Abstract
Protein posttranslational modifications (PTMs) arise in a number of normal cellular biological pathways and in response to pathology caused by inflammation and/or infection. Indeed, a number of PTMs have been identified and linked to specific autoimmune responses and metabolic pathways. One particular PTM, termed isoaspartyl (isoAsp or isoD) modification, is among the most common spontaneous PTM occurring at physiological pH and temperature. Herein, we demonstrate that isoAsp modifications arise within the ZAP70 protein tyrosine kinase upon T-cell antigen receptor (TCR) engagement. The enzyme protein L-isoaspartate O-methyltransferase (PCMT1, or PIMT, EC 2.1.1.77) evolved to repair isoaspartyl modifications in cells. In this regard, we observe that increased levels of isoAsp modification that arise under oxidative stress are correlated with reduced PIMT activity in patients with systemic lupus erythematosus (SLE). PIMT deficiency leads to T cell hyper-proliferation and hyper-phosphorylation through ZAP70 signaling. We demonstrate that inducing the overexpression of PIMT can correct the hyper-responsive phenotype in lupus T cells. Our studies reveal a phenotypic role of isoAsp modification and phosphorylation of ZAP70 in lupus T cell autoimmunity and provide a potential therapeutic target through the repair of isoAsp modification.
Introduction
Protein posttranslational modifications (PTMs) potentially regulate important biological functions of the modified proteins, such as protein maturation and stability, catalytic activity of the enzymes, cellular metabolism, and extracellular communications. As an inherent response to physiological changes, PTMs also allow immune cells to respond rapidly to both foreign and self-antigens. One notable example is within the signaling cascade, which is generally achieved by a series of tyrosine and serine/threonine protein kinases to phosphorylate signaling proteins in immune cells. For example, ERK and MEK are activated by phosphorylation in the Toll-like receptor (TLR) pathway triggered by bacteria or virus and in the T cell receptor (TCR) pathway upon the presentation of the foreign antigen peptide [Citation1–3]. T cell signaling abnormalities have been extensively investigated in many autoimmune diseases including systemic lupus erythematosus (SLE), multiple sclerosis (MS), and rheumatoid arthritis (RA) [Citation4–7]. Impaired ERK pathway signaling in lupus CD4 T cell subsets contributes to the disease pathogenesis of SLE [Citation8]. Mutation of phosphorylation sites of tyrosine 315 and 319 in ZAP70 is associated with autoimmune arthritis [Citation9].
In addition to phosphorylation, methylation is also linked to the onset and development of autoimmune diseases such as SLE and MS [Citation10]. Failure to maintain the methylation status of CpG dinucleotide triggers T cell autoreactivity and may contribute to the pathogenesis of SLE [Citation11,Citation12]. Other PTMs including symmetric dimethylated snRNPs, modified by protein arginine methyltransferase (PRMT), are recognized by anti-Sm autoantibodies in human lupus [Citation13,Citation14]. Of interest, evidence demonstrated that the PRMT family of enzymes serves as a key regulator in T cell activation [Citation15,Citation16]. For example, vav guanine nucleotide exchange factor 1 (Vav1) is a critical TCR signaling protein which requires arginine methylation by PRMT for further nuclear localization and IL-2 induction in response to CD28 costimulation. Moreover, transmethylation inhibitor, methyl 4-(adenin-9-yl)-2-hydroxybutanoate (DZ2002), was found to abolish Vav1 methylation in CD4 T cells and ameliorate autoimmune disease development in both experimental autoimmune encephalomyelitis (EAE) and MRL-Faslpr mouse models [Citation17]. Thus, it is important to study the PTMs and potential repair pathways that modulate T-cell responses as new therapeutic approaches that may alter the course of autoimmunity.
Isoaspartyl (isoAsp) modification is derived from aspartate isomerization or asparagine deamidation and is one of the most common spontaneous, nonenzymatic PTMs occurring in the physiological pH and temperature [Citation18]. IsoAsp modification of protein alters its structure and/or the biological functions associated with T cell immune responses [Citation19,Citation20]. Early studies demonstrated that isoAsp modification of asparagine 52 in the CD4 molecule reduced the binding affinity for HIV-1 gp120 [Citation21]. Notably, signaling of LFA-1 (a family of leukocyte integrins) through ZAP70 is critical for normal T cell functions [Citation22,Citation23], and isoAsp modification can cause switching of such integrin-ligand recognition [Citation24]. The isoAsp at residue 317 of the IL-1 receptor type II lost IL-1β binding capacity due to the conformational change introduced by isoAsp residue [Citation25].
Our earlier studies demonstrated that isoAsp levels accumulated systemically over age in lupus-prone MRL mice, including kidney and brain, the major target organs of autoimmune pathology in SLE [Citation26]. To keep the structural and functional integrity of proteins, the enzyme protein L-isoaspartate O-methyltransferase (PCMT1, or PIMT, EC 2.1.1.77) is the only known isoAsp repair enzyme present in eukaryotic cells, bacteria and plant cells [Citation27]. Increased isoAsp modification due to deficiency of PIMT leads to aberrant T cell hyperproliferation and autoantibody production, which are key phenotypes in human SLE [Citation28]. However, the precise molecular mechanism of isoAsp modification and the isoAsp repair enzyme, PIMT, in lupus T cell autoimmunity has yet to be defined.
In this work, we report evidence that isoAsp modification in erythrocytes under oxidative stress is higher in SLE patients compared to healthy donors/subjects. Moreover, the increased isoAsp modification is correlated to reduced PIMT enzyme activity, i.e. isoAsp repair efficiency. Overexpression of PIMT suppresses lupus T cell hyperproliferation mediated by the isoAsp-modified ZAP70 TCR signaling pathway. We found that Asp 92, Asp 290, Asp 303, and Asp 394 in ZAP70 are highly susceptible to isoAsp modification. Moreover, ZAP70 is abnormally isoAsp modified upon TCR engagement in PIMT-deficient T cells compared to wild-type T cells. In sum, our results reveal how isoAsp modification and PIMT repair processes influence T cell responses and suggest a novel therapeutic target, isoAsp-modified ZAP70, for the treatment of T cell-mediated disorders.
Methods
Mice
B10.BR, MRL/Mp (MRL+/+), and MRL/Mp-Faslpr (MRL/lpr) mice were obtained from The Jackson Laboratory (Bar Harbor, ME). AND transgenic mice expressing an α/β TCR (Vα11+, Vβ3+), which recognizes the amino acids 88-104 of pigeon cytochrome C (PCC 88-104), were maintained and screened as described previously [Citation26]. PIMT−/− mice were obtained by intercrossing PIMT+/− C57BL/6 mice and screened for PCR genotyping with the presence of the neo cassette and the absence of the PIMT gene from tail DNA, as described previously [Citation28]. All animals were bred and maintained in specific pathogen-free facilities at the Yale Animal Resources Center.
Patients and sample processing
Human peripheral blood was drawn from healthy (n = 28; 20 women, median age 41 years, range 26–56 years) and patients with SLE adults (n = 20; 20 women, median age 37.5 years, range 24–63 years). All SLE-affected individuals met the 1997 revised ACR criteria for diagnosis of SLE. The study was approved by the institutional review committees (IRB) of Yale University. The IRB-approved informed consent was obtained from all study subjects before blood sampling.
For oxidative treatment of human erythrocytes, heparinized human blood was centrifuged at 3,400 rpm for 10 min to remove the plasma and buffy coat and followed by washing the packed erythrocytes at least three times with isotonic saline (0.9% NaCl). Then, the packed erythrocytes were incubated with 500 µM t-butyl hydroperoxide (t-BHP) in 10% final hematocrit in a shaking incubator at 37 °C for 18 h. Oxidized erythrocytes were washed seven times with the same isotonic saline to remove t-BHP [Citation29].
Plasmid construction, recombinant retrovirus preparation, and T cell infection
For PIMT-MSCV-I-Thy1.1 construct, mouse PIMT gene was amplified by PCR from Amplified Mouse Brain cDNA library (Open biosystems) using primers (forward: 5′-GTGGTAGATCTTCTCACTCTTGGGGAAACTGCTAG-3′ and reverse: 5′-GGTGTCGACTGGTCAAGCTCACGTTGCTTTCA-3′), which includes Bgl II and Sal I sites. The amplified PCR product was confirmed by DNA sequencing and then cloned into MSCV-I-Thy1.1 retroviral vector. Retroviral transduction into murine T cells is described elsewhere [Citation30]. In brief, retroviral stock was prepared by cotransfection of MSCV-I-Thy1.1 (empty vector) or PIMT-MSCV-I-Thy1.1 construct and pEco retroviral packaging vector into 293T cells using LipofectAMINE 2000 (Invitrogen). Seventy-two hours post-transfection, virus-containing supernatant was harvested and filter sterilized. Murine primary CD4 T cells were purified by negative selection (StemCell Technologies) from spleen and lymph node and stimulated by Dynabeads Mouse T-activator CD3/28 (Invitrogen) in the presence of recombinant mouse IL-2 (R&D systems). After 24 hours, T cells were infected with the above virus stock by spinoculation in the presence of polybrene and rIL-2. Infected T cells were further expanded by restimulation with CD3/28 Dynabeads or pigeon cytochrome c 88-104 in the presence of mitomycin C-treated CH 27 B lymphoblastoid cells as antigen-presenting cells.
For characterization of retrotransduced PIMT enzyme in primary murine CD4 T cells, RT-PCR and immunoblot were performed in Thy1.1+CD4+ T cells after 5 days of retroviral transduction. RT-PCR primers for mouse PIMT and GAPDH are forward: 5′-GCCTCATATGCACGCATATGCACTAG-3′ and reverse: 5′-TCAGCGTATCCCATTCTTCC-3′ and forward: 5′-AAACCCATCACCATCTTCCA-3′ and reverse: 5′-GTGGTTCACACCCATCACAA-3′, respectively. The expected amplicon length of RT-PCR for mouse PIMT and GAPDH are 269bp and 198bp, respectively.
Adoptive transfer of retrotransduced PIMT-T cells into (MRLxB10) mPCC transgenic F1 recipient mice
The pigeon cytochrome C (PCC)-specific TCR-transgenic T cells were isolated from MRL.AND and B10.AND mice as donor cells. After retrovirus infection as described above, Thy1.1+-CD4 infected T cells were sorted by FACS for adoptive transfer. We used (MRLxB10) F1 mice with or without expression of PCC as a membrane Ag (mPCC-transgenic F1 or nontransgenic F1, respectively; generous gift of Dr. Joe Craft, Yale University) as the recipient mice [Citation31]. The recipient mice were i.p. injected with 3% thioglycollate to elicit antigen-presenting macrophages for 24 hours and then 5 × 106 CD4 T donor cells adoptive transferred via i.p. At different time points, cells from the peritoneal cavity, spleen and lymph nodes were harvested, stained with CD4-APC and analyzed by flow cytometry for the T cell functions described below.
T cell proliferation assay
For mouse samples, pooled axillary, inguinal, brachial and popliteal lymph nodes, and spleens from age-matched female mice were used for purification of T cells by negative selection using MHC class II and CD11b microbeads in the MACS system (Miltenyi Biotec, Auburn, CA). The resulting T cell populations were > 95% pure as determined by flow cytometry. The purified 4 x 105 CD4+ T cells were cultured with coated mouse anti-CD3 mAb (5µg/ml) and anti-CD28 mAb (1µg/ml) for 48 hours. For human samples, 1 x 105 PBMC cells were cultured with coated human anti-CD3 mAb (0.54µg/ml) for 48 hours. Then, cultures were pulsed with [3H] thymidine (ICN Chemicals, Irvine, CA) for 18 hours before being harvested onto filters with a semiautomatic cell harvester. Radioactivity was counted with a Betaplate liquid scintillation counter (Wallac, Gaithersburg, MD). Besides the thymidine incorporation assay, mouse CD4+ T cell proliferation was also examined by CellTraceTM CFSE cell proliferation kit (C34554, Molecular Probes) according to the manufacturer’s protocol.
Intracellular phospho-protein analysis by flow cytometry
Intracellular phosphoprotein staining is described elsewhere [Citation32]. In brief, cells need to be fixed, permeabilized before specific phospho-protein staining. For phospho-CREB (S133) and phospho-ZAP70 (Y319), cells were fixed with 2% paraformaldehyde (PFA) at 37 °C for 10 min, on ice for 1 min and centrifuge at 1200 rpm for 5 min, then permeabilized by adding ice-cold methanol to a final concentration of 90% on ice for 30 min. For phospho-MEK1/2 (S222) and phospho-ERK1/2 (T202/Y204), cells were fixed with CytoFix fixation buffer (BD554655) at 37 °C for 10 min and centrifuged at 1200 rpm for 5 min, then permeabilized with Perm Buffer III (BD558052) on ice for 30 min. After fixation and permeabilization, cells were washed with staining buffer (PBS with 0.5% BSA) and the following antibodies were used for staining, APC-conjugated anti-CD4 (RM4-5, BD553051), PE conjugated anti-CD90.1 (OX-7, BD554898) or FITC conjugated anti-CD90.1 (OX-7, BD554897), AF488 conjugated anti-phospho-CREB (Cell Signaling, 87G3, #9187), PE conjugated anti-phospho-ZAP70 (17A/P-ZAP70, BD557881), AF488 conjugated anti-phospho-ERK1/2 (20A, BD612592), anti-phospho-MEK1/2 (BD612566) and PE conjugated F(ab’) anti-rabbit IgG (BD558416). Stained cells were analyzed by FACSCalibur (BD Biosciences) with FlowJo software (Tree Star).
IsoAsp modification vapor assay and PIMT-specific activity assay
To measure isoAsp modification in membrane proteins, oxidized erythrocytes were hemolyzed in hypotonic buffer (5mM sodium phosphate, pH8.0, 25µM PMSF and 1mM EDTA). After centrifugation at 15,000 rpm for 20 min at 4 °C, the cytosolic supernatant was assayed for PIMT enzyme activity and the membrane pellet (after washing twice with same hypotonic buffer) was assayed for the content of isoAsp residues. For the isoAsp vapor assay, 1-2 mg of erythrocytes membrane protein or 1.5 µg of recombinant human ZAP70 protein (Sigma, Z2126) was incubated in reaction buffer (1mM EGTA, 0.004% sodium azide, 0.16% Triton X-100 and 0.1M sodium phosphate, pH6.8) containing with 8 µg recombinant human PIMT (hPIMTII-pDM2X-his) [Citation33,Citation34], 1µCi of S-adenosyl-L- [methyl-3H] methionine (3H-SAM; 0.55mCi/ml; Perkin Elmer), 20µM of cold SAM in a 50-µl final volume at 37 °C for 30min. Fifty µl of STOP solution (0.4M CAPS, pH10, 5% SDS, 2.2% methanol, 0.1% m-cresol purple) was added to stop the reaction, and then 50 µl of the mixture was immediately spotted 50 µl on the filter paper stuck in a microcentrifuge tube within a sealed vial containing 2.5 ml of scintillation vial at 37 °C for 1 hour to allow the 3H-methanol product of isoAsp 3H-methyl esters to diffuse into the scintillation fluid. The filter paper with the microcentrifuge tube was removed, and radioactivity was counted in a ß-counter. The amount of isoAsp formed was calculated by comparison with the radioactivity formed using a standard isoAsp containing delta sleep-inducing peptide (DSIP; Trp-Ala-Gly-Gly-isoAsp-Ala-Ser-Gly-Glu; W.M. Keck Foundation Biotechnology Resource Laboratory of Yale University) as the standard curve. Protein concentration was estimated by using the method of Bradford (Bio-Rad protein assay kit).
Endogenous PIMT enzyme activity was measured in the cytosol of erythrocytes. The assay is similar to the isoAsp Assay as mentioned above, except the cytosol was incubated with the isoAsp containing DSIP peptide at 37 °C for 5 min. Specific activity was presented as picomoles methyl groups transferred to isoAsp-DSIP per minute per milligram of protein.
Analysis of isoAsp modification of ZAP70 by immunoprecipitation, SDS-PAGE, and 3H fluorography (Hot Gel)
Isolated CD4 T cells from Pcmt1−/− (PIMT−/−) and wild-type mice were stimulated by anti-CD3 and anti-CD28 antibodies for 2 min. Then 70 µg of control or stimulated CD4 T cell lysate was incubated with 4 µg recombinant human PIMT, 50 mM K-MES, 30 µM AdoMet (4µCi of 3H-labeled) at pH6.2 in a 25 µl final volume for 30 min at 30 °C. Reactions were terminated by adding 6 µl of reducing solution (10%SDS and 500mM DTT) for 10 min at 50 °C. After in vitro 3H-methylation labeling by PIMT reaction, samples were precipitated with anti-ZAP70 (1E7.2, Santa Cruz, sc-574AC), mixed with Laemmli sample buffer and subjected to SDS-PAGE on NuPAGE Bis-Tris Novex 4–12% gradient gels using the MES running buffer (Invitrogen). Electrophoresis was carried out at 125 V for 1 h and gel was fixed by acid (45% MeOH, 10% acetic acid) for 30 min, washed with water for 10 min and soaked in 1M Na-salicylate for 30 min. Then, the gel was dried and exposed to Kodak X AR-5 film for 3 months for tritium detection.
Identification of isoAsp residues by mass spectrometry
For in vitro aging, recombinant human ZAP70 (Sigma-Aldrich, Z2126) was incubated at 37 °C for 2 weeks and kept at −80 °C until used. IsoAsp residue mapping of rhZAP70 was identified by PIMT-mediated 18O-labeling of isoaspartic acid by mass spectrometry as described previously with slight modification [Citation35]. In brief, a minimum 10 µg of rhZAP70, native or aged form, was denatured by NH4HCO3, reduced by DTT, tryptic digested, lyophilized and reconstituted with 18O-oxygen water (Cambridge Isotope Laboratories, OLM-240-97-1). Then, PIMT methylation was carried out as described above and then methylation reaction was quenched by guanidine HCl. After alkylation by iodoacetic acid, samples were separated on a Waters nanoACQUITY (75 µm × 250 mm eluted at 300nl/min) with MS analysis on an Orbitrap Elite mass spectrometer. Collected LC–MS/MS data were processed using Proteome Discoverer Software (v. 2.2, Thermo Fisher Scientific) and searches were carried out in MASCOT Search Engine (Matrix Science). The specific replacement of 16O with 18O at isoAsp residue results in a mass shift of 2 daltons. Manual MS/MS inspection of the modified peptides was also carried out to ensure correct assignments/localizations of the isoAsp sites.
Statistical analysis
Statistics were performed using a Student’s unpaired two-tailed t test unless indicated. A value of p < 0.05 was regarded as significant.
Results
IsoAsp PTMs and its repair enzyme, PIMT, are associated with lupus autoimmunity
CD4+ T cell responses from lupus-prone MRL mice and from human SLE patients (and normal human controls) were examined for response to stimulation with anti-CD3. Aberrant T cell hyperproliferation is characterized in both the lupus-prone mouse model () and in humans with SLE (). Previous data demonstrated that a lack of PIMT leads to a similar phenotype of T-cell hyperproliferation [Citation28]. Moreover, isoAsp modification within intracellular proteins in lupus-prone MRL T cells accumulates over age and is increased upon TCR engagement [Citation26]. Therefore, we next investigated whether levels of isoAsp modification and/or activity of its repair enzyme, PIMT, is associated to human lupus.
Figure 1. Isoaspartyl modification and its repair enzyme, PIMT, are associated with lupus autoimmunity. T-cell hyperproliferation was characterized in cells prepared from lupus-prone mice (a) and SLE patients (b). CD4 T cell proliferation assay either from lupus-prone MRL+/+, MRL/lpr and control B10. BR mice (n = 3 for each strain of mouse model) or from SLE patients (n = 20) and healthy donors/subjects (n = 10) were measured in traditional 3H-thymidine incorporation assay as described in Methods. (c) The isoAsp amount in erythrocyte membrane proteins under oxidative stress from SLE patients (n = 28) and healthy donors/subjects (n = 20) were measured by isoAsp vapor assay as described in Methods. (d) The linear association of PIMT and isoAsp amount (slope) was significantly different between healthy donors/subjects and SLE patients (p = 0.01). Specifically, with 1 unit increase in PIMT activity, SLE patients have 0.212 units decrease in fold change of isoAsp amount (oxidative stress group/control group). (*p < 0.05 and **p < 0.005 by Student’s t-test).
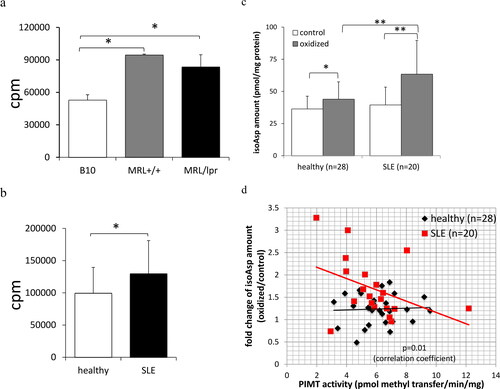
Erythrocytes provide an excellent source of cellular proteins for studying nonenzymatic isoAsp modification since de novo protein biosynthesis does not occur in RBCs. Thus, the ability to repair modified self-proteins completely relies on the existing expression level of PIMT [Citation36]. The increased oxidative stress in SLE patients plays a critical role in contributing to immune dysfunction as described in depth in our previous review and others [Citation37,Citation38]. Under oxidative stress, isoAsp levels in erythrocyte membrane proteins increase compared to control erythrocytes as shown in . However, t-BHT treated erythrocytes from SLE patients are more susceptible to forming isoAsp modification within membrane proteins compared to healthy donors/subjects (). Moreover, isoAsp formation is directly correlated to reduced isoAsp enzyme repair efficiency, i.e. PIMT specific activity, among SLE patients (). However, the fold changes of isoAsp modification under oxidative stress in donors/subjects compared to control group remains similar in healthy individuals regardless of differences in PIMT specific activity.
PIMT overexpression ameliorates aberrant lupus T cell hyperproliferation
Understanding that PIMT-deficient and MRL lupus-prone mice share the same phenotype of T cell hyperproliferation and elevated isoAsp levels in T cells, we next examined if T cell hyperproliferation could be corrected via repair of the isoAsp modification by the PIMT enzyme. The mouse PIMT gene was cloned into the MSCV-Thy1.1 retrovector that expresses Thy1.1 (CD90.1), a surface marker to allow tracking of the infected cell population by flow cytometry. The percentage of CD4+Thy1.1+ cells demonstrated that the infection efficiency of retrovirus with MSCV vector only (Mock) or PIMT-MSCV is similar in both PIMT+/+ and PIMT−/− T cells (). Then CD4+Thy1.1+ cells were purified and measured the expression of retrotransduced PIMT by RT-PCR () and by Western blot (). As indicated, PIMT protein is specifically produced by transfection of PIMT−/− T cells. Importantly, isoAsp detection (vapor assay) confirmed that the high isoAsp content in PIMT deficient-CD4 T cells could be repaired back to the native Asp amino acid by retrotransduced PIMT enzyme that isomerizes the isoAsp residue ().
Figure 2. Characterization of retrotransduced PIMT enzyme in primary murine CD4 T cells. (a) The infection efficiency of retrovirus carried PIMT (PIMT-MSCV) or MSCV vector only (Mock) was similar in all groups as determined by flow cytometry after five days infection, i.e. the percentage of CD4+Thy1.1+ cells. Retro-transduced PIMT mRNA expression by RT-PCR (b) and protein expression by western blot (c) was measured in purified CD4+Thy1.1+ T cells infected by PIMT-MSCV or vector only (Mock) as described in Methods; GAPDH serves as loading control as shown in the bottom panel in (b) and (c). (d) The isoAsp amount was measured by vapor assays as same as in from CD4+Thy1.1+ T cell lysate from PIMT-MSCV or vector only (Mock) group. Data represent mean ± SD and are representative of more than two independent experiments. (*p < 0.05 by Student’s t-test).
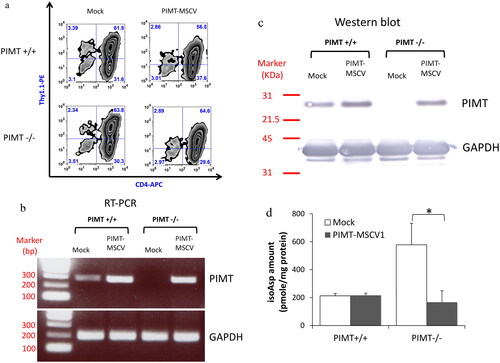
describes the strategy for MSCV retrovirus infection of PIMT−/− T cells, cell stimulation and CFSE proliferation assay for CD4 T cells. The PIMT-deficient CD4 T cells are hyperproliferative compared to wild-type CD4 T cells in vector-only control retrotransduced population. The overexpression of PIMT can suppress isoAsp-mediated T-cell hyperproliferation (Supplementary Figure 1a, top panel). As shown in , not all CD4 T cells are infected by retrovirus, serving as the perfect internal control. We found that there is no suppressive effect on T cell proliferation in the nonretrotransduced population (Thy1.1- T cells) either from wild type or PIMT−/− strain (Supplementary Figure 1a, bottom panel). Moreover, the inhibitory effect of retrotransduced PIMT on T cell proliferation lasts upon restimulation by anti-CD3/28 (). Although the aberrant T cell hyperproliferation in PIMT−/− mouse could not be completely corrected by retrotransduced PIMT enzyme, the percentage of alive proliferating PIMT−/− CD4 T cells (CD4+EMA-CD90.1+gated population) upon 2nd anti-CD3/28 stimulation is significantly inhibited by retrotransduced PIMT compared to cells transduced with vector only.
Figure 3. PIMT ameliorates aberrant lupus T cell hyperproliferation. (a) Schematic representation of strategy for MSCV retrovirus infection, cell stimulation and CFSE proliferation assay on CD4 T cells. The CD4 T cells labeled with CFSE dye and transduced with a retroviral vector expressing PIMT (MSCV-PIMT) or empty vector (Mock) were incubated for another four days to analysis T cell proliferation or stimulated either by CD3/CD28 Dynabeads or antigen again for further proliferation analysis. (b) Upon second stimulation by CD3/28 Dynabeads, flow cytometry of CFSE diluting index from CD4+ EMA-Thy1.1+ T cells transduced with PIMT-MSCV or Mock from PIMT−/− and wild type mouse. EMA (ethidium monoazide), a viability marker. Bottom, the percentages of proliferating CD4+ EMA-Thy1.1+ T cells are represented as mean ± SD. *p < 0.05 by Student’s t-test. (c) Flow cytometry of CFSE diluting index from CD4+ Thy1.1+ T cells transduced with PIMT-MSCV or Mock from B10 or MRL AND transgenic mouse upon PCC88-104 peptide stimulation in the presence of mitomycin c-treated CH27 B cells at Day 12. (d) CFSE-labeled MRL.AND transgenic CD4 T cells transduced with MSCV-PIMT or Mock were intraperitoneal (i.p.) injected into mPCC-transgenic (MRLxB10) F1 recipient mice. Before adoptive transfer, recipient mice were i.p. injected with 3% thioglycollate to elicit antigen-presenting macrophage. Three days later, CFSE diluting index of CD4+Thy1.1+ population was shown in peritoneal cavity (top panel) or in spleen (bottom panel). (n ≥ 3 per group). Data are representative of more than four independent experiments in (b, c) or two independent experiments in (d).
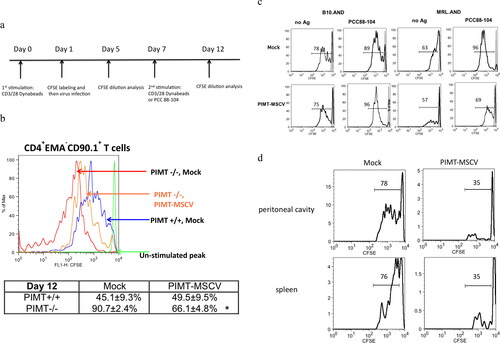
We next examined the inhibitory effect of PIMT on lupus T cell hyperproliferation. In the AND T cell transgenic mouse model, the CFSE proliferative index remains similar in Mock or PIMT-MSCV CD4 T cells upon 1st stimulation by anti-CD3/28 either from B10 or lupus MRL background (Supplementary Figure 1b). However, the inhibitory effect of PIMT (reduced from 96% of Mock to 69% of PIMT-MSCV) was observed in MRL AND T cells upon 2nd stimulation by PCC 88-104 peptide as antigen in the presence of CH27 cells as antigen-presenting cells (). In addition, there is no such proliferative inhibitory effect by retrotransduced PIMT found in B10.AND CD4 T cells () and in nonretrotransduced population (Thy1.1- CD4 T cells) (data not shown).
We next examined whether hyperactivation of autoreactive lupus CD4 T cells could be reduced by retrotransduced PIMT when T cells encounter the ubiquitous self-Ag in vivo. PCC-specific TCR-transgenic CD4 T cells, which were isolated from MRL.AND mice were infected with PIMT-MSCV or vector-only retrovirus, CFSE labeled, and adoptively transferred to F1 recipients [(MRL x B10) F1] with or without expression of PCC as a membrane Ag (mPCC-transgenic F1 or nontransgenic F1, respectively). There was no cell division in Thy1.1+CD4+ TCR-transgenic T cells in nontransgenic F1 mice based on CFSE dilution index (data not shown). However, PIMT-MSCV retrotransduced Thy1.1+CD4+ MRL TCR-transgenic T cells had significantly reduced proliferation compared to donor cells infected with vector only in peritoneal cavity or in spleen from mPCC-transgenic F1 recipients (). In addition, the adoptive transfers of B10.AND to nontransgenic or mPCC-expressing (MRL x B10) F1 recipients were performed in parallel. However, there was no difference in the percentage of proliferating Thy1.1+ CD4+ B10 TCR-transgenic cells found in mPCC-expressing (MRL x B10) F1 recipients between PIMT-MSCV and Mock group (data not shown). Taken together, our data demonstrated that PIMT ameliorates isoAsp modification-mediated T cell hyperproliferation in vitro and in vivo.
PIMT negatively regulates antigen triggered ZAP70 phosphorylation
Abnormalities of T cell signaling in SLE are characterized in both murine and human lupus [Citation4,Citation39]. We next investigated the downstream pathways of how PIMT activity may alter (reduce) T-cell signaling. CD4 T cells were isolated from B10 or MRL AND transgenic mice and infected with PIMT-MSCV or vector only as the similar strategy described in . In day 7, cells were re-stimulated with PCC88-104 as Ag for 30 min in the presence of CH27 cells as the antigen-presenting cells. The phosphorylation status of four TCR signaling molecules, ZAP70, MEK1/2, ERK1/2 and CREB, were simultaneously analyzed by flow cytometry. As shown in , ZAP70 was hyperphosphorylated upon Ag stimulation in Mock group of MRL.AND CD4 T cells compared to B10.AND CD4 T cells in either Thy1.1+ (top panel) or Thy1.1- (bottom panel) population. In addition, the hyperphosphorylation of ZAP70 upon Ag stimulation in MRL T cells was profoundly increased over age (11 months old compared to 14 weeks old). Interestingly, we found that tyrosine phosphorylation (Tyr 319) of ZAP70 upon Ag stimulation was suppressed by overexpression of PIMT in both B10 and MRL.AND CD4 T cells (, top panel, red dotted line). As an internal control, there was no difference in percentages of phospho-ZAP70 population in Thy1.1-CD4+ cells upon Ag stimulation between PIMT-MSCV and Mock group (, bottom panel). However, there was no such inhibitory effect of PIMT on another TCR signaling molecules, i.e. MEK1/2, ERK1/2 and CREB, as shown in . Except in old MRL.AND T cells, PIMT could suppress serine phosphorylation (Ser 222) of MEK1/2 upon Ag stimulation (from 43.7% to 22.6).
Figure 4. PIMT suppresses ZAP70 (Tyr 319) phosphorylation upon Ag stimulation. B10.AND and MRL.AND transgenic CD4 T cells were retrotransduced with PIMT-MSCV or vector only (Mock) as similar described in . After 30 min upon second stimulation by the PCC88-104 peptide, intracellular staining for phosphor-ZAP70 at Tyr 319 residue (a) is shown in CD4+Thy1.1+ (top panel) and in CD4+Thy1.1- (bottom panel) population. The other TCR signaling proteins, phosphor-MEK1/2, phosphor-ERK1/2, and phosphor-CREB, were simultaneously analyzed as shown in (b). Young, 12–14 weeks old. Old, 11 months old. Data are representative of two independent experiments.
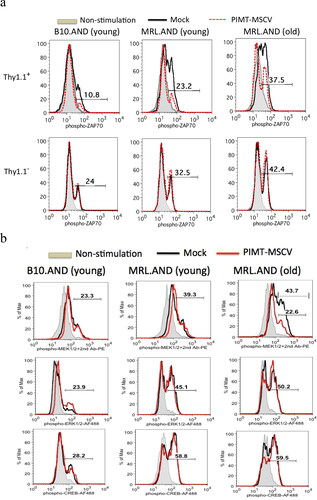
IsoAsp PTMs influence ZAP70 TCR signaling pathway
Upon TCR engagement, CD4-associated Lck kinase phosphorylates the ITAM motif of the CD3 complex. Then, ZAP70 is recruited by the doubly phosphorylated ITAMs and is subsequently phosphorylated at Tyr 319 by Lck, which leads to activate the ZAP70 catalytic activity and its autophosphorylation at Tyr 315 [Citation9]. In our study, MRL T cell hyperproliferation is suppressed by retrotransduced PIMT, but there is no proliferative inhibition by PIMT on normal T cells (). However, PIMT expression suppresses Tyr 319 phosphorylation of ZAP70 upon Ag stimulation in MRL CD4 T cells as well as in normal CD4 T cells (). A simple mechanism of how PIMT restores/repairs normal ZAP70 phosphorylation upon Ag stimulation in both normal and lupus-prone mouse strain is that PIMT repairs the isoAsp modifications in the ZAP70 protein itself. Although spontaneous isoAsp formation occurs in physiological pH and temperature, isoAsp formation can be exacerbated under microenvironmental changes such as oxidative stress, heat shock and aging [Citation40]. To test our hypothesis, recombinant human ZAP70 (rhZAP70) in a solution of 25 mL HEPES, 150 mM NaCl, 0.5mM DTT and 50% glycerol, pH 7.5, was incubated at 37 °C for 2 weeks followed by measuring the isoAsp content. We found that isoAsp content was dramatically increased in aged rhZAP70 protein compared to native form (). By examining the amino acid sequence of ZAP70, there are eight putative isoAsp hot spots, i.e. Asp/Asn-Gly, Asn-Ser and Asn-His, in ZAP70 protein (). Of interest, two of eight isoAsp hot spots, aa 288-291 (NSDG), are located in interdomain-B (I-B, SH2 kinase linker) and near two regulatory tyrosine residues (Tyr 315 and Tyr 319) [Citation41].
Figure 5. Isoaspartyl modification in aged ZAP70 protein. (a) The isoaspartyl (isoAsp) content was measured in aged (incubation for 2 weeks at 37 °C) or in native (unaged) form of human recombinant ZAP70 protein. Data are representative of two independent experiments. (b) Amino acid sequence of human ZAP70. Putative isoAsp hot spots are shown in red bold and Tyr 315 and Tyr319 phosphorylation sites are shown in blue bold and underlined. The locations of isoAsp residue identified by mass spectrometry (Supplementary Table 1) in this study are indicated with red reversed caret. (c) Top panel: The functional domains of ZAP70 structure. Phosphorylation site for downstream Lck kinase (Y319) is indicated at the bottom. The locations of isoAsp residue that arose during in vitro aging of human recombinant ZAP70 are indicated on the top, D92, D290, D303, and D394. I-A; interdomain A. I-B; interdomain B. Bottom panel: highlighted D92, D290, D303, and D394 residues in the crystal structure of human ZAP70 (PDB 2OZO).
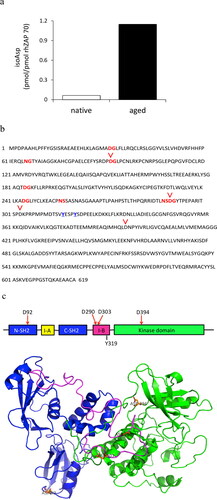
It is challenging to distinguish isoAsp residues from Asp or Asn since the residues have similar charges, structures and even identical masses. We performed a novel technique by using 18-oxygen labeled water coupled with PIMT methylation for isoAsp analysis as described previously [Citation35]. For example, the isoAsp-containing snRNP peptide, a known lupus autoantigen, can be successfully distinguished from Asp form of snRNP peptide by mass spectrometry analysis due to the two daltons shift from 16-oxygen to 18-oxygen introduced by PIMT reaction (data not shown). We then next identified the location of isoAsp residues and quantitated the isoAsp contents in aged rhZAP70 compared to native rhZAP70. We found that Asp 92 in N-SH2 domain, Asp 290 and Asp 303 in interdomain-B (I-B) and Asp 394 in kinase domain were converted to isoAsp during the in vitro aging process ( and Supplementary Table 1).
Next, we examined whether isoAsp modification accrues in ZAP70 protein in vivo. The PIMT-deficient mouse, which lacks the ability to repair isoAsp modifications, provides an ideal model to decipher the role of isoAsp modification on ZAP70 signaling molecule. In brief, purified CD4 T cells were stimulated by anti-CD3 and anti-CD28 for 2 minutes followed by immunoprecipitation of ZAP70. As shown in , ZAP70 protein was captured and confirmed by immunoblot (IB) (bottom panel; IB) and isoAsp modification of ZAP70 was visually revealed upon TCR engagement in PIMT deficient CD4 T cells (top panel; Hot Gel). Relevant to ZAP70 signaling functions, we found that ZAP70 is hyperphosphorylated at Tyr 319 upon TCR engagement in PIMT−/− CD4 T cells compared to wild-type CD4 T cells (). In sum, our data clearly indicate that isoAsp modification mediated by the ZAP70 TCR signaling pathway is involved in aberrant hyperproliferative CD4 T cell immune responses.
Figure 6. Isoaspartyl modification alters ZAP70 TCR signaling pathway. CD4 T cells were isolated from PIMT−/− or wild-type mice and stimulated with anti-CD3 and anti-CD28 mAb. (a) Cells lysates were labeled with 3H-SAM by in vitro PIMT reaction, then immunoprecipitated by polyclonal anti-ZAP70 antibody followed by further analysis for 3H fluorography (top panel, Hot Gel) or immunoblot (bottom panel, IB) in parallel as described in Methods. (b) Upon anti-CD3 and anti-CD28 mAb stimulation, intracellular staining for phosphor-ZAP70 at Tyr 319 residue at different time course in CD4 T cells is shown from PIMT +/+ (top panel) or from PIMT−/− (bottom panel) mice. Data are representative of two independent experiments.
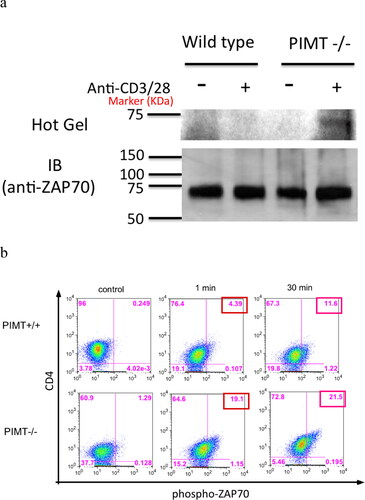
Discussion
ZAP70 is an essential TCR-proximal kinase responsible for T cell signaling [Citation9,Citation42]. The TCR signaling pathway is completely abolished in Jurkat-derived ZAP70 deficient cell line [Citation43]. Moreover, ZAP70-deficient patients with severe combined immunodeficiency (SCID) demonstrated a profound defect in T cell proliferation upon TCR engagement [Citation44]. As mentioned above, there are two SH2 domains (N-SH2 and C-SH2), two interdomains (I-A and I-B) and one tyrosine kinase domain in ZAP70. Recently, crystal structural studies reveal that ZAP70 is not completely activated while recruitment to doubly phosphorylated ITAMs until subsequent phosphorylation by Lck kinase at Tyr 315 and Tyr 319, which are located in I-B domain of ZAP70 [Citation41]. Therefore, I-B domain plays a critical role to switch from autoinhibited ZAP70 to fully activated ZAP70 in TCR signaling. In this study, we found that four amino acid residues, Asp 92, Asp 290, Asp 303 and Asp 394, are highly susceptible to isoAsp modification during in vivo aging process. Two of the isoAsp residues are located in I-B domain of ZAP70. Moreover, lack of isoAsp repair not only leads to isoAsp modification of ZAP70 but also hyperphosphorylation at Tyr 319 in ZAP70 upon TCR engagement (). Recently, other investigators also observed that isoAsp modification is highly associated to protein phosphorylation and acetylation [Citation45]. These observations suggest that isoAsp modification may be linked to other PTMs and synergistically modulates the biological function of proteins.
T cells either from mouse or man appears to have intrinsic abnormalities that may contribute to their ability to drive autoantibody production. In lupus T cells, aberrant TCR signaling such as hyperphosphorylated MEK or ERK is characterized as their hyper-responsive phenotype, which are extensively investigated in both drug-induced and idiopathic lupus erythematosus [Citation8]. Similarly, PIMT deficiency also results in aberrant MEK and ERK activation and autoantibody production [Citation28,Citation46,Citation47]. Recently, CREB (cAMP-responsive element-binding protein) and CREM (cAMP-responsive element modulator) are believed to play an important role to regulate cytokine production in lupus T cells [Citation39,Citation48]. In this study, ZAP70, CREB, ERK1/2 and MEK1/2 were simultaneously investigated to determine if overexpression of PIMT could modulate lupus T cell signaling. In analysis of protein motifs, there are no isoAsp hot spot sequences in CREB and only two isoAsp hot spots in ERK1/2 (Asn 218-Ser and Asn 255-His in ERK1; Asn 201-Ser and Asn 238-His in ERK2). There are eight isoAsp hot spots in MEK1/2, the same with ZAP70, but MEK1/2 is phosphorylated at Ser residue, not at Tyr residue in ZAP70. Of note, retrotransduced PIMT only can suppress Tyr 319-phosphorylation of ZAP70 in activated lupus T cells regardless of age () but only can suppress Ser 222-phosphrylation of MEK in elder activated lupus T cells (). We do not know if the phosphorylation site at Tyr versus Ser affects the susceptibility of protein phosphorylation to isoAsp modification and PIMT. Moreover, there is no isoAsp modification within ZAP70 in unstimulated PIMT deficient T cells until cells were stimulated by anti-CD3 and anti-CD28 mAb (). This observation suggests that isoAsp modification may serve as a new PTM biomarker for assessing cellular signaling transduction and that PIMT is responsible for isoAsp repair, and downstream pathways of phosphorylation and phosphatase in signaling cascade.
Besides the role of PTMs in immune cell signaling, they also are biomarkers of disease as targets of autoimmunity. It is well established that patients with RA make robust autoimmune responses to citrullinated fibrinogens found in the inflamed synovial tissue and detectable autoantibodies against cyclic citrullinated peptide (CCP) [Citation49]. Anti-CCP autoantibody becomes a standard biological marker for diagnosis and prognosis of RA. Moreover, emerging data revealed novel PTM biomarkers associated with autoimmune diseases and provide PTM-based therapeutic strategies [Citation50]. Recently, Colasanti T. et al. identified autoantibodies against to novel PTM antigens, homocysteinylated alpha 1 antitrypsin, from both seropositive and seronegative RA patients [Citation51]. Our recent studies demonstrated that citrullinated glucokinase and carbonylated beta subunit of prolyl-4-hydroxylase (P4Hb; also named protein disulphide isomerase, PDIA1) modulate both insulin homeostasis, the key biological function of pancreatic beta cells, and type 1 diabetes (T1D) autoimmunity [Citation52,Citation53]. Moreover, P4Hb/PDIA1 plasma levels increase in recent-onset T1D patients compared to non-diabetic controls, suggesting that P4Hb/PDIA1 is a potential noninvasive biomarker of pathology in T1D.
In a similar manner as described above, several PTMs are known to play a critical role in SLE autoimmunity, such as citrullination and carbamylation [Citation54]. For example, it is reported that protein citrullination level in PBMCs is increased in SLE patients [Citation55]. Of interest, autoantibodies against specific PTMs, malondialdehyde-acetaldehyde adducts (MAA), advanced glycation end-product (AGE) and carbamylation, were more prevalent in SLE patients compared to controls [Citation56]. Our previous data demonstrated that lupus-like T and B cell immune responses could be elicited with isoAsp-modified self-antigens, both snRNPs and cytochrome c [Citation57]. PTMs also clearly influence antigen processing pathways, likely contributing to aberrant autoimmune responses [Citation58]. Of interest, isoAsp residue was found in intact U1/Sm snRNP complexes as well as in histone H2B, key targets of autoantibodies in SLE [Citation59,Citation60].
Herein, we demonstrated that the level of isoAsp modification under oxidative stress is higher in peripheral erythrocytes from SLE patients compared to healthy individuals and such increase of isoAsp amount in SLE patients is dependent on the lack of isoAsp repair efficiency, i.e. PIMT activity. T cell hyperproliferation is a key phenotype in both murine and human models of SLE. Interestingly, our data shown that overexpression of PIMT suppresses isoAsp-mediated T cell hyperproliferation in PIMT deficient T cells and in lupus-prone MRL T cells but not in wild-type T cells. Our finding aligns with our previous study that the isoAsp content was higher in lupus-prone MRL T cells compared to wild-type T cells [Citation26]. In addition, we found that the ZAP70 TCR signaling pathway is down-regulated by overexpression of PIMT. Moreover, isoAsp-modified and aberrant hyperphosphorylated ZAP70 is triggered upon TCR engagement in PIMT-deficient T cells. Taken together, our studies provide a novel mechanism of how isoAsp modification and its repair enzyme, PIMT, alter T cell functions mediated by the ZAP70 TCR signaling pathway.
To date, PIMT is the only known isoAsp-repairing enzyme. Interestingly, Dai et al identified high isoAsp content in major urinary proteins (UNPs) from PIMT-deficient mice [Citation61]. The study suggests that there are alternative proteolytic and excretion pathways to metabolize isoAsp-containing proteins in vivo in addition to the PIMT enzyme. The alternative isoAsp-containing protein degradation pathways may compensate for lower PIMT activity that is found in polymorphisms of the PIMT gene.
SLE is an autoimmune disease thought to be caused by the combination of genetic and environmental factors such as infection, smoking, medications and ultraviolet (UV) light [Citation62]. Aberrant T cell function, either from intrinsic or extrinsic factors, contributes to the initiation of autoimmunity and the development of organ pathology in lupus. IsoAsp modification is a spontaneous PTM at physiological pH and temperature without any enzyme involved but isoAsp formation is highly dependent on subtle environmental changes including heat shock, oxidative stress, and UV irradiation. For example, UV-induced D-β-Asp- or L-isoAsp-formation can be found in elastic fibers of human skin [Citation63] and in human melanoma cells [Citation64], respectively. Herein, our study demonstrates that oxidative stress-induced isoAsp modification is elevated in SLE patients compared to healthy donors/subjects. Moreover, we describe a model system for studying the role of isoAsp modification and its repair enzyme, PIMT, in the pathways of TCR signaling and activation. Our results indicate that isoAsp-modified ZAP70 is a potential therapeutic target of PIMT repair for treating T-cell mediated lupus-like autoimmune disease.
Author contributions
M.L.Y. designed and performed the experiments and wrote the manuscript. T.T.L and J.K. did the mass spectrometry experiments and interpreted the results. I.K. collected human samples of SLE patients and healthy donors/subjects and provided advice for handling human samples. Z.S.Z. contributed the critical discussion for PIMT-mediated 18O-labeling of isoaspartic acid by mass spectrometry. S.G.C. contributed conceptual expertise, provided inputs into the design of the study, and edited the paper. M.J.M. supervised all the experiments and edited the manuscript.
Supplemental Material
Download MS Word (507.7 KB)Acknowledgments
We thank Renelle Gee and Anthony Ferrandino for technical assistant for the study; Susan Kaech (Yale School of Medicine, Connecticut) for providing the MSCV and pEco vector; Joe Craft and Ping Zhou (Yale School of Medicine, Connecticut) for providing mPCC transgenic mice; Min Sun Shin and Naeun Lee for purifying PBMC from human samples; Wuyi Meng (Chemical and Biophysical Instrumentation Center, Yale University) for critical discussion for ZAP70 crystal structure; and Yanhong Deng (Yale Center for Analytical Sciences, Yale School of Public Health) for statistical help of correlation analysis.
Disclosure statement
The authors declare no competing financial interests.
Data availability statement
The data that support the findings of this study are available from the corresponding author upon reasonable request.
Additional information
Funding
References
- Arthur JS, Ley SC. Mitogen-activated protein kinases in innate immunity. Nat Rev Immunol. 2013;13(9):1–13.
- Kortum RL, Rouquette-Jazdanian AK, Samelson LE. Ras and extracellular signal-regulated kinase signaling in thymocytes and T cells. Trends Immunol. 2013;34(6):259–268.
- Mills KH. TLR-dependent T cell activation in autoimmunity. Nat Rev Immunol. 2011;11(12):807–822.
- Crispin JC, Kyttaris VC, Juang YT, et al. How signaling and gene transcription aberrations dictate the systemic lupus erythematosus T cell phenotype. Trends Immunol. 2008;29(3):110–115.
- Katsuyama T, Tsokos GC, Moulton VR. Aberrant T cell signaling and subsets in systemic lupus erythematosus. Front Immunol. 2018;9:1088.
- Takeuchi Y, Hirota K, Sakaguchi S. Impaired T cell receptor signaling and development of T cell-mediated autoimmune arthritis. Immunol Rev. 2020;294(1):164–176.
- Camperio C, Muscolini M, Volpe E, et al. CD28 ligation in the absence of TCR stimulation up-regulates IL-17A and pro-inflammatory cytokines in relapsing-remitting multiple sclerosis T lymphocytes. Immunol Lett. 2014;158(1-2):134–142.
- Gorelik G, Richardson B. Key role of ERK pathway signaling in lupus. Autoimmunity. 2010;43(1):17–22.
- Wang H, Kadlecek TA, Au-Yeung BB, et al. ZAP-70: an essential kinase in T-cell signaling. Cold Spring Harb Perspect Biol. 2010;2(5):a002279.
- Lawson BR, Eleftheriadis T, Tardif V, et al. Transmethylation in immunity and autoimmunity. Clin Immunol. 2012;143(1):8–21.
- Sekigawa I, Kawasaki M, Ogasawara H, et al. DNA methylation: its contribution to systemic lupus erythematosus. Clin Exp Med. 2006;6(3):99–106.
- Zhang Y, Zhao M, Sawalha AH, et al. Impaired DNA methylation and its mechanisms in CD4(+)T cells of systemic lupus erythematosus. J Autoimmun. 2013;41:92–99.
- Brahms H, Raymackers J, Union A, et al. The C-terminal RG dipeptide repeats of the spliceosomal Sm proteins D1 and D3 contain symmetrical dimethylarginines, which form a major B-cell epitope for anti-Sm autoantibodies. J Biol Chem. 2000;275(22):17122–17129.
- Mahler M, Fritzler MJ, Bluthner M. Identification of a SmD3 epitope with a single symmetrical dimethylation of an arginine residue as a specific target of a subpopulation of anti-Sm antibodies. Arthritis Res Ther. 2005;7(1):R19–29.
- Parry RV, Ward SG. Protein arginine methylation: a new handle on T lymphocytes? Trends Immunol. 2010;31(4):164–169.
- Tanaka Y, Nagai Y, Okumura M, et al. PRMT5 is required for T cell survival and proliferation by maintaining cytokine signaling. Front Immunol. 2020;11:621.
- Blanchet F, Cardona A, Letimier FA, et al. CD28 costimulatory signal induces protein arginine methylation in T cells. J Exp Med. 2005;202(3):371–377.
- Clarke S. Aging as war between chemical and biochemical processes: protein methylation and the recognition of age-damaged proteins for repair. Ageing Res Rev. 2003;2(3):263–285.
- Doyle HA, Mamula MJ. Autoantigenesis: the evolution of protein modifications in autoimmune disease. Curr Opin Immunol. 2012;24(1):112–118.
- Doyle HA, Yang ML, Raycroft MT, et al. Autoantigens: novel forms and presentation to the immune system. Autoimmunity. 2014;47(4):220–233.
- Teshima G, Porter J, Yim K, et al. Deamidation of soluble CD4 at asparagine-52 results in reduced binding capacity for the HIV-1 envelope glycoprotein gp120. Biochemistry. 1991;30(16):3916–3922.
- Zhang Y, Wang H. Integrin signaling and function in immune cells. Immunology. 2012;135(4):268–275.
- Evans R, Lellouch AC, Svensson L, et al. The integrin LFA-1 signals through ZAP-70 to regulate expression of high-affinity LFA-1 on T lymphocytes. Blood. 2011;117(12):3331–3342.
- Corti A, Curnis F. Isoaspartate-dependent molecular switches for integrin-ligand recognition. J Cell Sci. 2011;124(Pt 4):515–522.
- Zhang Y, Martinez T, Woodruff B, et al. Hydrophobic interaction chromatography of soluble interleukin I receptor type II to reveal chemical degradations resulting in loss of potency. Anal Chem. 2008;80(18):7022–7028.
- Yang ML, Doyle HA, Gee RJ, et al. Intracellular protein modification associated with altered T cell functions in autoimmunity. J Immunol. 2006;177(7):4541–4549.
- Clarke S. Protein carboxyl methyltransferases: two distinct classes of enzymes. Annu Rev Biochem. 1985;54:479–506.
- Doyle HA, Gee RJ, Mamula MJ. A failure to repair self-proteins leads to T cell hyperproliferation and autoantibody production. J Immunol. 2003;171(6):2840–2847.
- Ingrosso D, D’Angelo S, di Carlo E, et al. Increased methyl esterification of altered aspartyl residues in erythrocyte membrane proteins in response to oxidative stress. Eur J Biochem. 2000;267(14):4397–4405.
- Lee J, Sadelain M, Brentjens R. Retroviral transduction of murine primary T lymphocytes. Methods Mol Biol. 2009;506:83–96.
- Bouzahzah F, Jung S, Craft J. CD4+ T cells from lupus-prone mice avoid antigen-specific tolerance induction in vivo. J Immunol. 2003;170(2):741–748.
- Krutzik PO, Nolan GP. Intracellular phospho-protein staining techniques for flow cytometry: monitoring single cell signaling events. Cytometry A. 2003;55(2):61–70.
- MacLaren DC, Clarke S. Expression and purification of a human recombinant methyltransferase that repairs damaged proteins. Protein Expr Purif. 1995;6(1):99–108.
- Villa ST, Xu Q, Downie AB, et al. Arabidopsis protein repair L-isoaspartyl methyltransferases: predominant activities at lethal temperatures. Physiol Plant. 2006;128(4):581–592.
- Liu M, Cheetham J, Cauchon N, et al. Protein isoaspartate methyltransferase-mediated 18O-labeling of isoaspartic acid for mass spectrometry analysis. Anal Chem. 2012;84(2):1056–1062.
- Galletti P, Ingrosso D, Manna C, et al. Protein damage and methylation-mediated repair in the erythrocyte. Biochem J. 1995;306 (Pt 2):313–325.
- Yang ML, Doyle HA, Clarke SG, et al. Oxidative Modifications in Tissue Pathology and Autoimmune Disease. Antioxid Redox Signal. 2018;29(14):1415–1431.
- Yan Z, Chen Q, Xia Y. Oxidative stress contributes to inflammatory and cellular damage in systemic lupus erythematosus: cellular markers and molecular mechanism. J Inflamm Res. 2023;16:453–465.
- Moulton VR, Tsokos GC. Abnormalities of T cell signaling in systemic lupus erythematosus. Arthritis Res Ther. 2011;13(2):207.
- Reissner KJ, Aswad DW. Deamidation and isoaspartate formation in proteins: unwanted alterations or surreptitious signals? Cell Mol Life Sci. 2003;60(7):1281–1295.
- Yan Q, Barros T, Visperas PR, et al. Structural basis for activation of ZAP-70 by phosphorylation of the SH2-kinase linker. Mol Cell Biol. 2013;33(11):2188–2201.
- Au-Yeung BB, Levin SE, Zhang C, et al. A genetically selective inhibitor demonstrates a function for the kinase Zap70 in regulatory T cells independent of its catalytic activity. Nat Immunol. 2010;11(12):1085–1092.
- Williams BL, Schreiber KL, Zhang W, et al. Genetic evidence for differential coupling of Syk family kinases to the T-cell receptor: reconstitution studies in a ZAP-70-deficient Jurkat T-cell line. Mol Cell Biol. 1998;18(3):1388–1399.
- Noraz N, Schwarz K, Steinberg M, et al. Alternative antigen receptor (TCR) signaling in T cells derived from ZAP-70-deficient patients expressing high levels of Syk. J Biol Chem. 2000;275(21):15832–15838.
- Qin Z, Kaufman RS, Khoury RN, et al. Isoaspartate accumulation in mouse brain is associated with altered patterns of protein phosphorylation and acetylation, some of which are highly sex-dependent. PLoS One. 2013;8(11):e80758.
- Furuchi T, Sakurako K, Katane M, et al. The role of protein L-isoaspartyl/D-aspartyl O-methyltransferase (PIMT) in intracellular signal transduction. Chem Biodivers. 2010;7(6):1337–1348.
- Ryu J, Song J, Heo J, et al. Cross-regulation between protein L-isoaspartyl O-methyltransferase and ERK in epithelial mesenchymal transition of MDA-MB-231 cells. Acta Pharmacol Sin. 2011;32(9):1165–1172.
- Rauen T, Hedrich CM, Tenbrock K, et al. cAMP responsive element modulator: a critical regulator of cytokine production. Trends Mol Med. 2013;19(4):262–269.
- Raptopoulou A, Sidiropoulos P, Katsouraki M, et al. Anti-citrulline antibodies in the diagnosis and prognosis of rheumatoid arthritis: evolving concepts. Crit Rev Clin Lab Sci. 2007;44(4):339–363.
- Yang ML, Kibbey RG, Mamula MJ. Biomarkers of autoimmunity and beta cell metabolism in type 1 diabetes. Front Immunol. 2022;13:1028130.
- Colasanti T, Sabatinelli D, Mancone C, et al. Homocysteinylated alpha 1 antitrypsin as an antigenic target of autoantibodies in seronegative rheumatoid arthritis patients. J Autoimmun. 2020;113:102470.
- Yang ML, Horstman S, Gee R, et al. Citrullination of glucokinase is linked to autoimmune diabetes. Nat Commun. 2022;13(1):1870.
- Yang ML, Connolly SE, Gee RJ, et al. Carbonyl posttranslational modification associated with early-onset type 1 diabetes autoimmunity. Diabetes. 2022;71(9):1979–1993.
- Ziegelasch M, van Delft MA, Wallin P, et al. Antibodies against carbamylated proteins and cyclic citrullinated peptides in systemic lupus erythematosus: results from two well-defined European cohorts. Arthritis Res Ther. 2016;18(1):289.
- Colasanti T, Spinelli FR, Barbati C, et al. Belimumab decreases autophagy and citrullination in peripheral blood mononuclear cells from patients with systemic lupus erythematosus. Cells. 2022;11(2):262–279.
- Monahan RC, van den Beukel MD, Borggreven NV, et al. Autoantibodies against specific post-translationally modified proteins are present in patients with lupus and associate with major neuropsychiatric manifestations. RMD Open. 2022;8(1):1–10.
- Mamula MJ, Gee RJ, Elliott JI, et al. Isoaspartyl post-translational modification triggers autoimmune responses to self-proteins. J Biol Chem. 1999;274(32):22321–22327.
- Doyle HA, Gee RJ, Mamula MJ. Altered immunogenicity of isoaspartate containing proteins. Autoimmunity. 2007;40(2):131–137.
- Zhu JX, Doyle HA, Mamula MJ, et al. Protein repair in the brain, proteomic analysis of endogenous substrates for protein L-isoaspartyl methyltransferase in mouse brain. J Biol Chem. 2006;281(44):33802–33813.
- Young AL, Carter WG, Doyle HA, et al. Structural integrity of histone H2B in vivo requires the activity of protein L-isoaspartate O-methyltransferase, a putative protein repair enzyme. J Biol Chem. 2001;276(40):37161–37165.
- Dai S, Ni W, Patananan AN, et al. Integrated proteomic analysis of major isoaspartyl-containing proteins in the urine of wild type and protein L-isoaspartate O-methyltransferase-deficient mice. Anal Chem. 2013;85(4):2423–2430.
- Zandman-Goddard G, Solomon M, Rosman Z, et al. Environment and lupus-related diseases. Lupus. 2012;21(3):241–250.
- Fujii N, Tajima S, Tanaka N, et al. The presence of D-beta-aspartic acid-containing peptides in elastic fibers of sun-damaged skin: a potent marker for ultraviolet-induced skin aging. Biochem Biophys Res Commun. 2002;294(5):1047–1051.
- D’Angelo S, Ingrosso D, Perfetto B, et al. UVA irradiation induces L-isoaspartyl formation in melanoma cell proteins. Free Radic Biol Med. 2001;31(1):1–9.