFR are presented in Figure S3. The four models were validated again by multivariate logistic regression after PSM (Figure S4 and Table S7). When Q1 was used as a reference in Model 4 (Figure S4(A)), the ORs for Q2, Q3, and Q4 were 1.147 (0.835–1.574), 0.785 (0.563–1.093), and 0.870 (0.619–1.221) (p for trend = 0.060), respectively, and remained consistent with those of the other three models. For U-Co (Figure S4(B)), the ORs across U-Co quartiles were 1.015 (0.767–1.344), 1.409 (1.059–1.875), and 2.013 (1.505–2.693) (all p for trend <0.001) and remained consistent in the other three models. The dose–response risk curve also suggested that there was a nonlinear relationship between log2-transformed U-Co levels and the incidence of kidney stones ().
Figure 5. Relative risk for kidney stones based on U-Cd and U-Co level after PSM. The shaded areas represent upper and lower 95% CIs. Adjustment factors are as same as which presented in extended model 4. Restricted cubic spline (RCS) plot of the association between log2-transformed U–Cd/UCr and U–Co/UCr levels and kidney stones. The solid and dashed lines represent the odds ratios and 95% confidence intervals. (A) U-Cd; (B) U-Co; (C) log2-transformed U-Cd/U-Cr; (D) log2-transformed U-Co/U-Cr.
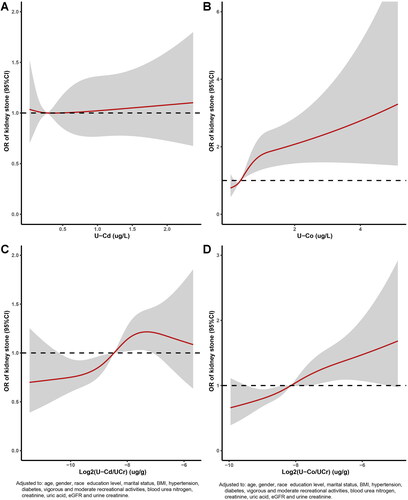
Discussion
The high incidence and recurrence rate of kidney stones have severe impacts on patients’ lives, simultaneously causing great social and economic losses. Therefore, it is urgent to explore the risk factors for kidney stones to reduce social and economic burdens and to improve the postoperative recovery of patients. In this study, we assessed the association between U-Co and Cd concentrations and kidney stone odds. First, we searched for urinary heavy metals associated with kidney stones. We found that the occurrence of kidney stones increased significantly with increasing U-Cd and U-Co concentrations. This was also confirmed by the dose–response curve. To exclude the effects of other variables as much as possible, PSM was used in our study. After adjusting for covariates, only biomarkers of U-Cos (p < 0.001) were linked to the occurrence of kidney stones.
With the development of industry and the aggravation of environmental pollution, various heavy metal pollutants have also received increasing amounts of attention. Increasing evidence has shown that long-term exposure to low concentrations of heavy metals may lead to a variety of medical conditions. One study demonstrated that urinary thallium intake is associated with cardiovascular disease [Citation18]. Multiple heavy metals in the human body have been studied and found to be associated with dyslipidemia and hypertension [Citation19,Citation20]. In addition, Pb and Cd suppress or damage endocrine and vascular endothelial functions and may cause atherosclerosis [Citation21,Citation22]. The formation of kidney stones was also closely related to heavy metal exposure, especially calcium exposure. A survey of a large sample of the general population showed that the nephrotoxic metals Cd, Pb, mercury, and arsenic were associated with the occurrence of kidney stones [Citation23].
Co is essential for human cellular metabolism as the active center of a group of coenzymes, which are part of the B12 vitamin family. Thus, it is considered an essential trace mineral [Citation24]. Glass, paint, and diet are the main sources of Co exposure in the general population, as are occupational exposure while working in the metal industry [Citation25,Citation26]. High levels of Co were noted to cause vision changes, peripheral neuropathy, hearing loss, hypothyroidism, and even cancer. Additionally, Co causes cardiovascular disease, including cardiomyopathy [Citation27]. An increasing number of studies have shown that the occurrence of kidney stones is related to oxidative stress and inflammation. Excess reactive oxygen species induce renal tubular epithelial cell damage and apoptosis. Apoptosis and changes in cell membrane structure can result in crystal adhesion and eventually trigger a cascade of kidney stones [Citation28,Citation29]. Co, a redox-active metal, can undergo redox cycling reactions and can generate reactive radicals such as superoxide anion radicals and nitric substances in biological systems [Citation30,Citation31]. Our study provided preliminary confirmation of the relationship between U-Co exposure and the incidence of kidney stones. U-Cos may contribute to the development and progression of kidney stones through increased oxidative stress.
Conclusion
We examined the associations between U-Co and the occurrence of kidney stones while controlling for potential variables. This study has several limitations. First, because this was a cross-sectional study, we were not sure about the relationship between U-Co exposure and kidney stones. However, further prospective studies are needed to verify its accuracy. Second, no consideration was given to the relationship between U-Co and kidney stone type. Third, our study lacked information on the potential pathological mechanisms underlying the correlation between nephrotoxic metal exposure and kidney stones. Finally, the frequency or extent of participants’ exposure to nephrotoxic metals could not be confirmed due to data limitations in the NHANES database. Thus, longitudinal studies are needed to confirm these findings. Given the severity of the correlation, interventions to reduce human exposure to Co may have implications for the primary prevention of kidney stones. However, further studies are needed to explore the potential underlying mechanisms involved and to identify causal relationships involved.
Ethical approval statement
The authors are accountable for all aspects of the work in ensuring that questions related to the accuracy or integrity of any part of the work are appropriately investigated and resolved. The study was conducted in accordance with the Declaration of Helsinki (as revised in 2013). This study used previously collected deidentified data, which were deemed exempt from review by the Ethics Committee of Shanghai Fourth People’s Hospital, School of Medicine, Tongji University.
Consent to participate
Not applicable.
Consent to publish
Not applicable.
Author contributions
(I) Conception and design: Jun Lu, Cheng Li. (II) Administrative support: Dongmei Hong and Qian Wu. (III) Provision of study materials or patients: Jun Lu and Yinghui Xia. (IV) Collection and assembly of data: Guozhong Chen and Tie Zhou. (V) Data analysis and interpretation: Dongmei Hong, Qian Wu, and Cheng Li. (VI) Manuscript writing: all authors. (VII) Final approval of manuscript: all authors.
Supplemental Material
Download PDF (959.1 KB)Acknowledgments
The authors thank the National Center for Health Statistics of the Centers for Disease Control and Prevention for sharing the data.
Disclosure statement
The authors declare that they have no competing interests.
Data availability statement
The data that support the findings of this study are available from the corresponding author upon reasonable request.
Additional information
Funding
References
- Thongprayoon C, Krambeck AE, Rule AD. Determining the true burden of kidney stone disease. Nat Rev Nephrol. 2020;16(12):1–9. doi: 10.1038/s41581-020-0320-7.
- Khan SR, Pearle MS, Robertson WG, et al. Kidney stones. Nat Rev Dis Primers. 2016;2(1):16008. doi: 10.1038/nrdp.2016.8.
- Scales CJ, Smith AC, Hanley JM, et al. Prevalence of kidney stones in the United States. Eur Urol. 2012;62(1):160–165. doi: 10.1016/j.eururo.2012.03.052.
- Pearle MS, Calhoun EA, Curhan GC. Urolithiasis. J Urol. 2005;173(3):848–857. doi: 10.1097/01.ju.0000152082.14384.d7.
- Jung H, Andonian S, Assimos D, et al. Urolithiasis: evaluation, dietary factors, and medical management: an update of the 2014 SIU-ICUD international consultation on stone disease. World J Urol. 2017;35(9):1331–1340. doi: 10.1007/s00345-017-2000-1.
- Li Z, Feng X, Li G, et al. Distributions, sources and pollution status of 17 trace metal/metalloids in the street dust of a heavily industrialized city of Central China. Environ Pollut. 2013;182:408–416. doi: 10.1016/j.envpol.2013.07.041.
- Järup L. Hazards of heavy metal contamination. Br Med Bull. 2003;68(1):167–182. doi: 10.1093/bmb/ldg032.
- Tchounwou PB, Yedjou CG, Patlolla AK, et al. Heavy metal toxicity and the environment. Exp Suppl. 2012;101:133–164. doi: 10.1007/978-3-7643-8340-4_6.
- Orr SE, Bridges CC. Chronic kidney disease and exposure to nephrotoxic metals. Int J Mol Sci. 2017;18(5):1039. doi: 10.3390/ijms18051039.
- Ekong EB, Jaar BG, Weaver VM. Lead-related nephrotoxicity: a review of the epidemiologic evidence. Kidney Int. 2006;70(12):2074–2084. doi: 10.1038/sj.ki.5001809.
- Roels HA, Hoet P, Lison D. Usefulness of biomarkers of exposure to inorganic mercury, lead, or cadmium in controlling occupational and environmental risks of nephrotoxicity. Ren Fail. 1999;21(3–4):251–262. doi: 10.3109/08860229909085087.
- Lunyera J, Smith SR. Heavy metal nephropathy: considerations for exposure analysis. Kidney Int. 2017;92(3):548–550. doi: 10.1016/j.kint.2017.04.043.
- Tsai TL, Kuo CC, Pan WH, et al. The decline in kidney function with chromium exposure is exacerbated with co-exposure to lead and cadmium. Kidney Int. 2017;92(3):710–720. doi: 10.1016/j.kint.2017.03.013.
- Valko M, Morris H, Cronin MT. Metals, toxicity and oxidative stress. Curr Med Chem. 2005;12(10):1161–1208. doi: 10.2174/0929867053764635.
- Thomas LD, Elinder CG, Tiselius HG, et al. Dietary cadmium exposure and kidney stone incidence: a population-based prospective cohort study of men & women. Environ Int. 2013;59:148–151. doi: 10.1016/j.envint.2013.06.008.
- Ferraro PM, Taylor EN, Gambaro G, et al. Caffeine intake and the risk of kidney stones. Am J Clin Nutr. 2014;100(6):1596–1603. doi: 10.3945/ajcn.114.089987.
- Desquilbet L, Mariotti F. Dose-response analyses using restricted cubic spline functions in public health research. Stat Med. 2010;29(9):1037–1057. doi: 10.1002/sim.3841.
- Wang S, Sun J, Tang C, et al. Association between urinary thallium exposure and cardiovascular disease in US adult population. Chemosphere. 2022;294:133669. doi: 10.1016/j.chemosphere.2022.133669.
- Huang YQ, Shen G, Lo K, et al. Association of circulating selenium concentration with dyslipidemia: results from the NHANES. J Trace Elem Med Biol. 2020;58:126438. doi: 10.1016/j.jtemb.2019.126438.
- Wang H, Li F, Xue J, et al. Association of blood cobalt concentrations with dyslipidemia, hypertension, and diabetes in a US population: a cross-sectional study. Medicine (Baltimore). 2022;101(2):e28568. doi: 10.1097/MD.0000000000028568.
- Iavicoli I, Fontana L, Bergamaschi A. The effects of metals as endocrine disruptors. J Toxicol Environ Health B Crit Rev. 2009;12(3):206–223. doi: 10.1080/10937400902902062.
- Prozialeck WC, Edwards JR, Nebert DW, et al. The vascular system as a target of metal toxicity. Toxicol Sci. 2008;102(2):207–218. doi: 10.1093/toxsci/kfm263.
- Sun Y, Zhou Q, Zheng J. Nephrotoxic metals of cadmium, lead, mercury and arsenic and the odds of kidney stones in adults: an exposure-response analysis of NHANES 2007–2016. Environ Int. 2019;132:105115. doi: 10.1016/j.envint.2019.105115.
- Rizzo G, Laganà AS, Rapisarda AMC, et al. Vitamin B12 among vegetarians: status, assessment and supplementation. Nutrients. 2016;8(12):767. doi: 10.3390/nu8120767.
- Barceloux DG. Cobalt. J Toxicol Clin Toxicol. 1999;37(2):201–206. 119 doi: 10.1081/clt-100102420.
- Lauwerys R, Lison D. Health risks associated with cobalt exposure–an overview. Sci Total Environ. 1994;150(1–3):1–6. doi: 10.1016/0048-9697(94)90125-2.
- Milon H, Morin Y, Bonenfant JL. [Epidemic of myocardosis in beer drinkers. Probable role of cobalt]. Arch Mal Coeur Vaiss. 1968;61(11):1561–1594.
- Thamilselvan V, Menon M, Thamilselvan S. Oxalate at physiological urine concentrations induces oxidative injury in renal epithelial cells: effect of alpha-tocopherol and ascorbic acid. BJU Int. 2014;114(1):140–150. doi: 10.1111/bju.12642.
- Kizivat T, Smolic M, Maric I, et al. Antioxidant pre-treatment reduces the toxic effects of oxalate on renal epithelial cells in a cell culture model of urolithiasis. Int J Environ Res Public Health. 2017;14(1):109.
- Matés JM, Segura JA, Alonso FJ, et al. Intracellular redox status and oxidative stress: implications for cell proliferation, apoptosis, and carcinogenesis. Arch Toxicol. 2008;82(5):273–299. doi: 10.1007/s00204-008-0304-z.
- Matés JM, Pérez-Gómez C, Núñez de Castro I, et al. Glutamine and its relationship with intracellular redox status, oxidative stress and cell proliferation/death. Int J Biochem Cell Biol. 2002;34(5):439–458. doi: 10.1016/s1357-2725(01)00143-1.