Abstract
Mesenchymal stem cells-derived exosomes (MSC-Exo) are considered to have great potential in the treatment of human diseases. However, the role of MSC-Exo in the process of diabetes with sepsis and the underlying molecular mechanism remain unclear. Human pulmonary microvascular endothelial cells (HPMECs) were treated with high glucose (HG) and lipopolysaccharide (LPS). Cell viability, migration, angiogenesis were analyzed by cell counting kit 8 assay, transwell assay and tube formation assay. Transmembrane electrical resistance (TER) detection and FITC-dextran assay were performed to evaluate cell barrier function. The protein levels of cell permeability-related markers, ferroptosis-related markers, exosomes-related markers, Nrf2 and HO-1 were examined using western bolt (WB) analysis. Besides, the levels of inflammation factors were tested by ELISA, and the levels of ferroptosis-related indicators were examined using corresponding assay kits. Flow cytometry was employed to analyze stem cell markers. The identification of MSC-Exo was performed using transmission electron microscopy, nanoparticle tracking analysis and WB analysis. DIO staining was used to examine the uptake of MSC-Exo by HPMECs. HG treatment suppressed HPMECs viability, migration, angiogenesis and TER, while promoted permeability, inflammation and ferroptosis. LPS treatment aggravated HG-induced HPMECs dysfunction, inflammation and ferroptosis. After HPMECs were co-cultured with MSC-Exo, cell injury induced by HG + LPS could be relieved. Moreover, MSC-Exo treatment enhanced the activity of Nrf2/HO-1 pathway in HG + LPS-induced HPMECs, and Nrf2-silenced MSC-Exo could promote HG + LPS-induced HPMECs injury. MSC-Exo alleviated HG + LPS-induced HPMECs injury via activating Nrf2/HO-1 pathway, confirming that it might be used for the treatment of diabetes with sepsis.
Introduction
People with diabetes often have poor immunity and increased susceptibility to a variety of infections, so the risk of sepsis is significantly increased [Citation1,Citation2]. When sepsis occurs, the activation of the neuroendocrine system and the release of many inflammatory mediators can lead to a sudden rise in blood glucose, resulting in injury to the cardiovascular system, nervous system and respiratory system [Citation3,Citation4]. The lungs are rich in connective tissue and microvascular circulation, making them vulnerable to hyperglycaemia and infection [Citation5,Citation6]. Therefore, elucidating the molecular mechanism affecting the injury of human pulmonary microvascular endothelial cells (HPMECs) caused by high glucose (HG) combined with lipopolysaccharide (LPS) is of great significance for treating diabetes with sepsis.
Mesenchymal stem cells (MSC) have the ability of self-renewal and multidirectional differentiation, and the therapeutic role of exosomes derived from MSC (MSC-Exo) in human diseases has been widely confirmed [Citation7,Citation8]. MSC-Exo could repress cell apoptosis to alleviate diabetic kidney disease [Citation9], and could enhance angiogenesis to accelerate diabetic wound healing [Citation10]. In sepsis-related studies, MSC-Exo had been confirmed to relieve sepsis-induced lung injury [Citation11] and myocardial injury [Citation12]. Therefore, MSC-Exo has great potential in the treatment of diabetes and sepsis. However, the role of MSC-Exo in HG + LPS-induced HPMECs injury and its underlying molecular mechanisms need to be further studied.
Ferroptosis is a new type of iron-dependent and non-apoptotic cell death induced by lipid peroxidation, has been confirmed to related with human disease progression [Citation13,Citation14]. Nrf2 is a key factor that controls cellular redox homeostasis, inflammation and ferroptosis [Citation15]. Studies have shown that activation of Nrf2/HO-1 pathway may also protect cells from ferroptosis in many human diseases, such as colorectal cancer [Citation16] and acute lung injury (ALI) [Citation17]. It had been reported that activated Nrf2/HO-1 pathway could inhibit HG-induced ferroptosis in diabetic osteoporosis cell models [Citation18]. Also, activating the Nrf2/HO-1 pathway was confirmed to protect against sepsis-induced ALI, which could suppress LPS-induced ferroptosis in alveolar epithelial cells [Citation19]. Importantly, previous study suggested that MSC-Exo had a promotion effect on the activity of Nrf2/HO-1 pathway [Citation20]. However, whether MSC-Exo ameliorates HPMECs injury in diabetes and sepsis by mediating the Nrf2/HO-1 pathway remains unclear.
The aim of this study was to reveal the role and potential molecular mechanism of MSC-Exo in HG + LPS-induced HPMECs injury. Through analysis, we determined that MSC-Exo inhibited HG + LPS-induced HPMECs dysfunction, inflammation, and ferroptosis by activating the Nrf2/HO-1 pathway, which might provide new ideas for the treatment of MSC-Exo in diabetes combined with sepsis.
Materials and methods
Cell culture and treatment
HPMECs were bought from Procell (Wuhan, China) and cultured in HPMECs complete medium (Procell) at 37 °C with 5% CO2. Cells treated with high concentration of D-glucose (30 mM, Sigma-Aldrich, St. Louis, MO, USA) for 24 h to mimic HG cell model with normal glucose (NG, 5.5 mM) as control. Besides, cells were treated with 1 µg/mL LPS (Sigma-Aldrich) under HG or NG conditions for 24 h to mimic diabetes with sepsis cell model.
MSC culture and transfection
Human marrow MSC (Procell) was cultured in specified MSC completed medium (Procell) at 37 °C with 5% CO2. Nrf2 small interfering RNA (si-Nrf2) and its control (si-NC) were transfected into MSC using Lipofectamine 3000 (Invitrogen, Carlsbad, CA, USA).
Cell counting kit 8 (CCK8) assay
In 96-well plates, HPMECs were incubated with CCK8 reagent (Dojindo, Kumamoto, Japan). Cell viability was determined at 450 nm using a microplate reader.
Transwell assay
HPMECs suspended with serum-free medium were seeded on the upper of transwell chambers (24-well; Corning Inc., Corning, NY, USA). Additionally, lower chamber was filled with complete medium. After cultured 24 h at 37 °C, the migrated cells were fixed and stained. Cells were photographed under a microscope to count migrated cell number.
Tube formation assay
HPMECs were collected and then seeded into 96-well plates pre-coated Matrigel (Corning Inc.). After incubated for 6 h, tube formation rate was assessed by counting the number of branch nodes and tube lengths under a microscope using the ImageJ plugin Angiogenesis Analyser.
Transmembrane electrical resistance (TER) detection
HPMECs were grown in electrode wells containing gold electrodes and cultured until 95% confluences. After equilibration for 1 h, TER was analysed using electrical cell-substrate impedance sensing system (Applied Biophysics, Troy, NY, USA).
FITC-dextran assay
HPMECs in serum-free medium were seeded on the upper of transwell chambers to form a cell monolayer over the membrane, with complete medium filled in lower chamber. Then, cells were incubated with FITC-dextran solution (Sigma-Aldrich) for 1 h, and fluorescence signal was detected using a microplate reader to assess cell permeability.
Western blot (WB) analysis
Total proteins extracted by RIPA buffer (Beyotime, Shanghai, China) were transferred onto PVDF membranes after separated by SDS-PAGE gel. Membrane was blocked and then incubated with anti-ZO-1 (13663, 1:1000, CST, Danvers, MA, USA), anti-VE-cadherin (2500, 1:1000, CST), anti-SLC7A11 (ab175186, 1:1000, Abcam, Cambridge, MA, USA), anti-GPX4 (67763-1-Ig, 1:5000, Proteintech, Rosemont, IL, USA), anti-CD9 (60232-1-Ig, 1:2000, Proteintech), anti-CD81 (66866-1-Ig, 1:3000, Proteintech), anti-TSG101 (28283-1-AP, 1:8000, Proteintech), anti-Calnexin (10427-2-AP, 1:10000, Proteintech), anti-Nrf2 (ab62352, 1:1000, Abcam), anti-HO-1 (ab189491, 1:2000, Abcam) and anti-GAPDH (ab9485, 1:2500, Abcam). Membrane was incubated with secondary antibody (ab205718 or ab205719, 1:20000, Abcam), and protein signals were exposed using ECL reagent (Beyotime).
ELISA
The culture medium of HPMECs was collected and centrifuged to obtain supernatants. TNF-α, IL-1β and IL-6 levels were detected with corresponding ELISA Kits (EK182, EK101b, EK106; Multisciences, Hangzhou, China) according to kit instructions.
Determine of ferroptosis
To assess cell ferroptosis, ROS, MDA, GSH and Fe2+ levels in HPMECs were detected basing on the instructions of ROS Assay Kit, MDA Assay Kit, GSH Assay Kit and Iron Assay Kit (Abcam), respectively.
Flow cytometry
MSC was incubated with FITC-labelled anti-CD34 (ab18227, Abcam), anti-CD45 (ab27287, Abcam), anti-CD105 (ab53318, Abcam), anti-CD73 (ab239246, Abcam) and anti-CD90 (ab226, Abcam). The signals of stem cell markers (CD105, CD73 and CD90) and haematopoietic markers (CD34 and CD45) were analysed by flow cytometry and FlowJo software.
Oil red O and alkaline phosphatase (ALP) staining
For adipogenic differentiation, MSC was seeded into 6-well plates and then induced with adipogenic induction solution for 10 days. Then, cells were fixed with 4% PFA and stained with Oil Red O solution (Beyotime) for 15 min. Adipogenesis was observed under a microscope.
For osteogenic differentiation, MSC in 6-well plates were induced with osteogenic differentiation solution for 14 days. After fixed with 4% PFA, cells were incubated with BCIP/NBT solution (Beyotime) for 15 min. ALP-positive cells were observed using a microscope.
Exosome isolation and identification
The exosomes were isolated from the culture supernatant of MSC by ultracentrifugation following the below steps: 500 × g for 10 min, 2000 × g for 30 min, 100,000 × g for 90 min, and 100,000 × g for 60 min. The morphology and size of MSC-derived exosomes (MSC-Exo) were analysed by transmission electron microscopy (TEM) and nanoparticle tracking analysis (NTA). Exosome protein markers (CD9, CD81 and TSG101) were detected using WB analysis with Calnexin as negative control.
Exosome uptake assay
MSC-Exo was incubated with 10 μM DIO (Beyotime) for 30 min. DIO-labelled MSC-Exo was harvested and then co-cultured with HPMECs for 24 h. Then, cells were fixed by paraformaldehyde and stained by DAPI, and then the uptake of DIO-labelled exosomes in HPMECs were observed under a fluorescence microscope.
Statistical analysis
Data are presented as mean ± SD using the GraphPad 7.0 software. Comparison between groups was analysed by Student’s t-test or ANOVA. p < 0.05 was considered statistically significant.
Results
LPS aggravated HG-induced HPMECs dysfunction
To explore the role of LPS on HG-induced HPMECs dysfunction, HPMECs were induced with HG and LPS. We found that HG treatment could reduce viability, migrated cell number, tube formation rate, and TER in HPMECs, whereas LPS could exacerbate these effects (). In addition, HG treatment could increase the permeability of HPMECs, and this effect could be promoted by LPS treatment (). By detecting cell permeability-related proteins, we confirmed that LPS treatment could accelerate the reducing effect of HG treatment on ZO-1 and VE-cadherin levels in HPMECs (). These results revealed that LPS exacerbated HG-induced HPMECs dysfunction.
Figure 1. LPS exacerbated HG-induced dysfunction in HPMECs. HPMECs were induced with HG and LPS for 24 h. CCK8 (A), transwell assay (B), tube formation assay (C) and TER assay (D) were used to test cell viability, migration, angiogenesis and TER. (E) FITC-dextran assay for the determination of cell permeability. (F) The protein levels of ZO-1 and VE-cadherin were measured by WB analysis. *p < 0.05; **p < 0.01.
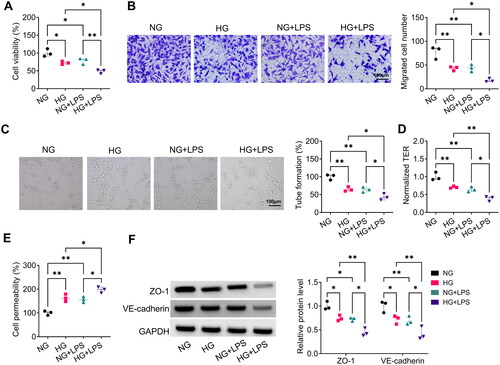
LPS accelerated HG-induced inflammation and ferroptosis in HPMECs
Then, we analysed the effect of LPS on HG-induced HPMECs inflammation and ferroptosis. Under HG-induced conditions, HPMECs had higher levels of proinflammatory cytokines (TNF-α, IL-1β and IL-6) and ferroptosis-related markers (ROS, MDA and Fe2+), while had lower level of GSH. Importantly, LPS could augment these effects (). Furthermore, LPS treatment enhanced the inhibition effect of HG treatment on the levels of ferroptosis-associated proteins SLC7A11 and GPX4 in HPMECs (). All results indicated that LPS could exacerbate HG-induced HPMECs injury.
Isolation and identification of MSC-Exo
First, we identified MSC and found that stem cell markers CD105, CD73 and CD90 were positive, while haematopoietic markers CD34 and CD45 were negative (). Also, oil red O and ALP staining showed that MSC had the potential of osteogenic and adipogenic differentiation (). After that, we isolated the MSC-Exo and observed its morphology, particle size and concentration using TEM and NTA (). In addition, exosomes markers (CD9, CD81 and TSG101) were detected in the isolated MSC-Exo (). Eventually, DIO labelling results showed that MSC-Exo could be uptake by HPMECs (). This indicated that the isolation of MSC-Exo was successful.
Figure 3. Isolation and identification of exosomes from MSC. (A) Flow cytometry was used to identify CD105, CD73, CD90, CD34, and CD45 in MSC. (B) The results of oil red O and ALP staining. (C) TEM was used to observe the MSC-Exo morphology. (D) NTA for measuring the size and concentration of MSC-Exo. (E) Exosome marker protein (CD63, CD81 and TSG101) and negative protein (Calnexin) were detected by WB analysis. (F) MSC-Exo was labelled with DIO to assess the uptake of MSC-Exo by HPMECs.
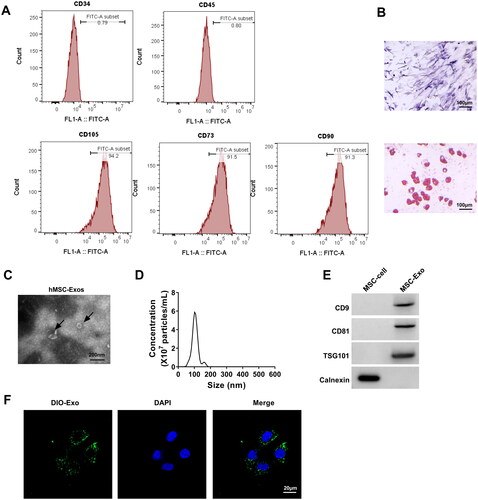
MSC-Exo alleviated HG + LPS-induced HPMECs dysfunction
To examine the effect of MSC-Exo on HG + LPS-induced dysfunction in HPMECs, MSC-Exo was co-incubated with HPMECs and then induced by HG and LPS. The experimental results showed that MSC-Exo could promote the viability, migration, angiogenesis, and TER in HG + LPS-induced HPMECs (). Besides, MSC-Exo reduced HPMECs permeability and enhanced the protein levels of ZO-1 and VE-cadherin under HG + LPS treatment conditions (). These findings demonstrated that MSC-Exo inhibited HG + LPS-induced HPMECs dysfunction.
Figure 4. MSC-Exo attenuated HG + LPS-induced HPMECs dysfunction. HPMECs were co-incubated with MSC-Exo and then induced using HG and LPS. Cell viability, migration, angiogenesis and TER were detected using CCK8 (a), transwell assay (B), tube formation assay (C) and TER assay (D). (E) FITC-dextran assay was used to test cell permeability. (F) WB analysis for detection the protein levels of ZO-1 and VE-cadherin. **p < 0.01; ***p < 0.001.
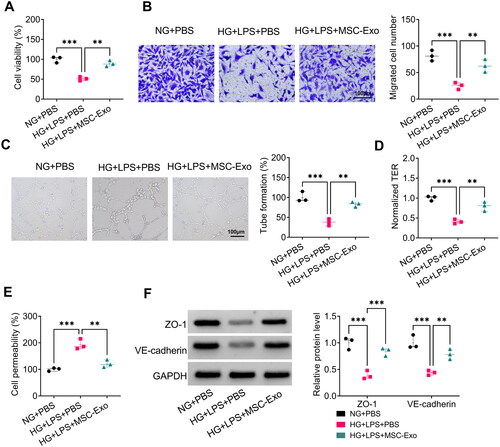
MSC-Exo mitigated HG + LPS-induced HPMECs inflammation and ferroptosis
Then, we tested the levels of proinflammatory cytokines and ferroptosis-related markers, and the results suggested that MSC-Exo co-culture could decrease the levels of TNF-α, IL-1β, IL-6, ROS, MDA and Fe2+, while increase the level of GSH in HG + LPS-induced HPMECs (). In addition, MSC-Exo treatment promoted the protein levels of SLC7A11 and GPX4 in HG + LPS-induced HPMECs (). The above indicated that MSC-Exo could alleviate HG + LPS-induced HPMECs injury.
Figure 5. MSC-Exo alleviated HG + LPS-induced injury in HPMECs. (A-C) The levels of TNF-α, IL-1β and IL-6 were detected by ELISA. (D-G) Ferroptosis was assessed by measuring ROS, MDA, GSH and Fe2+ levels. (H) The protein levels of SLC7A11 and GPX4 were tested by WB analysis. *p < 0.05; **p < 0.01; ***p < 0.001.
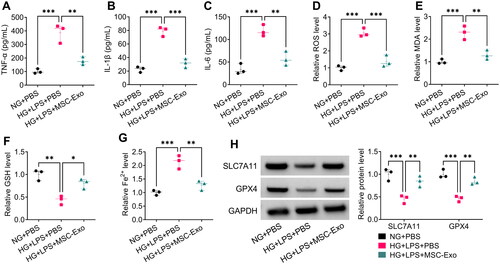
MSC-Exo activated Nrf2/HO-1 pathway in HG + LPS-induced HPMECs
Subsequently, we explored the effect of MSC-Exo on the protein expression of Nrf2 and HO-1 in HG + LPS-induced HPMECs. The protein levels of Nrf2 and HO-1 were reduced by HG + LPS treatment in HPMECs, and these effects could be mitigated by MSC-Exo (). To perform further analysis, MSC was transfected with si-Nrf2 to reduce Nrf2 protein expression (). After isolated exosome from MSC transfected with si-Nrf2 (si-Nrf2-MSC-Exo), we observed the low expression of Nrf2 in the si-Nrf2-MSC-Exo (). Then, HG + LPS-induced HPMECs were co-incubated with si-Nrf2-MSC-Exo, and we found that si-Nrf2-MSC-Exo significantly inhibited the protein levels of Nrf2 and HO-1 (). These results indicated that MSC-Exo might activate Nrf2/HO-1 pathway in HG + LPS-induced HPMECs.
Figure 6. MSC-Exo modulated the Nrf2/HO-1 pathway in HG + LPS-induced HPMECs. (A) The protein levels of Nrf2 and HO-1 were detected by WB analysis in HG + LPS-induced HPMECs co-incubated with MSC-Exo. (B) The transfection efficiency of si-Nrf2 in MSC was confirmed by WB analysis. (C) Exosome was collected from MSC transfected with si-Nrf2/si-NC, and then Nrf2 protein expression was detected by WB analysis. (D) WB analysis was used to measure the protein levels of Nrf2 and HO-1 in HG + LPS-induced HPMECs co-cultured with si-Nrf2-MSC-Exo or si-NC-MSC-Exo. *p < 0.05; **p < 0.01; ***p < 0.001.
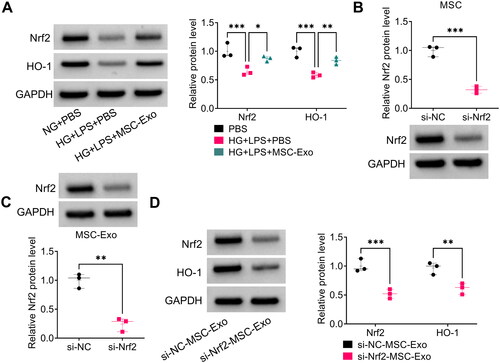
Nrf2 knockdown in MSC-Exo aggravated HG + LPS-induced HPMECs dysfunction
To demonstrate whether MSC-Exo alleviated HPMECs dysfunction by modulating Nrf2, we evaluated the effect of si-Nrf2-MSC-Exo on HG + LPS-induced HPMECs dysfunction. The results showed that si-Nrf2-MSC-Exo decreased viability, migration, angiogenesis and TER in HG + LPS-induced HPMECs (). Furthermore, si-Nrf2-MSC-Exo also could promote HPMECs permeability, while decrease the protein levels of ZO-1 and VE-cadherin under HG and LPS treatment (). Above all, MSC-Exo alleviated HG + LPS-induced HPMECs dysfunction by mediating Nrf2.
Figure 7. Effect of si-Nrf2-MSC-Exo on HG + LPS-induced dysfunction in HPMECs. HPMECs were co-incubated with si-Nrf2-MSC-Exo or si-NC-MSC-Exo and then induced using HG and LPS. Cell viability, migration, angiogenesis and TER were tested by CCK8 (A), transwell assay (B), tube formation assay (C) and TER assay (D). (E) Cell permeability was measured by FITC-dextran assay. (F) WB analysis was used to detect the protein levels of ZO-1 and VE-cadherin. *p < 0.05; **p < 0.01.
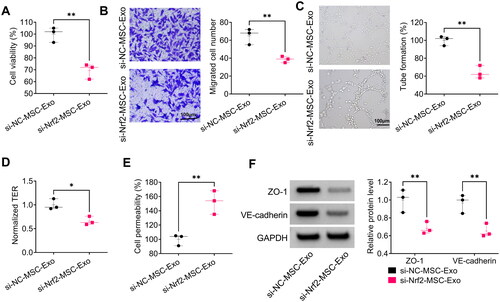
Nrf2-silenced MSC-Exo promoted HG + LPS-induced inflammation and ferroptosis in HPMECs
Moreover, si-Nrf2-MSC-Exo increased the levels of TNF-α, IL-1β, IL-6, ROS, MDA and Fe2+, while reduced GSH level in HG + LPS-induced HPMECs (). In addition, si-Nrf2-MSC-Exo also decreased the protein levels of SLC7A11 and GPX4 in HG + LPS-induced HPMECs (). These data suggested that MSC-Exo attenuated HG + LPS-induced HPMECs injury by modulating Nrf2.
Figure 8. Effect of si-Nrf2-MSC-Exo on HG + LPS-induced injury in HPMECs. (A-C) ELISA was used to detect the levels of TNF-α, IL-1β and IL-6. (D–G) Ferroptosis was assessed by measuring ROS, MDA, GSH and Fe2+ levels. (H) The protein levels of SLC7A11 and GPX4 were test by WB analysis. *p < 0.05; **p < 0.01.
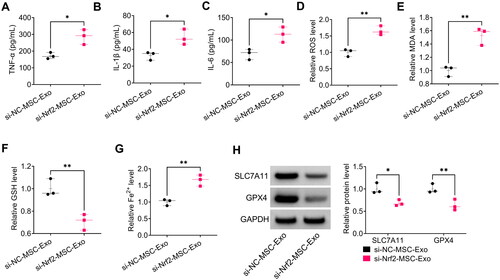
Discussion
Diabetes with sepsis is a very serious type of diabetes with infection and has become a common cause of increased mortality from diabetes [Citation1,Citation21]. Therefore, it is important to elucidate the potential mechanisms affecting diabetes complicated with sepsis for its treatment. In this study, we constructed diabetes cell models using HG-induced HPMECs and treated them with LPS to explore the effect of sepsis on the process of diabetes. The results showed that LPS could exacerbate the inhibitory effects of HG on HPMECs viability, migration, angiogenesis and TER, as well as the promotion effects on cell permeability, inflammation and ferroptosis. This data suggested that LPS could promote the injury of vascular endothelial cells induced by HG, confirming that the process of diabetes could be aggravated after secondary infection with sepsis.
More and more studies have confirmed the important role of stem cell-derived exosomes in the process of human diseases. Adipose-derived stem cells-derived exosomes (ADSCs-Exo) administration of COQ10 had been shown to improve cognitive and memory deficits in rat models of Alzheimer’s disease [Citation22]. At present, MSC has broad application prospects in the field of regenerative medicine, and their secreted exosomes have been widely used in clinical treatment of many diseases [Citation8,Citation23]. For example, MSC-Exo could reduce renal cell apoptosis and inflammation in ischemia-reperfusion acute renal injury models [Citation24]. Also, MSC-Exo alleviated osteoarthritis progression, which enhanced chondrocyte growth and improved IL-1β-induced chondrocyte injury [Citation25]. Importantly, MSC-Exo could inhibit CCl4-induced hepatocyte ferroptosis to protect against acute liver injury [Citation26], and had been shown to repress ferroptosis for alleviating hypoxia-induced myocardial injury [Citation27]. In this, we isolated exosomes from MSC and characterised them. After confirming that MSC-Exo could be taken up by HPMECs, we evaluated the effect of MSC-Exo on HG + LPS-induced cell injury. Similar to previous studies, our results showed that MSC-Exo could protect vascular endothelial cells from HG + LPS-induced injury, which could be demonstrated by co-culturing HPMECs with MSC-Exo effectively relieving cell dysfunction and inhibiting inflammation and ferroptosis under LPS + HG treatment. These results strongly support MSC-Exo as a potential biologic therapy for patients with diabetes combined with sepsis.
Nrf2 is a key factor in cellular defense against various stress injuries, and it mediates cellular inflammation, oxidative stress and apoptosis by regulating downstream HO-1 [Citation28,Citation29]. Studies had suggested that activating of Nrf2/HO-1 pathway suppressed hypoxia/reoxygenation-induced cardiomyocyte ferroptosis and oxidative stress [Citation30]. Nrf2/HO-1 pathway activated by Sirt5 could inhibit apoptosis and mitochondrial injury in cisplatin-induced acute kidney injury cell models [Citation31]. Activation of the Nrf2/HO-1 pathway might protect renal tubular epithelial cells from LPS-induced inflammation and apoptosis [Citation32]. In this, we found that Nrf2/HO-1 pathway was activated by MSC-Exo in HG + LPS-induced HPMECs. Further analysis showed that si-Nrf2-MSC-Exo co-culturing could promote HG + LPS-induced dysfunction, inflammation and ferroptosis in HPMECs, indicating that the protective effect of MSC-Exo on vascular endothelial cells was achieved by upregulating Nrf2 expression. These results provided evidence that MSC-Exo mediated HG + LPS-induced vascular endothelial cells injury by the activation of the Nrf2/HO-1 pathway.
Of course, there are some limitations to our study. At present, we have only carried out experiments at the cellular level, which cannot fully simulate the effect of MSC-Exo on the progression of diabetes with sepsis in vivo. In the future, further in vivo studies are needed to confirm the clinical therapeutic potential of MSC-Exo for diabetes complicated with sepsis. In addition, diabetes combined with sepsis can not only cause vascular endothelial cell injury, but also some other cells. In the future, the role of MSC-Exo needs to be explored in more cells.
To sum up, our study provides new evidence for the use of MSC-Exo in the treatment of diabetes combined with sepsis. Our results showed that MSC-Exo inhibited HG + LPS-induced vascular endothelial cells dysfunction, inflammation, and ferroptosis by activating Nrf2/HO-1 pathway (). These findings are expected to provide new ideas for the treatment of diabetes with sepsis.
Disclosure statement
No potential conflict of interest was reported by the author(s).
Additional information
Funding
References
- Frydrych LM, Bian G, O'Lone DE, et al. Obesity and type 2 diabetes mellitus drive immune dysfunction, infection development, and sepsis mortality. J Leukoc Biol. 2018;104(3):1–10.
- Costantini E, Carlin M, Porta M, et al. Type 2 diabetes mellitus and sepsis: state of the art, certainties and missing evidence. Acta Diabetol. 2021;58(9):1139–1151.
- de Souza Stork S, Hubner M, Biehl E, et al. Diabetes exacerbates sepsis-induced neuroinflammation and brain mitochondrial dysfunction. Inflammation. 2022;45(6):2352–2367.
- Jiang L, Cheng M. Impact of diabetes mellitus on outcomes of patients with sepsis: an updated systematic review and meta-analysis. Diabetol Metab Syndr. 2022;14(1):39.
- Kantroo V, Atreja A, Bhattacharya S, et al. The diabetic lung. J Pak Med Assoc. 2023;73:191–192.
- Kumar V. Pulmonary innate immune response determines the outcome of inflammation during pneumonia and sepsis-associated acute lung injury. Front Immunol. 2020;11:1722.
- Harrell CR, Jovicic N, Djonov V, et al. Mesenchymal stem cell-derived exosomes and other extracellular vesicles as new remedies in the therapy of inflammatory diseases. Cells. 2019;8(12):1605.
- Shen Z, Huang W, Liu J, et al. Effects of mesenchymal stem cell-derived exosomes on autoimmune diseases. Front Immunol. 2021;12:749192.
- Cui C, Zang N, Song J, et al. Exosomes derived from mesenchymal stem cells attenuate diabetic kidney disease by inhibiting cell apoptosis and epithelial-to-mesenchymal transition via miR-424-5p. FASEB J. 2022;36:e22517.
- Teng L, Maqsood M, Zhu M, et al. Exosomes derived from human umbilical cord mesenchymal stem cells accelerate diabetic wound healing via promoting M2 macrophage polarization, angiogenesis, and collagen deposition. Int J Mol Sci. 2022;23(18):10421.
- Wang X, Liu D, Zhang X, et al. Exosomes from adipose-derived mesenchymal stem cells alleviate sepsis-induced lung injury in mice by inhibiting the secretion of IL-27 in macrophages. Cell Death Discov. 2022;8(1):18.
- Li J, Jiang R, Hou Y, et al. Mesenchymal stem cells-derived exosomes prevent sepsis-induced myocardial injury by a CircRTN4/miR-497-5p/MG53 pathway. Biochem Biophys Res Commun. 2022;618:133–140.
- Jiang X, Stockwell BR, Conrad M. Ferroptosis: mechanisms, biology and role in disease. Nat Rev Mol Cell Biol. 2021;22(4):266–282.
- Mou Y, Wang J, Wu J, et al. Ferroptosis, a new form of cell death: opportunities and challenges in cancer. J Hematol Oncol. 2019;12(1):34.
- Dodson M, Castro-Portuguez R, Zhang DD. NRF2 plays a critical role in mitigating lipid peroxidation and ferroptosis. Redox Biol. 2019;23:101107.
- Yang J, Mo J, Dai J, et al. Cetuximab promotes RSL3-induced ferroptosis by suppressing the Nrf2/HO-1 signalling pathway in KRAS mutant colorectal cancer. Cell Death Dis. 2021;12(11):1079.
- Dong H, Qiang Z, Chai D, et al. Nrf2 inhibits ferroptosis and protects against acute lung injury due to intestinal ischemia reperfusion via regulating SLC7A11 and HO-1. Aging. 2020;12(13):12943–12959.
- Ma H, Wang X, Zhang W, et al. Melatonin suppresses ferroptosis induced by high glucose via activation of the Nrf2/HO-1 signaling pathway in type 2 diabetic osteoporosis. Oxid Med Cell Longev. 2020;2020:9067610–9067618.
- Tang X, Liu J, Yao S, et al. Ferulic acid alleviates alveolar epithelial barrier dysfunction in sepsis-induced acute lung injury by activating the Nrf2/HO-1 pathway and inhibiting ferroptosis. Pharm Biol. 2022;60(1):2286–2294.
- Liu J, Huang J, Zhang Z, et al. Mesenchymal stem cell-derived exosomes ameliorate delayed neurocognitive recovery in aged mice by inhibiting hippocampus ferroptosis via activating SIRT1/Nrf2/HO-1 signaling pathway. Oxid Med Cell Longev. 2022;2022:3593294–3593222.
- Balintescu A, Lind M, Franko MA, et al. Glycemic control and risk of sepsis and subsequent mortality in type 2 diabetes. Diabetes Care. 2022;45(1):127–133.
- Sheykhhasan M, Amini R, Soleimani Asl S, et al. Neuroprotective effects of coenzyme Q10-loaded exosomes obtained from adipose-derived stem cells in a rat model of Alzheimer’s disease. Biomed Pharmacother. 2022;152:113224.
- Guo M, Yin Z, Chen F, et al. Mesenchymal stem cell-derived exosome: a promising alternative in the therapy of Alzheimer’s disease. Alzheimers Res Ther. 2020;12(1):109.
- Huang J, Cao H, Cui B, et al. Mesenchymal stem cells-derived exosomes ameliorate ischemia/reperfusion induced acute kidney injury in a porcine model. Front Cell Dev Biol. 2022;10:899869.
- Liu Y, Lin L, Zou R, et al. MSC-derived exosomes promote proliferation and inhibit apoptosis of chondrocytes via lncRNA-KLF3-AS1/miR-206/GIT1 axis in osteoarthritis. Cell Cycle. 2018;17(21–22):2411–2422.
- Lin F, Chen W, Zhou J, et al. Mesenchymal stem cells protect against ferroptosis via exosome-mediated stabilization of SLC7A11 in acute liver injury. Cell Death Dis. 2022;13(3):271.
- Song Y, Wang B, Zhu X, et al. Human umbilical cord blood-derived MSCs exosome attenuate myocardial injury by inhibiting ferroptosis in acute myocardial infarction mice. Cell Biol Toxicol. 2021;37(1):51–64.
- Chen Z, Zhong H, Wei J, et al. Inhibition of Nrf2/HO-1 signaling leads to increased activation of the NLRP3 inflammasome in osteoarthritis. Arthritis Res Ther. 2019;21(1):300.
- Dang X, He B, Ning Q, et al. Alantolactone suppresses inflammation, apoptosis and oxidative stress in cigarette smoke-induced human bronchial epithelial cells through activation of Nrf2/HO-1 and inhibition of the NF-kappaB pathways. Respir Res. 2020;21(1):95.
- Liu XJ, Lv YF, Cui WZ, et al. Icariin inhibits hypoxia/reoxygenation-induced ferroptosis of cardiomyocytes via regulation of the Nrf2/HO-1 signaling pathway. FEBS Open Bio. 2021;11(11):2966–2976.
- Li W, Yang Y, Li Y, et al. Sirt5 attenuates cisplatin-induced acute kidney injury through regulation of Nrf2/HO-1 and bcl-2. Biomed Res Int. 2019;2019:4745132–4745111.
- Chen F, Zhu J, Wang W. Ulinastatin attenuates LPS-induced inflammation and inhibits endoplasmic reticulum stress-induced apoptosis in renal tubular epithelial cells via regulation of the TLR4/NF-kappaB and Nrf2/HO-1 pathways. Inflammation. 2021;44(6):2323–2332.