Abstract
The association between blood flow rate (BFR) and clinical outcomes in patients undergoing maintenance hemodialysis (MHD) is inconclusive. This retrospective study included 175 patients undergoing MHD treatment between July 2015 and March 2022, divided into two groups based on time-averaged effective blood flow rate (eBFR) median value. We investigated arteriovenous fistula (AVF) outcomes and the association of eBFR with all-cause mortality and new major adverse cardiovascular events (MACE). Mean ± SD and median time-averaged eBFR values were 276 ± 24 and 275 mL/min, respectively. After adjusting for relevant factors including age, sex, vintage, diabetes, CVD, receiving hemodiafiltration (HDF) treatment and spKt/V, Cox models indicated a low time-averaged eBFR (≤ 275 ml/min) was associated with increased risks of all-cause mortality (hazard ratio [HR] 14.18; 95% confidence interval [CI], 3.14–64.1) and new MACE (HR 3.76; 95% CI, 1.91–7.40) in MHD patients. Continuous Cox models demonstrated each 20 ml/min increase in eBFR linked to a 63% decrease in the risk of all-cause mortality (HR: 0.37, 95% CI: 0.23–0.59) and a 38% decrease in the occurrence of new MACE (HR: 0.62, 95% CI: 0.46–0.84). There was no significant difference in AVF outcomes between the two groups. Our study noted higher eBFR (>275 mL/min) is associated with lower risks of both all-cause mortality and new MACE compared with low eBFR (≤275 mL/min). Increased eBFR is not associated with a higher risk of AVF failure.
1. Introduction
Blood flow rate (BFR) is a key determinant of dialysis adequacy under the condition of three times per week for 4 h for each hemodialysis (HD) session [Citation1]. An increased BFR can significantly improve single-pool Kt/V (spKt/V) and may improve survival in maintenance hemodialysis (MHD) patients [Citation2, Citation3]. However, data from Japan have shown that a low BFR may contribute to longer survival compared with other countries because patients with a low BFR may have a high rate of arteriovenous fistula (AVF) survival [Citation4, Citation5]. Data from the Dialysis Outcomes and Practice Patterns Study (DOPPS) revealed that BFR varied greatly among the included countries [Citation6]. North America has the highest BFR (median = 400 mL/min), followed by Europe (median = 300 mL/min) and Japan (median = 200 mL/min) [Citation6]. Given the different dialysis practices in other countries, prescribing an appropriate BFR for MHD patients remains difficult.
Recent studies in Asia have investigated the relationship between BFR and mortality in MHD patients. One study from Korea, including 1,129 MHD patients, revealed that a BFR < 250 mL/min was associated with higher mortality [Citation7]. Another Chinese DOPPS, including 1,412 MHD patients, found that BFR had a negative linear relationship with all-cause mortality within a range of 200–300 mL/min [Citation8]. However, these studies did not assess the impact of BFR on AVF. Whether high BFR damages vascular access in patients is still controversial, and investigators have reported that a higher BFR correlates with higher rates of final AVF failure [Citation6]. However, another study found no association between vascular access failure and BFR (up to 390 mL/min) [Citation9].
Moreover, prescribed BFR (pBFR) rather than effective BFR (eBFR) has been employed in most studies [Citation6–8]. All dialysis delivery systems provide information about the pBFR through a selector switch setting or a digital output. However, there are certain situations where the effective blood flow rates (eBFR) can be significantly lower than the prescribed value. Primarily, the eBFR is lower than the pBFR due to low pressure in the prepump arterial blood line [Citation10]. Several variables, including vascular access recirculation and cardiopulmonary recirculation, needle size used for AVF, and blood viscosity, may influence BFR [Citation11]. The pBFR differs from the eBFR; in other words, it is higher than the eBFR. Remarkably, the degree of difference between the pBFR and the eBFR may vary among MHD individuals. Thus, eBFR is believed to be a more accurate marker for assessing blood flow velocity than pBFR when exploring the association between BFR and clinical outcomes.
Herein, we tested the association between time-averaged eBFR and clinical outcomes, specifically all-cause mortality and new major adverse cardiovascular events (MACE). Furthermore, we examined the effect of eBFR on AVF in MHD patients.
2. Patients and methods
2.1. Study design and data sources
This was a single-center, retrospective, observational study conducted at Peking University International Hospital. Patients undergoing MHD at our dialysis center between July 2015 and March 2022 were selected. The flow diagram of patient enrollment in the study is shown in . A past medical history of cardiovascular disease (CVD) included coronary heart disease, heart failure, cerebral infarction, and cerebral hemorrhage. If any of these conditions had occurred previously, it was considered as the presence of CVD. All patients used different dialyzers, including the FX80 (Fresenius Medical Care) and Revaclear 400 (Gambro Dialysatoren GmbH), for high-flux hemodialysis or online post-dilution hemodiafiltration (HDF) at a dialysis flow rate of 500 mL/min. The criteria for selecting the participants were as follows: ① age > 18 years; ② dialysis vintage > 6 months; ③ undergoing thrice-weekly dialysis for 4 h each time across the entire study duration; ④ using AVF for vascular access. The exclusion criteria were as follows: ① using a central venous catheter or an arteriovenous graft (AVG) as vascular access. ② HD combined with peritoneal dialysis. The study complied with the 1975 Helsinki Declaration and was approved by the Ethics Committee of Peking University International Hospital [2022-KY-0003-01]. The requirement for informed consent was waived because of the study’s retrospective nature.
2.2. Laboratory data and dialysis parameters
Blood samples, including pre- and postdialysis samples, were collected monthly as part of routine clinical testing. The measured pre-dialysis parameters included serum urea, β2-microglobulin (β2-MG), creatinine, albumin, C-reactive protein, calcium, phosphate, alkaline phosphatase, glucose, triglyceride, venous carbon dioxide, electrolytes, and hemoglobin. Postdialysis serum urea nitrogen and β2-microglobulin were obtained to calculate spKt/V and β2-MG reduction ratio (β2-MG RR). Additionally, predialysis parathyroid hormone levels were checked every 1–3 months. The lean tissue index (LTI) was measured using bioelectrical impedance assessment in all patients every three or six months. The left ventricular ejection fraction was assessed by echocardiography every year. Time-averaged values were defined as the mean of measurements from baseline to the end of follow-up.
Dialysis parameters, such as pBFR, eBFR, dialysis pattern, dialyzer, and interdialytic weight gain (IDWG), were recorded throughout the follow-up period. The average eBFR value was calculated using the dialysis electronic recording system and represents the mean eBFR from all dialysis records during the follow-up period. The median time-averaged eBFR value in this study was 275 mL/min. Patients were further divided into two groups: those with eBFR ≤ 275 mL/min and those with eBFR >275 mL/min, based on the median time-averaged eBFR value.
2.3. Outcomes
The clinical outcomes of the patients included all-cause mortality and new MACE. New MACE is a composite outcome that encompasses new-onset heart failure, myocardial infarction, intracerebral hemorrhage, cerebral infarction, and all-cause mortality. New-onset heart failure is characterized by the sudden onset of difficulty in breathing due to abnormal cardiac function. This abnormal function can be confirmed through biomarkers such as echocardiography or B-type natriuretic peptide (BNP). New myocardial infarction is defined as myocardial injury accompanied by clinical evidence of myocardial ischemia, elevated serum troponin levels, pathological Q waves or new ischemic changes on electrocardiogram, or severe coronary artery stenosis requiring stent implantation or balloon dilatation during angiography. Regional wall motion abnormalities found on myocardium or echocardiography also indicate new myocardial infarction. New intracerebral hemorrhage or infarction refers to the sudden onset of neurological abnormalities caused by either intracerebral hemorrhage or infarction. These abnormalities are confirmed through a CT scan or MRI. The AVF outcomes were defined as ① intervention: the need for surgical or percutaneous endovascular intervention due to AVF thrombosis and stenosis; ② final AVF failure: AVF abandoned. All AVF outcomes were obtained from electronic medical records.
2.4. Statistical methods
Continuous variables were expressed as mean ± standard deviation, and differences between the two groups were tested using an independent sample t-test. For the continuous variables that did not follow a normal distribution, we used the nonparametric Mann–Whitney U-test for comparisons. Categorical variables were expressed as n (%), and differences between the two groups were compared using the chi-square test.
Unadjusted analyses of the association between the eBFR and individual covariates were performed using binary logistic regression models. Kaplan–Meier curves were used to analyze all-cause mortality or new MACE in the two groups, and differences in survival curves were compared using log-rank statistics. Cox proportional hazards models used a conditional stepwise forward procedure to analyze the risk association between eBFR and the new MACE. Variables that demonstrated prognostic significance (p < 0.05) in the univariate analysis, along with clinically significant variables, were included in the adjusted Cox multivariable models. The study used three different models for analysis: Model 1, which was unadjusted; Model 2, which was adjusted for age, sex, vintage, diabetes, and previous history of cardiovascular disease (CVD); and Model 3, which was adjusted for the variables in Model 2 as well as HDF and spKt/V. Additionally, propensity score-adjusted Cox proportional hazard regression models were used to account for age and previous history of CVD, as the patients were not randomly assigned to the two different eBFR groups. Logistic regression was used to compute the propensity scores.
To examine the interaction between variables and eBFR concerning all-cause mortality and new MACE, patients were divided into subgroups according to various variables. Subgroup analyses were subsequently carried out using Cox regression. The results of these subgroups were illustrated in forest plots.
All statistical analyses were performed using IBM SPSS version 25 (IBM SPSS Statistics, IBM Corporation). Statistical significance was set at p < 0.05.
3. Results
3.1. Patients characteristics
A total of 175 patients were finally included in our study. The enrolled patients had a mean age of 59.1 ± 13.9 years, a median dialysis vintage of 4.3 (3.1, 6.8) years, and a median follow-up time of 32.8 (21.7, 44.1) months. Of the patients, 34.9% were female and 37.7% had diabetes. All patients receive dialysis three times a week, with the majority undergoing high-flux hemodialysis. Specifically, 65.1% of patients receive three dialysis sessions per week during the follow-up period, which includes multiple hemodiafiltration sessions. A total of 175 patients completed 8,217 pre- and post-dialysis laboratory tests. Of them, complete data were available for 156 patients (89.1%); 19 patients (10.9%) had partially missing data because they were transferred to other dialysis centers during the follow-up period. The number of laboratory tests missing was 211, accounting for 2.6% of the total 8,217 laboratory tests. Among the 19 patients, 57.9% were male and had a median age of 66 (59, 72) years. The follow-up time at our center was 21.5 (10.1, 35.1) months. Out of these patients, 12 belonged to the low eBFR group (eBFR ≤ 275 mL/min), with 41.7% being male and a median age of 65 (56, 79) years. The follow-up time for this group at our center was 19.6 months (11.2, 34.2). On the other hand, 7 patients were in the high eBFR group (eBFR > 275 mL/min), with 85.7% being male and a median age of 66 (59, 72) years. The follow-up time for this group at our center was 24.9 (4.8, 35.1) months. For these 19 patients, we performed telephone follow-ups to acquire information on survival and new MACE. One patient in the low eBFR group died, while the remaining patients did not experience any major adverse cardiovascular events (MACE). All patients had patent arteriovenous fistulas, and no adverse events were reported.
3.2. Characteristics of study population according to eBFR
The distribution of the time-averaged eBFR is shown in . Among these patients, the mean ± SD and median eBFR values were 276 ± 24 and 275 (199-348) mL/min, respectively. The baseline and time-averaged patient data are shown in and , respectively. The logistic regression analysis revealed that the patients with higher time-averaged eBFR were male [odds ratio [OR] 2.44 (95% confidence interval [CI], 1.04–5.72), p = 0.039] and had a higher body mass index (BMI) [OR 1.21 (95% CI, 1.07–1.35), p = 0.002], higher IDWG [OR 2.00 (95% CI, 1.17–3.42), p = 0.011], higher serum albumin [OR 1.22 (95% CI, 1.02–1.46), p = 0.026], and higher β2-MG RR [OR 1.10 (95% CI, 1.04–1.15), p < 0.001].
Table 1. Demographics and patient characteristics in two groups based on the time-averaged eBFR.
Table 2. The baseline and time-averaged values in two groups based on the time-averaged eBFR.
3.3. The effect of eBFR on all-cause mortality or new MACE
Among 175 patients, 25 died (14.3%) and 55 new MACEs occurred (31.4%) during the study period. Unadjusted Kaplan–Meier survival analysis showed that patients with low eBFR had higher risks of all-cause mortality and new MACE than patients with high eBFR (). Time-varying Cox proportional hazard models also showed worse outcomes in patients with low eBFR (). After adjusting for age, sex, vintage, diabetes, CVD, HDF, and spKt/V, patients with low eBFR (≤275 mL/min) had an approximately 13-fold higher risk of all-cause mortality than patients with high eBFR (>275 mL/min). When entering eBFR as a continuous variable in models, each 20 mL/min increase in eBFR was linked to a 49% decrease in the risk of all-cause mortality (HR: 0.51, 95% CI: 0.39–0.67; model 1). After adjustment for age, sex, vintage, diabetes, CVD, HDF, and spKt/V, each 20 mL/min increase in eBFR was linked to a 63% decrease in the risk of all-cause mortality (HR: 0.37, 95% CI: 0.23–0.59; model 3). Meanwhile, patients with low eBFR (≤275 mL/min) had a higher risk of new MACE (HR 3.76; 95% CI, 1.91–7.40; model 3) compared to those with high eBFR (>275 mL/min). Likewise, continuous variables models showed each 20 mL/min increase in eBFR was associated with a 38% decrease in the occurrence of new MACE (HR: 0.62, 95% CI: 0.46 − 0.84; model 1). As baseline characteristics, such as age and previous history of CVD, differed between the two groups, we used propensity score matching to adjust for confounding factors. After propensity score matching, 122 patients were matched, and the baseline characteristics were similar between the two groups (). Furthermore, propensity score-adjusted Cox proportional hazard models () revealed similar results: patients with high eBFR (> 275 mL/min) had decreased risks of both all-cause mortality and new MACE than patients with low eBFR (≤ 275 mL/min).
Figure 3. A. Unadjusted Kaplan–Meier curves for all-cause mortality in two groups based on time-averaged eBFR. B. Unadjusted Kaplan–Meier curves for new MACE in two groups based on time-averaged eBFR.
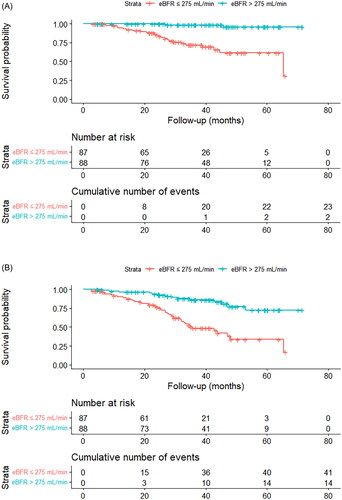
Table 3. The relationship between eBFR and clinical outcomes.
Table 4. The baseline characteristics and data in the two groups based on the time-averaged eBFR after propensity score matching.
3.4. Subgroup analyses
Subgroup analyses were conducted to examine the interaction between variables and eBFR with all-cause mortality and new MACE. There was a notable interaction between age and eBFR with the risk of all-cause mortality. A higher eBFR in patients aged ≥60 years was significantly linked to a lower risk of all-cause mortality compared to patients aged <60 years. For every 20 mL/min increase in eBFR, the risk of all-cause mortality in patients aged 60 ≥years decreased by 52% (HR: 0.48, 95% CI: 0.34–0.69) ().
Figure 4. A. Subgroup analyses for the relationship of eBFR and all-cause mortality in patients according to age, sex, BMI, diabetes, CVD, HDF ≥ 1/week, and ALB. BMI, body mass index; CVD, cardiovascular disease; HDF, hemodiafiltration; ALB, albumin; HR, hazard ratio; CI confidence interval. B. Subgroup analyses for the relationship of eBFR and new MACE in patients according to age, sex, BMI, diabetes, CVD, HDF ≥ 1/week, and ALB. BMI, body mass index; CVD, cardiovascular disease; HDF, hemodiafiltration; ALB, albumin; HR, hazard ratio; CI confidence interval.
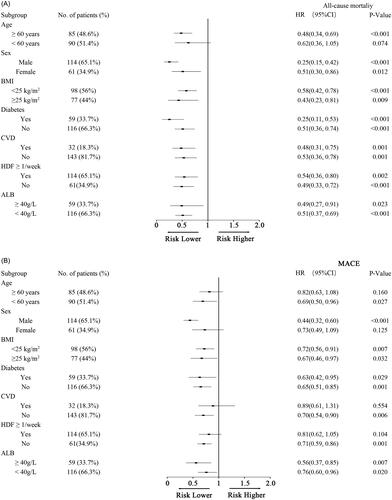
3.5. Outcomes of AVF
Meanwhile, significant interactions were observed between eBFR and age, gender, previous CVD history, and receipt of HDF treatment with new MACE occurrences (). Patients aged <60 years showed a 31% decrease in new MACE events for each 20 mL/min increase in eBFR (HR: 0.69, 95% CI: 0.50 − 0.96). However, this relationship was not statistically significant for patients aged 60 ≥years. Male patients experienced a notable reduction in new MACE events compared to females, with a 56% decrease for each 20 mL/min increase in eBFR (HR: 0.44, 95% CI: 0.32 − 0.60). Patients without a history of previous CVD had significantly fewer new MACE events than those with previous CVD, showing a 30% decrease for each 20 mL/min increase in eBFR (HR: 0.70, 95% CI: 0.54 − 0.90). Furthermore, patients not undergoing HDF treatment exhibited significantly fewer new MACE events compared to those receiving HDF, with a 29% decrease for each 20 mL/min increase in eBFR (HR: 0.71, 95% CI: 0.59 − 0.86).
Among 175 patients, 89.7% had forearm AVF, 6.3% had wrist AVF, and 4.0% had upper arm AVF. There was no significant difference in the AVF types between the low and high eBFR groups. 16-G needles were used in all patients. During the follow-up period, 9.1% of them had AVF thrombotic events, 24% had AVF stenosis, 1.1% had AVF aneurysmal dilation, and 26.3% underwent surgical intervention, including open surgery and percutaneous angioplasty, and 8.6% had final AVF failure. Among patients with low eBFR, 10.3% developed AVF thrombotic events and 27.6% developed AVF stenosis. Furthermore, 28.7% of these patients required surgical intervention, and 10.3% experienced final AVF failure. These rates were relatively higher compared to those observed in patients with high eBFR. However, no statistically significant differences were found between the two groups ().
Table 5. The outcome of AVF in two groups based on the time-averaged eBFR.
4. Discussion
The study revealed that higher eBFR was linked to a lower risk of all-cause mortality and new MACE. Among MHD patients, those with an eBFR > 275 mL/min (but < 350 mL/min) appeared to have a reduced risk of all-cause mortality and fewer new MACE events when compared to pa-tients with an eBFR ≤ 275 mL/min. To the best of our knowledge, this is the first study to use eBFR rather than pBFR to investigate the effect of BFR on clinical outcomes, specifically all-cause mortality and new MACE in MHD patients. In practice, BFR may be influenced by several factors, including access and cardiopulmonary recirculation, blood viscosity, and needle size. eBFR is commonly lower than pBFR; therefore, pBFR may overestimate BFR. A previous study reported that the blood flow rate decreased by 2% to 5% at a pre-pump pressure of −50 mmHg [Citation12]. Our study showed that eBFR was reduced by 2.8% to 6.4% compared to pBFR. Another strength of our research is that time-averaged variables, including time-averaged eBFR calculated using regular real-time measured values, may provide more accurate information about eBFR and be more relevant for investigating the association between eBFR and clinical outcomes.
The reasons for the better survival associated with a higher BFR are not clearly understood. It is well known that BFR can significantly influence spKt/V. Several previous studies have revealed that increased BFR may reduce low Kt/V prevalence and improve survival in HD patients [Citation3, Citation5, Citation13]. However, our Cox regression models showed that the association between higher eBFR and the risk of new MACE was independent of spKt/V. The Kidney Disease Outcomes Quality Initiative (KDOQI) guidelines recommend a target spKt/V of 1.4 for patients treated thrice weekly; however, no survival benefit was observed at higher levels of spKt/V [Citation14, Citation15]. In our study, both values of spKt/V in the high eBFR group (1.55 ± 0.26) and the low eBFR group (1.55 ± 0.21) were higher than 1.4. Therefore, factors other than spKt/V may contribute to the association between better survival and higher BFR. Logistic regression analysis showed that patients with a higher eBFR had a higher β2-MG RR. β2-MG is an excellent surrogate for medium-molecular uremic substances, and high serum β2-MG levels have been identified as strong indicators of poor outcomes in HD patients [Citation16]. A multicenter randomized controlled trial showed that high-efficiency post-dilution online HDF reduced all-cause mortality compared with conventional HD, partly due to the clearance of middle molecular uremic substances [Citation17]. In our study, all patients were treated with high-flux dialysis, and increased eBFR significantly increased the clearance of β2-MG. We speculate that the higher eBFR associated with better clinical outcomes may be partly due to the greater clearance of middle molecular weight uremic substances in our study.
In subgroup analyses, our results revealed significant interactions between age and eBFR regarding all-cause mortality. We found that a high eBFR was associated with a reduced risk of all-cause mortality among older patients, while this association was not observed in younger patients. Conversely, in patients aged <60 years, a high eBFR was significantly associated with a decreased risk of new MACE. The discrepancy in the prevalence of age between the high eBFR group and the low eBFR group could be a contributing factor. Furthermore, significant interactions were observed between eBFR and sex, previous history of CVD, and receiving HDF treatment with the occurrence of new MACE. For males, increasing eBFR significantly reduced the risk of new MACE compared to females. Similarly, increasing eBFR significantly reduced the risk of new MACE in patients without a previous history of CVD. However, this benefit was not observed in patients with a prior history of CVD. In patients who did not receive HDF treatment, increasing eBFR also significantly reduced the risk of new MACE. The reasons for these interactions are currently unclear. It is important to interpret the survival benefits of high eBFR in subgroup patients cautiously.
The effect of BFR on cardiovascular outcomes in patients undergoing HD remains unclear. A recent study showed that an appropriate increase in blood pump flow rate (BFR >220 mL/min) had little effect on cardiac parameters in HD patients with AVF [Citation18]. However, this study excluded patients with blood pump flow rates >300 mL/min and did not analyze cardiovascular outcomes. Our study showed that the risk of new MACE was significantly decreased in patients with high eBFR (eBFR >275 mL/min, but <350 mL/min), indicating high eBFR may potentially provide a cardiovascular benefit. The mechanism underlying this reduction in cardiovascular events is unknown. A Cochrane systematic review and meta-analysis revealed that high-flux HD reduced cardiovascular mortality compared with low-flux HD and postulated that a larger membrane pore size resulted in greater clearance of circulating molecules potentially pathogenic for cardiovascular disease [Citation19]. Our results showed that in the high eBFR group, the β2-MG RR was higher than that in the low eBFR group, which may contribute to a protective effect against cardiovascular diseases.
Another focus was the impact of increased BFR on AVF outcomes. Data from DOPPS 2 (2002-2004) and 3 (2005-2008) showed that the final AVF failure rates were higher in facilities with higher BFR [Citation6]. Previous studies revealed that a higher BFR produced disturbances in the laminar flow in the vascular access and increased the risk of platelet aggregation and coagulation, which might be associated with vascular access failure [Citation20, Citation21]. However, the factors influencing AVF survival include age, diabetes, prior catheter use, AVF type, anastomosis angle, and hemoglobin levels [Citation2–24]. A recent study that included 1,039 HD patients indicated that extracorporeal blood flow in the 310–390 mL/min range did not negatively affect vascular access [Citation9]. Similarly, we did not observe a difference in AVF outcomes between the high and low eBFR groups in the present study. However, the low eBFR group did experience complications and surgical interventions at slightly higher rates compared to the high eBFR group, although this difference was not statistically significant. Nevertheless, it is still unclear whether patients with high eBFR have a better prognosis due to fewer AVF complications in this study. Future research should focus on increasing the sample size to further investigate the relationship between eBFR and AVF outcomes in patients.
The present study has some limitations that should be considered. First, a single-center study with a small sample size and few endpoint events could not establish causality because of the retrospective study design. Second, residual renal function, a key determinant of β2-MG clearance, was not recorded. Third, the study did not collect baseline cardiac structural indicators, such as left ventricular mass index, or serum biomarkers like BNP. Including these additional measures could have provided a more accurate assessment of cardiac status. Fourth, discrepancies were found in the prevalence of previous cardiovascular disease history and age between the high eBFR group and the low eBFR group, attributed to the study’s retrospective design. This variation likely reflects differences in health status and comorbidities among patients, which may have introduced bias when evaluating the relationship between eBFR and clinical outcomes, specifically all-cause mortality and new MACE. Future prospective multicenter studies are necessary to improve the assessment and comprehension of the optimal eBFR and its impact on all-cause mortality and new MACE. Fifth, the patients used different dialyzers for high-flux HD or online post-dilution HDF. Sixth, we did not measure AVF flow, which may be an important factor in predicting AVF survival. Finally, the primary patency of the AVF was not available in our study, which might have produced a bias in AVF survival.
5. Conclusion
In conclusion, within the range of 200–350 mL/min in Chinese high-flux dialysis patients, high eBFR (> 275 mL/min) is associated with lower risks of both all-cause mortality and new MACE compared with low eBFR (≤ 275 mL/min). This beneficial effect on clinical outcomes is independent of spKt/V and may be related to the greater clearance of middle-molecular-weight uremic substances. Our results also indicated that increased eBFR may not be associated with a higher risk of AVF failure.
Acknowledgments
The authors thank the Taylor and Francis Editing Services for editing the manuscript.
Disclosure statement
No potential conflict of interest was reported by the author(s).
Additional information
Funding
References
- Hassell DR, van der Sande FM, Kooman JP, et al. Optimizing dialysis dose by increasing blood flow rate in patients with reduced vascular-access flow rate. Am J Kidney Dis. 2001; 38(5):1–12. doi:10.1053/ajkd.2001.28580.
- Gutzwiller JP, Schneditz D, Huber AR, et al. Increasing blood flow increases kt/V(urea) and potassium removal but fails to improve phosphate removal. Clin Nephrol. 2003; 59(2):130–136. doi:10.5414/cnp59130.
- AlSahow A, Muenz D, Al-Ghonaim MA, et al. Kt/V: achievement, predictors and relationship to mortality in hemodialysis patients in the Gulf cooperation council countries: results from DOPPS (2012-18). Clin Kidney J. 2021; 14(3):820–830. doi:10.1093/ckj/sfz195.
- Pisoni RL, Arrington CJ, Albert JM, et al. Facility hemodialysis vascular access use and mortality in countries participating in DOPPS: an instrumental variable analysis. Am J Kidney Dis. 2009; 53(3):475–491. doi:10.1053/j.ajkd.2008.10.043.
- Kimata N, Karaboyas A, Bieber BA, et al. Gender, low Kt/V, and mortality in japanese hemodialysis patients: opportunities for improvement through modifiable practices. Hemodial Int. 2014; 18(3):596–606. doi:10.1111/hdi.12142.
- Asano M, Thumma J, Oguchi K, et al. Vascular access care and treatment practices associated with outcomes of arteriovenous fistula: international comparisons from the dialysis outcomes and practice patterns study. Nephron Clin Pract. 2013;124(1-2):23–30. doi:10.1159/000353733.
- Chang KY, Kim SH, Kim YO, et al. The impact of blood flow rate during hemodialysis on all-cause mortality. Korean J Intern Med. 2016; 31(6):1131–1139. doi:10.3904/kjim.2015.111.
- Zhao X, Niu Q, Gan L, et al. Blood flow rate: an independent risk factor of mortality in chinese hemodialysis patients. Semin Dial. 2021;35(3):251–257. doi:10.1111/sdi.13023.
- Ponce P, Marcelli D, Scholz C, et al. Does the extracorporeal blood flow affect survival of the arteriovenous vascular access? Hemodial Int. 2015; 19(2):314–322. doi:10.1111/hdi.12240.
- Depner TA, Rizwan S, Stasi TA. Pressure effects on roller pump blood flow during hemodialysis. ASAIO Trans. 1990; 36(3):M456–9. doi.
- Ward RA. Blood flow rate: an important determinant of urea clearance and delivered Kt/V. Adv Ren Replace Ther. 1999;6(1):75–79. doi:10.1016/s1073-4449(99)70011-7.
- Schmidt DF, Schniepp BJ, Kurtz SB, et al. Inaccurate blood flow rate during rapid hemodialysis. Am J Kidney Dis. 1991;17(1):34–37. doi:10.1016/s0272-6386(12)80247-2.
- Acchiardo SR, Hatten KW, Ruvinsky MJ, et al. Inadequate dialysis increases gross mortality rate. Asaio J. 1992; 38(3):M282–5. doi:10.1097/00002480-199207000-00038.
- Eknoyan G, Beck GJ, Cheung AK, et al. Effect of dialysis dose and membrane flux in maintenance hemodialysis. N Engl J Med. 2002; 347(25):2010–2019. doi:10.1056/NEJMoa021583.
- National kidney F. KDOQI clinical practice guideline for hemodialysis adequacy: 2015 update. Am J Kidney Dis. 2015; 66(5):884–930. doi:10.1053/j.ajkd.2015.07.015.
- Canaud B, Morena M, Cristol JP, et al. Beta2-microglobulin, a uremic toxin with a double meaning. Kidney Int. 2006;69(8):1297–1299. doi:10.1038/sj.ki.5000389.
- Maduell F, Moreso F, Pons M, et al. High-efficiency postdilution online hemodiafiltration reduces all-cause mortality in hemodialysis patients. J Am Soc Nephrol. 2013;24(3):487–497. doi:10.1681/ASN.2012080875.
- Tang CY, Zhu CP, Wang RP, et al. Effect of blood pump flow and arteriovenous fistula blood flow on the blood pressure and cardiac function in patients undergoing maintenance hemodialysis. Ther Apher Dial. 2019; 23(6):556–561. doi:10.1111/1744-9987.12809.
- Palmer SC, Rabindranath KS, Craig JC, et al. High-flux versus low-flux membranes for end-stage kidney disease. Cochrane Database Syst Rev. 2012;2012(9):CD005016. doi:10.1002/14651858.CD005016.pub2.
- Wootton DM, Ku DN. Fluid mechanics of vascular systems, diseases, and thrombosis. Annu Rev Biomed Eng. 1999;1(1):299–329. doi:10.1146/annurev.bioeng.1.1.299.
- McCarley P, Wingard RL, Shyr Y, et al. Vascular access blood flow monitoring reduces access morbidity and costs. Kidney Int. 2001;60(3):1164–1172. doi:10.1046/j.1523-1755.2001.0600031164.x.
- Lin SL, Huang CH, Chen HS, et al. Effects of age and diabetes on blood flow rate and primary outcome of newly created hemodialysis arteriovenous fistulas. Am J Nephrol. 1998;18(2):96–100. doi:10.1159/000013315.
- Ravani P, Brunori G, Mandolfo S, et al. Cardiovascular comorbidity and late referral impact arteriovenous fistula survival: a prospective multicenter study. J Am Soc Nephrol. 2004; 15(1):204–209. doi:10.1097/01.asn.0000103870.31606.90.
- Fulker D, Kang M, Simmons A, et al. The flow field near a venous needle in hemodialysis: a computational study. Hemodial Int. 2013; 17(4):602–611. doi:10.1111/hdi.12029.