Abstract
Indigenous bacteria in the mammalian gastrointestinal tract comprise a rich ecosystem of organisms and secreted factors with direct effects on intestinal immunity. Commensal constituents of the gastrointestinal microbiota include the lactic acid bacteria such as Lactobacillus species. The genus Lactobacillus includes a restricted set of intestine-indigenous species from a pool of more than 100 Lactobacillus species. Scientific challenges include the abilities to define commensal species and specific bacterial clones with specific immunomodulatory activities. Our studies have resulted in the identification of Lactobacillus strains from mice and humans with potent anti-inflammatory effects. Commensal-derived Lactobacillus strains were isolated from healthy mice lacking evidence of intestinal inflammation and identified as candidate probiotics due to immunomodulatory activities. Less than 10% of commensal strains isolated from humans and mice had potent tumor necrosis factor (TNF)-α-inhibitory activity. These strains down-regulated the production of TNF-α by macrophage cell lines and successfully suppressed inflammation in mouse colitis models. Human-derived Lactobacillus reuteri strains have been identified with potent human TNF-α-inhibitory effects on lipopolysaccharide (LPS)-activated human myeloid cell lines and primary monocyte-derived macrophages from children with Crohn's disease. Human commensal-derived probiotics suppressed the transcription of human TNF-α by inhibition of MAP kinase signaling via AP-1. Alternatively, commensal-derived human L. reuteri strains suppressed nuclear factor (NF)-κB signaling in TNF-α-stimulated human myeloid cells. Suppression of NF-κB signaling in TNF-α-activated immune cells promotes apoptosis of activated immune cells and provides a novel mechanism for anti-inflammatory action in the mammalian intestine. In summary, commensal-derived probiotics provide opportunities for understanding mechanisms of microbial–host interactions in the gastrointestinal tract. By understanding mechanisms of probiosis, novel therapeutic strategies may be developed for prevention and treatment of inflammatory bowel disease and other intestinal disorders.
Commensal-derived probiotics
The commensal microbiota in the digestive tract is composed of hundreds of bacterial species and the latest estimates suggest that 800–1000 species may be present in the human gastrointestinal tract. According to a published study that included comprehensive DNA sequence analyses Citation[1], >80% of bacteria were unculturable and >60% of organisms were considered novel species. The sheer quantities of unknown elements of autochthonous microbiota highlight the amount of scientific exploration necessary to understand diverse biological functions in the intestinal tract. Many indigenous or commensal bacterial species remain to be defined in different mammals, including humans, although several species have been identified as indigenous organisms in particular animals. The genus Lactobacillus includes >100 different species, and only a restricted subset of species are considered to be truly commensal or indigenous microbes in the human intestine.
The rational selection of probiotic bacteria and prebiotics provides opportunities for the prevention and treatment of various digestive and systemic diseases, based on recent evidence from animal and human studies Citation[2–5]. ‘Old friends’ or beneficial microbes such as Lactobacillus strains may regulate immune responses and promote the robust development of the intestinal immune system in the host Citation[6–8]. Relative depletion or deficiencies of specific commensal microorganisms have been associated with an increased prevalence of autoimmune and allergic disorders in the developed world, and these findings have been articulated in the ‘hygiene hypothesis.’ For example, children with allergies in Estonia and Sweden yielded lower quantities of lactobacilli and greater quantities of coliforms and Staphylococcus aureus than non-allergic children Citation[9]. Restoration of depleted microbial populations with beneficial immunomodulatory functions may promote heath and reduce disease risk in pediatric and adult populations.
The intentional remodeling of microbial communities in the gastrointestinal tract may result in the modulation of immune responses in the intestinal mucosa Citation[10]. Commensal-derived probiotics are attractive candidates for new biological therapies because such beneficial bacteria may be well adapted to persistently colonize particular hosts. Rational screening and selection of commensal-derived probiotics may result in microbes that perform desired anti-inflammatory functions effectively in vivo.
Probiotics and innate immunity: cytokines and apoptosis
The innate immune system represents the primary interface between the microbial world and the host Citation[11], Citation[12]. The molecular bases of interactions between bacteria and the intestinal mucosa may hold the key to a greater understanding of intestinal responses to injury. A variety of bacteria directly modulate cytokine networks and innate immune responses by multiple mechanisms Citation[13]. Messenger molecules that are important for intercellular communication among bacteria (e.g. quorum sensing) may affect host intestinal physiology Citation[14], Citation[15]. Microbial and host response networks represent a poorly understood part of the intestinal landscape and may be important targets for anti-inflammatory probiotics-based strategies in vivo.
Intestinal epithelial cells (IECs) act as sentinels for the innate immune system and interact directly with commensal and pathogenic bacteria Citation[16–18]. Pattern recognition receptors including Toll-like receptors (TLRs) permit differential recognition of bacterial macromolecules such as lipopolysaccharide (LPS), peptidoglycans, and DNA Citation[19]. LPS derived from gram-negative bacteria binds accessory proteins such as CD14, ligates TLR4, and causes NF-κB activation, resulting in the production of proinflammatory chemokines (e.g. interleukin-8 (IL-8) and monocyte chemoattractant protein-1 (MCP-1)) by intestinal epithelial cells Citation[20–23]. Our studies have demonstrated that conditioned media of human-derived Lactobacillus reuteri strains suppress IL-8 production by human intestinal epithelial HT-29 cells ().
Figure 1. Inhibition of LPS-induced IL-8 production by L. reuteri-conditioned media. HT-29 cells were cultured and stimulated with LPS (25 ng/ml) supplemented with soluble CD14 (solid bars) in the presence of bacterial culture medium (MRS). Pretreatment of HT-29 cells (4 h) with Lactobacillus-conditioned media before LPS challenge significantly diminished the production of IL-8, compared with cells pretreated with MRS media alone (*p<0.05). Human IL-8 levels were measured by quantitative ELISAs (Quantikine, R&D Systems, Minneapolis, MN, USA). MRS, de Man, Rogosa, Sharpe bacteriologic medium.
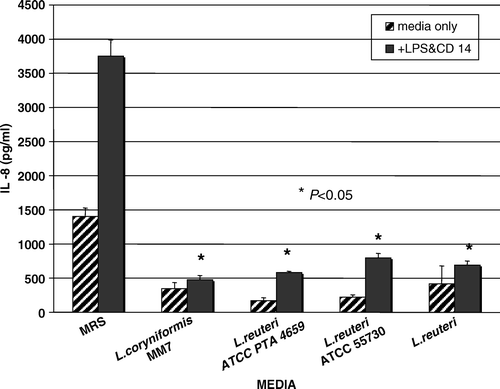
Human-derived L. reuteri and Lactobacillus coryniformis clones secrete soluble factors that inhibit elevations in human IL-8 production caused by bacteria-derived LPS (). Prior studies indicated that other Lactobacillus species could suppress IEC chemokine production Citation[24] including TNF-α-stimulated IL-8 production Citation[25] and activation of proapoptotic p38/mitogen-activated protein (MAP) kinases Citation[26]. Human TNF-α ligates the TNF receptors and leads to NF-κB activation via a TLR-independent, TRAF2-dependent mechanism Citation[27]. Similarly, our results indicate that Lactobacillus clones may diminish the induction of IL-8 mRNA and protein levels. These data, in addition to previously published data Citation[25], suggested that probiotic Lactobacillus modulates human IL-8 expression by inhibiting NF-κB activation at a terminal step in the pathway. Reduced levels of activated p65 were detected in nuclear extracts of HT-29 cells stimulated with TNF-α and treated with LactobacillusCitation[25], suggesting that commensal Lactobacillus clones inhibit translocation of NF-κB p50-p65 heterodimers. Alternatively, lactobacilli-derived factors may affect IL-8 mRNA stability as a result of alterations in cell signaling pathways. Downstream sequences in the 3′ untranslated region of the IL-8 gene played a role in the regulation of IL-8 expression Citation[28]. The p38 MAP kinase pathway contributed to IL-8 mRNA stability by affecting stabilization of mRNA via the 3′ untranslated region Citation[29]. Possibly, downstream effects on IL-8 mRNA stability and MAP kinase-activated protein kinase 2 may account, at least partly, for the diminution in IL-8 responses to bacterial NF-κB activating macromolecules. Interestingly, immunosuppressive glucocorticoids suppressed IL-8 production by destabilizing IL-8 mRNA Citation[30].
L. reuteri–IEC interactions played a significant role in the immunoprobiotic mode of action Citation[31]. Viable L. reuteri cells up-regulated nerve growth factor (NGF) in human epithelial cell lines while inhibiting, in a dose-dependent manner, the constitutive synthesis of IL-8 as well as TNF-α-induced IL-8 synthesis. Probiotic persistence and mucosal stimulation in the gut depended on viable probiotics Citation[31]. Only live L. reuteri inhibited IL-8 synthesis induced by S. enterica serovar Typhimurium Citation[31]. L. reuteri required pre-incubation and adherence of live cells for chemokine-inhibitory effects. Furthermore, only live L. reuteri inhibited nuclear translocation of NF-κB by preventing degradation of IκB.
The importance of TNF-α in chronic intestinal inflammation has been emphasized by the efficacy of the monoclonal chimeric anti-TNF-α antibody, infliximab, in the treatment of refractory Crohn's disease Citation[32–34]. TNF signaling pathways via specific TNF receptors represent possible targets for anti-inflammatory strategies. Soluble TNF-α binds to either TNFR1 or TNFR2 and triggers downstream signaling including NF-κB and MAP kinase activation Citation[35]. TNFR2 is essential for TNF-α signaling, including NF-κB activation, in murine macrophages Citation[36]. TNFR2 is also expressed in human intestinal T cells and contributed to experimental colitis in mouse models Citation[37]. Expression of TNFR2 was up-regulated in colonic epithelial and mononuclear cells and was associated with NF-κB activation and intestinal inflammation in the mouse TCRα subunit-deficient model Citation[38]. Probiotics reversed TNF-α-mediated effects in intestinal epithelial cells such as increased intestinal permeability and STAT1/3 activation by cytokines Citation[39]. Presumably, probiotics may interfere with TNF-mediated signaling in IECs and macrophages.
Commensal bacteria produce immunoregulatory factors, or ‘immunomodulins,’ that directly suppress cytokine production Citation[13]. Different Lactobacillus species, including L. reuteri, inhibited proinflammatory cytokine production in bone marrow-derived dendritic cells Citation[40]. In contrast, IL-10 production by dendritic cells was maintained at similar levels. Different Lactobacillus strains may differ with respect to immunosuppressive effects. In contrast to Lactobacillus crispatus, Lactobacillus casei and Lactobacillus bulgaricus strains down-regulated TNF-α production by human intestinal explants from patients with Crohn's disease Citation[41], Citation[42]. Viable bacteria were added to organ cultures of human ileal explants, and only L. casei and L. bulgaricus strains significantly inhibited intestinal TNF-α secretion in inflamed and non-inflamed tissues Citation[41]. The inhibition of cytokine production may result from the secretion of soluble mediators by probiotic bacteria. Supernatants of human-derived L. reuteri strains may suppress LPS-stimulated TNF-α-production by human monocytoid cells, in contrast to immunostimulatory L. reuteri strains which enhance human TNF production (). Lactic acid bacteria secreted anti-inflammatory metabolites (<10 kDa) that inhibited human TNF-α production by human peripheral blood mononuclear cells Citation[43].
Figure 2. Strain-dependent suppression of TNF-α in human monocytoid cells by Lactobacillus reuteri. Cell culture bioassays were performed to determine L. reuteri-mediated TNF-α suppression. Human monocytoid cell line, THP-1 (ATCC TIB-202), was co-incubated with Escherichia coli lipopolysaccharide (LPS) and conditioned media derived from four strains of L. reuteri. Secreted human TNF-α cytokine was then measured by TNF-α ELISA in the cell culture supernatants. Human-derived L. reuteri exhibited strain-dependent and cell-contact independent suppression of TNF-α production. L. reuteri strains ATCC PTA 4659 and ATCC PTA 6475 significantly suppressed TNF-α production by 88.7% and 92.4%, respectively, compared with the MRS bacterial medium only control. **Statistical significance with p<0.001. x-axis, bioassay cultures with or without LPS; y-axis, secreted TNF-α in pg/ml; MRS, de Man-Rogosa-Sharpe Lactobacillus-selective bacterial culture medium.
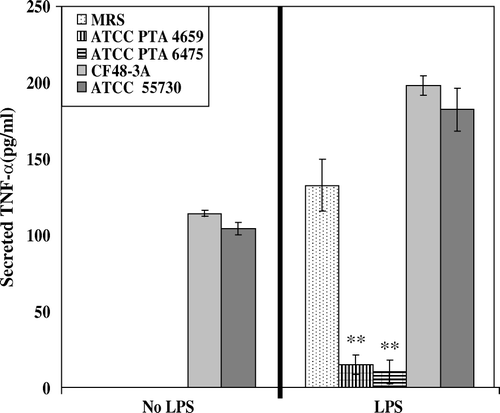
Commensal-derived probiotics may drive activated immune cells towards apoptosis, thereby depleting immune cells primed to produce proinflammatory cytokines. Our preliminary studies with human KBM-5 myeloid cells suggest that human-derived L. reuteri promotes apoptosis of TNF-activated cells (data not shown). Conjugated linoleic acids (CLAs) produced by probiotics (VSL#3) had anti-inflammatory features and stimulated the production of peroxisome proliferator activated receptor-γ (PPAR-γ) Citation[44]. CLA derived from VSL#3 also induced apoptosis of human intestinal epithelial cells Citation[44] and may confer anticarcinogenic effects due to pro-apoptotic probiotic functions. Prior studies demonstrated that avirulent Salmonella pullorum suppressed NF-κB activation in intestinal epithelial cells Citation[45]. NF-κB activation is linked with suppression of apoptosis, and this connection offers possible insights into alternative mechanisms of immunomodulation by probiotics. The selective promotion of apoptosis of activated macrophages and intestinal epithelial cells may serve to deplete cell populations and result in down-regulation of cytokine production in the intestinal mucosa. In contrast, a recent study reported that specific proteins secreted by probiotic Lactobacillus rhamnosus GG (LGG) suppress apoptosis of TNF-α-activated IECs Citation[46]. Another study documented that extracts of the probiotic Bifidobacterium infantis suppressed T-lymphocyte-induced apoptosis of human keratinocytes in an atopic dermatitis model Citation[47], and probiotics suppressed Citrobacter rodentium-induced apoptosis following colonic infection in mice Citation[48]. Perhaps probiotics have different effects on antigen-presenting cells (APCs) and epithelial cells, or different probiotic strains either promote or suppress apoptosis of specific cell types.
The concept of regulatory APCs including macrophages and dendritic cells has been proposed by Rook et al. Citation[7], and such regulatory APCs may be stimulated by commensal-derived probiotics to suppress proinflammatory immune responses. Probiotic components of VSL#3 selectively stimulated IL-10 production by dendritic cells Citation[49], providing evidence that probiotics may skew or promote the relative proliferation of immunoregulatory dendritic cell subpopulations. Probiotics that induce dendritic cell populations expressing the immunoregulatory enzyme indoleamine 1,3 dioxygenase (IDO) inhibit inflammation in the TNBS-induced colitis model Citation[50]. Probiotic-mediated regulation of dendritic cell functions required pattern recognition receptors and intact MyD88 signaling pathways. Probiotics may exert anti-inflammatory effects by enhancing the relative abundance of regulatory APC populations, thereby increasing the production of immunoregulatory cytokines such as IL-10.
Probiotics and adaptive immunity: effector and regulatory T lymphocytes
Commensal-derived probiotics may suppress effector T-cell responses by inhibiting the proliferation of effector T-helper type 1 (Th1) and type 2 (Th2) cells. Von der Weid et al. Citation[51] also investigated whether certain strains of lactic acid bacteria are able to antagonize specific T-helper functions in vitro and thus potentially prevent immunopathology. The bacteria were co-cultured with mixed lymphocyte cultures whereby CD4+ T cells from naïve BALB/c mice were stimulated by irradiated allogenic splenocytes. One strain of L. paracasei strongly inhibited the proliferative activities of CD4+ T cells in a dose-dependent fashion, and the suppression of cell proliferation was accompanied by diminished Th1 and Th2 effector cytokines, including interferon (IFN)-γ, IL-4, and IL-5. At the same time, the counter-regulatory cytokines, IL-10 and TGF-β, were maintained and markedly increased, respectively, in a dose-dependent manner. Administration of probiotics, Lactobacillus salivarius 433118 and Bifidobacterium infantis 35624, resulted in significant attenuation of colitis and arthritis in IL-10 knockout mice associated with reduced Th1 cytokines, while levels of TGF-β were maintained Citation[52].
Commensal-derived probiotics may promote the proliferation of regulatory T lymphocytes, resulting in cell contact-dependent immunoregulation and the secretion of anti-inflammatory cytokines. Oral administration of L. casei reduces skin inflammation in a model of allergic contact dermatitis through the expansion of CD4+ IL-10-secreting T cells Citation[53]. Co-culture of probiotic L. paracasei in mixed leukocyte reaction systems inhibited both Th1 and Th2 effector cytokines and resulted in the relative expansion of IL-10-producing Tr1 cells and TGF-β-secreting Th3 cells Citation[51]. Combination probiotic therapy reduced the severity of recurrent Th1-mediated murine colitis by induction of IL-10-producing regulatory T lymphocytes Citation[54]. The concept of ‘old friends’ has been described Citation[7] and postulates that indigenous and environmental microbiota stimulate the expansion of regulatory T cells and APC clones. Lactobacillus species that represent indigenous components of the intestinal microbiota may expand regulatory T-cell (Treg, Tr1, Th3) populations either directly or via other cell types such as dendritic cells. The concept of ‘bystander suppression’ Citation[7] states that ‘old friends’ such as Lactobacillus strains may activate regulatory T-cell or APC populations. L. reuteri strains, may instruct APCs to direct T cells towards regulatory functions. The stimulatory effects of probiotic Lactobacillus, including L. reuteri strains, on immune cell functions may play a central role in immunoprobiosis.
Probiotics and regulation of key signaling pathways in immune cells
Bacteria-derived LPS and other molecules bind to TLRs on the cell surface and trigger various signaling pathways in the host Citation[55], Citation[56]. Recent studies indicate that commensal bacteria may modulate intestinal homeostasis by constitutive signaling via pattern recognition receptors Citation[57]. The lack of MyD88 and intact NF-κB signaling resulted in diminished survival of mice in an acute intestinal injury model including gamma irradiation Citation[58]. Furthermore, the removal of commensal bacteria by antibiotic treatment diminished survival, suggesting that maintenance of an intact microbiota and NF-κB signaling were important for intestinal homeostasis Citation[58]. Mice lacking IκB-kinase (IKK)-beta are deficient in NF-κB activation and yielded evidence of enhanced radiation-induced epithelial cell apoptosis in the intestine Citation[59]. Our own studies have shown that specific human-derived L. reuteri strains may inhibit NF-κB signaling in a human myeloid cell line () and modulate injury repair capacity in the intestine. Probiotic mechanisms of action may include cross-talk between multiple signaling pathways in epithelial and immune cells. Commensal bacteria and commensal-derived probiotics may promote the development of oral tolerance in the gastrointestinal tract. Salmonella enterica facilitates the production of factors associated with macrophage tolerance such as regulatory NF-κB subunits p50 and p52 Citation[60]. The genes associated with macrophage tolerance are stimulated by pattern recognition receptors other than TLR4 Citation[60], and may result in regulated immune responses to commensal bacteria.
Figure 3. Lactobacillus reuteri inhibits TNF-induced NF-κB activation. KBM-5 cells (2×106 cells/well) were incubated with different L. reuteri conditioned media (20%) for 16 h, activated with TNF (0.1 nM) for 30 min, and analyzed for NF-κB activation by EMSA. The dried gels were visualized with a Storm 820 and radioactive bands were quantified using ImageQuant software (Amersham Pharmacia Biotechnology, Piscataway, NJ, USA). In the presence of TNF-α, pretreatment with L. reuteri ATCC PTA 6475 significantly inhibited NF-κB activation compared with strain ATCC PTA 4659.
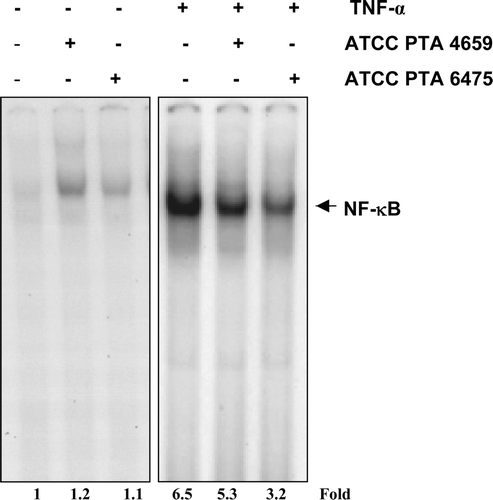
Commensal-derived probiotics may suppress signaling pathways such as NF-κB by any of several mechanisms. Avirulent Salmonella may suppress the degradation of the NF-κB complex inhibitor, IκB Citation[45]. IκB was effectively stabilized in the cytoplasm by the ability of the non-pathogenic Salmonella to inhibit the ubiquitination of the IκB-α. Avirulent enteric bacteria inhibited IκB-α proteasomal degradation and thereby NF-κB translocation to the nucleus. The commensal bacterium, Bacteroides thetaiotamicronCitation[61], suppressed NF-κB signaling by a different mechanism. B. thetaiotamicron inhibited the function of the proinflammatory transcription factor, NF-κB, in pathogen-challenged Caco-2 cells by triggering association of the RelA subunit of NF-κB with the nuclear hormone receptor and transcription factor PPAR-γ. Kelly et al. Citation[61] proposed that the PPAR-γ/RelA complex is rapidly exported from the nucleus, resulting in attenuated expression of NF-κB-regulated inflammatory genes.
Probiotics may secrete different factors that modulate immune signaling pathways by cell contact-independent mechanisms. Soluble factors from LGG activate MAP kinases and induce cytoprotective heat shock protein genes Citation[62]. A fungal probiotic agent, “Saccharomyces boulardii,” inhibited ERK1/2 MAP kinase and may account for its protection against Clostridium difficile-induced disease Citation[63]. Probiotic-treated IECs demonstrated reduced activation of SOCS3, STAT1, and STAT3, and the suppression of STAT signaling may account for diminished deleterious effects of proinflammatory cytokines TNF-α and IFN-γ Citation[39]. IL-6 is a proinflammatory cytokine that signals through STAT3, and the STAT3 signaling pathway contributes to intestinal inflammation. Production of IL-6 was inhibited by a polysaccharide-peptidoglycan complex of L. casei strain Shirota, and this mechanism of immunosuppression is due to inhibition of nuclear translocation of NF-κB Citation[64].
Summary and Conclusions
An increased understanding of proinflammatory signaling pathways in enterocytes and how these pathways may be targeted by probiotic bacteria may offer hope for refinements in treatment strategies for immune-mediated diseases. The abilities of specific human-derived Lactobacillus clones to abrogate mucosal immune responses or induce tolerance will create opportunities for alternative therapies. Conditions such as infectious gastroenteritis or inflammatory bowel disease (IBD) may be modulated by probiotic bacteria. Probiotic Lactobacillus clones may affect key signaling pathways that are shared among different inflammatory diseases. Probiotic strains with different features such as immunostimulatory or anti-inflammatory functions may be translated to diverse medical applications at the bedside in the future.
Acknowledgements
The authors would like to thank Tiffany Morgan and Karla Sternberg for helping to prepare this manuscript. The authors also thank Bharat Aggarwal and Jeremy Pena for technical assistance. This work was supported by several awards to J.V., including National Institutes of Health grant (R01 DK065075) and Senior Research Award from the Crohn's & Colitis Foundation of America (CCFA). J.V. also acknowledges support from the Defense Advanced Research Projects Agency (DARPA) and the US Office of Naval Research.
Declaration of interest: The authors only report unrestricted research support from BioGaia AB. The authors alone are responsible for the content and writing of the paper.
References
- Eckburg PB, Bik EM, Bernstein CN, Purdom E, Dethlefsen L, Sargent M, et al. Diversity of the human intestinal microbial flora. Science 2005; 308: 1635–8
- Gionchetti P, Rizzello F, Campieri M. Probiotics in gastroenterology. Curr Opin Gastroenterol 2002; 18: 235–9
- Sanders ME. Probiotics: considerations for human health. Nutr Rev 2003; 61: 91–9
- Sartor RB. Therapeutic manipulation of the enteric microflora in inflammatory bowel diseases: antibiotics, probiotics, and prebiotics. Gastroenterology 2004; 126: 1620–33
- Fedorak RN, Madsen KL. Probiotics and the management of inflammatory bowel disease. Inflamm Bowel Dis 2004; 10: 286–99
- Madsen K. Probiotics and the immune response. J Clin Gastroenterol 2006; 40: 232–4
- Rook GA, Adams V, Hunt J, Palmer R, Martinelli R, Brunet LR. Mycobacteria and other environmental organisms as immunomodulators for immunoregulatory disorders. Springer Semin Immunopathol 2004; 25: 237–55
- Guarner F, Bourdet-Sicard R, Brandtzaeg P, Gill HS, McGuirk P, van Eden W, et al. Mechanisms of disease: the hygiene hypothesis revisited. Nat Clin Pract Gastroenterol Hepatol 2006; 3: 275–84
- Bjorksten B, Naaber P, Sepp E, Mikelsaar M. The intestinal microflora in allergic Estonian and Swedish 2-year-old children. Clin Exp Allergy 1999; 29: 342–6
- Rastall RA, Gibson GR, Gill HS, Guarner F, Klaenhammer TR, Pot B, et al. Modulation of the microbial ecology of the human colon by probiotics, prebiotics and synbiotics to enhance human health: an overview of enabling science and potential applications. FEMS Microbiol Ecol 2005; 52: 145–52
- Aderem A, Ulevitch RJ. Toll-like receptors in the induction of the innate immune response. Nature 2000; 406: 782–7
- Janeway CA, Jr, Medzhitov R. Innate immune recognition. Annu Rev Immunol 2002; 20: 197–216
- Wilson M, Seymour R, Henderson B. Bacterial perturbation of cytokine networks. Infect Immun 1998; 66: 2401–9
- Sperandio V, Torres AG, Jarvis B, Nataro JP, Kaper JB. Bacteria-host communication: the language of hormones. Proc Natl Acad Sci U S A 2003; 100: 8951–6
- Kaper JB, Sperandio V. Bacterial cell-to-cell signaling in the gastrointestinal tract. Infect Immun 2005; 73: 3197–209
- Pitman RS, Blumberg RS. First line of defense: the role of the intestinal epithelium as an active component of the mucosal immune system. J Gastroenterol 2000; 35: 805–14
- Maaser C, Kagnoff MF. Role of the intestinal epithelium in orchestrating innate and adaptive mucosal immunity. Z Gastroenterol 2002; 40: 525–9
- Stadnyk AW. Intestinal epithelial cells as a source of inflammatory cytokines and chemokines. Can J Gastroenterol 2002; 16: 241–6
- Janssens S, Beyaert R. Role of Toll-like receptors in pathogen recognition. Clin Microbiol Rev 2003; 16: 637–46
- Savkovic SD, Koutsouris A, Hecht G. Activation of NF-kappaB in intestinal epithelial cells by enteropathogenic Escherichia coli. Am J Physiol 1997; 273: C1160–7
- Cario E, Rosenberg IM, Brandwein SL, Beck PL, Reinecker HC, Podolsky DK. Lipopolysaccharide activates distinct signaling pathways in intestinal epithelial cell lines expressing Toll-like receptors. J Immunol 2000; 164: 966–72
- Funda DP, Tuckova L, Farre MA, Iwase T, Moro I, Tlaskalova-Hogenova H. CD14 is expressed and released as soluble CD14 by human intestinal epithelial cells in vitro: lipopolysaccharide activation of epithelial cells revisited. Infect Immun 2001; 69: 3772–81
- Bocker U, Yezerskyy O, Feick P, Manigold T, Panja A, Kalina U, et al. Responsiveness of intestinal epithelial cell lines to lipopolysaccharide is correlated with Toll-like receptor 4 but not Toll-like receptor 2 or CD14 expression. Int J Colorectal Dis 2003; 18: 25–32
- Wallace TD, Bradley S, Buckley ND, Green-Johnson JM. Interactions of lactic acid bacteria with human intestinal epithelial cells: effects on cytokine production. J Food Prot 2003; 66: 466–72
- Bai AP, Ouyang Q, Zhang W, Wang CH, Li SF. Probiotics inhibit TNF-alpha-induced interleukin-8 secretion of HT29 cells. World J Gastroenterol 2004; 10: 455–7
- Yan F, Polk DB. Probiotic bacterium prevents cytokine-induced apoptosis in intestinal epithelial cells. J Biol Chem 2002; 277: 50959–65
- Chen G, Goeddel DV. TNF-R1 signaling: a beautiful pathway. Science 2002; 296: 1634–5
- Yu Y, Chadee K. The 3′-untranslated region of human interleukin-8 mRNA suppresses IL-8 gene expression. Immunology 2001; 102: 498–505
- Winzen R, Kracht M, Ritter B, Wilhelm A, Chen CY, Shyu AB, et al. The p38 MAP kinase pathway signals for cytokine-induced mRNA stabilization via MAP kinase-activated protein kinase 2 and an AU-rich region-targeted mechanism. EMBO J 1999; 18: 4969–80
- Chaudhary LR, Avioli LV. Regulation of interleukin-8 gene expression by interleukin-1beta, osteotropic hormones, and protein kinase inhibitors in normal human bone marrow stromal cells. J Biol Chem 1996; 271: 16591–6
- Ma D, Forsythe P, Bienenstock J. Live Lactobacillus reuteri is essential for the inhibitory effect on tumor necrosis factor alpha-induced interleukin-8 expression. Infect Immun 2004; 72: 5308–14
- Targan SR, Hanauer SB, van Deventer SJ, Mayer L, Present DH, Braakman T, et al. A short-term study of chimeric monoclonal antibody cA2 to tumor necrosis factor alpha for Crohn's disease. Crohn's Disease cA2 Study Group. N Engl J Med 1997; 337: 1029–35
- Present DH, Rutgeerts P, Targan S, Hanauer SB, Mayer L, van Hogezand RA, et al. Infliximab for the treatment of fistulas in patients with Crohn's disease. N Engl J Med 1999; 340: 1398–405
- Rutgeerts P, Van Assche G, Vermeire S. Optimizing anti-TNF treatment in inflammatory bowel disease. Gastroenterology 2004; 126: 1593–610
- Aggarwal BB. Signalling pathways of the TNF superfamily: a double-edged sword. Nat Rev Immunol 2003; 3: 745–56
- Mukhopadhyay A, Suttles J, Stout RD, Aggarwal BB. Genetic deletion of the tumor necrosis factor receptor p60 or p80 abrogates ligand-mediated activation of nuclear factor-kappa B and of mitogen-activated protein kinases in macrophages. J Biol Chem 2001; 276: 31906–12
- Holtmann MH, Douni E, Schutz M, Zeller G, Mudter J, Lehr HA, et al. Tumor necrosis factor-receptor 2 is up-regulated on lamina propria T cells in Crohn's disease and promotes experimental colitis in vivo. Eur J Immunol 2002; 32: 3142–51
- Mizoguchi E, Mizoguchi A, Takedatsu H, Cario E, de Jong YP, Ooi CJ, et al. Role of tumor necrosis factor receptor 2 (TNFR2) in colonic epithelial hyperplasia and chronic intestinal inflammation in mice. Gastroenterology 2002; 122: 134–44
- Resta-Lenert S, Barrett KE. Probiotics and commensals reverse TNF-alpha- and IFN-gamma-induced dysfunction in human intestinal epithelial cells. Gastroenterology 2006; 130: 731–46
- Christensen HR, Frokiaer H, Pestka JJ. Lactobacilli differentially modulate expression of cytokines and maturation surface markers in murine dendritic cells. J Immunol 2002; 168: 171–8
- Borruel N, Carol M, Casellas F, Antolin M, de Lara F, Espin E, et al. Increased mucosal tumour necrosis factor alpha production in Crohn's disease can be downregulated ex vivo by probiotic bacteria. Gut 2002; 51: 659–64
- Borruel N, Casellas F, Antolin M, Llopis M, Carol M, Espiin E, et al. Effects of nonpathogenic bacteria on cytokine secretion by human intestinal mucosa. Am J Gastroenterol 2003; 98: 865–70
- Menard S, Candalh C, Bambou JC, Terpend K, Cerf-Bensussan N, Heyman M. Lactic acid bacteria secrete metabolites retaining anti-inflammatory properties after intestinal transport. Gut 2004; 53: 821–8
- Ewaschuk JB, Dieleman LA. Probiotics and prebiotics in chronic inflammatory bowel diseases. World J Gastroenterol 2006; 12: 5941–50
- Neish AS, Gewirtz AT, Zeng H, Young AN, Hobert ME, Karmali V, et al. Prokaryotic regulation of epithelial responses by inhibition of I((-( ubiquitination. Science 2000; 289: 1560–3
- Yan F, Cao H, Cover TL, Whitehead R, Washington MK, Polk DB. Soluble proteins produced by probiotic bacteria regulate intestinal epithelial cell survival and growth. Gastroenterology 2007; 132: 562–75
- Cinque B, Di Marzio L, Della Riccia DN, Bizzini F, Giuliani M, Fanini D, et al. Effect of Bifidobacterium infantis on interferon-gamma-induced keratinocyte apoptosis: a potential therapeutic approach to skin immune abnormalities. Int J Immunopathol Pharmacol 2006; 19: 775–86
- Johnson-Henry KC, Nadjafi M, Avitzur Y, Mitchell DJ, Ngan BY, Galindo-Mata E, et al. Amelioration of the effects of Citrobacter rodentium infection in mice by pretreatment with probiotics. J Infect Dis 2005; 191: 2106–17
- Hart AL, Lammers K, Brigidi P, Vitali B, Rizzello F, Gionchetti P, et al. Modulation of human dendritic cell phenotype and function by probiotic bacteria. Gut 2004; 53: 1602–9
- Foligne B, Zoumpopoulou G, Dewulf J, Ben Younes A, Chareyre F, Sirard JC, et al. A key role of dendritic cells in probiotic functionality. PLoS ONE ;:e 2007; 2: 313
- von der Weid T, Bulliard C, Schiffrin EJ. Induction by a lactic acid bacterium of a population of CD4(+) T cells with low proliferative capacity that produce transforming growth factor beta and interleukin-10. Clin Diagn Lab Immunol 2001; 8: 695–701
- McCarthy J, O'Mahony L, O'Callaghan L, Sheil B, Vaughan EE, Fitzsimons N, et al. Double blind, placebo controlled trial of two probiotic strains in interleukin 10 knockout mice and mechanistic link with cytokine balance. Gut 2003; 52: 975–80
- Chapat L, Chemin K, Dubois B, Bourdet-Sicard R, Kaiserlian D. Lactobacillus casei reduces CD8+ T cell-mediated skin inflammation. Eur J Immunol 2004; 34: 2520–8
- Di Giacinto C, Marinaro M, Sanchez M, Strober W, Boirivant M. Probiotics ameliorate recurrent Th1-mediated murine colitis by inducing IL-10 and IL-10-dependent TGF-beta-bearing regulatory cells. J Immunol 2005; 174: 3237–46
- Beutler B. TLR4: central component of the sole mammalian LPS sensor. Curr Opin Immunol 2000; 12: 20–6
- Akira S, Takeda K. Toll-like receptor signalling. Nat Rev Immunol 2004; 4: 499–511
- Madara J. Building an intestine – architectural contributions of commensal bacteria. N Engl J Med 2004; 351: 1685–6
- Rakoff-Nahoum S, Paglino J, Eslami-Varzaneh F, Edberg S, Medzhitov R. Recognition of commensal microflora by Toll-like receptors is required for intestinal homeostasis. Cell 2004; 118: 229–41
- Egan LJ, Eckmann L, Greten FR, Chae S, Li ZW, Myhre GM, et al. I((-kinase(-dependent NF-kappaB activation provides radioprotection to the intestinal epithelium. Proc Natl Acad Sci U S A 2004; 101: 2452–7
- Li Q, Cherayil BJ. Role of Toll-like receptor 4 in macrophage activation and tolerance during Salmonella enterica serovar Typhimurium infection. Infect Immun 2003; 71: 4873–82
- Kelly D, Campbell JI, King TP, Grant G, Jansson EA, Coutts AG, et al. Commensal anaerobic gut bacteria attenuate inflammation by regulating nuclear-cytoplasmic shuttling of PPAR-gamma and RelA. Nat Immunol 2004; 5: 104–12
- Tao Y, Drabik KA, Waypa TS, Musch MW, Alverdy JC, Schneewind O, et al. Soluble factors from Lactobacillus GG activate MAPKs and induce cytoprotective heat shock proteins in intestinal epithelial cells. Am J Physiol Cell Physiol 2006; 290: C1018–30
- Chen SL, Hung CS, Xu J, Reigstad CS, Magrini V, Sabo A, et al. Identification of genes subject to positive selection in uropathogenic strains of Escherichia coli: a comparative genomics approach. Proc Natl Acad Sci U S A 2006; 103: 5977–82
- Matsumoto S, Hara T, Hori T, Mitsuyama K, Nagaoka M, Tomiyasu N, et al. Probiotic Lactobacillus-induced improvement in murine chronic inflammatory bowel disease is associated with the down-regulation of pro-inflammatory cytokines in lamina propria mononuclear cells. Clin Exp Immunol 2005; 140: 417–26