Abstract
Previous studies have highlighted the significant role of complement activation in kidney injuries induced by rhabdomyolysis, intravascular hemolysis, sepsis, and ischemia-reperfusion. Nevertheless, the specific role and mechanism of complement activation in acute kidney injury (AKI) caused by wasp venom remain unclear. The aim of this study was to elucidate the specific complement pathway activated and investigate complement activation in AKI induced by wasp venom. In this study, a complement-depleted mouse model was used to investigate the role of complement in wasp venom-induced AKI. Mice were randomly categorized into control, cobra venom factor (CVF), AKI, and CVF + AKI groups. Compared to the AKI group, the CVF + AKI group showed improved pathological changes in kidneys and reduced blood urea nitrogen (BUN) levels. The expression levels of renal complement 3 (C3), complement 5 (C5), complement 1q (C1q), factor B (FB), mannose-binding lectin (MBL), and C5b-9 in AKI group were upregulated compared with the control group. Conversely, the renal tissue expression levels of C3, C5, C1q, FB, MBL, and C5b-9 were decreased in the CVF + AKI group compared to those in the AKI group. Complement activation occurs through all three pathways in AKI induced by wasp venom. Furthermore, complement depletion by CVF attenuates wasp venom-induced nephrotoxicity, suggesting that complement activation plays a primary role in the pathogenesis of wasp venom-induced AKI.
Acute kidney injury (AKI) is a common complication of various diseases in clinical practice, and is associated with high morbidity and mortality [Citation1,Citation2]. Multiple wasp stings can cause renal injury and an inflammatory response in the kidneys and are a common cause of animal toxin-related AKI in Asia [Citation3, Citation4]. Rhabdomyolysis and intravascular hemolysis are secondary to AKI induced by wasp venom [Citation5, Citation6]. Complement 3 (C3) deposits in the renal tubules of a wasp venom-induced AKI rat model suggest complement activation [Citation7]. However, the precise pathological mechanisms underlying wasp venom-induced AKI have not been comprehensively elucidated. Previous studies have highlighted the significant role of complement activation in kidney injuries induced by rhabdomyolysis, intravascular hemolysis, sepsis, and ischemia-reperfusion [Citation8–12]. Nevertheless, the specific role and mechanism of complement activation in AKI caused by wasp venom remain unclear.
Complement activation involves the activation of C3 through three pathways: the classical (CP), alternative (AP), and lectin pathways (LP). All three pathways lead to C3 activation [Citation13], followed by the formation of C5b-9, also known as the membrane attack complex. C5b-9 forms lytic pores on the outer membranes of target cells, leading to host cell lysis [Citation14].
The aim of this study was to elucidate the specific complement pathway activated and investigate complement activation in AKI induced by wasp venom. We used a mouse model of wasp venom-induced AKI to determine the levels of the complement factors, namely, complement 1q (C1q), factor B (FB), and mannose-binding lectin (MBL), which activate CP, AP, and LP, respectively, and analyze the expression of C3, complement 5 (C5), and C5b-9.
All animal experiments were approved by the Institutional Animal Care and Use Committee of Xiangyang Central Hospital (approval number: XYZXYY2022-M16). C57 male mice (20–25 g) were randomly assigned to four groups (n = 6/group). Wasp venom was obtained using a wasp venom collector (FF-3, XieKe Biotech, China) by electrical stimulation [Citation15]. The AKI group received injections of wasp venom (Vespa mandarinia) at five sites on the dorsal surface, whereas the control group received a saline solution containing 0.9% at the same locations [Citation16]. In the complement-depletion model, cobra venom factor (CVF, A600, Quidel, San Diego, CA) was dissolved in saline solution (50 µg/kg) and administered via intraperitoneal injection 6 h prior to the administration of wasp venom. After 24 h, kidney tissue samples and blood were collected, and all animals were euthanized. For histopathological assessment, the renal tissues were fixed in buffered neutral formalin, embedded in paraffin, and sectioned into 2 μm slices. Pathological changes in kidneys were assessed using hematoxylin and eosin (HE) staining. Blood urea nitrogen (BUN) level was measured using an automatic biochemical analyzer.
Immunofluorescence assays were performed on paraffin-embedded renal tissue sections, following the method described in our previous study [Citation17]. The present study used the following antibodies: anti-C3 (1:500, ab200999, Abcam, Cambridge, UK), anti-C1q (1:500, ab182451, Abcam), anti-FB (1:500, 10170-1-AP, Proteintech, Wuhan, China), and anti-MBL (1:500, HM1038, Hycult Biotech, Uden, The Netherlands). Western blot was conducted as previously described [Citation18] using primary antibodies specific to the following proteins: anti-C3 (1:1000, ab200999, Abcam), anti-C5 (1:1000, DF7719, Affinity Biosciences, China), anti-C1q (1:1000, ab182451, Abcam), anti-FB (1:1000, 10170-1-AP, Proteintech), anti-MBL (1:1000, HM1038, Hycult Biotech), and anti-GAPDH (1:5000, 60004-1-Ig, Proteintech). Total RNA was extracted from the renal tissues using the TRIzol reagent (TSINGKE, Beijing, China), and 1 μg of RNA was reverse transcribed using SynScript III RT Enzyme Mix (TSK3225, TSINGKE, Beijing, China). Real-time polymerase chain reaction (PCR) analysis was conducted using specific primer sequences as follows: C1q forward, CAAAGGAGAGAGAGGGGAGC; C1q reverse, GGTCCCTGATATTGCCTGGA; FB forward, GTAGCCAATACCGCCTTGAAG; FB reverse, TGTCAGGGAGGATAGGAATGC; MBL forward, TTGCTCTGTGATAG CCTGTG; MBL reverse, AGGGCTTCCAACACTTCC; GAPDH forward: AGTATGACT CCACTCACGGC; GAPDH reverse, CACCAGTAGACTCCACGACA. Real-time quantitative PCR was performed using the QuantStudio 12K Flex Real-Time PCR System (Applied Biosystems, Foster City, CA, USA), and gene expression levels were quantified based on the 2-ΔΔCt method. The C5b-9 levels in kidneys were measured using an enzyme-linked immunosorbent assay (ELISA) kit (E-EL-M1129c, Elabscience, Wuhan, China) as previously described [Citation17].
Histopathological evaluation of the renal tissues of mice in all groups was conducted using HE staining. Twenty-four hours post injection of wasp venom, tubular injury was evident, characterized by the loss of brush border cells, tubular epithelial cell degeneration, necrosis, and the accumulation of cellular debris in the tubular lumen (). Both the control and CVF groups exhibited normal renal histological structure. Notably, pathological changes in the kidney were improved in the CVF + AKI group compared to those in the AKI group (). The BUN levels for the control and CVF groups did not differ significantly (). Mice injected with wasp venom demonstrated a significantly elevated level of BUN compared to those of the control and CVF groups. Furthermore, the BUN level was reduced in the CVF + AKI group compared with the AKI group ().
Figure 1. Complement depletion via CVF attenuates nephrotoxicity induced by wasp venom in mice.
(A) Pathological changes in the kidney of mice in the control, CVF, AKI, and CVF + AKI groups. All images were acquired from HE-stained sections (Scale bar: 50 μm). (B) Tubular injury score in four groups. (C) Renal function was assessed using BUN in all groups. All analyses of the data were presented as means and standard deviations. *p < 0.05 versus control or CVF group, #p < 0.05 versus AKI group.
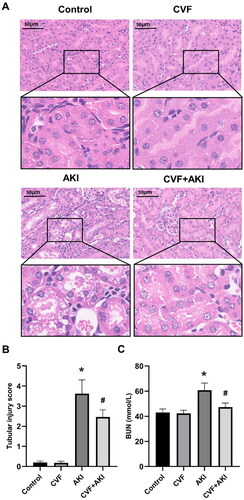
To confirm the activation of complement in the kidney and its associated pathways in the model of AKI induced by wasp venom, we assessed the expression of renal C3, C5, and the markers of all three complement pathways – C1q (CP), FB (AP), MBL (LP) in – using immunofluorescence (), western blot (), and real-time PCR (). Moreover, ELISA was employed to assess the formation of the renal C5b-9 complement complex (). The expression levels of C3, C5, C1q, FB, MBL, and C5b-9 in kidneys in the AKI group were upregulated compared to those in the control group. Conversely, renal tissue expression levels of C3, C5, C1q, FB, MBL, and C5b-9 were decreased in the CVF + AKI group compared to those in the AKI group.
Figure 2. Evaluation of renal C3 and markers for all three complement pathways in the model of wasp venom-induced AKI.
Analysis of changes in C3, C1q, FB, and MBL in the kidneys of mice in the control, AKI, and CVF + AKI groups using immunofluorescence.
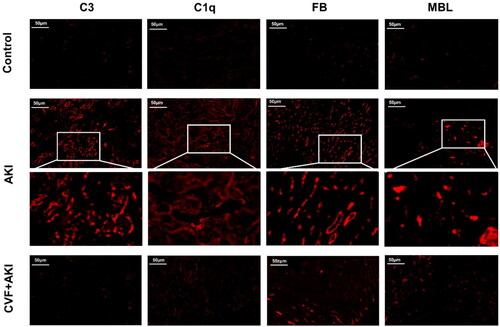
Figure 3. Complement factors C3 and C5 in kidneys were activated through all three pathways in the model of wasp venom-induced AKI.
Western blot was used to quantify C3, C5, C1q, FB, and MBL protein levels in the kidneys. *p < 0.05 versus control group, #p < 0.05 versus AKI group.
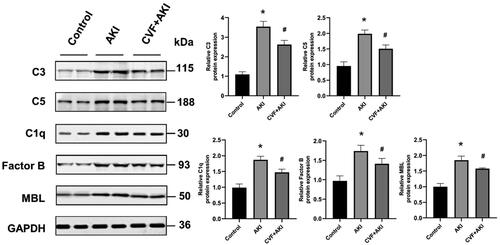
Figure 4. Assessment of renal C1q, FB, MBL and C5b-9 in the model of wasp venom-induced AKI.
(A) The mRNA expression levels of C1q, FB, and MBL in the kidneys of the three groups. (B) C5b-9 was measured using ELISA in three groups. *p < 0.05 versus control group, #p < 0.05 versus AKI group.
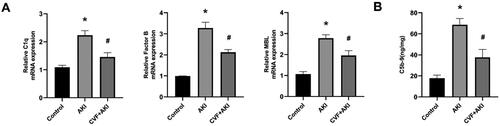
Wasp stings could lead to various clinical complications, including anaphylaxis, AKI, acute hepatic injury, myocardial damage, and nervous system damage. AKI was found to be one of the most common complications of wasp stings [Citation19]. In our recent study, we observed increased renal C3 expression in wasp venom-induced AKI rats compared to those without wasp venom [Citation7]. However, the specific complement pathway and mechanisms underlying this observation remain unclear. Elevated C3 levels in kidneys indicate local complement activation [Citation20]. Renal complement activation plays a crucial role in kidney injury associated with hemolysis, rhabdomyolysis, ischemia-reperfusion, and sepsis [Citation8–12]. However, the specific triggers of complement activation in wasp venom-induced AKI are still not well understood. No commercial wasp venom antibodies were suitable for immunofluorescent staining or western blotting; therefore, we were unable to directly detect the wasp venom in renal tubular epithelial cells. Wasp venom can cause rhabdomyolysis and intravascular hemolysis, resulting in the release of myoglobin and free hemoglobin. Heme, the core chemical structure in hemoglobin and myoglobin [Citation21], has been implicated in triggering rapid complement deposition in the kidney [Citation10]. Our previous study revealed that heme oxygenase 1, a critical enzyme for heme metabolism, is one of the most upregulated genes in a rat model of wasp venom-induced AKI [Citation22], suggesting the significant role of heme in AKI induced by wasp venom. The indirect effects of heme, resulting from rhabdomyolysis and intravascular hemolysis, may contribute to complement activation in the kidney of a wasp venom-induced AKI model.
C3 is the central component of the complement cascade, and all three complement activation pathways, CP, AP, and LP, converge at C3. In our study, the expression levels of C1q, FB, and MBL in the kidneys of mice were measured to elucidate the complement activation pathways in wasp venom-induced AKI. Our data suggest the presence of all three complement activation pathways in the mouse kidney of wasp venom-induced AKI. This finding is consistent with the activations of all three complement pathways observed in ischemia-reperfusion injury and rhabdomyolysis-related AKI [Citation8, Citation23]. A different study has demonstrated that other animal toxins, such as snake venom, also activate complement through all three pathways [Citation24]. However, Boudhabhay et al. reported that renal complement activation in glycerol-induced rhabdomyolysis-related AKI is primarily induced by both LP and AP [Citation12]. These discrepancies can be attributed to several factors. The components of wasp venom are complex, potentially involving multiple mechanisms in AKI induced by wasp venom beyond just rhabdomyolysis. Further investigation is required to determine the specific complement activation pathway that plays a major role in AKI induced by wasp venom.
Previous studies have provided evidence that local complement activation in the kidney can induce cell death and inflammation during AKI development [Citation12, Citation25]. Our previous studies have shown that cell death (apoptosis, necroptosis) and inflammation in the kidney are significant features of wasp venom-induced AKI [Citation17, Citation22]. Additionally, C5b-9 has been found to play a pathological role in the kidneys. Consistent with the present study, quantitative analysis has demonstrated an increased expression of the terminal complement activation product C5b-9 in the kidneys of wasp venom-induced AKI. C5b-9 can form a lytic pore on the outer membrane of target cells, facilitating the influx of Ca2+ and leading to intracellular calcium overload, ATP depletion, and eventually cell death [Citation8]. In a model of renal ischemia-reperfusion injury, mice deficient in C5b-9 production exhibited attenuated renal damage [Citation26]. Additionally, C5b-9 has a regulatory function in inflammation [Citation27]. Transcriptomic data from a rhabdomyolysis-induced AKI model indicated that complement activation upregulates the inflammatory response [Citation12]. C5b-9 may contribute to wasp venom-induced AKI by inducing kidney cell death and promoting inflammation. Our recent studies have highlighted the significant contribution of cell death and inflammation to the development of AKI induced by wasp venom [Citation11, Citation17, Citation22]. These findings suggest an association between complement activation and cell death and inflammation in wasp venom-induced AKI. However, the specific pathways of complement that regulate inflammation and the pathogenic effects of other complement cascade products, such as C3a and C5a, in our model remain unclear. Therefore, further investigation is required to determine the underlying mechanisms.
The safety of complement depletion by CVF has been confirmed by the present and previous studies as there were no changes in morphology or renal function after CVF injection alone in animals [Citation8]. CVF acts as a C3/C5 convertase, rapidly cleaving C3 and C5 [Citation28]. CVF administration is an effective and long-lasting method of complement elimination. The degree of CVF depleted in the complement system depends on the amount of CVF [Citation29]. In the present investigation, we explored the role of complement by depleting complement prior to the injection of wasp venom. Our results demonstrated that CVF injection effectively blocks all three complement activation pathways and prevents downstream production of C3, C5, and C5b-9 in a wasp venom-induced AKI model. These findings are consistent with other studies indicating the potential role of CVF in glycerol-induced rhabdomyolysis-associated AKI. Furthermore, complement elimination by CVF ameliorated changes in renal tissue morphology and decline in renal function, suggesting a key role of complement activation in the pathogenesis of wasp venom-induced AKI. Wasp venom-induced AKI can also be caused by acute interstitial nephritis. Acute interstitial nephritis induced by wasp stings is probably related to hypersensitivity reactions by allergenic components of wasp venom [Citation3]. However, the critical determinants of acute interstitial nephritis induced by wasp stings are unknown.
Conclusively, the present study reveals that complement activation occurs through all three pathways in AKI induced by wasp venom. This activation can be attributed to the presence and role of heme. Importantly, complement depletion demonstrates the ability to attenuate the nephrotoxicity induced by wasp venom. Complement inhibitors, pegcetacoplan (C3 inhibitor) and eculizumab (C5 inhibitor), are currently used clinically, and could be potential therapeutic candidates for wasp venom-induced AKI.
Disclosure statement
No potential conflict of interest was reported by the author(s).
Additional information
Funding
References
- Hoste EAJ, Kellum JA, Selby NM, et al. Global epidemiology and outcomes of acute kidney injury. Nat Rev Nephrol. 2018;14(10):607–625. doi:10.1038/s41581-018-0052-0.
- Kidney Disease: improving Global Outcomes (KDIGO) Acute Kidney Injury Work Group. KDlGO clinical practice guideline for acute kidney injury. Kidney Int Suppl. 2012;2(1):1–138.
- Yu F, Wang L, Yuan H, et al. Wasp venom-induced acute kidney injury: current progress and prospects. Ren Fail. 2023;45(2):2259230.
- Wang M, Prince S, Tang Y, et al. Macroscopic hematuria in wasp sting patients: a retrospective study. Ren Fail. 2021;43(1):500–509. doi:10.1080/0886022X.2021.1896547.
- Yuan H, Gao Z, Chen G, et al. An integrative proteomics metabolomics based strategy reveals the mechanisms involved in wasp sting induced acute kidney injury. Toxicon. 2022;205:1–10. doi:10.1016/j.toxicon.2021.11.005.
- Vikrant S, Parashar A. Acute kidney injury due to multiple hymenoptera stings – a clinicopathological study. Clin Kidney J. 2017;10(4):532–538. doi:10.1093/ckj/sfx010.
- Han J, Cui L, Yu F, et al. Megalin blockade with cilastatin ameliorates multiple wasp sting-induced acute kidney injury in rats. Toxicon. 2022;220:106960. doi:10.1016/j.toxicon.2022.106960.
- Huang X, Zhao W, Zhang L, et al. The role of complement activation in rhabdomyolysis-induced acute kidney injury. PLoS One. 2018;13(2):e0192361. doi:10.1371/journal.pone.0192361.
- Keshari RS, Popescu NI, Silasi R, et al. Complement C5 inhibition protects against hemolytic anemia and acute kidney injury in anthrax peptidoglycan-induced sepsis in baboons. Proc Natl Acad Sci USA. 2021;118(37):1–12.
- Merle NS, Grunenwald A, Rajaratnam H, et al. Intravascular hemolysis activates complement via cell-free heme and heme-loaded microvesicles. JCI Insight. 2018;3(12):1–17. doi:10.1172/jci.insight.96910.
- Chen Y, Lin L, Rao S, et al. Complement C3 mediates podocyte injury through TLR4/NFΚB-P65 signaling during ischemia-reperfusion acute kidney injury and post-injury fibrosis. Eur J Med Res. 2023;28(1):135. doi:10.1186/s40001-023-01054-1.
- Boudhabhay I, Poillerat V, Grunenwald A, et al. Complement activation is a crucial driver of acute kidney injury in rhabdomyolysis. Kidney Int. 2021;99(3):581–597. doi:10.1016/j.kint.2020.09.033.
- van Zanden JE, Jager NM, Seelen MA, et al. Brain death-induced lung injury is complement dependent, with a primary role for the classical/lectin pathway. Am J Transplant. 2021;21(3):993–1002. doi:10.1111/ajt.16231.
- Serna M, Giles JL, Morgan BP, et al. Structural basis of complement membrane attack complex formation. Nat Commun. 2016;7(1):10587. doi:10.1038/ncomms10587.
- Zhou ST, Luan K, Ni LL, et al. A strategy for quality control of Vespa magnifica (Smith) venom based on HPLC fingerprint analysis and multi-component separation combined with quantitative analysis. Molecules. 2019;24(16):2920. doi:10.3390/molecules24162920.
- Yu F, Cui L, Gao Z, et al. A rat model of acute kidney injury caused by multiple subcutaneous injections of Asian giant hornet (Vespa mandarina Smith) venom. Toxicon. 2022;213:23–26. doi:10.1016/j.toxicon.2022.04.008.
- Lv Y, Lu L, Yu F, et al. STING deficiency protects against wasp venom-induced acute kidney injury. Inflamm Res. 2023;72(7):1427–1440. doi:10.1007/s00011-023-01749-5.
- Yuan H, Gao Z, Lu X, et al. Role of collectin-11 in innate defence against uropathogenic Escherichia coli infection. Innate Immun. 2021;27(1):50–60. doi:10.1177/1753425920974766.
- Ruwanpathirana P, Priyankara D. Clinical manifestations of wasp stings: a case report and a review of literature. Trop Med Health. 2022;50(1):82. doi:10.1186/s41182-022-00475-8.
- McCullough JW, Renner B, Thurman JM. The role of the complement system in acute kidney injury. Semin Nephrol. 2013;33(6):543–556. doi:10.1016/j.semnephrol.2013.08.005.
- Fakih TM, Kurniawan F, Yusuf M, et al. Molecular dynamics of cobalt protoporphyrin antagonism of the cancer suppressor REV-ERBbeta. Molecules. 2021;26(11):3251. doi:10.3390/molecules26113251.
- Wang R, Gao D, Yu F, et al. Phospholipase A2 inhibitor varespladib prevents wasp sting-induced nephrotoxicity in rats. Toxicon. 2022;215:69–76. doi:10.1016/j.toxicon.2022.06.007.
- Bongoni AK, Vikstrom IB, McRae JL, et al. A potent truncated form of human soluble CR1 is protective in a mouse model of renal ischemia-reperfusion injury. Sci Rep. 2021;11(1):21873. doi:10.1038/s41598-021-01423-y.
- Delafontaine M, Villas-Boas IM, Pidde G, et al. Venom from Bothrops lanceolatus, a snake species native to Martinique, potently activates the complement system. J Immunol Res. 2018;2018:3462136. doi:10.1155/2018/3462136.
- Tang X, Wei T, Guan M, et al. Phospholipase A(2) induces acute kidney injury by complement mediated mitochondrial apoptosis via TNF-alpha/NF-kappaB signaling pathway. Food Chem Toxicol. 2023;172:113591. doi:10.1016/j.fct.2022.113591.
- Zhou W, Farrar CA, Abe K, et al. Predominant role for C5b-9 in renal ischemia/reperfusion injury. J Clin Invest. 2000;105(10):1363–1371. doi:10.1172/JCI8621.
- Bekassy Z, Lopatko Fagerström I, Bader M, et al. Crosstalk between the renin-angiotensin, complement and kallikrein-kinin systems in inflammation. Nat Rev Immunol. 2022;22(7):411–428. doi:10.1038/s41577-021-00634-8.
- Mershon KL, Vasuthasawat A, Lawson GW, et al. Role of complement in protection against Cryptococcus gattii infection. Infect Immun. 2009;77(3):1061–1070. doi:10.1128/IAI.01119-08.
- Vogel CW, Fritzinger DC. Cobra venom factor: structure, function, and humanization for therapeutic complement depletion. Toxicon. 2010;56(7):1198–1222. doi:10.1016/j.toxicon.2010.04.007.