Abstract
Liver fibrosis is the pathological process of chronic liver diseases induced by hepatic stellate cells. Proanthocyanidin A2 (PA2) has multiple pharmacological activities. In this study, we aimed to explore the effects of PA2 on hepatic stellate cell (HSC) activation in liver fibrosis. LX-2 cells were treated with TGF-β1 to establish a fibrosis cell model. Cell viability was evaluated using cell counting kit-8. The levels of fibrosis-related factors (collagen I, fibronectin, and α-SMA) were examined using quantitative real-time polymerase chain reaction, western blot, and immunofluorescence assay. The molecular mechanisms of PA2 were evaluated by RNA-seq, bioinformatic analysis, and western blot. The results showed that PA2 suppressed cell viability, and downregulated fibrosis-related factors induced by TGF-β1, suggesting PA2 suppressed the activation of HSCs. PA2 treatment-induced differentially expressed mRNAs are predicted to be associated with the PPAR-γ pathway. PA2 reversed the downregulation of PPAR-γ and the upregulation of phosphorylated (p)-Smad2 and Smad3. A rescue experiment illustrated that the inactivation of the PPAR-γ pathway reversed the effects of PA2 on cell viability and HSC activation. In conclusion, PA2 inhibited TGF-β1-induced activation of HSCs by activating the PPAR-γ/Smad pathway. The findings suggested that PA2 may be an effective treatment for liver fibrosis.
1. Introduction
Liver fibrosis is a pathological process of almost all chronic liver diseases [Citation1]. Early hepatic fibrosis is reversible, while late hepatic fibrosis is the main risk factor to cause liver cancer that threat to human health seriously [Citation2]. Hepatitis B and C virus infections are common causes of fibrosis, which can also be induced by alcohol, fatty liver disease, hepatitis, and cholestasis [Citation3]. Changing diet, strengthening exercise, and controlling metabolic syndrome can help slow the progression of fibrosis [Citation4]. However, there is no standard cure for advanced liver fibrosis. The most typical characteristic of liver fibrosis is the excessive accumulation of extracellular matrix (ECM). When the liver is damaged, hepatic stellate cells (HSCs) are activated to promote the secretion of ECM by liver myoblasts, leading to fibrous scarring [Citation5–7]. Thus, inhibiting the activation of HSCs and promoting ECM degradation may play an anti-fibrosis effect. However, the pathological role and mechanism of HSCs in liver fibrosis have not been fully elucidated.
Proanthocyanidin A2 (PA2) belongs to the tea polyphenol family and has a variety of biological activities, such as anti-virus, anti-inflammation, anti-oxidation, and anti-cancer [Citation8,Citation9]. Previous studies showed that PA2 plays a central role in several diseases, including liver disease, vascular proliferative diseases, and virus-infected diseases [Citation10–12]. PA2 can be isolated from flowers, seeds, and pericarp of litchi [Citation9,Citation13,Citation14]. However, the involvement of PA2 influences in the progression of liver fibrosis is unknown, Thus, we sought to evaluate the impacts of PA2 on the activation of HSCs in liver fibrosis.
Peroxisome proliferator-activated receptors (PPARs) contain three transcription factors: PPARalpha (PPARα), PPARbeta/delta (PPARβ/δ), and PPARgamma (PPARγ) [Citation15]. PPARs are involved in numerous human diseases [Citation16]. PPARα regulates energy homeostasis and contributes to Alzheimer’s Disease. PPARβ/δ plays an important role in cancers and neurodegenerative disorders. And all these PPARs participate in cardiovascular events. Activation of the PPAR-γ pathway is involved in inhibiting vascular calcification, neuroprotection, and attenuating liver fibrosis [Citation17–19]. It is related to HSCs activation, oxidative stress, fatty degeneration, and senescence [Citation19,Citation20]. Therefore, we considered that PA2 mediated the PPAR-γ pathway to regulate the activation of HSCs.
In addition, the transforming growth factor (TGF)-β1/Smad pathway is involved in liver fibrosis. When the liver is damaged, inflammatory factors including TGF-β1 are released to further activate HSCs in vitro and in vivo [Citation21]. Moreover, PPAR-γ has been reported to suppress the activation of the TGF-β1/Smad signalling pathway in HSCs, thereby attenuating fibrosis [Citation22]. Thus, the effects of PA2 on the regulation of the PPAR-γ/Smad pathway in HSCs needed to be explored.
In this study, based on the biological activities of PA2, we speculated that PA2 attenuated liver fibrosis via inactivating the HSCs by regulating the PPAR-γ pathway. The findings suggested that PA2 might be a novel drug for liver fibrosis treatment.
2. Materials and methods
2.1. Reagents
PA2 (; HPLC ≥ 98%) was purchased from Yuanye Bio-Technology (Shanghai, China). TGF-β1 was purchased from ProSpec (East Brunswick, USA). Cell counting kit-8 (CCK-8), and T0070907 (a PPAR-γ antagonist) were purchased from MedChemExpress (Monmouth Junction, USA). TRIzol, SuperScript IV VILO reverse transcription premix, PowerUp SYBR Green master mix, and Dynabeads mRNA purify kit were purchased from Invitrogen (Carlsbad, USA). Bicinchoninic Acid (BCA) protein assay kit, enhanced chemiluminescence (ECL) reagent and DAPI were purchased from Sigma-Aldrich (St. Louis, USA).
2.2. Cell culture
Hepatic stellate cells (LX-2) were purchased from Procell (Wuhan, China). The cells were cultured in DMEM supplemented with 10% FBS and 1% penicillin/streptomycin at 37 °C with 5% CO2.
2.3. Determination of cell survival rate
LX-2 cells were seeded into 96-well plates at 2000 cells/well concentration. Subsequently, the cells were treated with various concentrations of PA2 (0, 25, 50, 100, and 200 μM) with or without 5 ng/mL TGF-β1 for 24 h [Citation23]. To inhibit the PPAR-γ pathway, the LX-2 cells were treated with 5 μM T0070907 for 24 h. Then, cell viability was examined. CCK-8 solution (10 μL) was added to each well and incubated with the cells for 4 h. The absorbance value per well was detected at 450 nm.
2.4. Quantitative real-time polymerase chain reaction (qPCR)
Total RNA was extracted by the TRIzol reagent and 1 μg RNA was reverse transcribed to the first chain of cDNA using the SuperScript IV VILO reverse transcription premix. Subsequently, qPCR was conducted by the PowerUp SYBR Green master mix following the manufacturer’s protocol on LightCycler 480 real-time PCR system (Roche, Basel, Switzerland). GAPDH was applied as the internal control. mRNA expression was quantified using the 2-ΔΔct method. The primer sequences are shown as follows: collagen I forward: 5′-CTGGAAAGAATGGAGATGATGG-3′, reverse: 5′-CCAAACCACTGAAACCTCTG-3′; fibronectin 5′-CCATCGCAAACCGCTGCCAT-3′, reverse: 5′-AACACTTCTCAGCTATGGGCTT-3′; α-SMA forward: 5′-GAGAAGAGTTACGAGTTGCC-3′, reverse: 5′-ATGATGCTGTTGTAGGTGGT-3′; GAPDH forward: 5′-AAGAAGGTGGTGAAGCAGGC-3′, reverse: 5′-TCCACCACCCTGTTGCTGTA-3′.
2.5. Western blot
All antibodies used in western blot were purchased from Abcam (Cambridge, USA) including anti-collagen I (ab34710, 1:5000), anti-fibronectin (ab2413, 1:1000), anti-α-SMA (ab108531, 1:1000), anti-GAPDH (ab9485, 1:2500), anti-PPAR-γ (ab178860, 1:1000), anti-Smad2 (ab40855, 1:5000), anti-phosphorylation(p)-Smad2 (ab300079, 1:1000), anti-Smad3 (ab40854, 1:2000), anti-p-Smad3 (ab52903, 1:2000), and goat anti-rabbit IgG (ab6721, 1:3000).
For detecting protein levels, LX-2 cells were lysed in RIPA buffer. After measuring protein concentration using the BCA kit, cell lysates were run on the 10% SDS-PAGE and transferred to PVDF membranes. The membranes were incubated with specific primary antibodies at 4 °C overnight. Then, the membranes were incubated with a secondary antibody for 1 h at room temperature. The band signals were visualised using the ECL reagent.
2.6. Immunofluorescence assay
Cell slides were washed with PBS, fixed with 4% paraformaldehyde for 20 min, and transparent with 0.2% Triton X 100 for 10 min. After blocking using normal goat serum, the sliders were incubated with anti-fibronectin (ab268020, 1:50, Abcam) at 4 °C overnight and incubated with goat anti-rabbit IgG (ab150077, 1:500, Abcam) at 37 °C for 1.5 h. DAPI is used to analyse nuclear localisation. After the sliders were sealed, the results were observed under a confocal laser microscope (KEYENCE, Osaka, Japan).
2.7. RNA sequencing
LX-2 cells were treated with PA2 and distilled water (negative control). Total TNA was isolated from LX-2 cells. The purity of total RNA was measured by ultraviolet spectrophotometer (Thermo Fisher Scientific, Waltham, USA), and the integrity was evaluated by agarose gel electrophoresis. RNA was purified using the Dynabeads mRNA purify kit. Then, the cDNA library was constructed by reverse transcribing cDNA first and second chains. Finally, the sequencing was performed on an Illumina HiSeq 4000 according to the manufacturer’s protocol.
2.8. Bioinformatic analysis
The KEGG enrichment analysis of differentially expressed mRNAs and the related genes of the PPAR-γ pathway was predicted using the INPUT platform (http://cbcb.cdutcm.edu.cn/INPUT/Home/).
2.9. Statistical analysis
The data in this study are presented as the mean ± SD by analysis using the GraphPad Prism 7.0 software. Student’s t-test and one-way ANOVA were used for compared the differences between groups. p < 0.05 was the statistical significance.
3. Results
3.1. PA2 inhibits cell survival
To screen the concentrations of PA2, LX-2 cells were treated with 25, 50, 100, and 200 μM PA2, and cell survival was evaluated by CCK-8 assay. The results indicated that compared with the control group, PA2 did not affect cell survival at the concentrations of 25 and 50 μM, and significantly suppressed cell survival at 100 and 200 μM in a dose-dependent manner (). Thus, LX-2 cells treated with 50 μM PA2 were used in the following study.
3.2. PA2 inhibits TGF-β1-induced activation of HSCs
Subsequently, the effects of PA2 on HSC activation were investigated. Cell viability was markedly increased by TGF-β1 treatment, and PA2 inhibited cell viability (). The levels of fibrosis markers including collagen I, fibronectin, and α-SMA were elevated by TGF-β1, which were abrogated by PA2 (). In addition, the levels of fibronectin were also evaluated by IF staining assay and the results showed that TGF-β1 increased fibronectin levels, while PA2 reversed the increase ().
Figure 2. PA2 inhibits TGF-β1-induced HSC activation. (A) Cell viability of LX-2 cells after being treated with TGF-β1 and PA2. The expression of (B) collagen I, (C) fibronectin, and (D) α-SMA was measured using qPCR. (E) Western blot evaluated the collagen I, fibronectin, and α-SMA levels. (D) The fluorescence signal of fibronectin in LX-2 cells. n = 3. ***p < 0.001 and **p < 0.01 vs. the control group. ###p < 0.001 and ##p < 0.01 vs. the TGF-β1 group.
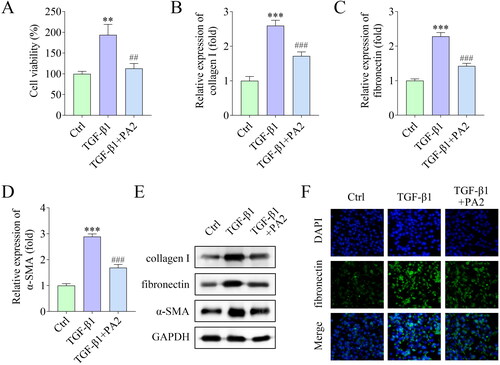
3.3. PA2 promotes the activation of the PPAR-γ signalling pathway
To explore the underlying mechanism, RNA-seq was performed in LX-2 cells treated with PA2 and distilled water. The results showed that there are multiple mRNAs are differentially expressed. The top ten upregulated mRNAs and downregulated mRNAs were shown in the heatmap (). The data illustrated that these differentially expressed mRNAs are related to the PPAR-γ signalling pathway, insulin resistance, fatty acid metabolism, and primary bile acid biosynthesis (). Moreover, the results of KEGG analysis indicated that the PPAR-γ signalling pathway is related to several mRNAs, including HMGCS1, FADS2, ACADM, SLC27A5, RXRG, APOA5, CPT1A, and FABP1 (). The protein levels of PPAR-γ were downregulated by TGF-β1 treatment and were reversed by PA2 (). The levels of PPAR-γ-regulated Smad pathway-related factors were also examined. TGF-β1 treatment increased the levels of p-Smad2, and p-Smad3, while PA2 abrogated the increase in their levels (). The levels of Smad2 and Smad3 were not regulated by TGF-β1 and PA2 ().
Figure 3. PA2 promotes the activation of the PPAR-γ signalling pathway. (A) the heatmap showed that the top ten upregulated and top ten downregulated mRNAs were analysed using RNA-seq. (B) the enrichment analysis of the Dysregulated mRNAs was analysed by the bioinformatic analysis. (C) The PPAR-γ signalling pathway-related factors were predicted using the bioinformatic analysis. (D) The protein levels of PPAR-γ, Smad2, p-Smad2, Smad3, and p-Smad3 were measured. n = 3.
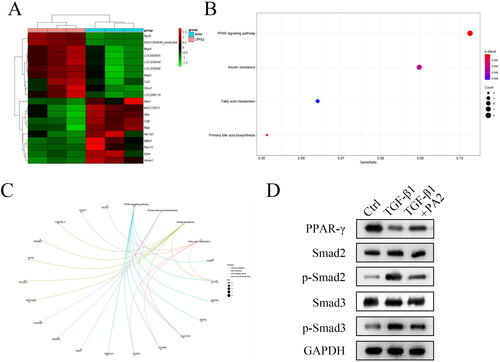
3.4. PA2 inhibits TGF-β1-induced HSC activation via the PPAR-γ pathway
Finally, a rescue experiment was performed. T0070907 was added to treat LX-2 cells to inactivate the PPAR-γ pathway. PA2 inhibited cell viability, which was promoted by T0070907 (). The mRNA and protein levels of collagen I, fibronectin, and α-SMA downregulated by PA2 were abrogated by T0070907 (). The fluorescence signal of fibronectin was decreased by PA2 and was elevated by T0070907 ().
Figure 4. PA2 inhibited TGF-β1-induced HSC activation via the PPAR-γ pathway. (A) Cell viability of LX-2 cells after being treated with TGF-β1, PA2, and T0070907. The expression of (B) collagen I, (C) fibronectin, and (D) α-SMA was measured using qPCR. (C) Western blot evaluated the collagen I, fibronectin, and α-SMA levels. (D) The levels of fibronectin were assessed by IF assay. n = 3. ***p < 0.001 vs. the control group. ###p < 0.001 and ##p < 0.01 vs. the TGF-β1 group. &&p < 0.01 and &p < 0.05 vs the TGF-β1 + PA2 group.
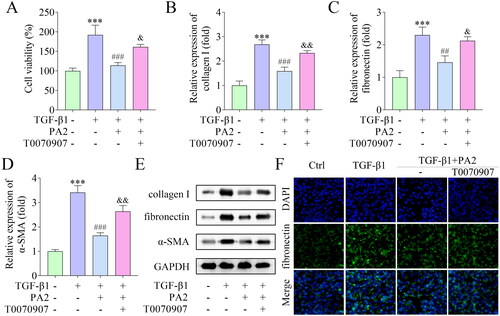
4. Discussion
Liver fibrosis is the main cause of death from chronic liver diseases. Effective and specific anti-fibrosis drugs have significant benefits for the treatment of many liver diseases [Citation24]. PA2 has a variety of biological activities, indicating its potential value in various diseases. For example, because of its anti-adhesion effect, PA2 isolated from cranberry inhibits the adhesion of Escherichia coli and Proteus mirabilis to urinary epithelial cells, thereby alleviating urinary tract infection [Citation25]. Additionally, PA2 suppresses IL-4-induced CCL26 production, leading to difficulty maintaining and progressing allergic asthma disease [Citation26]. Moreover, PA2 decreases proinflammatory cytokine levels and suppresses oxidative stress of LPS-treated Raw264.7 cells, protecting cells from inflammatory damage by regulating the NF-κB, MAPK, and Nrf2 signalling pathways [Citation27]. However, most research has focused on how to efficiently extract PA2 from a variety of plants [Citation28,Citation29], and there is still little research on PA2 in human diseases. At present, there is no relevant study on whether it can play a role in the progression of liver fibrosis. Litchi has a good effect on relieving symptoms of various diseases, such as chest tightness, flatulence, cough, neuralgia, etc. [Citation30]. Researchers are concerned about the presence of polyphenols in the peel, such as epicatechin and procyanidin [Citation30,Citation31]. PA2 is enriched in Litchi. Thus, we focused on the role of PA2 in liver fibrosis. In view of the important role of HSCs in the progression of hepatic fibrosis, we studied the effect of PA2 on HSC activation. Collagen I, fibronectin, and α-SMA are critical markers of activated HSCs, which levels are upregulated in activated HSCs in response to liver injury [Citation32]. In the present study, the results illustrated that PA2 inhibited cell viability and downregulated the levels of fibrosis markers induced by TGF-β1. The results suggest that PA2 inactivated the HSC activation and has an anti-liver fibrotic effect.
To explore the molecular mechanism by which PA2 acted. Through RNA-seq analysis, we found that PA2 induced differential expression of several genes. Among these genes, the top 10 up-regulated and the top 10 down-regulated genes can be significantly enriched into the PPAR-γ pathway. Thus, we sought to identify the role of the PPAR-γ signalling pathway in hepatic fibrosis. PPAR-γ pathway is associated with liver diseases, including fatty liver, hepatitis, and cholestatic liver diseases [Citation33,Citation34]. PPAR-γ pathway is involved in inflammation, lipid metabolism, and senescence in liver fibrosis [Citation19]. Multiple studies have shown that PPAR-γ is involved in the activation of HSCs. For instance, promoting the activation of the PPAR-γ pathway inhibits the activation and autophagy of HSCs, then attenuates CCl4-induced liver fibrosis [Citation31]. Methoxyeugenol activates PPAR-γ to inhibit HSCs activation, leading to the inhibition of cell proliferation, contractility loss, and downregulation of fibroblast markers [Citation20]. Additionally, PPAR-γ agonist inhibits the activation of HSCs, thereby attenuating liver fibrosis and inflammation [Citation35]. PPAR-γ plays a crucial role in fibrosis diseases of multiple organs by modulating the downstream pathways, such as MAPK, TGF-β, and NF-κB signalling pathways [Citation36]. The TGF-β/Smad pathway is involved in renal, hepatic, pulmonary and cardiac fibrosis. TGF-β1 directly activates downstream Smad signalling, including Smad2 and Smad3, inducing pro-fibrotic gene overexpression [Citation37]. Several studies have revealed that the TGF-β/Smad pathway is blocked when HSC activation is inhibited [Citation23, Citation38]. In this study, TGF-β1 decreased PPARγ levels and increased p-Smad2 and p-Smad3 levels, whereas PA2 reversed the effects of TGF-β1, suggesting that PA2 inactivating the PPAR-γ/Smad pathway. Moreover, inactivation of the PPAR-γ pathway abrogated the suppression of cell viability and fibrosis, suggesting that PA2 attenuated liver fibrosis by activating the PPAR-γ pathway.
In conclusion, the results of this study first showed that PA2 attenuated TGF-β1-induced activation of HSCs by activating the PPAR-γ/Smad signalling pathway. The findings provide solid theoretical support for the application of PA2 in the treatment of hepatic fibrosis. However, we only studied the PPAR-γ/Smad pathway as the underlying mechanism of PA2. Whether other members of PPARs are involved in HSC activation remains further studied.
Authors’ contributions
YX, XL, LW, MH and YL made substantial contributions to conception and design, or acquisition of data, or analysis and interpretation of data; YX was involved in drafting the manuscript and revising it critically for important intellectual content and given final approval of the version to be published.
Disclosure statement
No potential conflict of interest was reported by the author(s).
Data availability statement
The datasets used and/or analysed during the current study are available from the corresponding author upon reasonable request.
Additional information
Funding
References
- Parola M, Pinzani M. Liver fibrosis: pathophysiology, pathogenetic targets and clinical issues. Mol Aspects Med. 2019;65:1–7.
- Roehlen N, Crouchet E, Baumert TF. Liver fibrosis: mechanistic concepts and therapeutic perspectives. Cells. 2020;9(4):875.
- Altamirano-Barrera A, Barranco-Fragoso B, Méndez-Sánchez N. Management strategies for liver fibrosis. Ann Hepatol. 2017;16(1):48–56.
- Campos-Murguía A, Ruiz-Margáin A, González-Regueiro JA, et al. Clinical assessment and management of liver fibrosis in non-alcoholic fatty liver disease. World J Gastroenterol. 2020;26(39):5919–5943.
- Kisseleva T, Brenner D. Molecular and cellular mechanisms of liver fibrosis and its regression. Nat Rev Gastroenterol Hepatol. 2021;18(3):151–166.
- Higashi T, Friedman SL, Hoshida Y. Hepatic stellate cells as key target in liver fibrosis. Adv Drug Deliv Rev. 2017; 121:27–42.
- Hernandez-Gea V, Friedman SL. Pathogenesis of liver fibrosis. Annu Rev Pathol. 2011;6:425–456.
- Zhang M, Wu Q, Chen Y, et al. Inhibition of proanthocyanidin A2 on porcine reproductive and respiratory syndrome virus replication in vitro. PLoS One. 2018;13(2):e0193309.
- Yang DJ, Chang YZ, Chen YC, et al. Antioxidant effect and active components of litchi (Litchi chinensis sonn.) flower. Food Chem Toxicol. 2012;50(9):3056–3061.
- Lin JT, Chang YY, Chen YC, et al. Litchi (Litchi chinensis sonn.) flower proanthocyanidin fraction exhibited protective efficacy to suppress nickel-induced expression for vascular endothelial growth factor in HepG2 cells. J Food Biochem. 2019;43(7):e12882.
- Zhang L, Shao J, Zhou Y, et al. Inhibition of PDGF-BB-induced proliferation and migration in VSMCs by proanthocyanidin A2: involvement of KDR and jak-2/STAT-3/cPLA2 signaling pathways. Biomed Pharmacother. 2018;98:847–855.
- Gallina L, Dal Pozzo F, Galligioni V, et al. Inhibition of viral RNA synthesis in canine distemper virus infection by proanthocyanidin A2. Antiviral Res. 2011;92(3):447–452.
- Ma Q, Xie H, Li S, et al. Flavonoids from the pericarps of Litchi chinensis. J Agric Food Chem. 2014;62(5):1073–1078.
- Xu X, Xie H, Wang Y, et al. A-type proanthocyanidins from lychee seeds and their antioxidant and antiviral activities. J Agric Food Chem. 2010;58(22):11667–11672.
- Korbecki J, Bobiński R, Dutka M. Self-regulation of the inflammatory response by peroxisome proliferator-activated receptors. Inflamm Res. 2019;68(6):443–458.
- Wagner N, Wagner KD. The role of PPARs in disease. Cells. 2020;9(11):2367.
- Li H, Yang M. Ligustrazine activate the PPAR-γ pathway and play a protective role in vascular calcification. Vascular. 2022;30(6):1224–1231.
- Prashantha Kumar BR, Kumar AP, Jose JA, et al. Minutes of PPAR-γ agonism and neuroprotection. Neurochem Int. 2020;140:104814.
- Han X, Wu Y, Yang Q, et al. Peroxisome proliferator-activated receptors in the pathogenesis and therapies of liver fibrosis. Pharmacol Ther. 2021;222:107791.
- de Souza Basso B, Haute GV, Ortega-Ribera M, et al. Methoxyeugenol deactivates hepatic stellate cells and attenuates liver fibrosis and inflammation through a PPAR-ɣ and NF-kB mechanism. J Ethnopharmacol. 2021;280:114433.
- Yu Q, Cheng P, Wu J, et al. PPARγ/NF-κB and TGF-β1/smad pathway are involved in the anti-fibrotic effects of levo-tetrahydropalmatine on liver fibrosis. J Cell Mol Med. 2021;25(3):1645–1660.
- Zhao C, Chen W, Yang L, et al. PPARgamma agonists prevent TGFbeta1/Smad3-signaling in human hepatic stellate cells. Biochem Biophys Res Commun. 2006;350(2):385–391.
- Mu M, Zuo S, Wu RM, et al. Ferulic acid attenuates liver fibrosis and hepatic stellate cell activation via inhibition of TGF-β/smad signaling pathway. Drug Des Devel Ther. 2018;12:4107–4115.
- Schuppan D, Ashfaq-Khan M, Yang AT, et al. Liver fibrosis: direct antifibrotic agents and targeted therapies. Matrix Biol. 2018;68-69:435–451.
- Nicolosi D, Tempera G, Genovese C, et al. Anti-adhesion activity of A2-type proanthocyanidins (a cranberry major component) on uropathogenic E. coli and P. mirabilis strains. Antibiotics. 2014;3(2):143–154.
- Coleman SL, Kruger MC, Sawyer GM, et al. Procyanidin A2 modulates IL-4-Induced CCL26 production in human alveolar epithelial cells. Int J Mol Sci. 2016;17(11):1888.
- Wang QQ, Gao H, Yuan R, et al. Procyanidin A2, a polyphenolic compound, exerts anti-inflammatory and anti-oxidative activity in lipopolysaccharide-stimulated RAW264.7 cells. PLoS One. 2020;15(8):e0237017.
- Oldoni TL, Melo PS, Massarioli AP, et al. Bioassay-guided isolation of proanthocyanidins with antioxidant activity from peanut (Arachis hypogaea) skin by combination of chromatography techniques. Food Chem. 2016;192:306–312.
- Sintara M, Wang Y, Li L, et al. Quantification of cranberry proanthocyanidins by normal-phase high-performance liquid chromatography using relative response factors. Phytochem Anal. 2020;31(6):874–883.
- Chen Y, Li H, Zhang S, et al. Anti-myocardial ischemia effect and components of litchi pericarp extracts. Phytother Res. 2017;31(9):1384–1391.
- Sui Y, Zheng Y, Li X, et al. Characterization and preparation of oligomeric procyanidins from litchi chinensis pericarp. Fitoterapia. 2016;112:168–174.
- Tsuchida T, Friedman SL. Mechanisms of hepatic stellate cell activation. Nat Rev Gastroenterol Hepatol. 2017;14(7):397–411.
- Chyau CC, Wang HF, Zhang WJ, et al. Antrodan alleviates high-fat and high-fructose diet-induced fatty liver disease in C57BL/6 mice model via AMPK/Sirt1/SREBP-1c/PPARγ pathway. Int J Mol Sci. 2020;21(1):360.
- Kersten S, Stienstra R. The role and regulation of the peroxisome proliferator activated receptor alpha in human liver. Biochimie. 2017;136:75–84.
- Ni XX, Li XY, Wang Q, et al. Regulation of peroxisome proliferator-activated receptor-gamma activity affects the hepatic stellate cell activation and the progression of NASH via TGF-β1/smad signaling pathway. J Physiol Biochem. 2021;77(1):35–45.
- Li J, Guo C, Wu J. The agonists of peroxisome proliferator-activated receptor-γ for liver fibrosis. Drug Des Devel Ther. 2021;15:2619–2628.
- Hu HH, Chen DQ, Wang YN, et al. New insights into TGF-β/smad signaling in tissue fibrosis. Chem Biol Interact. 2018;292:76–83.
- Xiang D, Zou J, Zhu X, et al. Physalin D attenuates hepatic stellate cell activation and liver fibrosis by blocking TGF-β/smad and Yap signaling. Phytomedicine. 2020;78:153294.