Abstract
Background
The Coronavirus disease 2019 (COVID-19) pandemic has brought a heavy burden to the world, interestingly, it shares many clinical symptoms with systemic lupus erythematosus (SLE). It is unclear whether there is a similar pathological process between COVID-9 and SLE. In addition, we don’t know how to treat SLE patients with COVID-19. In this study, we analyse the potential similar pathogenesis between SLE and COVID-19 and explore their possible drug regimens using bioinformatics and systems biology approaches.
Methods
The common differentially expressed genes (DEGs) were extracted from the COVID-19 datasets and the SLE datasets for functional enrichment, pathway analysis and candidate drug analysis.
Result
Based on the two transcriptome datasets between COVID-19 and SLE, 325 common DEGs were selected. Hub genes were identified by protein-protein interaction (PPI) analysis. few found a variety of similar functional changes between COVID-19 and SLE, which may be related to the pathogenesis of COVID-19. Besides, we explored the related regulatory networks. Then, through drug target matching, we found many candidate drugs for patients with COVID-19 only or COVID-19 combined with SLE.
Conclusion
COVID-19 and SLE patients share many common hub genes, related pathways and regulatory networks. Based on these common targets, we found many potential drugs that could be used in treating patient with COVID-19 or COVID-19 combined with SLE.
1. Introduction
As of October 2023, the global COVID-19 pandemic burden is estimated to have reached 600 million cases, with large outbreaks occurring in different countries [Citation1]. The development of severe COVID-19 is due to immune dysregulation [Citation2,Citation3], which causes a variety of pulmonary and extrapulmonary manifestations, leading to respiratory failure and multi-organ failure [Citation4].
Systemic lupus erythematosus (SLE) is a chronic immune-mediated rheumatologic disease (IMRD) with various clinical manifestations and complex pathogenesis [Citation5]. The incidence of SLE in France is approximately 0.3–5.1 per 100,000 persons per year, and the prevalence is 6.5–85 per 100,000 persons per year [Citation6]. Many patients require long-term treatment with nonsteroidal anti-inflammatory drugs, immunosuppressants, or biologic agents [Citation7].
Many clinical manifestations of COVID-19 are similar to those of SLE, includes autoantibody production, immune cell dysfunction, joint pain, myocarditis and interstitial pneumonia [Citation8–14]. Here are many case reports about patients who developed SLE after being infected with COVID-19 [Citation15]. We suggest that there may be similar pathological processes between COVID-19 and SLE. Interestingly, these SLE patients was found to be resist to severe COVID-19 [Citation16], this may be bonus from the long-term immunosuppressive drug use [Citation17]. controversies on whether SLE patients should continue medication after COVID-19 are always existed [Citation16,Citation18,Citation19]. Moreover, lacking of effective and safe drugs for patients with COVID-19, especially for those patients with COVID-19 plus SLE, undoubtedly hinders the treatment.
In this study, we used bioinformatics analysis and statistical sorting to identify the common differentially expressed genes between COVID-19 and SLE patients. We enriched the biological pathways and related transcriptional regulation. In addition, we identified potential treatment options for COVID-19 patients with SLE.
2. Methods
2.1. Data acquisition
In this study, the transcriptome data of peripheral blood samples from COVID-19 patients and SLE patients were collected from the GEO data platform. Three COVID-19 databases were used in this study. Their GEO accession ids were GSE215865, GSE196822 and GSE211979. Using GSE215865 database, a total of 64 COVID-19 nucleic acid test negative samples and 345 COVID-19 nucleic acid test positive samples were obtained after excluding duplicate samples [Citation20]. The database GSE196822 contains the mRNA high-throughput sequencing results of peripheral blood samples from 9 healthy volunteers and 34 Indian patients with COVID-19 of different severity [Citation21]. The database GSE211979 contains the transcriptome sequencing results of peripheral blood samples from 5 control subjects and 16 COVID-19 patients within 72 h after diagnosis [Citation22]. The SLE patient databases used in this study include GSE112087 and GSE211700, which contain the transcriptome test results of peripheral blood samples from 31 SLE patients and 21 healthy controls and 10 healthy people and 20 SLE patients respectively [Citation23,Citation24].
2.2. Identification of common differentially expressed genes (DEGs)
Used the "DESeq2" and the "edgeR" package in R software (4.2.1), for three COVID − 19 data sets and two SLE dataset, |Log2 Fold Change|>0.585 and |adj.P.Val.|<0.05 as the execution criterion to find the respective DEGs for each dataset. The "venn" package in R software (4.2.1) was used to screen out DEGs that appeared in at least two databases in the COVID-19 dataset and differentially expressed DEGs in the two SLE datasets. The DEGs selected from COVID-19 data and the intersection DEGs of SLE were analysed to screen out the common DEGs that were differentially expressed and up-regulated or down-regulated in the two diseases.
2.3. Functional and pathway enrichment analysis of common DEGs
To reveal the functions of DEGs, Gene Ontology (GO) enrichment analysis and Kyoto Encyclopaedia of Genes and Genomes (KEGG) pathway were performed using Enrichr database analysis. The "clusterProfiler" package in R software (4.2.1) was used to screen out the common DEGs for potential biological functions and physiological pathways. The GO terms were composed of the following three parts: biological process (BP), cellular component (CC) and molecular function (MF), and the p-value < 0.1 and Q values < 0.25 was used as a standardised measure.
2.4. Analysis of protein-protein interaction (PPI) network
PPI is the study of how proteins work together in cells to perform cellular functions in a coordinated manner. Studying PPI can provide a better understanding of molecular mechanisms in cells and is used for various biological analyses [Citation25]. The search tool (STRING) used to retrieve interacting genes is a database used to analyse functional protein association networks [Citation26]. The selected DEGs were submitted to the STRING database for searching the interacting genes, and the functional protein association network was analysed [Citation26], and all the composite scores > 0.4 were extracted.
2.5. Extraction of hub genes
Based on the PPI network results, all nodes in the PPI network were calculated by the Cytoscape (3.9.1) plug-in cytoHubba [Citation27]. In this analysis, the genes with the highest result ranking values were considered as hub genes, which are essential to ensure the stability of the entire network. Cytoscape software (3.9.1) was used for visualisation.
2.6. Screening for regulating transcription factors (TFs) and miRNAs
TFs play a central role in controlling many biological processes when cells recognise various internal and external stimuli and respond appropriately, thus ensuring the correct expression of specific genes [Citation28,Citation29]. ChEA3 is a web-based tool for TFs enrichment analysis, based on the overlap between given lists of differentially expressed genes, and previously annotated TF targets assembled from multiple resources. We screened TFs using ChEA3 and selected the top 10 based on their average intergrated ranks across libraries. The miRNA plays a role in post-transcriptional regulation of target gene expression in fundamental biological processes [Citation30]. We performed the search of miRNAs on miRNet, a miRNA-centric network analysis platform, with Betweenness as the criterion and ranking for screening. Cytoscape software (3.9.1) was used for visualisation.
2.7. Matching of drug candidates
The DGIdb network platform was used to screen common DEGs related drugs and query gene interactions of SLE basic drugs.
3. Result
3.1. Identification of DEGs and common DEGs between COVID-19 and SLE
We have drawn this flow chart () to show the processing and key steps of the dataset in this study. In order to explore whether there is a similar pathogenesis or pathological mechanism between COVID-19 and SLE, we searched and downloaded the microarray dataset of transcriptome results of peripheral blood samples from COVID-19 and SLE patients from the GEO database, and sorted out and analysed their respective DEGs. The results show that three data sets for COVID-19. There were 965, 3934 and 5237 genes in GSE211979, GSE215865, GSE196822, respectively, satisfying the two conditions: |Log2 Fold Change|>0.585 and |adj.P.Val|<0.05. In SLE database, GSE112087 and GSE211700, 1642 and 5797 genes were screened out under the conditions of |Log2 Fold Change|>0.585 and |adj.P.Val|<0.05, respectively (). The volcano map shows 5 datasets DEG for COVID-19 and SLE, respectively, and the labelled genes are the 15 up-regulated genes and 15 down-regulated genes with the largest change multiples in each dataset (, Supplemental Table S1–S5). Because the high variability of novel coronavirus may lead to differences in gene expression of different subtypes, genes with different expression in at least two COVID-19 datasets were selected as representative genes for novel coronavirus, while the intersection of DEG in two databases was selected for SLE data. Cross-analysis results shown in the Venn diagram indicated that a total of 2418 COV-2 representative genes and 747 SLE representative genes were found (), and 325 common DEG genes were finally identified as the target gene set for our study (). The specific genetic information and trends are shown in the Supplementary Table S6, suggesting that SLE and COVID-19 have a large amount of DEG, and there may be a certain correlation.
Figure 2. Visualisation the number of common differentially expressed genes between two datasets, COVID-19 and SLE. (A) Comparing the number of differentially expressed genes between COVID-19 and SLE. (B) The volcano plot of differentially expressed genes in 5 datasets. (C) Venn plot of the results of cross-analysis of the three COVID-19 data sets. (D) Venn plot of the cross-analysis results of the 2 SLE datasets. (E) Venn diagram showing the overlap of differentially expressed genes between COVID-19 and SLE.
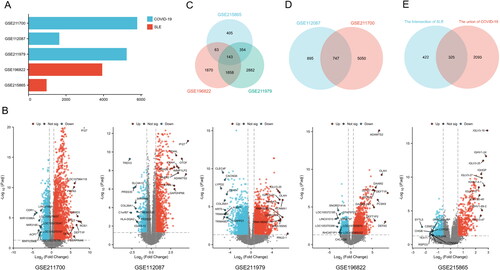
Table 1. Matching of drug candidates.
3.2. Functional enrichment analysis of common DEGs
To gain more insight into the biological roles of these 325 common DEGs, GO annotation and KEGG pathway enrichment analysis were performed (Supplementary Table S7). In , the common functional pathways in the enrichment results are shown. In BP, the p values of "defense response to virus," "defense response to symbiont" and "response to virus" were small and related to virus infection and defense. In addition, "response to type I interferon," "response to interferon-beta" and "regulation of innate immune" were used response "and other regulatory related pathways related to innate or adaptive immunity were also enriched. In CC, "specific granule," "secretory granule lumen," "cytoplasmic vesicle lumen," and "vesicle lumen" showed the most prominent structural and functional changes related to cellular endocytosis or secretion (). KEGG enrichment results showed that "Influenza A," "Epstein–Barr virus infection," "Measles" and "Hepatitis C" reflected the characteristics of multiple viral infection routes ().
3.3. PPI network and hub genes of common DEGs
The shows the architecture of the interaction network between the common DEGs of SLE and COVID-19. The shows the results of the top 20 hub genes screening and ranking based on DEGs interactions, Including PBK, NEK2, KIF20A, KIF11, CDK1, CDC45, CDC20, BUB1B, BUB1, BIRC5, AURKB, KIF2C, KIF15, RRM2, DLGAP5, TPX2, CDCA5, ANLN, KIFC1, and CCNB2. Targeting these core genes can be used to explore therapeutic options for COVID-19 (Supplementary Table S8).
3.4. Construction of regulatory networks
In order to identify the main common variation at the transcription level of the two diseases and further explore the similar pathological molecular mechanism of the two diseases, we constructed the interaction network of two types of gene expression regulators: transcription factors (TFs) and miRNAs, and the results are shown in Supplementary Tables S8 and S9. The 10 regulatory TFS with the most significant correlation with the common DEGs, including FOXM1, CENPA, ZNF367, PLSCR1, STAT1, DNMT1, E2F7, TFDP1, BATF2 and MXD3, are shown in for their primary interactions with the common DEGs. The top 10 miRNAs with the highest correlation scores, including hsa-mir-124-3p, hsa-mir-129-2-3p, hsa-mir-1-3p, hsa-mir-16-5p, hsa-mir-34a-5p, hsa-mir-27a-3p, hsa-mir-107, hsa-mir-155-5p, hsa-mir-146a-5p and hsa-mir-103a-3p, and their interactions with common DEGs are shown in . In essence, there was a strong interaction between them and DEGs.
3.5. Matching potential drugs
Among the matched drugs, we screened the drugs that had entered the clinical stage IV and ranked them by the number of common DEGs. shows the top 12 drugs (METHOTREXATE, CISPLATIN, DOXORUBICIN, FLUOROURACIL, CELECOXIB, INDOMETHACIN, FLUTAMIDE, CAPECITABINE, DOXYCYCLINE, IRINOTECAN, PACLITAXEL, and TESTOSTERONE). The study also retrieved the effects of drugs commonly used in SLE patients on the hub genes matched by COVID-19 targets. shows the top 30 hub genes in COVID-19 representative genes as well as their primary interaction genes. contains 10 commonly used drugs for SLE that are closely related to COVID-19 differential genes and their target genes in COVID-19 and SLE.
Figure 8. Identification of the top 30 hub genes of DEGs of COVID-19 ranked by their scores. In the middle circle, the central genes represent the top 30 hub genes ranked by their scores and their interactions with other DEGs of COVID-19.
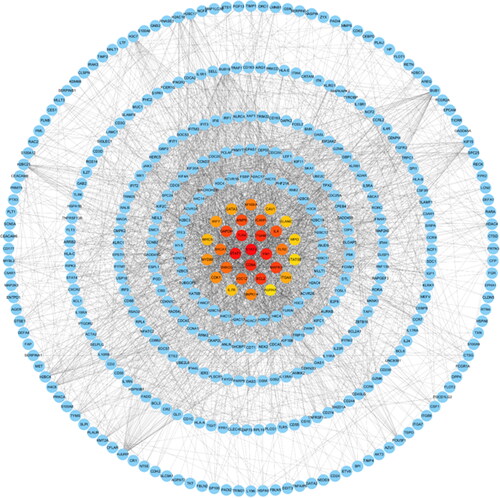
Table 2. Target genes of SLE drugs in COVID-19 and SLE.
4. Discussion
The COVID-19 pandemic caused by SARS-CoV-2 infection has placed a huge burden on the world. Despite the urgent need for effective treatment strategies, there is still no specific antiviral treatment for COVID-19, and guidelines vary from country to country [Citation31]. In clinical practice, many patients with SLE have been found to have a more complicated situation after being complicated with COVID-19 [Citation32]. The mortality rate of SLE patients with underlying diseases was 1.52 to 1.98 times higher than that of the general population [Citation33]. The most common cause of death was infection, accounting for 66% of all deaths, and pneumonia was the most common infection in patients with SLE [Citation34]. This may be caused by the impairment of the innate immune system of the disease, or it may be related to the application of glucocorticoids or immunosuppressants [Citation35,Citation36]. Based on the above ideas, SLE patients have multiple risk factors for more severe COVID-19. In fact, many studies have found no significant difference in the prevalence or severity of COVID-19 in patients with SLE compared with the general population [Citation37–39], and immunosuppressive drugs do not appear to increase the risk or severity of COVID-19 [Citation40]. Some studies have even suggested that SLE is a protective factor for the reduction of ICU treatment in COVID-19 [Citation16], and some SLE drugs can reduce the risk of severe COVID-19 [Citation18]. In the face of such controversial issues, this study attempts to explore whether there is a correlation between SLE and COVID-19 in the molecular mechanism of the disease and to provide ideas for the research of treatment through experimental evidence based on the transcriptional level.
Our results showed that 325 common DEGs were present in both SLE and COVID-19. Based on PPI network, hub genes (PBK, NEK2, KIF20A, KIF11, CDK1, CDC45, CDC20, BUB1B, BUB1, BIRC5, AURKB, KIF2C, KIF15, RRM2, DLGAP5, TPX2, CDCA5, ANLN, KIFC1 and CCNB2) were calculated. Hub genes and their corresponding hub proteins can be used as effective reference targets for disease treatment or intervention. BIRC5 is a mitotic spindle checkpoint gene and plays an important role in tumorigenesis, metastasis and drug resistance [Citation41]. BIRC5 is positively correlated with the activation of CD56 natural killer cells and CD4 T cells, and plays a role in immunity [Citation42]. The above evidence supports the potential of these hub genes as key targets for the treatment of COVID-19 or rheumatic diseases. Some studies have suggested that PBK (PDZ-binding kinase) plays a regulatory role in cell cycle regulation and cell mitosis [Citation43]. Excessive activation of PBK promotes inflammation and proliferation of tumour cells by activating downstream signalling cascades and transcription factors [Citation44]. KIF20AKIF20A encodes a protein with protein kinase binding activity that regulates microbundle formation, midbody shedding, and cell division. It is upregulated in a variety of cancers and can regulate cell proliferation and metastasis by activating JAK/STAT3 pathway [Citation45]. CDK1 is a cyclin-dependent kinase that regulates cell cycle progression. Several viruses can control CDK expression to create a suitable cellular environment for virus replication [Citation46]. BIRC5 is a mitotic spindle checkpoint gene and a well-known cancer therapeutic target [Citation41]. BIRC5 promotes cell proliferation, migration and invasion through the β-catenin pathway, transforming growth factor-β (TGF-β) pathway and PI3K/Akt pathway, respectively [Citation47].
In the past, a number of studies have found that SLE and COVID-19 have similar pathogenesis, such as interferon production, elevated autoantibody titres, immune system disorders, and the formation of NETs [Citation5,Citation12,Citation48–50]. GO annotation and KEGG functional enrichment were used to explore the similar pathogenesis pathways between them. The results showed that the BP of common DEGs in the two diseases were mainly concentrated in antiviral and immune regulation, especially for antiviral response, cell cycle regulation, type I interferon production and regulation, neutrophil and T cell regulation, etc. Some studies have suggested that type I interferon is one of the main pathogenic factors of SLE [Citation51]. And SLE patients with high evidence of type I interferon are more active and more likely to develop nephritis and other serious manifestations [Citation51]. And at least 10% of patients with life-threatening COVID-19 have the auto-Abs neutralise the ability of the corresponding type I interferon [Citation52]. These results suggest that the abnormal production and function of type I interferon may play an important role in the pathogenesis of these two diseases. With regard to neutrophils, this is consistent with the discovery of MPO in hub genes. Neutrophil counts are found to be elevated in patients with COVID-19 [Citation53], and it has also been suggested that neutrophils may play an important role in the response to viral diseases through NETs and protect the host during the viral response by capturing and eliminating different pathogens [Citation54,Citation55]. T cells are a double-edged sword in COVID-19, with both positive and negative effects [Citation56,Citation57]. At present, the treatment of COVID-19 includes both adoptive T cell therapy and activated T cell response, as well as Th1 activator and Th17 blocker used in clinical trials [Citation58–61]. More clinical and molecular experiments are needed to verify this in the future. Interestingly, in BP, multiple pathways related to antiviral invasion were also enriched, which were also reflected in KEGG enrichment results, which may indicate that the occurrence of SLE may be related to viral infection or immune response after infection. Some studies have also proposed the same view [Citation62,Citation63], which can help us to better explore the pathogenesis and aetiology of SLE. By matching the enriched pathways in the differential genes of SLE and COVID-19, we found that the two diseases not only have similar functional pathways, but also have the same regulatory changes in the process of the disease, which further supports our conjecture that the two diseases have similar pathogenesis.
To better understand the pathogenesis of COVID-19 and its similar pathological processes with SLE, we also established a regulatory network of common DEGs by retrieving related TFs and miRNAs. TFs are proteins that coordinate gene expression in a specific cell type in a spatial and temporal manner [Citation64]and can act simultaneously on multiple PPIs through their disordered structural features to regulate biological processes [Citation65]. The miRNAs are small non-coding RNAs that regulate gene expression by recognising homologous sequences and interfering with transcription, translation or epigenetic processes [Citation66]. Among the TFs screening results, FOXM1 was found to regulate phosphorylated STAT3 to affect pulmonary fibrosis [Citation67]. STAT1 can bind to other TFs to form an interferon gene stimulator [Citation68]. TFDP1 was identified in animal models as one of the components of the virus acting on the host complex [Citation69]. And IRF7 is involved in the reprogramming of macrophage inflammation by spike proteins in SARS-CoV-2 infection [Citation70]. Among the top miRNAs in the network, hsa-mir-146a-5p was correlated with IFI44, and it was positively correlated with activated dendritic cells [Citation71]. And Studies have found that miR-124-3p is involved in the regulation of macrophage polarisation, thereby changing cell proliferation [Citation72]. All these regulators can be investigated as possible targets for the treatment of COVID-19, but most of them are still unclear of relevance to COVID-19 and need to be further verified by large number of experiments.
It is worth noting that SLE patients are accompanied by immune abnormalities [Citation73]. At present, there is no specific anti COVID-19 drug for SLE patients, so it is necessary to develop drugs targeting SLE patients with SARS-CoV-2 infection. Using the common DEGs in SLE and COVID-19 as the target, we successfully found many compounds and drugs have the potential to treat SLE patients with SARS-CoV-2 infection. Cisplatin is the first platinum complex approved by the US FDA and is currently widely used in the treatment of malignant tumours [Citation13]. As metal-based compounds, some studies have identified their antiviral properties relative to enveloped viruses, and extrapolating them to COVID-19 could be an effective way to manipulate the resistance and mutation problems faced by current anti-COVID-19 drugs [Citation74]. SARS-CoV-2 proteome analysis suggested 0.8-fold enrichment or depletion of cysteine residues in the functional domain. However, the enrichment of arginine residues was 4.6-fold, indicating that SARS-CoV-2 is resistant to oxidants and sensitive to methylglyoxal (MG) [Citation75]. doxorubicin can increase glucose metabolism by increasing the expression of glucose transporters GLUT1 and hexokinase-2, leading to the formation of MG [Citation76]. With regard to Fluorouracil, some scholars have summarised its research in COVID-19 and proposed targeted experimental scheme suggestions, suggesting that fluorouracil has certain therapeutic potential [Citation77].
Nest, we evaluated the potential of drugs for SLE in the treatment of COVID-19. Interestingly, we found a strong intersection between targets of SLE drugs and COVID-19 DEGs (). Many reports have indicated that methotrexate has certain protective effects on SARS-CoV-2 infection via downregulating ACE2 [Citation78]. According to increasing evidence, Dexamethasone reduces the severity of inflammation and prevent severe respiratory complications in COVID-19 patients [Citation79,Citation80]. The use of azathioprine in COVID-19 is controversial, with some studies indicating that the proportion of patients with severe COVID-19 is significantly higher with combination therapy and thiopurine monotherapy than with TNF antagonist monotherapy [Citation81]. Azathioprine should be used with great caution in patients with clinical infection. Cyclosporine exhibit substantial anti-SARS-CoV-2 antiviral activity [Citation82]. The use of prednisone is controversial, and some clinical investigations have found that prednisone can reduce the risk of hospitalisation in patients with COVID-19 by 50 to 60% [Citation83]. And one study of long-term olfactory dysfunction in COVID-19 patients found that the use of steroids, including prednisone, can reduce the occurrence of this condition [Citation84]. However, some studies have proposed that the dose of prednisone is positively correlated with a higher risk of hospitalisation in SLE patients complicated with COVID-19 [Citation18]. Similar investigations have found that even low doses of prednisone are associated with severe outcomes [Citation85]. In COVID-19 patients with acute kidney injury and acute respiratory distress syndrome, methylprednisolone has shown to reduced risk of coronavirus death [Citation86]. In the early days of the COVID-19 pandemic, hydroxychloroquine had high hopes. However, several large randomised trials have not shown a mortality reduction or other clinical benefit of hydroxychloroquine among hospitalised patients with COVID-19 [Citation87–89]. It is possible that early reports of positive effects of hydroxychloroquine were biased and side effects were overlooked [Citation90]. However, we should not ignore that chloroquine may not directly kill the virus, but may indirectly benefit patients by regulating the immune system [Citation91,Citation92]. Experimentally, tacrolimus has been shown to strongly inhibit the growth of human coronavirus SARS-CoV, human CoV-NL63, and human CoV-229E [Citation93]. Clinically, there have also been cases that tacrolimus may be effective in the treatment of interstitial lung disease after COVID-19 in the acute phase. However, it does not prevent the progression of pulmonary fibrosis [Citation94]. One caveat, however, is that SARS-CoV-2 infection may increase serum tacrolimus levels, which may cause side effects [Citation95]. Therefore, tacrolimus should be used with caution, and serum tacrolimus levels should be measured if necessary. Our findings may provide the basis for novel therapeutic strategies that improves the anti-SARS-CoV-2 treatment in SLE patients.
However, the above conclusions are only based on the relevant results obtained from our bioinformatics calculation and statistical analysis. To discuss how to reduce the severe disease rate and mortality of COVID-19 patients in the acute outbreak period, many relevant clinical trials or molecular experiments are still in progress or need further exploration. Future long-term cohort studies are needed to validate this hypothesis and evaluate the specific therapeutic effects and safety of potential drugs in the two diseases.
5. Conclusion
Based on transcriptional level evidence, this study analysed the common DEGs of COVID-19 and SLE, explored the signalling pathways, hug genes and regulatory networks, and confirmed that the two diseases had similar pathogenesis to a certain extent. On this basis, small molecular compound drugs were screened to provide potential molecular targets for the treatment of COVID-19 or SLE combined with COVID-19, hoping to help the formulation and development of precision treatment drug regimens for relevant patients.
Authors’ contributions
YLW and YHL wrote the manuscript, XFB and YL provided the ideas for the study, YLW, YHL, and YZ performed the data collection, analysis, and interpretation of the results, XFB provided guidance on the drug screening, and XFB and YL jointly supervised the preparation of the manuscript.
Supplemental Material
Download Zip (1.4 MB)Disclosure statement
The authors declare that the research was conducted in the absence of any commercial or financial relationships that could be construed as a potential conflict of interest.
Additional information
Funding
References
- Center for Systems Science and Engineering at Johns Hopkins University: COVID-19 dashboard; 2022. Available from: https://coronavirus.jhu.edu/map.html.
- Gustine JN, Jones D. Immunopathology of hyperinflammation in COVID-19. Am J Pathol. 2021; Jan191(1):1–14. PubMed PMID: 32919977; PubMed Central PMCID: PMCPMC7484812. eng. doi:10.1016/j.ajpath.2020.08.009
- To KK, Sridhar S, Chiu KH, et al. Lessons learned 1 year after SARS-CoV-2 emergence leading to COVID-19 pandemic. Emerg Microb Infect. 2021; Dec10(1):507–535. PubMed PMID: 33666147; PubMed Central PMCID: PMCPMC8006950. eng. doi:10.1080/22221751.2021.1898291
- Chan JF, Yuan S, Kok KH, et al. A familial cluster of pneumonia associated with the 2019 novel coronavirus indicating person-to-person transmission: a study of a family cluster. Lancet (London, England). 2020; Feb 15395(10223):514–523. PubMed PMID: 31986261; PubMed Central PMCID: PMCPMC7159286. eng. doi:10.1016/S0140-6736(20)30154-9
- Kiriakidou M, Ching CL. Systemic lupus erythematosus. Ann Internal Med. 2020; Jun 2172(11):Itc81–itc96. PubMed PMID: 32479157; eng. doi:10.7326/AITC202006020
- Arnaud L, Fagot JP, Mathian A, et al. Prevalence and incidence of systemic lupus erythematosus in France: a 2010 nation-wide population-based study. Autoimmun Rev. 2014; Nov13(11):1082–1089. PubMed PMID: 25172239; eng. doi:10.1016/j.autrev.2014.08.034
- Fortuna G, Brennan MT. Systemic lupus erythematosus: epidemiology, pathophysiology, manifestations, and management. Dental Clin N Am. 2013; Oct57(4):631–655. PubMed PMID: 24034070; eng. doi:10.1016/j.cden.2013.06.003
- Reyes Gil M, Barouqa M, Szymanski J, et al. Assessment of lupus anticoagulant positivity in patients with coronavirus disease 2019 (COVID-19). JAMA Netw Open. 2020; Aug 33(8):e2017539. PubMed PMID: 32785632; eng. doi:10.1001/jamanetworkopen.2020.17539
- Pascolini S, Vannini A, Deleonardi G, et al. COVID-19 and immunological dysregulation: can autoantibodies be useful? Clin Transl Sci. 2021; Mar14(2):502–508. PubMed PMID: 32989903; PubMed Central PMCID: PMCPMC7536986. eng. doi:10.1111/cts.12908
- Berzuini A, Bianco C, Paccapelo C, et al. Red cell-bound antibodies and transfusion requirements in hospitalized patients with COVID-19. Blood. 2020; Aug 6136(6):766–768. PubMed PMID: 32559762; PubMed Central PMCID: PMCPMC7414594. eng. doi:10.1182/blood.2020006695
- Oliviero B, Varchetta S, Mele D, et al. Expansion of atypical memory B cells is a prominent feature of COVID-19. Cell Mol Immunol. 2020; Oct17(10):1101–1103. PubMed PMID: 32879471; PubMed Central PMCID: PMCPMC7463104. eng. doi:10.1038/s41423-020-00542-2
- Zuo Y, Yalavarthi S, Shi H, et al. Neutrophil extracellular traps in COVID-19. JCI Insight. 2020; Jun 4;5(11). PubMed PMID: 32329756; PubMed Central PMCID: PMCPMC7308057. eng. doi: 10.1172/jci.insight.138999.
- Borges do Nascimento IJ, von Groote TC, O’Mathúna DP, et al. Clinical, laboratory and radiological characteristics and outcomes of novel coronavirus (SARS-CoV-2) infection in humans: a systematic review and series of meta-analyses. PLoS One. 2020;15(9):e0239235. PubMed PMID: 32941548; PubMed Central PMCID: PMCPMC7498028 eng. doi:10.1371/journal.pone.0239235
- Conti F, Ceccarelli F, Perricone C, et al. Flare, persistently active disease, and serologically active clinically quiescent disease in systemic lupus erythematosus: a 2-year follow-up study. PLoS One. 2012;7(9):e45934. PubMed PMID: 23029327; PubMed Central PMCID: PMCPMC3448702. eng. doi:10.1371/journal.pone.0045934
- Bonometti R, Sacchi MC, Stobbione P, et al. The first case of systemic lupus erythematosus (SLE) triggered by COVID-19 infection. Eur Rev Med Pharmacol Sci. 2020; Sep24(18):9695–9697. PubMed PMID: 33015814; eng.
- Marques CDL, Kakehasi AM, Pinheiro MM, et al. High levels of immunosuppression are related to unfavourable outcomes in hospitalised patients with rheumatic diseases and COVID-19: first results of ReumaCoV brasil registry. RMD Open. 2021; Jan7(1):e001461. PubMed PMID: 33510041; PubMed Central PMCID: PMCPMC7844930. eng. doi:10.1136/rmdopen-2020-001461
- Rúa-Figueroa I, Rúa-Figueroa D, Pérez-Veiga N, et al. Antimalarials exert a cardioprotective effect in lupus patients: insights from the spanish society of rheumatology lupus register (RELESSER) analysis of factors associated with heart failure. Semin Arthritis Rheum. 2022; Feb52:151946. PubMed PMID: 35033377; PubMed Central PMCID: PMCPMC8720299. eng. doi:10.1016/j.semarthrit.2021.11.012
- Gianfrancesco M, Hyrich KL, Al-Adely S, et al. Characteristics associated with hospitalisation for COVID-19 in people with rheumatic disease: data from the COVID-19 global rheumatology alliance physician-reported registry. Ann Rheum Dis. 2020; Jul79(7):859–866. PubMed PMID: 32471903; PubMed Central PMCID: PMCPMC7299648. eng. doi:10.1136/annrheumdis-2020-217871
- Carvalho JS, Dos Reis Neto ET, Kakehasi AM, et al. Factors associated with poor outcomes in SLE patients with COVID-19: data from ReumaCoV-Brazil register. Lupus. 2023; Jan32(1):42–53. PubMed PMID: 36300790; PubMed Central PMCID: PMCPMC9614598. eng. doi:10.1177/09612033221135884
- Thompson RS, Wilkins L, Cheng E, et al. GSE215865 2022 [updated Dec 06, 2022]. Available from:https://www.ncbi.nlm.nih.gov/geo/query/acc.cgi.
- Banerjee U, Rao P, Reddy M, et al. A 9-gene biomarker panel identifies bacterial coinfections in culture-negative COVID-19 cases. Mol Omics. 2022; Sep 2618(8):814–820. PubMed PMID: 35971789; eng. doi:10.1039/D2MO00100D
- Majumder N, Deepak V, Hadique S, et al. Redox imbalance in COVID-19 pathophysiology. Redox Biol. 2022; Oct56:102465. PubMed PMID: 36116160; PubMed Central PMCID: PMCPMC9464257. eng. doi:10.1016/j.redox.2022.102465
- Figgett WA, Monaghan K, Ng M, et al. Machine learning applied to whole-blood RNA-sequencing data uncovers distinct subsets of patients with systemic lupus erythematosus. Clin Transl Immunology. 2019;8(12):e01093. PubMed PMID: 31921420; PubMed Central PMCID: PMCPMC6946916. eng. doi:10.1002/cti2.1093
- Yang M, Wang P, Liu T, et al. High throughput sequencing revealed enhanced cell cycle signaling in SLE patients. Sci Rep. 2023; Jan 413(1):159. PubMed PMID: 36599883; PubMed Central PMCID: PMCPMC9812989. eng. doi:10.1038/s41598-022-27310-8
- Franceschini A, Szklarczyk D, Frankild S, et al. STRING v9.1: protein-protein interaction networks, with increased coverage and integration. Nucleic Acids Res. 2013; Jan41(Database issue):D808–15. (Database issue): PubMed PMID: 23203871; PubMed Central PMCID: PMCPMC3531103. eng.
- Szklarczyk D, Morris JH, Cook H, et al. The STRING database in 2017: quality-controlled protein-protein association networks, made broadly accessible. Nucleic Acids Res. 2017; Jan 445(D1):D362–d368. PubMed PMID: 27924014; PubMed Central PMCID: PMCPMC5210637. eng. doi:10.1093/nar/gkw937
- Chin CH, Chen SH, Wu HH, et al. cytoHubba: identifying hub objects and Sub-networks from complex interactome. BMC Syst Biol. 2014;8 Suppl 4(Suppl 4):S11. PubMed PMID: 25521941; PubMed Central PMCID: PMCPMC4290687. eng.
- Simon I, Barnett J, Hannett N, et al. Serial regulation of transcriptional regulators in the yeast cell cycle. Cell. 2001; Sep 21106(6):697–708. PubMed PMID: 11572776; eng. doi:10.1016/S0092-8674(01)00494-9
- Accili D, Arden KC. FoxOs at the crossroads of cellular metabolism, differentiation, and transformation. Cell. 2004; May 14117(4):421–426. PubMed PMID: 15137936; eng. doi:10.1016/S0092-8674(04)00452-0
- Bartel DP. MicroRNAs: genomics, biogenesis, mechanism, and function. Cell. 2004; Jan 23116(2):281–297. PubMed PMID: 14744438; eng. doi:10.1016/S0092-8674(04)00045-5
- Tsang HF, Chan LWC, Cho WCS, et al. An update on COVID-19 pandemic: the epidemiology, pathogenesis, prevention and treatment strategies. Expert Rev Anti Infect Ther. 2021; Jul19(7):877–888. PubMed PMID: 33306423; eng. doi:10.1080/14787210.2021.1863146
- Fernandez-Ruiz R, Paredes JL, Niewold TB. COVID-19 in patients with systemic lupus erythematosus: lessons learned from the inflammatory disease. Transl Res. 2021; Jun232:13–36. PubMed PMID: 33352298; PubMed Central PMCID: PMCPMC7749645. eng. doi:10.1016/j.trsl.2020.12.007
- Bournia VK, Fragoulis GE, Mitrou P, et al. All-cause mortality in systemic rheumatic diseases under treatment compared with the general population, 2015-2019. RMD Open. 2021; Nov7(3):e001694. PubMed PMID: 34728554; PubMed Central PMCID: PMCPMC8565571. eng. doi:10.1136/rmdopen-2021-001694
- Lai CC, Sun YS, Chen WS, et al. Risk factors for mortality in systemic lupus erythematosus patients: analysis of adult and pediatric cohorts in Taiwan. J Chin Med Assoc. 2022; Nov 185(11):1044–1050. PubMed PMID: 36343272; eng. doi:10.1097/JCMA.0000000000000783
- Danza A, Ruiz-Irastorza G. Infection risk in systemic lupus erythematosus patients: susceptibility factors and preventive strategies. Lupus. 2013; Oct22(12):1286–1294. PubMed PMID: 24098001; eng. doi:10.1177/0961203313493032
- Caza T, Oaks Z, Perl A. Interplay of infections, autoimmunity, and immunosuppression in systemic lupus erythematosus. Int Rev Immunol. 2014; Jul-Aug33(4):330–363. PubMed PMID: 24471448; eng. doi:10.3109/08830185.2013.863305
- Akiyama S, Hamdeh S, Micic D, et al. Prevalence and clinical outcomes of COVID-19 in patients with autoimmune diseases: a systematic review and meta-analysis. Ann Rheum Dis. 2021; Mar80(3):384–391. PubMed PMID: 33051220; PubMed Central PMCID: PMCPMC7554412. eng. doi:10.1136/annrheumdis-2020-218946
- Zen M, Fuzzi E, Astorri D, et al. SARS-CoV-2 infection in patients with autoimmune rheumatic diseases in northeast Italy: a cross-sectional study on 916 patients. J Autoimmun. 2020; Aug112:102502. PubMed PMID: 32527675; PubMed Central PMCID: PMCPMC7832807. eng. doi:10.1016/j.jaut.2020.102502
- Mathian A, Mahevas M, Rohmer J, et al. Clinical course of coronavirus disease 2019 (COVID-19) in a series of 17 patients with systemic lupus erythematosus under long-term treatment with hydroxychloroquine. Ann Rheum Dis. 2020; Jun79(6):837–839. PubMed PMID: 32332072; eng. doi:10.1136/annrheumdis-2020-217566
- Thanou A, Sawalha AH. SARS-CoV-2 and systemic lupus erythematosus. Curr Rheumatol Rep. 2021; Jan 2823(2):8. PubMed PMID: 33511495; PubMed Central PMCID: PMCPMC7842169. eng. doi:10.1007/s11926-020-00973-w
- Cao Y, Zhu W, Chen W, et al. Prognostic value of BIRC5 in lung adenocarcinoma lacking EGFR, KRAS, and ALK mutations by integrated bioinformatics analysis. Dis Markers. 2019;2019:5451290–5451212. PubMed PMID: 31093306; PubMed Central PMCID: PMCPMC6481100. eng.
- Kee JY, Hong SH. Ginsenoside Rg3 suppresses mast cell-mediated allergic inflammation via mitogen-activated protein kinase signaling pathway. J Ginseng Res. 2019; Apr43(2):282–290. PubMed PMID: 30976166; PubMed Central PMCID: PMCPMC6437450. eng. doi:10.1016/j.jgr.2018.02.008
- Gaudet S, Branton D, Lue RA. Characterization of PDZ-binding kinase, a mitotic kinase. Proc Natl Acad Sci U S A. 2000; May 997(10):5167–5172. PubMed PMID: 10779557; PubMed Central PMCID: PMCPMC25800. eng. doi:10.1073/pnas.090102397
- Ishikawa C, Senba M, Mori N. Mitotic kinase PBK/TOPK as a therapeutic target for adult T‑cell leukemia/lymphoma. Int J Oncol. 2018; Aug53(2):801–814. PubMed PMID: 29901068; eng.
- Xiong M, Zhuang K, Luo Y, et al. KIF20A promotes cellular malignant behavior and enhances resistance to chemotherapy in colorectal cancer through regulation of the JAK/STAT3 signaling pathway. Aging (Albany NY). 2019; Dec 1611(24):11905–11921. PubMed PMID: 31841120; PubMed Central PMCID: PMCPMC6949076. eng. doi:10.18632/aging.102505
- Samy A, Maher MA, Abdelsalam NA, et al. SARS-CoV-2 potential drugs, drug targets, and biomarkers: a viral-host interaction network-based analysis. Sci Rep. 2022; Jul 1312(1):11934. PubMed PMID: 35831333; PubMed Central PMCID: PMCPMC9279364. eng. doi:10.1038/s41598-022-15898-w
- Zhuang Z, Chen Q, Zhong X, et al. Ginsenoside Rg3, a promising agent for NSCLC patients in the pandemic: a large-scale data mining and systemic biological analysis. J Ginseng Res. 2023; Mar47(2):291–301. PubMed PMID: 36249948; PubMed Central PMCID: PMCPMC9553969. eng. doi:10.1016/j.jgr.2022.09.006
- Ward SE, Curley GF, Lavin M, et al. Von willebrand factor propeptide in severe coronavirus disease 2019 (COVID-19): evidence of acute and sustained endothelial cell activation. Br J Haematol. 2021; Feb192(4):714–719. PubMed PMID: 33326604; eng. doi:10.1111/bjh.17273
- Devreese KMJ, Linskens EA, Benoit D, et al. Antiphospholipid antibodies in patients with COVID-19: a relevant observation? J Thromb Haemost. 2020; Sep18(9):2191–2201. PubMed PMID: 32619328; PubMed Central PMCID: PMCPMC7361253. eng. doi:10.1111/jth.14994
- Java A, Apicelli AJ, Liszewski MK, et al. The complement system in COVID-19: friend and foe? JCI Insight. 2020; Aug 6;5(15). PubMed PMID: 32554923; PubMed Central PMCID: PMCPMC7455060 Alexion Pharmaceuticals and Novartis Pharmaceuticals and serving as a consultant for Gemini Therapeutics. JPA reports serving as a consultant for Celldex Therapeutics, Clinical Pharmacy Services, Kypha Inc., Achillion Pharmaceuticals Inc., and BioMarin Pharmaceutical Inc. and stock or equity options in Compliment Corporation, Kypha Inc., Gemini Therapeutics, and AdMiRx Inc. AHJK reports personal fees (<$10,000) from Exagen Diagnostics Inc. and GlaxoSmithKline. eng. doi:10.1172/jci.insight.140711
- Postal M, Vivaldo JF, Fernandez-Ruiz R, et al. Type I interferon in the pathogenesis of systemic lupus erythematosus. Curr Opin Immunol. 2020; Dec67:87–94. PubMed PMID: 33246136; PubMed Central PMCID: PMCPMC8054829. eng. doi:10.1016/j.coi.2020.10.014
- Bastard P, Rosen LB, Zhang Q, et al. Autoantibodies against type I IFNs in patients with life-threatening COVID-19. Science. 2020; Oct 23370(6515). PubMed PMID: 32972996; PubMed Central PMCID: PMCPMC7857397. eng. doi:10.1126/science.abd4585
- Wang D, Hu B, Hu C, et al. Clinical characteristics of 138 hospitalized patients with 2019 novel coronavirus-Infected pneumonia in wuhan, China. Jama. 2020; Mar 17323(11):1061–1069. PubMed PMID: 32031570; PubMed Central PMCID: PMCPMC7042881. eng. doi:10.1001/jama.2020.1585
- Saitoh T, Komano J, Saitoh Y, et al. Neutrophil extracellular traps mediate a host defense response to human immunodeficiency virus-1. Cell Host Microbe. 2012; Jul 1912(1):109–116. PubMed PMID: 22817992; eng. doi:10.1016/j.chom.2012.05.015
- Jenne CN, Wong CH, Zemp FJ, et al. Neutrophils recruited to sites of infection protect from virus challenge by releasing neutrophil extracellular traps. Cell Host Microbe. 2013; Feb 1313(2):169–180. PubMed PMID: 23414757; eng. doi:10.1016/j.chom.2013.01.005
- García LF. Immune response, inflammation, and the clinical spectrum of COVID-19. Front Immunol. 2020;11:1441. PubMed PMID: 32612615; PubMed Central PMCID: PMCPMC7308593. eng. doi:10.3389/fimmu.2020.01441
- Tan L, Wang Q, Zhang D, et al. Lymphopenia predicts disease severity of COVID-19: a descriptive and predictive study. Signal Transduct Target Ther. 2020; Mar 275(1):33. PubMed PMID: 32296069; PubMed Central PMCID: PMCPMC7100419. eng. doi:10.1038/s41392-020-0148-4
- Zhang N, Li C, Hu Y, et al. Current development of COVID-19 diagnostics, vaccines and therapeutics. Microbes Infect. 2020; Jul-Aug22(6-7):231–235. PubMed PMID: 32387332; PubMed Central PMCID: PMCPMC7200352. eng. doi:10.1016/j.micinf.2020.05.001
- Irvani SSN, Golmohammadi M, Pourhoseingholi MA, et al. Effectiveness of interferon beta 1a, compared to interferon beta 1b and the usual therapeutic regimen to treat adults with moderate to severe COVID-19: structured summary of a study protocol for a randomized controlled trial. Trials. 2020; Jun 321(1):473. PubMed PMID: 32493468; PubMed Central PMCID: PMCPMC7268172. eng. doi:10.1186/s13063-020-04382-3
- Wu D, Yang XO. TH17 responses in cytokine storm of COVID-19: an emerging target of JAK2 inhibitor fedratinib. J Microbiol Immunol Infect. 2020; Jun53(3):368–370. PubMed PMID: 32205092; PubMed Central PMCID: PMCPMC7156211. eng. doi:10.1016/j.jmii.2020.03.005
- Toor SM, Saleh R, Sasidharan Nair V, et al. T-cell responses and therapies against SARS-CoV-2 infection. Immunology. 2021; Jan162(1):30–43. PubMed PMID: 32935333; PubMed Central PMCID: PMCPMC7730020. eng. doi:10.1111/imm.13262
- Nelson P, Rylance P, Roden D, et al. Viruses as potential pathogenic agents in systemic lupus erythematosus. Lupus. 2014; May23(6):596–605. PubMed PMID: 24763543; eng. doi:10.1177/0961203314531637
- Esposito S, Bosis S, Semino M, et al. Infections and systemic lupus erythematosus. Eur J Clin Microbiol Infect Dis. 2014; Sep33(9):1467–1475. PubMed PMID: 24715155; eng. doi:10.1007/s10096-014-2098-7
- Spitz F, Furlong EE. Transcription factors: from enhancer binding to developmental control. Nat Rev Genet. 2012; Sep13(9):613–626. PubMed PMID: 22868264; eng. doi:10.1038/nrg3207
- Francois M, Donovan P, Fontaine F. Modulating transcription factor activity: interfering with protein-protein interaction networks. Semin Cell Dev Biol. 2020; Mar99:12–19. PubMed PMID: 30172762; eng. doi:10.1016/j.semcdb.2018.07.019
- Chen L, Heikkinen L, Wang C, et al. Trends in the development of miRNA bioinformatics tools. Brief Bioinform. 2019; Sep 2720(5):1836–1852. PubMed PMID: 29982332; PubMed Central PMCID: PMCPMC7414524. eng. doi:10.1093/bib/bby054
- Bisserier M, Milara J, Abdeldjebbar Y, et al. AAV1.SERCA2a gene therapy reverses pulmonary fibrosis by blocking the STAT3/FOXM1 pathway and promoting the SNON/SKI axis. Mol Ther. 2020; Feb 528(2):394–410. PubMed PMID: 31879190; PubMed Central PMCID: PMCPMC7001085. eng. doi:10.1016/j.ymthe.2019.11.027
- Schneider WM, Chevillotte MD, Rice CM. Interferon-stimulated genes: a complex web of host defenses. Annu Rev Immunol. 2014;32(1):513–545. PubMed PMID: 24555472; PubMed Central PMCID: PMCPMC4313732. eng. doi:10.1146/annurev-immunol-032713-120231
- Zhu JY, Wang G, Huang X, et al. SARS-CoV-2 Nsp6 damages drosophila heart and mouse cardiomyocytes through MGA/MAX complex-mediated increased glycolysis. Commun Biol. 2022; Sep 305(1):1039. PubMed PMID: 36180527; PubMed Central PMCID: PMCPMC9523645. eng. doi:10.1038/s42003-022-03986-6
- Umar S, Palasiewicz K, Meyer A, et al. Inhibition of IRAK4 dysregulates SARS-CoV-2 spike protein-induced macrophage inflammatory and glycolytic reprogramming. Cell Mol Life Sci. 2022; May 1979(6):301. PubMed PMID: 35588018; PubMed Central PMCID: PMCPMC9118817. eng. doi:10.1007/s00018-022-04329-8
- Zheng Q, Wang D, Lin R, et al. IFI44 is an immune evasion biomarker for SARS-CoV-2 and Staphylococcus aureus infection in patients with RA. Front Immunol. 2022;13:1013322. PubMed PMID: 36189314; PubMed Central PMCID: PMCPMC9520788. eng. doi:10.3389/fimmu.2022.1013322
- Liu Y, Li L, Song X. Exosomal circPVT1 derived from lung cancer promotes the progression of lung cancer by targeting miR-124-3p/EZH2 axis and regulating macrophage polarization. Cell Cycle. 2022; Mar21(5):514–530. PubMed PMID: 35025697; PubMed Central PMCID: PMCPMC8942510. eng. doi:10.1080/15384101.2021.2024997
- Tsokos GC. Systemic lupus erythematosus. N Engl J Med. 2011;365(22):2110–2121. doi:10.1056/NEJMra1100359
- Gopal J, Muthu M, Sivanesan I. A comprehensive survey on the expediated anti-COVID-19 options enabled by metal Complexes-Tasks and trials. Molecules. 2023; Apr 1028(8):3354. PubMed PMID: 37110587; PubMed Central PMCID: PMCPMC10143858. eng. doi:10.3390/molecules28083354
- Al-Motawa MS, Abbas H, Wijten P, et al. Vulnerabilities of the SARS-CoV-2 virus to Proteotoxicity-Opportunity for repurposed chemotherapy of COVID-19 infection. Front Pharmacol. 2020;11:585408. PubMed PMID: 33162891; PubMed Central PMCID: PMCPMC7581855. eng. doi:10.3389/fphar.2020.585408
- Fitch CA, Platzer G, Okon M, et al. Arginine: its pKa value revisited. Protein Sci. 2015; May24(5):752–761. PubMed PMID: 25808204; PubMed Central PMCID: PMCPMC4420524. eng. doi:10.1002/pro.2647
- Ahmad SI. 5-Fluorouracil in combination with deoxyribonucleosides and deoxyribose as possible therapeutic options for the coronavirus, COVID-19 infection. Med Hypotheses. 2020; Sep142:109754. PubMed PMID: 32438240; PubMed Central PMCID: PMCPMC7194918 competing financial interests or personal relationships that could have appeared to influence the work reported in this paper. eng. doi:10.1016/j.mehy.2020.109754
- Schälter F, Dürholz K, Bucci L, et al. Does methotrexate influence COVID-19 infection? Case series and mechanistic data. Arthritis Res Ther. 2021; Jun 1023(1):166. PubMed PMID: 34112217; PubMed Central PMCID: PMCPMC8190723. eng. doi:10.1186/s13075-021-02464-4
- Selvaraj V, Dapaah-Afriyie K, Finn A, et al. Short-Term dexamethasone in sars-CoV-2 patients. R I Med J (2013). 2020; Jun 19103(6):39–43. PubMed PMID: 32570995; eng.
- Ferreto LED, Bortoloti DS, Fortes PCN, et al. Dexamethasone for treating SARS-CoV-2 infection: a systematic review and meta-analysis. Sao Paulo Med J. 2021;139(6):657–661. PubMed PMID: 34644768; PubMed Central PMCID: PMCPMC9634846. eng. doi:10.1590/1516-3180.2021.0120.r1.30062021
- Ungaro RC, Brenner EJ, Gearry RB, et al. Effect of IBD medications on COVID-19 outcomes: results from an international registry. Gut. 2021; Apr70(4):725–732. PubMed PMID: 33082265; PubMed Central PMCID: PMCPMC8136807. eng. doi:10.1136/gutjnl-2020-322539
- Devaux CA, Melenotte C, Piercecchi-Marti MD, et al. Cyclosporin A: a repurposable drug in the treatment of COVID-19? Front Med (Lausanne). 2021;8:663708. PubMed PMID: 34552938; PubMed Central PMCID: PMCPMC8450353. eng. doi:10.3389/fmed.2021.663708
- Szente Fonseca SN, de Queiroz Sousa A, Wolkoff AG, et al. Risk of hospitalization for covid-19 outpatients treated with various drug regimens in Brazil: Comparative analysis. Travel Med Infect Dis. 2020; Nov-Dec38:101906. PubMed PMID: 33137493; PubMed Central PMCID: PMCPMC7604153. eng. doi:10.1016/j.tmaid.2020.101906
- Vaira LA, Hopkins C, Petrocelli M, et al. Efficacy of corticosteroid therapy in the treatment of long- lasting olfactory disorders in COVID-19 patients. Rhinology. 2021; Feb 159(1):21–25. PubMed PMID: 33290446; eng.
- Ugarte-Gil MF, Alarcón GS, Izadi Z, et al. Characteristics associated with poor COVID-19 outcomes in individuals with systemic lupus erythematosus: data from the COVID-19 global rheumatology alliance. Ann Rheum Dis. 2022; Jul81(7):970–978. PubMed PMID: 35172961; PubMed Central PMCID: PMCPMC8882632. eng. doi:10.1136/annrheumdis-2021-221636
- Saggi SJ, Nath S, Culas R, et al. Early experience with methylprednisolone on SARS-CoV-2 infection in the african American population, a retrospective analysis. Clin Med Insights Circ Respir Pulm Med. 2020;14:1179548420980699. PubMed PMID: 33402859; PubMed Central PMCID: PMCPMC7745550. eng.
- Horby P, Mafham M, Linsell L, et al. Effect of hydroxychloroquine in hospitalized patients with covid-19. N Engl J Med. 2020; Nov 19383(21):2030–2040. PubMed PMID: 33031652; PubMed Central PMCID: PMCPMC7556338. eng.
- Tang W, Cao Z, Han M, et al. Hydroxychloroquine in patients with mainly mild to moderate coronavirus disease 2019: open label, randomised controlled trial. BMJ. 2020; May 14369:m1849. PubMed PMID: 32409561; PubMed Central PMCID: PMCPMC7221473 at www.icmje.org/coi_disclosure.pdf and declare: no support from any organisation for the submitted work other than those listed above; no financial relationships with any organisations that might have an interest in the submitted work in the previous three years; no other relationships or activities that could appear to have influenced the submitted work. eng.
- Cavalcanti AB, Zampieri FG, Rosa RG, et al. Hydroxychloroquine with or without azithromycin in mild-to-Moderate covid-19. N Engl J Med. 2020; Nov 19383(21):2041–2052. PubMed PMID: 32706953; PubMed Central PMCID: PMCPMC7397242. eng. doi:10.1056/NEJMoa2019014
- Bellos I. A metaresearch study revealed susceptibility of covid-19 treatment research to white hat bias: first, do no harm. J Clin Epidemiol. 2021; Aug136:55–63. PubMed PMID: 33775812; PubMed Central PMCID: PMCPMC7997163. eng. doi:10.1016/j.jclinepi.2021.03.020
- Savarino A, Boelaert JR, Cassone A, et al. Effects of chloroquine on viral infections: an old drug against today’s diseases? Lancet Infect Dis. 2003; Nov3(11):722–727. PubMed PMID: 14592603; PubMed Central PMCID: PMCPMC7128816. eng. doi:10.1016/S1473-3099(03)00806-5
- Rainsford KD, Parke AL, Clifford-Rashotte M, et al. Therapy and pharmacological properties of hydroxychloroquine and chloroquine in treatment of systemic lupus erythematosus, rheumatoid arthritis and related diseases. Inflammopharmacology. 2015; Oct23(5):231–269. PubMed PMID: 26246395; eng. doi:10.1007/s10787-015-0239-y
- Carbajo-Lozoya J, Müller MA, Kallies S, et al. Replication of human coronaviruses SARS-CoV, HCoV-NL63 and HCoV-229E is inhibited by the drug FK506. Virus Res. 2012; Apr323(1):198952–198117. PubMed PMID: 22349148; PubMed Central PMCID: PMCPMC7114512. eng.
- Ohgushi M, Ogo N, Yanagihara T, et al. Tacrolimus treatment for Post-COVID-19 interstitial lung disease. Intern Med. 2022; Feb 1561(4):585–589. PubMed PMID: 34866097; PubMed Central PMCID: PMCPMC8907778. eng. doi:10.2169/internalmedicine.7971-21
- Chalklin CG, Koimtzis G, Khalid U, et al. SARS-CoV-2 infection can lead to an increase in tacrolimus levels in renal transplant patients: a cohort study. Transpl Int. 2022;35:10127. PubMed PMID: 35387396; PubMed Central PMCID: PMCPMC8977848. eng. doi:10.3389/ti.2022.10127