Abstract
Acetaminophen (APAP)-induced acute kidney injury (APAP-AKI) has turned into one of reasons for clinic obtained renal insufficiency. Magnesium hydride (MgH2), as a solid-state hydrogen source, might be potentially applied in clinical practice. The current study aimed to investigate the protective effect of MgH2 against APAP-AKI. The results showed that MgH2 improved renal function and histological injury in mice of APAP-AKI. MgH2 also had protective effects on APAP-induced cytotoxicity in HK-2 cells. In addition, the increased level of reactive oxygen species (ROS) and expressions of inflammatory cytokines (TNF-α and IL-1β) and pro-apoptotic factors (Bad, Bax, Caspase3, and CytC) induced by APAP were downregulated with MgH2 treatment. Furthermore, the expressions of molecules related to TXNIP/NLRP3/NF-κB pathway (TXNIP, NLRP3, NF-κB p65 and p-NF-κB p65) in renal tissues and HK-2 cells were enhanced by APAP overdose, which were reduced by MgH2 administration. Collectively, this study indicated that MgH2 protects against APAP-AKI by alleviating oxidative stress, inflammation and apoptosis via inhibition of TXNIP/NLRP3/NF-κB signaling pathway.
1. Introduction
Acetaminophen (APAP), also known as paracetamol, is commonly used as an analgesic and antipyretic agent in clinical practice. However, excessive, combined, or long-term medication can lead to significant hepatic and renal damage, even death. Previous survey showed that renal insufficiency occurs in approximately 1–12% of patients with APAP overdose [Citation1–3]. The mechanisms of APAP-induced acute kidney injury (APAP-AKI) are very complex and need further investigation. It has been reported that oxidative stress, inflammatory response, mitochondrial dysfunction, apoptosis, endoplasmic reticulum stress and changes in glomerular hemodynamics are implicated in this process [Citation4]. Oxidative stress is one of the most critical and direct factors leading to APAP-AKI. APAP is metabolized by cytochrome P450 to N-acetyl-p-benzoquinone imine (NAPQI), which is reduced by glutathione (GSH) at therapeutic doses. Excessive APAP leads to elevated NAPQI level and GSH depletion, resulting in the accumulation of endogenous reactive oxygen species (ROS). ROS will further initiate inflammatory response and apoptosis from different signals, promoting APAP-AKI [Citation5,Citation6]. Some natural products that possess antioxidant effects can elucidate protective effect against APAP-AKI [Citation7]. However, there is lack of specific antidote for APAP-AKI. For this purpose, mechanistic insight into APAP-AKI would enable development of targeted therapeutic approaches.
Thioredoxin (TRX)-interacting protein (TXNIP) has been shown to be a key signaling molecule that links oxidative stress to inflammasome activation [Citation8]. Previous studies have found that TXNIP/NOD-like receptor protein 3 (NLRP3) inflammasome activation is associative with the development of APAP-induced liver injury. Specifically, APAP-induced ROS overproduction promotes the separation of TXNIP from TRX-1 and further linkage to NLRP3 inflammasome, aggravating hepatic damage. Besides, this condition can be inhibited by nuclear factor-erythroid 2-related factor 2 (Nrf2) activation [Citation9]. Nrf2 belongs to the leucine zipper family and is an important transcription factor regulating oxidative stress. Nrf2 induction suppressing TXNIP/NLRP3 signaling is not only associated with elimination of ROS, but also enhanced transcription of TRX-1 [Citation10]. It has been reported that induction of Nrf2 exerts protective effects against oxidative stress, inflammation, and apoptosis in the progress of renal injury in vivo and in vitro model of APAP-AKI [Citation4,Citation11]. In addition, NLRP3 inflammasome activation exists in APAP-AKI and inhibition of NLRP3 can alleviate renal injury [Citation12]. However, it is not clarified whether TXNIP and its mediated signaling play a significant role in APAP-AKI.
Molecular hydrogen (H2), a small molecule with the capacity to efficiently enter the plasma membrane and other cell organelles, exerts multiple biological effects involving anti-oxidation, anti-inflammation, anti-apoptosis, and anti-fibrosis in many diseases of different systems [Citation13]. Previous studies have demonstrated that H2 can protect cisplatin-induced, ferric nitrilotriacetate-induced, cyclosporine A-induced, sepsis-induced, oxalate-induced and glycerol-induced renal injury [Citation14–19]. So far, there is no report on the protective effect of H2 on APAP-AKI. Magnesium hydride (MgH2), as a solid-state H2 source with high H2-storage capacity, can produce a desired quantity of H2 following hydrolysis reaction [Citation20]. As the by-product is poison-free, it is possible to utilize MgH2 for biological application in clinical practice. Therefore, this study investigated the preventive effects of MgH2 on oxidative stress, inflammation and apoptosis in APAP-AKI. We hypothesized that MgH2 attenuates APAP-AKI via suppressing TXNIP/NLRP3 signaling.
2. Materials and methods
2.1. Animal experiments
Eight-week-old male C57BL/6 mice (n = 24) were purchased from Shanghai SLAC Lab Animal Co., Ltd. (Shanghai, China) and allowed to acclimatize to the lab environment for one week. The mice were kept under controlled conditions, temperature 25 ± 2 °C, humidity 55 ± 5%, 12 h light/dark cycles, and were given free access to food and tap water. The animal protocols were approved by Committee on Ethic of Medical Research, Naval Medical University (No: NMUMREC-2021–006). The mice were randomly assigned into four groups: Group 1 (Control group, n = 6) was administered with an equivalent measure of isotonic saline water by stomach tube for 3 days and administered with equal isotonic saline on the third day by intraperitoneal injection (i.p.); Group 2 (APAP group, n = 6) was administered with equal isotonic saline water intragastrically for 3 days and administered with APAP (500 mg/kg, i.p.) on the third day [Citation4]; Group 3 [Low dose MgH2 (LMH) group, n = 6] was administered with low dose MgH2 (50 mg/kg) intragastrically for 3 days and administered with APAP on the third day; Group 4 [High dose MgH2 (HMH) group, n = 6] was administered with high dose MgH2 (100 mg/kg) for 3 days and administered with APAP on the third day. APAP was purchased from the MedChem Express (Shanghai, China) and MgH2 was purchased from the Kemike Wuhan New Material Technology Co., Ltd (Wuhan, China). At the end of the experiment, the mice were all anesthetized. Blood was collected by eyeball extraction and serum was obtained after centrifugation at 3000 rpm for 10 min and stored at −80 °C. After in situ cardio-perfusion, the right kidneys were immediately removed and stored at −80 °C. Next, the left kidneys were removed and fixed in 10% buffered formalin.
2.2. Determination of biochemical indexes
The levels of serum creatinine (SCr) and blood urea nitrogen (BUN) were detected by creatinine assay and urea assay kits respectively (Jiancheng Bioengineering, China).
2.3. Histology and immunohistochemical staining
The kidney samples that fixed in 10% buffered formalin were embedded in paraffin and sectioned at a thickness of 4 μm and submitted to hematoxylin and eosin (HE) staining. For Masson staining, the hydrated sections were stained with Masson’s Trichrome stain kit (Servicebio, China) according the manufacturer’s instruction. Periodic acid Schiff (PAS) staining was carried out using a Periodic acid-Schiff stain kit (Servicebio, China) according to the supplier’s instructions. For immunohistochemistry analysis, the sections were incubated with citrate antigen retrieval solution for 20 min at 95 °C. After that, the sections were incubated with primary antibodies against TXNIP (1:200, Cloud-Clone, China) and p-nuclear factor-κB (NF-κB) p65 (1:200, Cell Signaling Technology, USA) overnight at 4 °C and then with HRP-tagged goat anti-rabbit antibody (1:500, Proteintech, USA) at 37 °C for 50 min. Finally, the sections were stained with diaminobenzidine and counter-stained with hematoxylin.
2.4. Terminal deoxynucleotidyl transferase dUTP Nick-End labeling (TUNEL) assay
The TUNEL assay was carried out using the TUNEL apoptosis Assay Kit (Beyotime Biotechnology, China). The kidney sections were pretreated with proteinase K (20 mg/mL) for 20 min at 37 °C and washed three times with PBS and then incubated with the TUNEL reaction mixture for 1 h at 37 °C in a dark chamber. Confocal microscopy was then used to observe the positive staining.
2.5. Cell culture and treatment
The human renal proximal tubular epithelial cell line (HK-2) was purchased from American Type Culture Collection (ATCC, USA). HK-2 cells were cultured in Dulbecco’s Modified Eagle Medium/F12 (DMEM/F12; HyClone, USA) supplemented with 10% fetal bovine serum and 1% penicillin/streptomycin (Gibco, USA) and brooded at 37 °C in a 5% CO2 incubator. Cells were divided into four groups: (1) Control group was treated with PBS at an indicated concentration and time; (2) APAP group was pretreated with PBS for 24 h and incubated with APAP (10 mM) for 24 h; (3) LMH group was treated with low dose MgH2 (0.2 mM) 24 h prior to the application of APAP; (4) HMH group was treated with high dose MgH2 (0.4 mM) 24 h prior to the application of APAP. The concentrations of APAP and MgH2 were selected based on the results of the Cell Counting Kit-8 (CCK-8) assay.
2.6. Cell viability test
CCK-8 (Yeasen Biotechnology, China) was used to detect cell viability. HK-2 cells were seeded in 96-well plates at 2000 cells/well and then treated with PBS, APAP only, MgH2 only or APAP and MgH2 after 24 h of culture. Approximately 10 μL of CCK8 reagent was added to each well and incubated at 37 °C for 2 h. The optical density (OD) value was measured at a wavelength of 450 nm using a micro-plate reader.
2.7. Mitochondrial membrane potential (MMP) assay
The MMP level in HK-2 cells was determined using the JC-1 assay (MedChemExpress, USA). Cells were washed with PBS and then stained with JC-1 at 37 °C in the dark for 30 min. Subsequently, HK-2 cells were washed twice with PBS and the fluorescent images were observed and captured using confocal microscopy. In the normal state, JC-1 aggregates in the mitochondrial matrix to form polymers, producing red fluorescence signals; when the MMP level decreases, JC-1 disaggregates to monomers which produce green fluorescence. The red-to-green fluorescence ratio was employed to evaluate the changes in the MMP level.
2.8. Immunofluorescence
HK-2 cells were fixed with 4% formaldehyde for 15 min at 37 °C, washed with PBS, and permeabilized with 0.3% Triton X-100 for 10 min. After blocking in 5% BSA for 30 min, samples were immunolabeled with primary antibodies against TXNIP (1: 200) and p-NF-κB p65 (1:200) overnight at 4 °C. Cells were washed and further incubated with Alexa Fluor 488-tagged goat anti-rabbit antibody (1:500, Abcam, UK) in the dark for 1 h at 37 °C. Nuclei were counterstained with DAPI (Beyotime Biotechnology, China) for 10 min at room temperature. The fluorescent images were observed and captured using confocal microscopy.
2.9. Detection of ROS level
The intracellular ROS level in kidneys was detected using dihydroethidium (DHE; Beyotime Biotechnology, China). The kidney samples were washed twice with PBS and incubated with 20 μM DHE diluted in 2 mL serum-free DMEM without light at 37 °C. After 30 min incubation, the treated renal tissues were washed three times with PBS. Nuclei were counterstained with DAPI and digital images of the sections were captured using confocal microscopy. The oxidation-sensitive fluorescent probe, 2, 7-dichlorodihydrofluorescein diacetate (DCFH-DA; Beyotime Biotechnology, China), was utilized to measure the ROS level in HK-2 cells. In brief, cells were incubated with 10 μM DCFH-DA at 37 °C for 30 min and then washed with serum-free medium three times. The fluorescence intensity of HK-2 cells was observed under the confocal microscopy.
2.10. Western blotting analysis
Proteins from cells and renal tissues were extracted with lysis buffer (KeyGEN, China) supplemented with phenylmethylsulfonyl fluoride (PMSF), phosphatase inhibitors, and protease inhibitors. Equal amounts of the protein samples were loaded on 10% sodium dodecyl sulfate-polyacrylamide gel electrophoresis (SDS-PAGE) and then transferred onto a nitrocellulose filter membrane (GE Healthcare Life Sciences, UK). After blocking in 5% bovine serum for 2 h, the membranes were incubated at 4 °C overnight with primary antibodies against neutrophil gelatinase-associated lipocalin (NGAL; 1:1000, Cloud-Clone, China), kidney injury molecule-1 (KIM-1; 1:1000, Cloud-Clone, China), inducible nitric oxide synthase (iNOS; 1:1000, Cell Signaling Technology, USA), tumor necrosis factor-α (TNF-α; 1:1000, Servicebio, China), interleukin-1β (IL-1β; 1:1000, Cloud-Clone, China), Bcl2-antagonist of cell death (Bad; 1:1000, Cloud-Clone, China), Bcl2 associated X protein (Bax; 1:1000, Cell Signaling Technology, USA), Caspase3 (1:1000, Proteintech, USA), cytochrome C (CytC; 1:1000, Cell Signaling Technology, USA), TXNIP (1:1000), NLRP3 (1:1000, Cell Signaling Technology, USA), NF-κB p65 (1:1000, Cell Signaling Technology, USA), p-NF-κB p65 (1:1000), β-Actin (1:1000, Cell Signaling Technology, USA), β-Tubulin (1:1000, Abways, China) and GAPDH (1:1000, Servicebio, China). Then the membranes were washed three times with Tween 20/Tris-buffered saline (TBST) and incubated with the secondary antibody (LI-COR Biosciences, USA) for 2 h at room temperature. After washing three times with TBST, the fluorescence signal of the secondary antibody could be detected by the Odyssey Fluorescence Imaging System (LI-COR Biosciences, USA).
2.11. Reverse transcription-quantitative polymerase chain reaction (RT-qPCR)
Total RNA from the renal tissues was isolated using TRIzol reagent (Invitrogen, USA) according to the manufacturer’s instructions. RNA concentration and purity were determined by 260/280 nm absorbance. Reverse transcription kits (Vazyme, China) were used to reverse transcribe RNA into cDNA. The expressions of TNF-α and IL-1β were determined in the SYBR Green PCR kits (Yeasen Biotechnology, China). The primer sequences are as follows: TNF-α: forward: 5′- CTCGAACCCCGAGTGACAAG −3′ and reverse: 5′-TATCTCTCAGCTCCACGCCA −3′; IL-1β: forward: 5′- GCTTCAGGCAGGCAGTATCA −3′ and reverse: 5′- AGTCACAGAGGATGGGCTCT −3′.
2.12. Statistical analysis
Experimental data were expressed as mean ± standard error of the mean (SEM) and were analyzed with SPSS 22.0 statistical software (SPSS Inc., USA). Student’s t-test compared results between two groups. One-way analysis of ANOVA was employed for the comparisons among multiple groups followed by Tukey’s post-test. A p value of < 0.05 was considered statistically significant.
3. Results
3.1. MgH2 improves renal injury induced by APAP
The levels of SCr and BUN in serum of mice were significantly elevated in the APAP group as compared to the control group. In contrast, the contents of these biomarkers were significantly reduced with MgH2 administration in low and high dose (). The results of HE staining in the kidney of the control group showed that the kidney structure was intact and the morphology of each cell was normal. In the APAP group, obvious renal changes were examined such as tubular dilation, degeneration, necrosis, and desquamation of epithelial cells. Administration of MgH2 improved APAP-induced histological damages in the kidney (). Masson staining revealed that the deposition of collagen fibers in the tubulointerstitium and glomeruli was obviously increased in the APAP group as compared to the control group, which can be decreased by high dose MgH2 administration (). The results of PAS staining showed that protein excretion and tubular epithelial breaks caused by APAP were improved by low and high-dose MgH2 administration (). As new biomarkers of renal injury, the protein expressions of KIM-1 and NGAL in kidneys of mice were analyzed. The expressions of KIM-1 and NGAL were enhanced in the APAP group compared to the control group, which were downregulated by low and high-dose MgH2 administration ().
Figure 1. MgH2 ameliorates APAP-induced renal dysfunction, histological injury and oxidative stress in mice.
(A, B) The levels of SCr and BUN in serum of mice were determined by corresponding kits.
(C–E) The renal histological changes in mice were observed by HE staining (C), Masson staining (D) and PAS staining (E).
(F, G) The protein expressions of NGAL, KIM-1 and iNOS in kidneys of mice were detected by western blotting.
(H) The intracellular ROS level in renal tissues of mice was detected using DHE.
The results were expressed as mean ± SEM. Statistical comparisons were performed using a Newman–Keuls test (*p < 0.05 vs. Control group, #p < 0.05 vs. APAP group).
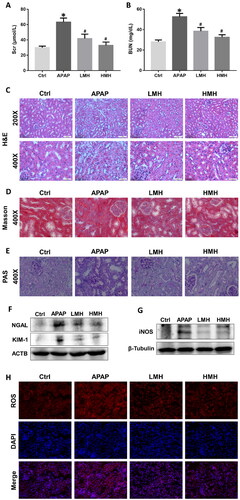
3.2. MgH2 attenuates APAP-AKI by inhibiting oxidative stress, inflammation, and apoptosis
As one of the oxidative stress markers, the protein expressions of iNOS in kidneys of mice were increased in the APAP group than the control group, which were reduced by MgH2 administration (). The intracellular ROS level in kidneys of mice was exhibited by red fluorescence in renal slices. The fluorescence showed that increased renal ROS production induced by APAP in mice was reduced with MgH2 administration (). When it comes to the inflammation indexes, the mRNA expressions of TNF-α and IL-1β in kidneys of mice were upregulated in the APAP group than the control group, which were reversed by MgH2 administration (). Similarly, western blotting showed that increased protein expressions of TNF-α and IL-1β in kidneys caused by APAP were reduced with MgH2 administration in low and high dose (). The protein expressions of Bad, Bax, Caspase3 and CytC in kidneys of mice were detected by western blotting so as to reflect apoptosis. It was demonstrated that Bad, Bax, Caspase3, and CytC protein expressions were enhanced by APAP, which were diminished with low- and high-dose MgH2 administration (). In addition, we performed TUNEL staining of renal tissues to detect apoptosis and found that TUNEL-positive tubular cells were appeared in the APAP group, but the number of these cells was decreased by MgH2 administration ().
Figure 2. MgH2 improves APAP-induced inflammation and apoptosis in kidneys of mice.
(A, B) The mRNA expressions of TNF-α and IL-1β in kidneys of mice were detected by RT-qPCR.
(C, D) The protein expressions of TNF-α, IL-1β, Bad, Bax, Caspase3 and CytC in kidneys of mice were detected by western blotting.
(E) Tubular cell apoptosis in kidneys of mice was observed by TUNEL staining.
The results were expressed as mean ± SEM. Statistical comparisons were performed using a Newman–Keuls test (*p < 0.05 vs. Control group, #p < 0.05 vs. APAP group).
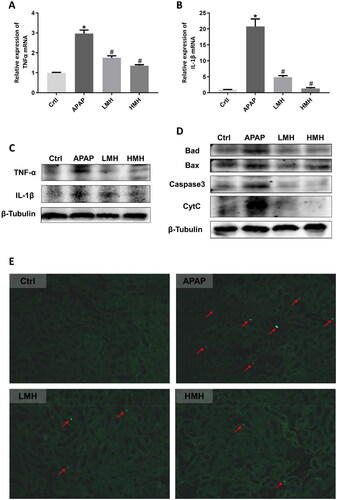
3.3. MgH2 protects HK-2 cells against APAP-induced cytotoxicity
APAP-induced cytotoxic model in HK-2 cells was created to see the therapeutic effects of MgH2. First, we administered HK-2 cells with different concentrations of MgH2 (10, 20, 50, 100, 200, 400, 600, 800, and 1000 μM) and found that the cell viability was basically unchanged under the maximum concentration of 1 mM MgH2 (). Then, HK-2 cells were administered with different concentrations of APAP (5, 10, 20, 40, 60, 80, 100 mM) and the cell viability was lowered with the increasing concentration of APAP. Our data indicated that the concentration of 5 mM APAP is cytotoxic to HK-2 cells and determined the protective effect of MgH2 (0.2 and 0.4 mM) on APAP-induced decreased cell viability (). Moreover, the increased protein expression of KIM-1 in HK-2 cells induced by APAP was reduced by MgH2 administration ().
Figure 3. MgH2 alleviates APAP-induced cytotoxicity, oxidative stress, mitochondrial dysfunction and inflammation in HK-2 cells.
(A-C) HK-2 Cell viability was detected by CCK-8 assay. (A) HK-2 cells was administered with different concentrations of MgH2 (10, 20, 50, 100, 200, 400, 600, 800 and 1000 μM); (B) HK-2 cells was administered with different concentrations of APAP (5, 10, 20, 40, 60, 80, 100 mM) with or without MgH2 (0.4 mM); (C) HK-2 cells was administered with APAP (10 mM) with or without MgH2 (0.2, 0.4 mM).
(D) The ROS level in HK-2 cells was determined by DCFH-DA.
(E) The mitochondrial function of HK-2 cells was assessed by JC-1 staining.
(F, G) The protein expressions of iNOS, KIM-1 and IL-1β in HK-2 cells were detected by western blotting.
The results were expressed as mean ± SEM. Statistical comparisons were performed using t-test (*p < 0.05 vs. APAP group) or Newman–Keuls test (*p < 0.05 vs. Control group, #p < 0.05 vs. APAP group).
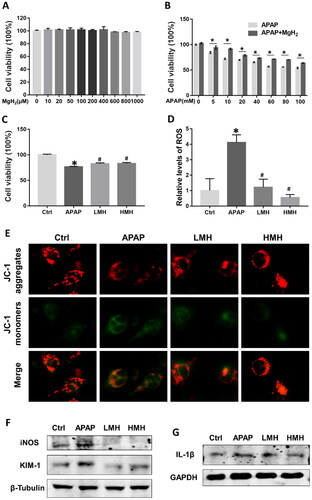
3.4. MgH2 protects HK-2 cells against APAP-induced oxidative stress, inflammation, and apoptosis
The ROS level in HK-2 cells was significantly elevated in the APAP group as compared to the control group. After MgH2 administration, the ROS level was remarkably downregulated (). Besides, the protein expressions of iNOS and TNF-α in HK-2 cells were increased in the APAP group than the control group, which were reduced by MgH2 administration (). The MMP level, assessed by JC-1 staining, was used to evaluate apoptosis of HK-2 cells. Notably, exposing HK-2 cells to APAP caused decreased MMP level, which was elevated to near-normal levels with MgH2 administration in low and high dose ().
3.5. MgH2 mitigates APAP-induced nephrotoxicity via suppressing TXNIP/NLRP3/NF-κB pathway in vitro and in vivo
The study evaluated whether TXNIP/NLRP3/NF-κB signaling pathway participated in the effects of MgH2 on APAP-AKI. In vitro, western blotting was utilized to detect the protein expressions of molecules in TXNIP/NLRP3/NF-κB signaling. As compared to the control group, the expressions of TXNIP, NLRP3, p-NF-κB p65, and NF-κB p65 in HK-2 cells were enhanced in the APAP group, which were downregulated by MgH2 administration (). Immunofluorescence also suggested that increased expressions of TXNIP and p-NF-κB p65 induced by APAP were suppressed with MgH2 administration in low and high dose (). In mice model of APAP-AKI, the western blotting results demonstrated that the expressions of TXNIP, NLRP3, p-NF-κB p65 and NF-κB p65 were upregulated in the APAP group than the control group. But the expressions of these molecules were reversed through low- and high-dose MgH2 administration (). Likewise, immunohistochemistry showed that increased expressions of TXNIP and p-NF-κB p65 caused by APAP were diminished by MgH2 administration ().
Figure 4. MgH2 suppresses APAP-induced TXNIP/NLRP3/NF-κB signaling pathway activation in HK-2 cells.
(A) The protein expressions of TXNIP, NLRP3, NF-κB p65 and p-NF-κB p65 in HK-2 cells were detected by western blotting.
(B, C) The protein expressions of TXNIP and p-NF-κB p65 in HK-2 cells were detected with immunofluorescence assay.
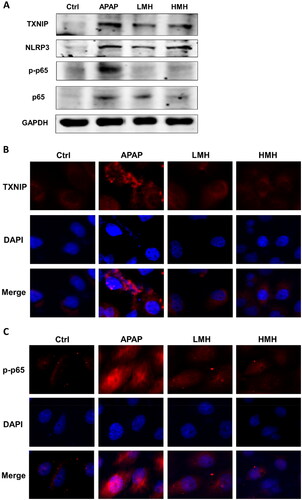
Figure 5. MgH2 suppresses APAP-induced TXNIP/NLRP3/NF-κB signaling pathway activation in kidneys of mice.
(A) The protein expressions of TXNIP, NLRP3, NF-κB p65 and p-NF-κB p65 in kidneys of mice were detected by western blotting.
(B, C) The protein expressions of TXNIP and p-NF-κB p65 in kidneys of mice were detected with immunohistochemistry staining.
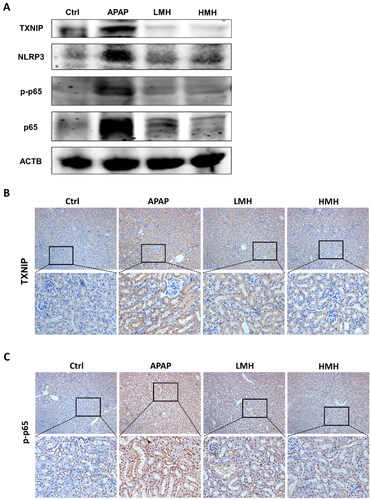
4. Discussion
APAP is a widely used antipyretic and analgesic over-the-counter drug that has a wide safety margin at therapeutic doses. However, it is easy to be overused due to the individual difference and its overdose can cause life-threatening renal injury [Citation1]. The clinical treatment for APAP-AKI is extremely limited since the most effective first-line antidote, N-Acetylcysteine, cannot completely protect against nephrotoxicity [Citation3]. Thus, there is an urgent need for alternative, safe and more widely applicable medicines to prevent or treat APAP-AKI. In this study, we focused on the protective effect of MgH2 on APAP-AKI and its potential molecular mechanisms.
APAP-induced renal insufficiency is consistent with acute tubular necrosis since APAP can directly affect proximal tubules. This injury suggests that APAP can be used as a drug-induced AKI model [Citation4]. As compared to SCr and BUN, KIM-1 and NGAL are considered as more sensitive and reliable biomarkers in AKI [Citation21,Citation22]. Our results demonstrated that the levels of SCr and BUN in serum and the expressions of KIM-1 and NGAL were upregulated in APAP-AKI, which were significantly reversed with the pretreatment of MgH2. In addition, the renal histopathology reflected that MgH2 mitigated tubular damages, indicating that MgH2 effectively attenuated APAP-AKI.
Generally, APAP-induced nephrotoxicity is frequently associated with oxidative stress. Elevated NAPQI level derived from APAP overdose causes depletion of GSH, leading to intracellular ROS generation in the kidney [Citation1] (). In our study, the results showed that the intracellular ROS level was significantly elevated in vivo and vitro model of APAP-AKI, which was also supported by previous studies [Citation2,Citation12]. It has been reported that H2 can not only suppress oxygen radicals selectively, but also enhance antioxidant enzyme activity and gene expression to alleviate oxidative stress [Citation23,Citation24]. MgH2 can produce H2 following hydrolysis reaction in the stomach: MgH2 + 2H2O → Mg(OH)2 +2H2. Our results suggested that pretreatment of MgH2 ameliorated APAP-induced renal oxidative stress.
Figure 6. MgH2 protects against APAP-AKI by inhibiting TXNIP/NLRP3/NF-κB pathway. APAP overdose leads to elevated NAPQI level and GSH depletion, resulting in enhanced renal ROS production. ROS can lead to mitochondrial dysfunction and activate TXNIP/NLRP3/NF-κB signaling pathway, resulting in apoptosis and inflammation. Besides, TXNIP can further aggravate accumulation of ROS and oxidative stress. MgH2 may alleviate APAP-AKI through improving oxidative stress, inflammation and fibrosis via suppressing TXNIP/NLRP3/NF-κB pathway.
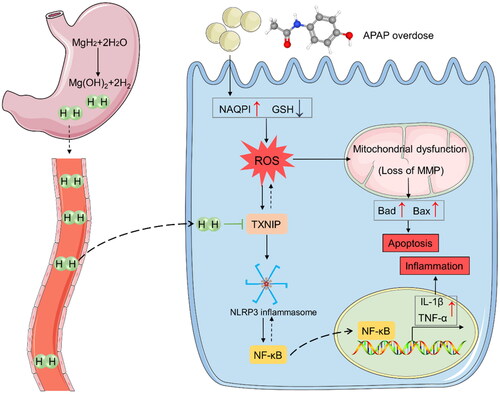
In the presence of excess APAP, depletion of GSH in the kidney causes NAPQI to bind to mitochondrial proteins and leads to mitochondrial dysfunction, thereby disrupting mitochondrial membrane permeability [Citation25]. On one hand, mitochondrial dysfunction can further accelerate the development of oxidative stress in the kidney. On the other hand, mitochondrial dysfunction can increase expressions of mitochondria-associated apoptotic proteins such as Bax and Caspase3 [Citation26]. Previous studies have proved that mitochondrial dysfunction and increased apoptotic gene expression exist in APAP-AKI [Citation5,Citation12], which are in consistence with our study. Specifically, the results exhibited that the MMP level in tubular cells was reduced while apoptotic cells and expressions of apoptotic proteins in renal tissues were increased by APAP administration. H2 was reported to keep normal mitochondrial function to relieve apoptosis [Citation27]. Besides, H2 treatment can provide protection in renal injury with different etiological factors by inhibiting apoptosis [Citation19,Citation28]. In our study, the suppression of apoptotic gene expression and the decrease in apoptotic cells in the kidney as a result of MgH2 supplementation are some other interesting findings.
There is growing evidence to show that inflammation is also accountable for the APAP-induced pathogenesis in the kidney. Moreover, ROS overproduction induced by APAP overdose can further provoke or aggravate inflammation, prompting the formation of APAP-AKI [Citation5]. In this study, we observed that the expressions of inflammatory cytokines, including TNF-α and IL-1β, were increased by excessive APAP treatment. Our findings are consistent with earlier reports, indicating that inhibition of inflammation may provide a possible therapy for APAP-AKI [Citation2,Citation12]. Anti-inflammatory property is regarded as one of the characteristics of H2. It has been reported that H2 therapy can reduce the levels of inflammatory cytokines and elevate anti-inflammatory cytokines in different kidney diseases [Citation17,Citation18,Citation29]. In the present study, we found that MgH2 treatment alleviated APAP-induced renal inflammation.
TXNIP is an endogenous inhibitor and regulator of TRX, which is a major cellular antioxidant and antiapoptotic protein. Overexpression of TXNIP inhibits the activity of TRX and thus can modulate the cellular redox state and stimulate oxidative stress [Citation30]. Additionally, alterations in TXNIP expression regulate its ability to affect some other biological processes. Firstly, TXNIP plays a crucial role in the development of inflammatory processes via regulation of NLRP3 inflammasome activation and IL1β maturation. Secondly, TXNIP can promote cell apoptosis via redox-independent activation of caspases. Thirdly, TXNIP plays an important role as a metabolic regulator [Citation31]. The activation of NLRP3 inflammasome has been implicated in various kidney diseases. Formation of NLRP3 inflammasome can increase the expression of cleaved Caspase-1 and IL-1β that participate in the pathological process of renal injury [Citation30,Citation32]. The transcription factor NF-κB is triggered in response to oxidative stress and then translocates into the nucleus and promotes the transcription of TNF-α and IL-1β [Citation33]. Moreover, NLRP3 inflammasome can accelerate NF-κB activation and promote NF-κB-mediated inflammatory response [Citation34]. It has been demonstrated that TXNIP-mediated NLRP3 inflammasome activation was involved in APAP-induced hepatic injury [Citation9,Citation35]. Our work found that the expressions of TXNIP, NLRP3 and NF-κB were increased in renal tissues and HK-2 cells with overdose APAP administration. We speculated that ROS generated by overdose APAP can lead to mitochondrial dysfunction and activate TXNIP/NLRP3/NF-κB signaling pathway, resulting in apoptosis and inflammation. Furthermore, TXNIP can aggravate accumulation of ROS and oxidative stress (). Previous studies demonstrated that H2 treatment can alleviate myocardial ischemia-reperfusion injury and sepsis-associated encephalopathy by inhibiting NLRP3 pathway [Citation36,Citation37]. In our study, we observed that MgH2 downregulated increased expressions of TXNIP, NLRP3 and NF-κB induced by APAP, suggesting that MgH2 might improve APAP-AKI via regulating TXNIP/NLRP3/NF-κB pathway ().
However, there were some limitations in this study. Since H2 diffuses rapidly and widely, there are still no effective ways to detect the diffusion of H2 in vitro and in vivo. In addition, the direct targets of H2 remain unclear so that further experimental studies are required to detect the specific mechanism of hydric action in cells and animals. Nevertheless, our study can provide foundations for further exploration of the reno-protective role of MgH2 against APAP-AKI.
5. Conclusion
The present study implicates that MgH2 can effectively relieve oxidative stress, inflammation and apoptosis during APAP-induced renal injury. The underlying mechanism is inactivation of the TXNIP/NLRP3/NF-κB pathway. Therefore, our study provides novel evidence of the benefit for the application of MgH2 in the prevention and treatment of APAP-AKI.
Author contributions
Hongtao Lu, Jin Cheng and Xuejun Sun designed this study; Yachen Si, Lulu Liu, Yinyin Zhang, Hongxia Li, Tingting Zhao and Su Liu performed the experiments and data analyses; Yachen Si, Lulu Liu and Hongtao Lu wrote the manuscript. All authors have reviewed the results and approved the final version of the manuscript.
Ethical approval
The animal study protocol was approved by Committee on Ethic of Medical Research, Naval Medical University (registration number: NMUMREC-2021–006).
Supplemental Material
Download PDF (546.4 KB)Disclosure statement
No potential conflict of interest was reported by the author(s). The authors declare that the research was conducted in the absence of any commercial or financial relationships that could be construed as a potential conflict of interest.
Data availability statement
All data generated or analyzed during this study are included in this article. Further inquiries can be directed to the corresponding author.
Additional information
Funding
References
- Mazer M, Perrone J. Acetaminophen-induced nephrotoxicity: pathophysiology, clinical manifestations, and management. J Med Toxicol. 2008;4(1):1–12. doi:10.1007/BF03160941.
- Ghosh J, Das J, Manna P, et al. Acetaminophen induced renal injury via oxidative stress and TNF-alpha production: therapeutic potential of arjunolic acid. Toxicology. 2010;268(1-2):8–18. doi:10.1016/j.tox.2009.11.011.
- Akakpo JY, Ramachandran A, Orhan H, et al. 4-methylpyrazole protects against acetaminophen-induced acute kidney injury. Toxicol Appl Pharmacol. 2020;409:115317. doi:10.1016/j.taap.2020.115317.
- Ruan H, Luo J, Wang L, et al. Sika deer antler protein against acetaminophen-induced nephrotoxicity by activating Nrf2 and inhibition FoxO1 via PI3K/akt signaling. Int J Biol Macromol. 2019;141:961–987. doi:10.1016/j.ijbiomac.2019.08.164.
- Alshahrani S, Ashafaq M, Hussain S, et al. Renoprotective effects of cinnamon oil against APAP-Induced nephrotoxicity by ameliorating oxidative stress, apoptosis and inflammation in rats. Saudi Pharm J. 2021;29(2):194–200. doi:10.1016/j.jsps.2021.01.002.
- Dua TK, Palai S, Roy A, et al. Protective effect of probiotics against acetaminophen induced nephrotoxicity. Mol Biol Rep. 2022;49(8):8139–8143. doi:10.1007/s11033-022-07534-7.
- Alqahtani QH, Fadda LM, Alhusaini AM, et al. Involvement of Nrf2, JAK and COX pathways in acetaminophen-induced nephropathy: role of some antioxidants. Saudi Pharm J. 2023;31(10):101752. doi:10.1016/j.jsps.2023.101752.
- Zhou R, Tardivel A, Thorens B, et al. Thioredoxin-interacting protein links oxidative stress to inflammasome activation. Nat Immunol. 2010;11(2):136–140. doi:10.1038/ni.1831.
- Lv H, Zhu C, Wei W, et al. Enhanced Keap1-Nrf2/trx-1 axis by daphnetin protects against oxidative stress-driven hepatotoxicity via inhibiting ASK1/JNK and txnip/NLRP3 inflammasome activation. Phytomedicine. 2020;71:153241. doi:10.1016/j.phymed.2020.153241.
- Lv H, Yang H, Wang Z, et al. Nrf2 signaling and autophagy are complementary in protecting lipopolysaccharide/d-galactosamine-induced acute liver injury by licochalcone A. Cell Death Dis. 2019;10(4):313. doi:10.1038/s41419-019-1543-z.
- Ruan H, Wang L, Wang J, et al. Sika deer antler protein against acetaminophen-induced oxidative stress and apoptosis in HK-2 cells via activating Nrf2/keap1/HO-1 pathway. J Food Biochem. 2019;43(12):e13067. doi:10.1111/jfbc.13067.
- Shen Y, Jin X, Chen W, et al. Interleukin-22 ameliorated acetaminophen-induced kidney injury by inhibiting mitochondrial dysfunction and inflammatory responses. Appl Microbiol Biotechnol. 2020;104(13):5889–5898. doi:10.1007/s00253-020-10638-4.
- Huang CS, Kawamura T, Toyoda Y, et al. Recent advances in hydrogen research as a therapeutic medical gas. Free Radic Res. 2010;44(9):971–982. doi:10.3109/10715762.2010.500328.
- Kitamura A, Kobayashi S, Matsushita T, et al. Experimental verification of protective effect of hydrogen-rich water against cisplatin-induced nephrotoxicity in rats using dynamic contrast-enhanced CT. Br J Radiol. 2010;83(990):509–514. doi:10.1259/bjr/25604811.
- Li FY, Zhu SX, Wang ZP, et al. Consumption of hydrogen-rich water protects against ferric nitrilotriacetate-induced nephrotoxicity and early tumor promotional events in rats. Food Chem Toxicol. 2013;61:248–254. doi:10.1016/j.fct.2013.10.004.
- Lu Y, Li CF, Ping NN, et al. Hydrogen-rich water alleviates cyclosporine A-induced nephrotoxicity via the Keap1/Nrf2 signaling pathway. J Biochem Mol Toxicol. 2020;34(5):e22467. doi:10.1002/jbt.22467.
- Yao W, Guo A, Han X, et al. Aerosol inhalation of a hydrogen-rich solution restored septic renal function. Aging (Albany NY). 2019;11(24):12097–12113. doi:10.18632/aging.102542.
- Si Y, Liu L, Cheng J, et al. Oral Hydrogen-Rich water alleviates Oxalate-Induced kidney injury by suppressing oxidative stress, inflammation, and fibrosis. Front Med (Lausanne). 2021;8:713536. doi:10.3389/fmed.2021.713536.
- Xue JL, Liu BY, Zhao M, et al. Inhalation of 4% and 67% hydrogen ameliorates oxidative stress, inflammation, apoptosis, and necroptosis in a rat model of glycerol-induced acute kidney injury. Med Gas Res. 2023;13(2):78–88. doi:10.4103/2045-9912.345169.
- Zhang J, Li Z, Wu Y, et al. Recent advances on the thermal destabilization of Mg-based hydrogen storage materials. RSC Adv. 2018;9(1):408–428. doi:10.1039/c8ra05596c.
- Vaidya VS, Ozer JS, Dieterle F, et al. Kidney injury molecule-1 outperforms traditional biomarkers of kidney injury in preclinical biomarker qualification studies. Nat Biotechnol. 2010;28(5):478–485. doi:10.1038/nbt.1623.
- Haase M, Bellomo R, Devarajan P, et al. Accuracy of neutrophil gelatinase-associated lipocalin (NGAL) in diagnosis and prognosis in acute kidney injury: a systematic review and meta-analysis. Am J Kidney Dis. 2009;54(6):1012–1024. doi:10.1053/j.ajkd.2009.07.020.
- Tao G, Song G, Qin S. Molecular hydrogen: current knowledge on mechanism in alleviating free radical damage and diseases. Acta Biochim Biophys Sin (Shanghai). 2019;51(12):1189–1197. doi:10.1093/abbs/gmz121.
- Qiu P, Liu Y, Zhang J. Recent advances in studies of molecular hydrogen against sepsis. Int J Biol Sci. 2019;15(6):1261–1275. doi:10.7150/ijbs.30741.
- Hua H, Ge X, Wu M, et al. Rotenone protects against Acetaminophen-Induced kidney injury by attenuating oxidative stress and inflammation. Kidney Blood Press Res. 2018;43(4):1297–1309. doi:10.1159/000492589.
- Doke T, Susztak K. The multifaceted role of kidney tubule mitochondrial dysfunction in kidney disease development. Trends Cell Biol. 2022;32(10):841–853. doi:10.1016/j.tcb.2022.03.012.
- Yonamine R, Satoh Y, Kodama M, et al. Coadministration of hydrogen gas as part of the carrier gas mixture suppresses neuronal apoptosis and subsequent behavioral deficits caused by neonatal exposure to sevoflurane in mice. Anesthesiology. 2013;118(1):105–113. doi:10.1097/ALN.0b013e318275146d.
- Guan P, Sun ZM, Luo LF, et al. Hydrogen protects against chronic intermittent hypoxia induced renal dysfunction by promoting autophagy and alleviating apoptosis. Life Sci. 2019;225:46–54. doi:10.1016/j.lfs.2019.04.005.
- Cardinal JS, Zhan J, Wang Y, et al. Oral hydrogen water prevents chronic allograft nephropathy in rats. Kidney Int. 2010;77(2):101–109. doi:10.1038/ki.2009.421.
- Xiao YD, Huang YY, Wang HX, et al. Thioredoxin-Interacting protein mediates NLRP3 inflammasome activation involved in the susceptibility to ischemic acute kidney injury in diabetes. Oxid Med Cell Longev. 2016;2016:2386068–2386017. doi:10.1155/2016/2386068.
- Spindel ON, World C, Berk BC. Thioredoxin interacting protein: redox dependent and independent regulatory mechanisms. Antioxid Redox Signal. 2012;16(6):587–596. doi:10.1089/ars.2011.4137.
- Bivol LM, Iversen BM, Hultström M, et al. Unilateral renal ischaemia in rats induces a rapid secretion of inflammatory markers to renal lymph and increased capillary permeability. J Physiol. 2016;594(6):1709–1726. doi:10.1113/JP271578.
- Perkins ND. Integrating cell-signalling pathways with NF-kappaB and IKK function. Nat Rev Mol Cell Biol. 2007;8(1):49–62. doi:10.1038/nrm2083.
- Samra YA, Said HS, Elsherbiny NM, et al. Cepharanthine and piperine ameliorate diabetic nephropathy in rats: role of NF-kappaB and NLRP3 inflammasome. Life Sci. 2016;157:187–199. doi:10.1016/j.lfs.2016.06.002.
- Woolbright BL, Jaeschke H. Role of the inflammasome in acetaminophen-induced liver injury and acute liver failure. J Hepatol. 2017;66(4):836–848. doi:10.1016/j.jhep.2016.11.017.
- Nie C, Ding X, A R, et al. Hydrogen gas inhalation alleviates myocardial ischemia-reperfusion injury by the inhibition of oxidative stress and NLRP3-mediated pyroptosis in rats. Life Sci. 2021;272:119248. doi:10.1016/j.lfs.2021.119248.
- Xie K, Zhang Y, Wang Y, et al. Hydrogen attenuates sepsis-associated encephalopathy by NRF2 mediated NLRP3 pathway inactivation. Inflamm Res. 2020;69(7):697–710. doi:10.1007/s00011-020-01347-9.