Abstract
Retinal fibrosis was a key characteristic of diabetes retinopathy (DR). Apelin was found to be a candidate for tissue fibrosis. Nevertheless, the role of Apelin in the Müller cells in DR remains unclear. This study identified the function and mechanism of Apelin in Müller cells and the fibrosis of retinal tissue. Western blot was carried out to detect the Apelin, GFAP, Collagen I, α-SMA, JAK2 and STAT3 protein levels. Masson staining was performed to display the histopathological changes in retinal tissue of diabetic mellitus (DM) rats. The immunofluorescence staining was conducted to evaluate the Apelin levels in the retinal tissue. The levels of GFAP, Collagen I and α-SMA in the retinal tissue of DM rats was visualised by the immunohistochemistry staining. The results showed that Apelin, GFAP, Collagen I andα-SMA expression was prominently elevated in the retinal tissue of DM rats and high glucose (HG)-exposed Müller cells. The results of Masson staining showed that the epiretinal fibrotic membrane was observed in DM rats. Apelin knockdown declined the GFAP, Collagen I andα-SMA levels. Besides, the protein levels of p-JAK2 and p-STAT3 were elevated in the HG-treated Müller cells, while Apelin knockdown declined them. FLLL32 treatment neutralised the role of Apelin. In conclusion, Apelin facilitated the fibrogenic activity of Müller cells through activating the JAK2/STAT3 signalling pathway, and thus inducing the retinal fibrosis in DR.
Introduction
Diabetes retinopathy (DR) is a most common and serious complication of diabetes. It can easily cause visual impairment in the elderly [Citation1,Citation2]. At present, the treatment of DR includes retinal laser photocoagulation and vitrectomy, but these can only alleviate symptoms but not fundamentally prevent the progress of DR [Citation3]. Tissue fibrosis caused by abnormal proliferation of retina or vitreous often leads to serious visual impairment [Citation4]. Tissue and organ fibrosis is a chronic progressive pathological change. The main characteristic was extraordinary increase extracellular matrix deposition, resulting in scar formation and loss of function of affected organs or tissues [Citation5]. Müller cells are the most common type of glial cells that span the entire neural retinal layer and playing a vital role insuring the normal physiological activity of the retina [Citation6–8]. In recent years, it has been gradually recognised that the growth and development of Müller cells participate in the pathogenesis of DR. In the early stage of DR, the activated müller cells secrete various cytokines to regulate the immune and inflammatory response of retinal cells [Citation9,Citation10]. Additionally, müller cells are involved in protecting and supporting nerve cells. It is found that it can up-regulated VEGF expression and fibrosis factors in high glucose environment, so as to aggravate the lesions of DR [Citation11]. Therefore, the research focusing on retinal fibrosis of müller cells may provide new therapeutic strategies for DR in the future.
As a bioactive peptide, Apelin can combined with the orphan G protein-coupled receptor, angiotensin receptor AT-1-associated receptor protein (APJ), and exert autocrine, paracrine and circulating hormone functions [Citation12]. Its original precursor peptide consists of 77 amino acids, and there is a high degree of homology among various species. The decomposed peptide fragments are more common with Apelin-12, Apelin-13 and Apelin-36 [Citation13]. For the past few years, scholars have revealed that Apelin is associated with the secretion of some fibrogenic cytokines and participates in the pathological process of organ fibrosis [Citation14], but its mechanism is different for different tissues, which is still controversial [Citation15]. Apelin is considered to be an adverse factor to accelerate disease progression in the hepatobiliary system. Apelin is activated in the liver tissue of patients with hepatic fibrosis caused by biliary atresia, and the expression of Apelin is positively linked to the degree of hepatic fibrosis [Citation16]. However, in the cardiovascular system, Apelin is regarded as an anti-fibrosis polypeptide. Animal experiments showed that intraperitoneal injection of Pyr-apelin13 into rats with pulmonary hypertension could significantly reduce the secretion of pro-fibrotic factors and improve heart function [Citation17]. However, the regulation of Apelin on retinal fibrosis in diabetes is rarely reported.
Therefore, this study was carried out to investigate whether Apelin affected the fibrosis of Müller cells in DR progression. Moreover, we performed mechanism studies to show the regulatory role of Apelin on the activation of JAK2/STAT3 pathway.
Materials and methods
Animal study
The animal experimental protocol was approved by the Medical Ethics Committee of Eye Institute and Affiliated Xiamen Eye Centre of Xiamen University. For the diabetic mellitus (DM) model establishment, the rats were intraperitoneally injected with streptozotocin (55 mg/kg) for once and continuous fed with high-fat diet. After 72 h, the random fasting blood glucose ≥16.7 mmol/L was determined as successful modelling. The rats injected with the equal amount of solvent citric acid buffer (pH 4.5) and fed a normal diet were the control group. Then, the rats were fed continuously and randomly divided into control group (6 rats) and DM 4 weeks group (6 rats), DM 8 weeks group (6 rats), and DM 16 weeks group (6 rats). Additionally, after successful modelling, the rat was divided in to control group (6 rats), DM 16 weeks group (6 rats), DM 16 weeks + Lv-sh-NC group, and DM 16 weeks + Lv-sh-Apelin group. For Apelin knockdown, the rats were anaesthetised by intraperitoneal injection of chloral hydrate. A 30 G microsyringe was used to puncture the vitreous cavity along the 2 mm posterior of the corneal and scleral limbus, and 2 μL of empty vector lentiviral particles (Lv-sh-NC) and shRNA-Apelin lentiviral particles (Lv-sh-Apelin) were injected respectively. 12 weeks after lentiviral injection, the rats were all killed after intraperitoneal injection of excess 10% chloral hydrate. The retinas were separated from the eyeballs of rats. In addition, all rats received visual acuity and contrast sensitivity testing at 2, 4, 6 and 8 weeks.
Masson staining
The retinal tissues were fixed in 4% paraformaldehyde for 24 h, and the paraffin sections at the thick of 5 μm were made. Then paraffin sections were dewaxed, stained with iron haematoxylin for 10 min; differentiated with 1% hydrochloric acid alcohol, treated 0.6% ammonia back to blue, washed with running water; stained with Ponceau for 7 min, washed with distilled water; differentiated with phosphomolybdic acid for 3 min, stained with aniline blue for 5 min, dehydrated and sealed after differentiation with glacial acetic acid. Finally, the sections were observed under the microscope.
Immunofluoresence staining
Retinal tissue was made into 30 μm Frozen section. Then the sections were treated with Apelin antibody (dilution ratio 1:400) and incubated overnight at 4 °C. Next day, the sections were rewarmed at 37 °C for 1 h, and rinsed with TBST for 10 min. Then the secondary antibody (dilution ratio 1:600) was added to incubate with the sections at 37 °C for 1 h. After 10 min of TBST rinsing, the sections were sealed. Subsequently, the sections were stained with DAPI (10 μg/ml) for 5 min. At last, the fluorescence microscope (Olympus, Japan) was utilised to visualise the sections and the images was took.
Immunohistochemistry staining
Retinal sections were routinely dewaxed and rehydrated with gradient alcohol, and then transferred to PBS buffer for incubation for 5 min. Next, the sections were incubated with hydrogen peroxide for 10 min. After blocking in normal goat serum at 25 °C for 30 min, the sections were treated with the primary antibody at 4 °C overnight. After washing, the sections were treated with the secondary antibody at 25 °C for 20 min. After wash, the sections were added with fresh DAB working solution, and observed under the microscope. Afterwards, the sections were re-stained with haematoxylin for 2 min and sealed. Finally, sections were shown and photographed under a microscope (Leica, Germany).
Cell culture and treatment
The immortalised Müller cell line (MIO-M1) were presented by Professor Zhang xue of Ophthalmology, Southeast University. The MIO-M1 cells were cultured in DMEM (11965092, Invitrogen, CA, USA) containing 10% foetal bovine serum (30044333, Invitrogen) at 37 °C with 5% CO2. The medium was replaced every 3 days and the suspended cells were removed. After the cells adhered to 80% confluence, they were passaged at a ratio of 1:2. For high glucose (HG) treatment, the MIO-M1 cells were treated with 35 mmol/L glucose for 3h, 6h, and 12h. For the JAK2/STAT3 signalling pathway inhibition, 5 μM FLLL32 treated MIO-M1 cells for one day.
Cell transfection
For the Apelin knockdown, the small interfering RNA Apelin (si-Apelin 1# and 2#) and normal contorls (si-NC) was purchased (Genscript, Nanjing, China). For Apelin overexpression, the Apelin overrexpressing vector and empty vector was purchased (Genscript). These plasmids were transfected into the MIO-M1 cells with Lipofectamine 2000 kit (12566014, Invitrogen). All operations follow the instructions of the kit.
qRT-PCR
Total RNA of the cells was extracted by Trizol reagent according to the instructions (R0016, Beyotime, Shanghai, China). After detecting the purity and concentration, reverse transcription was carried out in line with the instructions of the reverse transcription kit (AU341-02-V2, TransGen Biotech, Beijing, China). A SYBR Green (AQ311-01, TransGen Biotech, Beijing, China) was used to amplify and quantify target genes. GAPDH was selected as the internal control. The primer sequences are shown below: Apelin, forward: 5′-CTCTGGACCGTGTTTCGGAG-3′, reverse: 5′-GGTACGTGTAGGTAGCCCACA-3′; Reaction procedure: pre denaturation at 95 °C for 30 s; PCR reaction was carried out at 95 °C for 15 s and 60 °C for 30 s for 40 cycles. The 2-ΔΔCT method was used to calculate the relative expression.
Western blot
The protein of renal tissue and cell of each group were extracted with RIPA (P0013B, Beyotime), and the concentration was determined by a BCA kit (P0010, Beyotime). 50 μg/well sample was added, and then electrophoresis, transmembrane, and blocking was carried out. After the membranes were incubated with primary antibodies (Apelin (1:1000, ab133624, Abcam, USA), GFAP (1:500, ab7260, Abcam), Collagen I (1:800, ab138492, Abcam), α-SMA (1:1200, ab240678, Abcam), p-JAK2 (1:600, ab32101, Abcam), JAK2 (1:2000, ab108596, Abcam), p-STAT3 (1:800, ab267373, Abcam), STAT3 (1:600, ab68153, Abcam) and β-Actin (1:4000, ab8226, Abcam)) overnight at 4 °C, the corresponding secondary antibody was incubated with the membranes at room temperature for 1 h. Finally, ECL luminescent solution (Beyotime) was added for the visualisation of the bands. The gray value of protein band were analysed by Image lab program. β-actin was the endogenous control. The ratio of the gray value of the target protein to β-actin violates the final result.
Statistical analysis
Spss 22.0 software was applied for the statistical analysis. The measurement data are shown as mean ± standard deviation. Multi group comparison was analysed by one way ANOVA, while pairwise comparison used the student-t test. The difference was statistically significant (p < 0.05).
Results
Apelin promoted the retinal fibrogenesis in the DM rats
The results of HE staining illustrated that the epiretinal fibrotic membrane was observed in the week 8 and week 16 DM rats, which was less enriched in the week 4 DM rats and control rats (). Besides, the immunofluorescence () and western blot () assays showed that Apelin levels were prominently increased in DM rats in a time dependent manner. Moreover, GFAP, Collagen I and α-SMA protein levels in the retinal tissues of the DM rats were prominently increased from 4 to 16 weeks (). Additionally, we found that the visual acuity () of the rats were significantly decreased and contrast sensitivity () was significantly increased in a time dependent manner.
Figure 1. Apelin promoted the retinal fibrogenesis in the DM rats.
(A)The HE staining of the retinal tissues of the DM rats. (B) The immunofluorescence staining of the retinal tissues of the DM rats. (C-H) The western blot results showed the protein levels of Apelin, GFAP, Collagen I and α-SMA were enhanced in the DM rats. The visual acuity (I) and contrast sensitivity (J) of the DW rats were measured. **P < 0.01, ***P < 0.001.
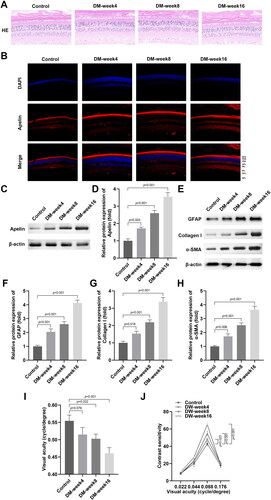
HG stimulation promoted the production of extracellular matrix markers in the MIO-M1 cells
After MIO-M1 cells were exposed to HG, the Apelin protein levels were prominently up-regulated in a time dependent manner (). Furthermore, the protein levels of GFAP, Collagen I and α-SMA were also dramatically up-regulated in a time dependent way in the HG stimulated MIO-M1 cells ().
Figure 2. HG stimulation promoted the production of extracellular matrix markers in the MIO-M1 cells.
(A-B) The western blot results showed that HG stimulation enhanced the protein levels of Apelin in the MIO-M1 cells. (C-F) The western blot results showed that HG stimulation enhanced the protein levels of GFAP, Collagen I and α-SMA in the MIO-M1 cells. **P < 0.01, ***P < 0.001.
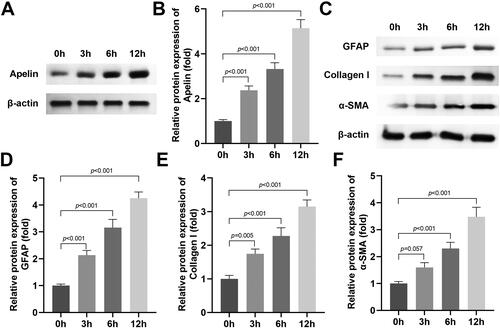
Apelin knockdown suppressed the production of extracellular matrix markers in the HG stimulated MIO-M1 cells
After si-Apelin 1# and 2# transfection, the Apelin mRNA and protein levels were prominently downregulated (), si-Apelin 1# was selected for the next experiment because of it’s better transfection efficiency. Subsequently, we found that Apelin knockdown dramatically decrased the protein levels of GFAP, Collagen I and α-SMA in the HG stimulated MIO-M1 cells ().
Figure 3. Apelin knockdown inhibited the production of extracellular matrix markers in the HG stimulated MIO-M1 cells.
(A-C) Si-Apelin 1# and 2# transfection declined the mRNA and protein levels of Apelin. (D-G) The western blot results showed that si-Apelin transfection declined the protein levels of GFAP, Collagen I and α-SMA in the HG stimulated MIO-M1 cells. ***P < 0.001, ##P < 0.01.
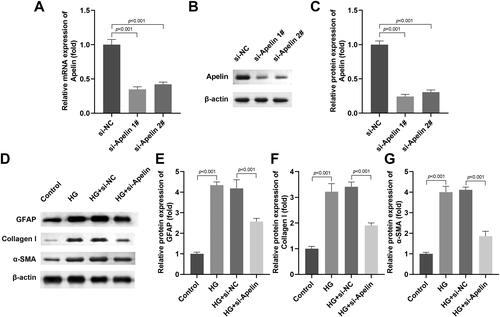
Apelin knockdown inhibited the production of extracellular matrix marker in vivo
After LV-sh-Apelin injection, the Apelin levels in the retinal tissue were prominently downregulated at mRNA and protein levels (). Then the HE staining indicated that Apelin knockdown relieved the production of epiretinal fibrotic membrane in the DM rats (). Besides, the results of immunofluorescence staining displayed that Apelin knockdown depleted the accumulation of Apelin in the retinal tissues of the DM rats () The immunohistochemistry staining showed that the upregulation of Collagen I levels in the DM group were decreased after Apelin knockdown (). The protein levels of GFAP, Collagen I and α-SMA in the retinal tissues of the DM rats were prominently decreased after Apelin knockdown (). Additionally, we found that the decrease of visual acuity () and the increase of contrast sensitivity () in the DW rats were significantly reversed after Apelin knockdown.
Figure 4. Apelin knockdown inhibited the production of extracellular matrix marker in vivo.
(A-C) LV-sh-Apelin declined the mRNA and protein levels of Apelin. (D) The HE staining of the retinal tissues of the DM rats. (E) The immunofluorescence staining of Apelin in retinal tissues of the DM rats. (F) The immunohistochemistry staining of Collagen I in retinal tissues of the DM rats. (G-J) The western blot results showed that the protein levels of GFAP, Collagen I and α-SMA in the retinal tissues of the DM rats were prominently decreased after Apelin knockdown. The visual acuity (K) and contrast sensitivity (L) of the DW rats were measured. ***P < 0.001, ##P < 0.01.
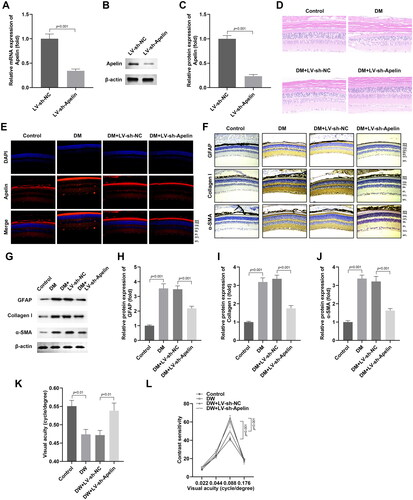
Apelin modulated the JAK2/STAT3 pathway in the HG stimulated MIO-M1 cells
Then, we explored the role of Apelin displayed in cell growth and development related signalling pathway (JAK2/STAT3, Wnt/β-catenin, and NFκB). We found that Apelin significantly inhibited the JAK2/STAT3 signalling pathway (), while showed no effects on the Wnt/β-catenin (), and NFκB () signalling pathway. Therefore, we selected JAK2/STAT3 pathway for the further investigation. As shown in , HG stimulation dramatically promoted the phosphorylation of JAK2 and STAT3 in the MIO-M1 cells, which indicated that JAK2/STAT3 signalling pathway was activated after HG stimulation. Moreover, Apelin knockdown reduced the levels of phosphorylated JAK2 and STAT3 in the HG stimulated MIO-M1 cells, which indicated the suppression of JAK2/STAT3 pathway.
Figure 5. Apelin modulated the JAK2/STAT3 signalling pathway in the HG stimulated MIO-M1 cells.
The effects of Apelin on the JAK2/STAT3 (A-C), Wnt/β-catenin (D-F), and NFκB (G-H) signalling pathway in the MIO-M1 cells were detected by western blot. (J-L) After si-Apelin transfection, the protein levels of p-JAK2 and p-STAT3 in the HG stimulated MIO-M1 cells were assessed with western blot. ***P < 0.001, ##P < 0.01.
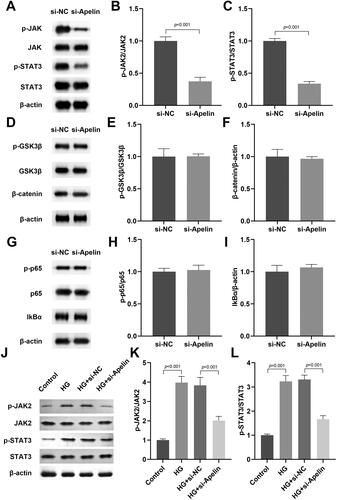
Activation of JAK2/STAT3 signalling pathway neutralised the role of si-Apelin in the HG stimulated MIO-M1 cells
After Apelin transfection, the Apelin mRNA and protein levels were prominently up-regulated (). Next, we confirmed that Apelin overexpression aggravated JAK2 and STAT3 phosphorylation in the HG stimulated MIO-M1 cells, while FLLL32 treatment prominently inhibited it (). In addition, Apelin overexpression aggravated the production of GFAP, Collagen I and α-SMA in the HG stimulated MIO-M1 cells, while FLLL32 treatment prominently declined them ().
Figure 6. Activation of JAK2/STAT3 signalling pathway neutralised the role of si-Apelin in the HG stimulated MIO-M1 cells.
(A-C) Apelin transfection enhanced the mRNA and protein levels of Apelin. After Apelin and FLLL32 treatment, (D-F) the protein levels of p-JAK2 and p-STAT3 in the HG stimulated MIO-M1 cells were assessed with western blot. (G-J) The protein levels of GFAP, Collagen I and α-SMA in the HG stimulated MIO-M1 cells were assessed with western blot. ***P < 0.001, ##P < 0.01, &P < 0.05.
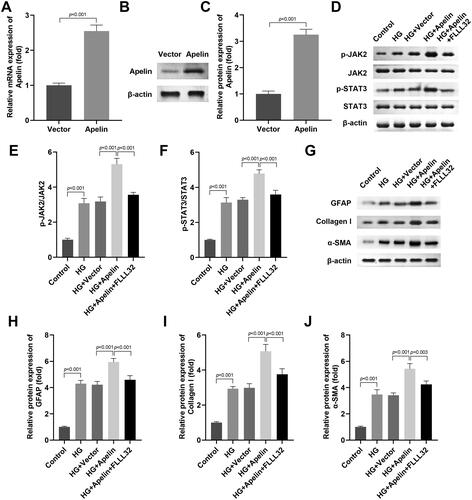
Discussion
Here, we confirmed that Apelin promoted the retinal fibrosis in DR via promoting the extracellular matrix markers production and fibrogenic activity in vivo and in vitro. Additionally, JAK2/STAT3 signalling pathway was activated for the participation of Apelin in the retinal fibrosis process.
It has been demonstrated that myofibroblasts lead to the excessive and uncontrolled production of extracellular matrix markers proteins in the epiretinal membrane in DR [Citation18,Citation19]. Müller cells were demonstrated to act as fibroblasts in the retina tissues [Citation20]. In healthy retinal, Müller cells exist in the inner layer of the retina and are in a stable state. However, in DR progression, Müller cells are stimulated with various growth factors and eventually lead to extracellular matrix markers deposition [Citation21]. As reporter by previous study, activated Müller cells promoted the production of many myofibroblasts markers markers, including GAFP, collagen I, and α-SMA [Citation22]. Similarly, our study also confirmed that the GAFP, collagen I, and α-SMA levels were prominently elevated in the HG stimulated Müller cells and DM rats.
In 1998, Tatemoto et al. [Citation23] got the endogenous ligand Apelin of APJ from the secretion of bovine stomach by reverse pharmacology. Apelin can be found in various tissues and organs of human, including adipose tissue, kidney, lung, liver, cardiovascular system and central nervous system [Citation12,Citation24]. It has various biological effects, including reducing blood pressure, enhancing myocardial contractility, regulating the release of hormones from the hypothalamus and pituitary, promoting drainage and regulating feeding [Citation25,Citation26]. Since its discovery, Apelin has been the focus of attention of scholars from all over the world. Recently, growing evidence has reported that Apelin is interrelated with some fibrogenic cytokines and may be involved in the occurrence of organ fibrosis [Citation27,Citation28], but its mechanism of action is also different for different organs, and there are still many disputes. For example, Lv et al. [Citation29] found that Apelin suppressed the Ang II induced atrial fibrosis, indicating that Apelin was a promising therapeutic target for atrial fibrosis. Apelin promoted liver fibrosis according to the in vivo and in vitro assays, and the mechanism may be related to the apelin level of pro-fibrosis genes through ERK signalling pathway. However, on the contrary, Wang et al. [Citation28] confirmed that high levels of Apelin enhanced the expressions of α-SMA as well as cyclinD1 in the liver, which induced the liver fibrosis. Similarly, our study showed that Apelin expression was high in the HG stimulated Müller cells and DM rats. Apelin knockdown effectively inhibited the retinal fibrosis progression in the DM rat and decreased the GAFP, collagen I, and α-SMA levels in vivo and in vitro.
In recent years, tyrosine kinase-2/signal transducers and activators of transcription-3 (JAK2/STAT3) signalling pathway was the key regulator in modulating the formation of fibrosis in different tissues and organs. In the experimental study on pulmonary fibrosis, it was found that the activation of JAK2/STAT3 signalling pathway regulates the mesenchymal transformation of pulmonary fibrosis model in vitro [Citation30]. In the study of the mechanism related to inhibiting liver fibrosis, it is found that Panax notoginseng saponins can reduce liver fibrosis by suppressing the JAK2/STAT3 signalling pathway, which may be one of the mechanisms of inhibiting liver fibrosis [Citation31]. Zhang et al. [Citation32] found that inactivating of JAK2/STAT3 signalling pathway can prevent fibroblast collagen release and myofibroblast differentiation. Therefore, we hypothesised that JAK2/STAT3 pathway might also be involved in the progression of retinal fibrosis. The results confirmed that HG stimulation activated the JAK2/STAT3 signalling pathway in the Müller cells. Apelin knockdown blocked the activation of JAK2/STAT3 signalling pathway. Interestingly, FLLL32 treatment, a JAK2 inhibitor, suppressed the JAK2/STAT3 signalling pathway activation and neutralised the role of Apelin in the HG stimulated Müller cells.
In conclusion, our findings clarified that Apelin accelerated DR-induced retinal fibrosis via facilitating the fibrogenic activity of Müller cells through activating the JAK2/STAT3 signalling pathway. These findings might provide new direction for the treatment of DR.
Authors’ contributions
All authors participated in the design, interpretation of the studies and analysis of the data and review of the manuscript. Y L drafted the work and revised it critically for important intellectual content; Q H and B W were responsible for the acquisition, analysis, or interpretation of data for the work
Ethics approval and consent to participate
The animal experimental protocol was approved by the Medical Ethics Committee of Eye Institute and Affiliated Xiamen Eye Centre of Xiamen University.
Acknowledgments
Not applicable.
Availability of data and materials
All data included in this study are available upon request by contact with the corresponding author.
Disclosure statement
All authors have completed the ICMJE uniform disclosure form. The authors have no conflicts of interest to declare.
Additional information
Funding
References
- Singh PK, Tandon R. Correlation study of apolipoprotein’a’ with diabetic retinopathy in patients of type 2 diabetes mellitus with retinopathy. J Assoc Physicians India. 2020;68:1.
- Yin L, Zhang D, Ren Q, et al. Prevalence and risk factors of diabetic retinopathy in diabetic patients: a community based cross-sectional study. Medicine (Baltimore). 2020;99(9):e19236.
- Alasil T, Waheed NK. Pan retinal photocoagulation for proliferative diabetic retinopathy: pattern scan laser versus argon laser. Curr Opin Ophthalmol. 2014;25(3):164–10.
- Kempegowda P, Sunsoa H, Chandan JS, et al. Retinopathy and microalbuminuria are common microvascular complications in cystic fibrosis-related diabetes. Ther Adv Endocrinol. 2020;11:1905080292.
- Parola M, Pinzani M. Pathophysiology of organ and tissue fibrosis. Mol Aspects Med. 2019;65:1.
- Coughlin BA, Feenstra DJ, Mohr S. Muller cells and diabetic retinopathy. Vision Res. 2017;139:93–100.
- Goldman D. Müller glial cell reprogramming and retina regeneration. Nat Rev Neurosci. 2014;15(7):431–442.
- Eastlake K, Lamb W, Luis J, et al. Prospects for the application of Muller glia and their derivatives in retinal regenerative therapies. Prog Retin Eye Res. 2021;85:100970.
- Fan J, Shen W, Lee SR, et al. Targeting the Notch and TGF-beta signaling pathways to prevent retinal fibrosis in vitro and in vivo. Theranostics. 2020;10(18):7956–7973.
- Lei X, Zhang J, Shen J, et al. EPO attenuates inflammatory cytokines by muller cells in diabetic retinopathy. Frontiers Biosci. 2011;3:201. (Elite edition)
- Rezzola S, Guerra J, Krishna CA, et al. VEGF-Independent activation of muller cells by the vitreous from proliferative diabetic retinopathy patients. Int J Mol Sci. 2021;22(4):2179.
- Antushevich H, Wójcik M. Review: apelin in disease. Clin Chim Acta. 2018;483:241–248.
- Castan-Laurell I, Dray C, Valet P. The therapeutic potentials of apelin in obesity-associated diseases. Mol Cell Endocrinol. 2021;529:111278.
- Chen L, Zhou T, White T, et al. The Apelin-Apelin receptor axis triggers cholangiocyte proliferation and liver fibrosis during mouse models of cholestasis. Hepatology. 2021;73(6):2411–2428.
- Yan J, Wang A, Cao J, et al. Apelin/APJ system: an emerging therapeutic target for respiratory diseases. Cell Mol Life Sci. 2020;77(15):2919–2930.
- Chen W, Oue T, Ueno T, et al. Apelin is a marker of the progression of liver fibrosis and portal hypertension in patients with biliary atresia. Pediatr Surg Int. 2013;29(1):79–85.
- Falcao-Pires I, Goncalves N, Henriques-Coelho T, et al. Apelin decreases myocardial injury and improves right ventricular function in monocrotaline-induced pulmonary hypertension. Am J Physiol Heart Circ Physiol. 2009;296(6):H2007–H2014.
- Yang W, Zhang S, Zhu J, et al. Gut microbe-derived metabolite trimethylamine N-oxide accelerates fibroblast-myofibroblast differentiation and induces cardiac fibrosis. J Mol Cell Cardiol. 2019;134:119–130.
- Kimura T, Monslow J, Klampatsa A, et al. Loss of cells expressing fibroblast activation protein has variable effects in models of TGF-β and chronic bleomycin-induced fibrosis. Am J Physiol Lung Cell Mol Physiol. 2019;317(2):L271–L282.
- Devoldere J, Peynshaert K, De Smedt SC, et al. Müller cells as a target for retinal therapy. Drug Discov Today. 2019;24(8):1483–1498.
- Roy S, Amin S, Roy S. Retinal fibrosis in diabetic retinopathy. Exp Eye Res. 2016;142:71–75.
- Graca AB, Hippert C, Pearson RA. Muller glia reactivity and development of gliosis in response to pathological conditions. Adv Exp Med Biol. 2018;1074:303–308.
- Habata Y, Fujii R, Hosoya M, et al. Apelin, the natural ligand of the orphan receptor APJ, is abundantly secreted in the colostrum. Biochim Biophys Acta. 1999;1452(1):25–35.
- Liu Q, Jiang J, Shi Y, et al. Apelin/apelin receptor: a new therapeutic target in polycystic ovary syndrome. Life Sci. 2020;260:118310.
- Zhou JX, Shuai NN, Wang B, et al. Neuroprotective gain of apelin/APJ system. NEUROPEPTIDES. 2021;87:102131.
- Cheng J, Luo X, Huang Z, et al. Apelin/APJ system: a potential therapeutic target for endothelial dysfunction-related diseases. J Cell Physiol. 2019;234(8):12149–12160.
- Huang S, Chen L, Lu L, et al. The apelin–APJ axis: a novel potential therapeutic target for organ fibrosis. Clin Chim Acta. 2016;456:81–88.
- Wang Y, Song J, Bian H, et al. Apelin promotes hepatic fibrosis through ERK signaling in LX-2 cells. Mol Cell Biochem. 2019;460(1-2):205–215.
- Lv W, Zhang L, Cheng X, et al. Apelin inhibits angiotensin II-Induced atrial fibrosis and atrial fibrillation via TGF-β1/Smad2/α-SMA pathway. Front Physiol. 2020;11:583570.
- Qin Y, Zhao P, Chen Y, et al. Lipopolysaccharide induces epithelial–mesenchymal transition of alveolar epithelial cells cocultured with macrophages possibly via the JAK2/STAT3 signaling pathway. Hum Exp Toxicol. 2020;39(2):224–234.
- Hui J, Gao J, Wang Y, et al. Panax notoginseng saponins ameliorates experimental hepatic fibrosis and hepatic stellate cell proliferation by inhibiting the Jak2/Stat3 pathways. J Tradit Chin Med, 2 2016;36:217–224
- Zhang Y, Dees C, Beyer C, et al. Inhibition of casein kinase II reduces TGFβ induced fibroblast activation and ameliorates experimental fibrosis. Ann Rheum Dis. 2015;74(5):936–943.