Abstract
Yinchenhao Decoction (YCHD) is a classic prescription in traditional Chinese medicine (TCM). It appears to play an important role in anti-inflammation and autoimmunity protection. As one of the key active ingredients in YCHD, quercetin is a novel anti-inflammatory metabolite that exerts protective effects in many autoimmune diseases. However, its role in autoimmune hepatitis (AIH)-related hepatic injury has not been studied. The aim of this study was to reveal the hepatocyte protective mechanism of quercetin. In this study, we used Concanavalin A (Con A) to establish an in vitro hepatocyte injury-associated AIH model. Brl3a hepatocyte injury was induced by the supernatant of J774A.1 cells treated with Con A. We found that quercetin mitigated Con A-induced via macrophage-mediated Brl3a hepatocyte injury. Quercetin administration reduced the levels of alanine transaminase (ALT) and aspartate transaminase (AST) in the supernatant of Con A-treated Brl3a cells and attenuated the infiltration of J774A.1 macrophages induced by Con A. Moreover, quercetin effectively inhibited the expression of proinflammatory cytokines including interleukin-1β (IL-1β) by Con A. Furthermore, quercetin decreased hepatocyte apoptosis and ferroptosis levels in the macrophage-induced hepatocyte injury model. In conclusion, our study indicates that quercetin alleviates macrophage-induced hepatocyte damage by reducing the inflammatory response, apoptosis and ferroptosis. Our work suggests that quercetin might be a potential therapeutic strategy for AIH.
1. Introduction
Autoimmune hepatitis (AIH) is considered an immune disorder-induced hepatic injury mediated by abnormal activation of immunocytes and the production of proinflammatory mediators, characterized by chronic hepatitis of different severity [Citation1]. The rising trend of AIH prevalence has been observed worldwide, the lack of adequate treatment options for AIH eventually leads to long-term liver damage and damage from cirrhosis, dysfunction and even organ failure [Citation2,Citation3]. The pathogenesis of AIH is mainly related to genetics, environmental factors and abnormal immune tolerance, etc., but its mechanism has not been fully clarified [Citation4–6]. Research has shown that macrophages are involved in antigen processing, presentation to naive CD4+ T helper cells, and induction of T cell activation to produce inflammatory cytokines and mediate hepatocyte damage. In addition, macrophages have also been demonstrated to directly mediate hepatocyte injury by expressing inflammatory factors and inducing oxidative stress [Citation7–10]. Clarifying the molecular mechanism of macrophage-induced hepatocyte damage is conducive to providing new targets and ideas for the diagnosis and treatment of AIH.
Currently, glucocorticoids alone or combined with azathioprine are commonly used to treat AIH. This treatment can reduce liver inflammation and improve liver injury, but it has high hepatotoxicity and other side effects, is easy to develop drug resistance, and has a high risk of recurrence after drug withdrawal [Citation11]. Therefore, there is an urgent need to find a safe and effective treatment for AIH. TCM was widely considered an alternative or complementary therapy for the treatment of various diseases. Clinical studies have shown that TCM has achieved favorable results in improving the clinical manifestations of AIH and reducing liver function indicators such as transaminase. It is well tolerated and can improve the quality of life of patients [Citation12]. As a classic TCM, YCHD, which was first described in the Treatise on Febrile Diseases (Shanghan Lun), is widely used to treat various liver diseases [Citation13]. Modern studies believe that YCHD can promote gallbladder and protect liver by lowering lipid and blood glucose, anti-inflammatory and so on [Citation14,Citation15]. However, its mechanism of action on AIH is not very clear yet. With the rapid development of bioinformatics and systems biology, network pharmacology provides a new method to reveal the complex mechanisms of TCM. According to our results of Data Mining technology and network pharmacology method, it was shown that YCHD is the most effective prescription for improving liver function in patients with AIH, and quercetin may be the key ingredient of YCHD that plays a role in liver protection. Quercetin, an important member of the flavonoid family, has a wide range of biological effects, which may play a protective role in liver cells through anti-inflammatory, antioxidant stress, anti-apoptosis and ferroptosis, and play a bidirectional regulatory role in inflammation and autoimmunity [Citation16–18]. Ferroptosis, an iron-dependent, novel programmed mode of cell death, was different from necrosis, apoptosis, and autophagy and was first proposed by Stockwell in 2012 [Citation19,Citation20]. Therefore, quercetin has a high potential for application in the clinical treatment of AIH. However, whether quercetin plays a therapeutic role in the pathological process of macrophage-induced liver injury remains to be further studied.
Therefore, we established co-culture models of J774A.1 macrophages and Brl3a hepatocyte to partially simulate the macrophage-mediated hepatocyte inflammatory injury during the pathogenesis of AIH in vitro. We investigated the effect of quercetin on a Con A-induced AIH model and found that quercetin protected Brl3a cells from macrophages-mediated injury. Furthermore, quercetin reduced hepatocyte inflammation, apoptosis, and ferroptosis in Con A-induced AIH models. Therefore, our current study shows that quercetin might be a potential therapeutic strategy for AIH. It provides a new idea to study the molecular mechanism of TCM compound YCHD in treating autoimmune hepatitis.
2. Materials and methods
2.1. Reagent
The murine macrophage cell lines J774A.1 macrophages and Brl3a were purchased from Procell Life Science & Technology Co., Ltd. (Wuhan, China), the cells were in good condition without mycoplasma contamination. Con A and quercetin were bought from Sigma Aldrich (St. Louis, MO, USA). Nitric Oxide Assay Kits were obtained from Beyotime Biotechnology (Shanghai, China, S0021S). The alanine transaminase (ALT), aspartate transaminase (AST), reactive oxygen species assay kit (ROS), malondialdehyde (MDA) assay kit (colorimetric method), and trace reduced glutathione assay kit (GSH) were purchased from Nanjing Jiancheng Institute of Biotechnology (Nanjing, China, C009-2-1, C010-2-1, E004-1-1, A003-4-1, A006-2-1). Enhanced Mitochondrial Membrane Potential Assay Kit (JC-1) and Nitric Oxide Assay Kit were brought from Beyotime Biotechnology (C2003S, S0021S). Fetal bovine serum and high glucose Dulbecco’s modified Eagle’s medium (DMEM) were purchased from Wuhan Doctor Biology Engineering Co., Ltd. (Wuhan, China). Bio-Swamp Life Science Co. (Wuhan, China) provided the Mouse Interleukin-1 (IL-1β) ELISA Kit. Annexin V-FITC/PI Apoptosis Detection Kit was purchased from Dalian Meilun Biotechnology Co., Ltd. (Dalian, China, MA0220). IL-1-APC was purchased from Shanghai Universal Biotech Co., Ltd. (Shanghai, China). Cell Counting Kit-8 was purchased from Wuhan Boster Biological Technology, Ltd. (Wuhan, China, AR1160-500). A total iron colorimetric kit was purchased from Elabscience Biotechnology Co., Ltd. (Wuhan, China, E-BC-K772-M). All other chemicals used were of the highest analytical grade.
2.2. Data preparation
Data mining technology was used in the early stages of this study to investigate the rules of TCM diagnosis and treatment of AIH. By searching the medical records of AIH traditional Chinese medicine treatment from 1990 to 2021, including the CNKY, VIP journal database, Wanfang database, and Chinese Biomedical Database, the prescription with the highest frequency of AIH treatment was selected.
Different herbal compounds in YCHD were obtained by the TCMSP method and screened for oral bioavailability (OB) ≥30% and drug-likeness (DL) ≥0.18. AIH-related targets were obtained from three existing resources with the keyword “autoimmune hepatitis.” Using the following three databases to collect AIH disease targets: the OMIM database, GeneCards, and DrugBank. Then Uniprot was used to correct the gene names of the above compounds.
Based on their interaction data for YCHD, we constructed the interaction network of active components and putative targets of YCHD therapy and used Cytoscape software (version 3.5.0) to visualize the interactive network. Degree, betweenness centrality, and closeness centrality were analyzed by the Network Analyzer plugin in Cytoscape, which was used to measure the topological importance of nodes in the network. The key components of YCHD in the treatment of AIH were analyzed so as to screen out the key active components of YCHD in the treatment of AIH.
2.3. Optimization of Con A-induced J774A.1 macrophages inflammation model
To determine the optimal concentration and action time for Con A, the Con A binding capacity of J774A.1 macrophages was further evaluated at different conditions. J774A.1 macrophages were incubated with different concentrations of Con A-FITC (0, 10, 20, 40, and 80 μg/ml) for 12 h, and the number of FITC-positive cells in J774A.1 macrophages was evaluated by flow cytometry. Then, J774A.1 macrophages were incubated with Con A-FITC at different concentrations for 0 h, 6 h, 12 h, and 24 h, and the number of FITC-positive cells in J774A.1 macrophages was also tested by flow cytometry. The above experiments were carried out to determine whether the binding of Con A and macrophage J774A.1 macrophages was concentration and time-dependent, with the goal of determining the optimal concentration and time for Con A.
2.4. Cell viability assay
Cell counting kit 8 was used for cell activity detection. Cells were seeded into a 96-well plate (1 × 104 cells/well). After the cells had adhered, various concentrations of quercetin (0, 25, 50, 100, 200, 400 μM) were added and the cells were treated for 12 h, 24 h and 48 h. Each well received 10 μl of CCK-8 and was incubated for 1 h. The absorbance at 450 nm was measured by a microplate reader.
2.5. Cell culture
The murine macrophage cell lines J774A.1 macrophages and Brl3a cells were cultured in DMEM supplemented with 10% fetal bovine serum. The cultures were maintained at 37 °C inside an incubator (95% air, 5% CO2). The co-culture experiment was performed in a 6-well transwell dish. The Brl3a cells (2 × 105 cells/ml) were seeded at the bottom well, and the J774A.1 macrophages (2 × 105 cells/ml) were seeded in the upper inserts (0.4 μm pore size) containing DMEM serum medium. The cells were divided into three groups: normal, Con A, and Con A + quercetin. The normal group did not receive treatment. The Con A group was treated with 40 μg/ml Con A for 12 h in the upper layer of the transwell chamber, and the Con A + quercetin group was pre-treated with 100 μM quercetin for 12 h and then treated with 40 μg/mL Con A for 12 h. It should be noted that because the ferroptosis inhibitor control group, the ConA + Ferrostain-1 group, was also required in the ferroptosis experimental part, it was necessary to include this group for ferroptosis-related experiments. The ConA + Ferrostain-1 group was pre-treated for 12 h with 2 μM Ferrostain-1 before being treated for 12 h with 40 μg/mL Con A.
2.6. Detection of hepatocyte injury related indexes and inflammatory factors
Brl3a cell supernatants were collected after centrifugation at 1500 rpm for 5 min. Subsequently, the activities of hepatic enzymes ALT and AST were measured according to the manufacturer’s instructions. Logarithmically grown Brl3a cells were digested and collected with trypsin to detect NO content. The supernatants of J774A.1 macrophages were collected at 12 h after Con A treatment (40 μg/ml), and then the level of IL-1β was measured according to the manufacturer’s instructions by flow cytometry and ELISA, respectively.
2.7. Western blot analysis
Cells were lysed in Radio Immunoprecipitation Assay (RIPA) buffer with 1% phenylmethylsulphonyl fluoride (PMSF) to extract protein. The samples were separated on a 10% SDS polyacrylamide gel and transferred to polyvinylidene difluoride membranes in a wet transfer apparatus. The membranes were pre-incubated at room temperature in 1% BSA for 1.5 h and incubated overnight at 4 °C with the appropriate primary antibody. The membranes were washed with Tris-buffered saline containing Tween (TBST) three times for 10 min each and then incubated with horseradish peroxidase-conjugated secondary antibodies for 2 h at room temperature. Following additional washes, the immunoreactive proteins on the membrane were observed with enhanced chemiluminescence plus reagents. The intensities of the immunoreactive proteins were measured via computerized image analysis. All the experiments were repeated at least three times.
2.8. Apoptosis detected
The Annexin V-FITC/PI Apoptosis Detection Kit was used to detect the apoptosis. Brl3a cells were collected after treatment, 100 μl binding buffer was added to each tube, followed by FITC and PI reagents, and then the tubes were incubated for 15 min. Finally, the apoptosis level was detected by flow cytometry according to the instructions.
JC-1 is an ideal fluorescent probe for detecting mitochondrial membrane potential. Normal, healthy mitochondrial membrane potential is polar, and the mitochondria have high mitochondrial membrane potential. JC-1 depends on its polarity and is rapidly ingested into the mitochondria, where it aggregates in the matrix of the mitochondria in the form of a polymer (J-aggregates), which produces red fluorescence. At a low mitochondrial membrane potential, depolarization of the mitochondrial membrane potential causes JC-1 to be released from within the mitochondria and exist in the cytoplasm in the form of a monomer. Instead of being able to gather in the matrix of mitochondria, JC-1 can produce green fluorescence. Changes in the mitochondrial membrane potential are represented by the conversion of fluorescence, and in this experiment, the ratio of PE (red) JC-1 aggregates to FITC (green) JC-1 decreases was used to represent the mitochondrial membrane potential. The relative ratio of red and green fluorescence is used to express values for mitochondrial membrane potential. The lower the red-green ratio, the lower the mitochondrial membrane potential.
Mitochondrial membrane potential (JC-1) was determined as follows: The Brl3a cells (1 × 106 cells/well) were cultured in a 6-well plate and treated with three different experimental conditions. The same volume of JC-1 was added and incubated with the cells for 20 min. Then, cells were washed twice with PBS and finally detected by a fluorescent inverted microscope and flow cytometry.
2.9. Detection of indicators related to ferroptosis
For the detection of ROS generation in J774A.1 cells, 2′, 7′-dichlorofluorescein-diacetate (DCFH-DA) was used. All groups of J77A.1 cells were washed with cold PBS three times. Then, the cells were then re-suspended in serum-free culture medium mixed with 10 μM DCFH-DA. The stained cells were detected for ROS generation by a fluorescence microplate reader and fluorescent inverted microscope.
Using a GSH assay kit to detect the intracellular total GSH level according to the manufacturer’s protocols. The relative levels were analyzed on the microplate reader. J774A.1 cells were digested by trypsin and used for subsequent experiments. The MDA level was evaluated according to the manufacturer’s instructions. The relative iron concentration in cells was determined by an iron assay kit according to the instructions.
2.10. Statistical analysis
All data were expressed as mean ± SD, t test was conducted on two sets of data using GraphPad Prism 7.0 software. Followed by one-way analysis of variance (ANOVA) was carried out to draw comparison between more than two groups, with p < 0.05 treated as statistically significant.
3. Result
3.1. Data mining and network pharmacology results
YCHD is widely used in clinical practice for hepatobiliary-related diseases. YCHD mainly protects the liver by inhibiting liver steatosis, apoptosis, necrosis, anti-inflammation, and immune regulation. It can be used to treat AIH, hepatitis B, liver fibrosis, cholestasis, non-alcoholic fatty liver and other diseases [Citation15]. According to data mining, the clinical application frequency of YCHD was the highest (24/149, 16.10%) by searching the medical records of TCM diagnosis and treatment of AIH from 1990 to 2021 on CNKI.com, VIP journal database, Wanfang database, and China Biomedical database (). Through data mining and complex network analysis technology, SPSS 21.0 software was used for clustering analysis of pharmaceutical flavors with frequencies > 3, and it was obtained that the frequency of artemisia capillaris, gardenia, and rhubarb was quite high, at 25 times (). Network pharmacology is regarded as a powerful tool for identifying components and investigating the mechanisms of Chinese herbal medicine. In this study, the key active ingredients in YCHD were analyzed by network pharmacology. The Venn diagram of “drug-disease-related target” () was plotted, which acquired 171 targets of YCHD for AIH treatment. A total of 35 chemical active ingredients in the YCHD formula were harvested from the TCMSP database, among which 13 were artemisia capillaris, 12 were gardenia, and 10 were rhubarb. The target prediction function of the TCMSP database was utilized. Subsequent to data screening and duplicate value removal, 171 predicted targets of chemical active ingredients in the YCHD formula were yielded. The Cytoscape software was employed to complete the relationship network diagram of the effective chemical ingredients (). The targets obtained in each database were intersected, screened, and processed through the GeneCards database, which yielded 1369 disease-related targets of AIH. The top five core active ingredients for the treatment of AIH were quercetin, beta-sitosterol, kaempferol, eupatin, and stigmasterol. Quercetin has the most targets and may be the key active ingredient of YCHD in the treatment of AIH.
Figure 1. Network pharmacological analysis of key active ingredients in Yinchenhao decoction.
The treatment of AIH compound frequency ratio statistics pie chart (A). Blue represents Yinchenhao decoction (16%), orange represents Chaihu Shugan Powder (12%), gray represents Xiaoyao Powder (12%), and yellow represents All-Along Decoction (9%). Single drug cluster dendrogram for AIH (B). Cluster analysis by SPSS, the abscissa for drug use frequency, ordinate for drug name, among them, the red fonts are artemisia capillaris, gardenia and rhubarb, respectively. The three drugs were grouped into one type, and the combined use frequency was 24 times. Venn diagram of YCHD and AIH candidate targets (C). The blue part is the target of AIH, the gray part is the target of YCHD, and the 171 intersections are potential targets of YCHD in the treatment of AIH. Network diagram of “active ingredient-target” of YCHD (D). The blue color refers to 171 putative targets of YCHD for the treatment of AIH. The yellow color represents the ingredient of artemisia capillaris, the orange color represents the ingredient of Gardenia, and the green color represents the ingredient of rhubarb. The gray circle represents β-sitosterol, which is a common component of artemisia capillaris, gardenia, and rhubarb.
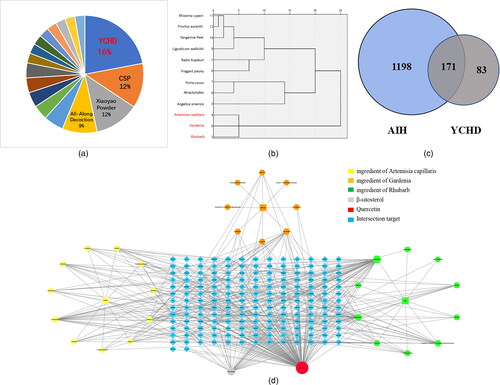
3.2. Optimization of Con A model and selection of quercetin action concentration and time
J774A.1 macrophages were incubated with different concentrations of Con A-FITC (0, 10, 20, 40, and 80 μg/ml) for 12 h. The MFI of Con A expression increased gradually as the concentration of Con A increased. Con A concentration of 40 μg/mL and 80 μg/mL showed no significant difference in the MFI of Con A expression. Therefore, the concentration of Con A was selected as 40 μg/mL in subsequent experiments ( and ). The cells were incubated with 40 and 80 μg/mL Con A-FITC and J774A.1 cells for 0 h, 6 h, 12 h, and 24 h, respectively. The results showed that the binding of Con A to macrophages is time dependent, and there was no statistical significance in the percentage of Con A positive at 12 h and 24 h. Therefore, the action time of Con A was selected as 12 h in subsequent experiments ( and ). The CCK8 results showed that there was no statistical significance in the activity of Brl3a cells when the concentration of quercetin was 0-100 μM, but the activity of Brl3a cells decreased significantly when the concentration reached 200 μM. Therefore, the action concentration of quercetin was selected as 100 μM in the subsequent experiment. Moreover, the activity of quercetin was higher at 12 h than at 24 h and 48 h, so the action time of quercetin was selected as 12 h in the subsequent experiment ().
Figure 2. Selection of concentration and time of Con A and quercetin.
Different concentrations of Con A-FITC (0, 10, 20, 40, 80 μg/ml) were incubated with J774A.1 cells for 12 h. With the increase in Con A concentration, the MFI of Con A expression gradually increased (A). Bar chart shows the median fluorescence intensity of Con A expression on J774A.1 cells after loading different concentrations of Con A (B), results quantified by flow cytometry. With the prolongation of Con A (6, 12, and 24 h), the percentage of Con A positive gradually increased, and the percentage of Con A positive cells basically reached saturation when Con A was treated for 12 h (C). Bar chart shows the percentage of Con A positive in J774A.1 cells at different time points post adding Con A into J774A.1 cells (D), results quantified by flow cytometry. Figure E shows the line plots of Brl3a cell activity at different quercetin concentrations, with red, green and black lines representing 12 h, 24 h and 48 h. *, P < 0.05; **, P < 0.01.
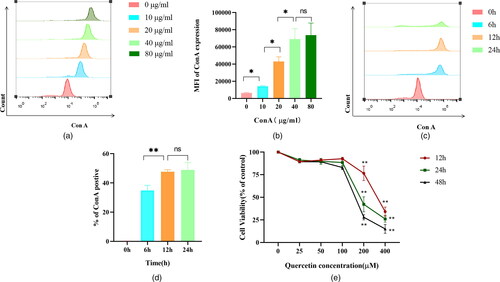
3.3. Quercetin alleviates injury of Brl3a cells by reducing inflammatory cytokines
The levels of ALT and AST in the supernatant of Brl3a cells in the Con A group were significantly higher than those in the normal group in the experiment of co-culture of Brl3a cells and J774A.1 cells. ALT and AST levels in the Con A + quercetin group were significantly lower than in the Con A group ( and ). NO content was significantly increased in Con A group, but decreased after quercetin treatment (). The production of intracellular cytokine IL-1β was first detected by flow cytometry, and the results were expressed as average fluorescence intensity. The higher the peak value shifted to the right, the higher the average fluorescence intensity was ( and ). Then the level of IL-1β in the J774A.1 cell supernatant was assessed by ELISA. We detected the level of cytokines IL-1β and found quercetin inhibited the release of IL-1β induced by Con A (). This suggests that quercetin can reduce the production of Con A-induced inflammatory cytokines in J774A.1 cells.
Figure 3. Quercetin reduces Con A-induced hepatocyte damage and levels of cytokines.
Quercetin mitigated Con A-induced hepatocyte damage in Brl3a. Figures A, B, and C represent the expression levels of ALT, AST and NO, respectively. IL-1β production was divided into three groups (Control, Con A, and Con A + quercetin), flow cytometry was used to detect it, and the negative control group was used to gate it. The more the peak shape of the single parameter histogram shifted to the right, the stronger the fluorescence intensity was (D), and the data on IL-1β were expressed as the mean fluorescence intensity (E). To increase the confidence of the results, the cytokine IL-1β was also detected by ELISA (F). Data are expressed as mean ± SEM. *P < 0.05, **P < 0.01, ***P < 0.001.
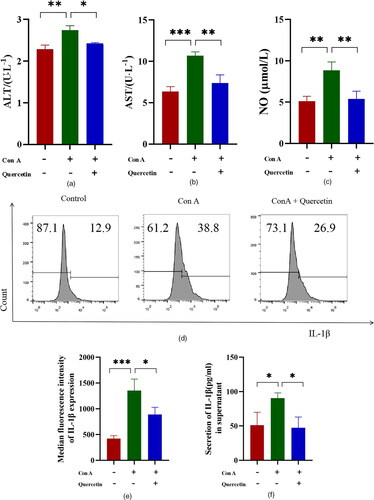
3.4. Quercetin attenuated the apoptosis of Brl3a cells
We determined the expression of apoptosis-associated proteins by Western blot. The proapoptotic proteins such as caspase 3, caspase 8, and caspase 9 of Con A group were greatly upregulated in comparison to the control groups. Compared with the Con A group, 100 μg/ml quercetin pre-treatment significantly down-regulated the expression of caspase 3, caspase 8, and caspase 9 (). These results show that quercetin inhibited Brl3a apoptosis through regulating the expression of apoptosis-associated proteins.
Figure 4. Quercetin inhibits hepatocyte apoptosis induced by Con A.
The effect of quercetin on the Con A-induced Caspases 3, 8, and 9 signaling pathway was determined by western blotting. The experiments were repeated at least three times, the difference was statistically significant (A, B and C). The apoptotic of Brl3a cells using annexin-V staining of tested cancer cell lines. Dots represent cells as follows: lower left quadrant, normal cells (FITC−/PI−); lower right quadrant, early apoptotic cells (FITC+/PI−); upper left quadrant, necrotic cells (FITC−/PI+); upper right quadrant, late apoptotic cells (FITC+/PI+). Con A significantly induced apoptosis in hepatocytes, whereas quercetin attenuated this change (D). The apoptosis rate was expressed as the sum of early and late apoptosis rates (E). The ratio of PE (red) J-aggregates and FITC (green) J-monomers is used to represent the mitochondrial membrane potential. The higher the proportion of green, the higher the apoptosis rate, and the lower the red/green ratio, the lower the mitochondrial membrane potential (F). The scale bar is 100 μm in the lower right corner of the image. The result showed that aggregates/monomers MFI decreased significantly in Con A group, and the expression level of JC-1 increased after quercetin treatment (G). Data are expressed as mean ± SD. *P < 0.05, **P < 0.01, ***P < 0.001.
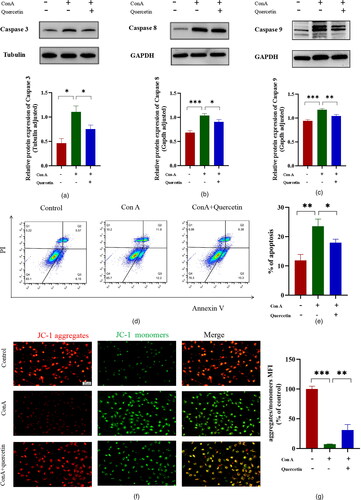
After the cells were examined by flow cytometry, which was used to detect apoptosis, the results revealed that apoptosis was more evident in the Con A group than in the control group and significantly decreased in the Con A + quercetin group compared with the Con A group. There was a significant difference in apoptosis rate among all groups ( and ). The results of this experiment showed that the proportion of green fluorescence was higher in the Con A group and it decreased after quercetin treatment ( and ). This suggests that quercetin in Con A-induced hepatocyte injury may be regulated through inhibiting apoptosis.
3.5. Quercetin inhibits ferroptosis in Brl3a cells
The accumulation of ROS in cells is a typical feature of ferroptosis and a key factor in the induction of ferroptosis. We measured the levels of reactive oxygen species in Brl3a cells by the DCFH probe labelling method. The results showed that, compared with the control group, the ROS expression level of Brl3a in the Con A group was significantly increased. And quercetin or ferrostain-1 efficiently reversed the increase in ROS expression level ( and ). GSH is an important antioxidant in the body, and the essence of ferroptosis is the depletion of GSH. We detected the GSH content in the Brl3a cells, and the results showed that Con A significantly reduced the GSH content, and the treatment with quercetin or ferrostain-1 significantly increased the GSH content (). The Fe content can indicate the degree of ferroptosis. The results showed the Fe content of the cells in the Con A group was significantly increased compared with the control group, while the Fe content was significantly reduced after the cells were treated with quercetin or ferrostain-1 (). MDA can reflect the degree of tissue lipid peroxidation damage. As shown in , Con A significantly increased the Brl3a cells MDA content. When the cells were treated with quercetin or ferrostain-1, the MDA level was significantly reduced compared with the Con A group (). Western blot showed that Con A significantly inhibited the expression of GPX4, and quercetin or ferrostain-1 attenuated this change ( and ). Our findings further suggest that ferroptosis is associated with Con A-induced hepatocyte injury and that quercetin has a role in ameliorating Con A-induced hepatocyte injury, the role of quercetin in ferroptosis is consistent with that of Ferrostatin-1.
Figure 5. Quercetin inhibits hepatocyte ferroptosis induced by Con A.
Representative images of immunofluorescence staining for ROS (green) in Brl3a cells (A). The scale bar is 100 μm in the lower right corner of the image. The quantitative analysis of ROS was detected by a fluorescent enzyme labelling instrument, and the results showed that ROS content increased in the Con A group and decreased after quercetin or ferrostain-1 treatment (B). Those levels of (C) GSH, (D) Fe and (E) MDA in Brl3a, as detected using Elisa assay kits. Western blot showing protein expression of GPX4 in the control, Con A, Con A + quercetin and Con A + Ferrostatin-1 groups. Tubulin was used as a loading control (F, G). The data shown are the mean ± SD. *P < 0.05, **P < 0.01, ***P < 0.001.
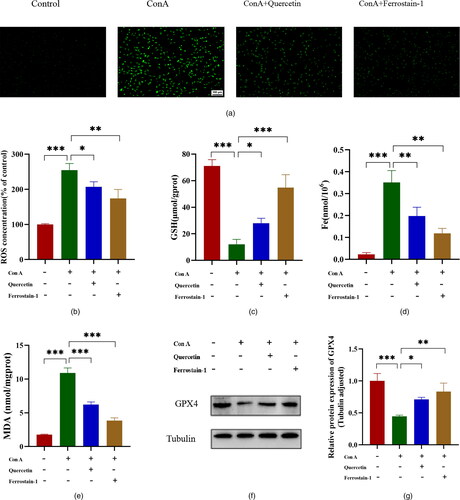
4. Discussion
AIH pathophysiology is characterized by immune-mediated hepatocyte injury associated with the destruction of liver cells, causing inflammation, liver failure, and fibrosis [Citation21,Citation22]. The pathogenesis of AIH is closely related to abnormal immune tolerance. T lymphocytes, B lymphocytes, natural killer cells, and other cells are involved in the pathogenesis of AIH. Among them, NO can play a regulatory role as a messenger molecule in the immune response of AIH. Macrophages can promote the expression of INOS, catabolize arginine, and produce NO. Excessive NO will aggravate oxidative stress and lead to liver dysfunction [Citation23,Citation24]. The current standard therapy exists many shortcomings of AIH. The use of alternative regimens is particularly important for AIH patients who have gastrointestinal intolerance, myelosuppression and other side effects, and those who do not respond well to standard treatment. Basic studies and clinical researches have focused on alternative treatments, and many have been achieved, but the outcomes are still not ideal. Budesonide, mycophenolate mofetil, and calcineurin inhibitors have been used as alternative therapies for AIH. The drug metabolism and mechanism of action of budesonide are similar to those of prednisone, with a high affinity for glucocorticoid receptors. Budesonide has a high first-pass clearance rate in the liver (≥ 90%), and its anti-inflammatory effect is five times as good as that of prednisone, while its metabolites have no glucocorticoid activity [Citation25]. Mycophenolate mofetil is an inosine monophosphate inhibitor. Mycophenolic acid, a metabolite of mycophenolate mofetil, can inhibit the activity of inosine monophosphate dehydrogenase, thereby inhibiting the proliferation of both T and B lymphocytes [Citation26]. Calcineurin inhibitors are a kind of large-ring lactone class immune inhibitors, and their immune inhibitory effect is stronger. They mainly inhibit the activation of CD4+ T lymphocytes, and T helper cells rely on B lymphocyte proliferation, inhibiting the production of a variety of cytokines and the expression of receptors [Citation27]. Therefore, recent scientific research has focused on new alternative drugs for AIH with high efficiency and low toxicity.
As one of the significant ingredients of YCHD, quercetin is a common flavonol with unique biological properties that may improve mental and physical performance and reduce infection risk [Citation28,Citation29]. Several studies have investigated quercetin’s effects on cellular processes involved in different human pathologies [Citation30]. Anti-inflammatory, antioxidant, and anticancer activities are some of the mainly described quercetin mechanisms of action [Citation31]. Besides, the therapeutic potential of this flavonoid has been evaluated in a broad variety of human disorders [Citation32]. However, the study of quercetin in AIH has not yet been reported.
Our research group has conducted transwell co-culture experiments previously, and the technology has been mature and stable. In addition, our previous ALT/AST tests showed that Con A could not directly damage Brl3a cells. Therefore, the co-culture method was adopted in this study to partially simulate the macrophage-induced hepatocyte injury process in vitro. It is well known that ALT and AST levels are commonly used to assess liver and hepatocyte damage [Citation33]. In this study, the co-culture model of J774A.1 and Brl3a was established, Con A acts on the secretion of inflammatory cytokine IL-1β by macrophages, resulting in hepatocyte injury and the release of ALT and AST. The inflammation in AIH is mediated by a wide range of immunoregulatory cytokines. As a key pro-inflammatory cytokine, IL-1β is involved in a variety of autoimmune inflammatory responses and a variety of cellular activities, including cell proliferation, differentiation and apoptosis. Abnormally elevated levels of IL-1β have been detected in the serum and liver of patients with AIH, suggesting the critical role of the proinflammatory cytokine in the pathogenesis of AIH. It has been shown that activated macrophages can accelerate the injury of hepatocytes by the secretion of inflammatory cytokines [Citation34]. In this study, Con A-induced hepatocyte injury was induced by the detection of IL-1β and the increase of ALT and AST levels. Quercetin treatment decreased supernatant ALT and AST levels and IL-1β production. Our study indicated that quercetin plays an anti-inflammatory role in AIH.
Apoptosis is an important process to maintain the homeostasis between cell proliferation and death in normal tissues. Both excessive and insufficient apoptosis can accelerate the development of liver diseases such as acute viral hepatitis, liver fibrosis, liver cancer, and AIH [Citation35,Citation36]. The decline of mitochondrial membrane potential is a landmark event in the early stage of cell apoptosis. Changes in the physiological ultrastructure of mitochondria will lead to cell apoptosis eventually. In this study, excessive apoptosis of hepatocyte was observed in the J774A.1/Brl3a co-culture model induced by Con A. Challenging cells with Con A also dramatically upregulated the pro-apoptotic protein levels of caspases 3, 8, and 9. At the same time, the mitochondrial membrane potential level was significantly increased, and the apoptosis rate was also significantly increased. However, pre-treatment with quercetin significantly inhibited the levels of apoptosis in hepatocytes challenged with Con A, demonstrating the hepatoprotective effects of quercetin. Therefore, the protective effects of quercetin on Con A-induced hepatocyte injury may be mediated by its anti-apoptotic effects.
Ferroptosis is characterized by intracellular lipid peroxide accumulation and redox imbalance. The occurrence of ferroptosis is accompanied by a disordered iron flow and a significant increase in Ros [Citation37,Citation38]. Iron is an essential element, but iron overload can cause various types of cell death, including ferroptosis. The use of small-molecule compounds to regulate ferroptosis and then intervene in the occurrence of related diseases has become a focal point of etiological research and treatment [Citation39]. Thus, our data clearly illustrate that ferroptosis may be the main underlying mechanism that mediates Con A-induced hepatocyte injury. The crucial role of GSH in the regulation of hepatocyte cell death has been documented when an increase in ROS or a depletion of GSH occurs. GPX4 is a glutathione and selenium-dependent glutathione peroxidase that can detoxify lipid hydroperoxides formed during oxidative stress. The results of ROS detection and quantitative analysis in this test showed that there was only a small amount of green fluorescence in the control group. However, the average fluorescence intensity of ROS in the Con A group was significantly higher than that in the control group, indicating that Con A induced the production of a large amount of ROS. Significantly, the ROS fluorescence intensity of cells in the quercetin group was lower than that of cells in the Con A group, suggesting that quercetin can decrease ROS production and further reduce the oxidative damage to cells induced by Con A. Combined with the changes of GPX4, Fe, GSH and MDA contents, it was suggested that quercetin had a certain protective effect on ferroptosis injury induced by Con A.
Our current results revealed that quercetin has a protective effect on hepatocytes, and its mechanism of action may be related to inflammation, apoptosis, and ferroptosis. Due to the low incidence of AIH and relatively little experience in the diagnosis and treatment of traditional Chinese medicine at present, the literature and medical records included in this study are limited, and the results obtained may not be comprehensive enough. First of all, the amount of basic literature selected by data mining is not large enough to sum up the comprehensive understanding of modern doctors about this disease. Secondly, the research in network pharmacology completely relies on the imperfect defects of some network databases, which may lead to bias in the results of network drug analysis. In addition, the predicted treatment of AIH by quercetin, an active ingredient of traditional Chinese medicine, has only been experimentally verified in in vitro cell models, not in vivo animal models or in vitro patient cell experiments.
Therefore, the sample size should be increased in future studies. The next step of this study is to establish a co-culture model of human hepatocyte HL7702 and human macrophage U937 and further verify the hepatocyte protective effect of quercetin in this model. The hepatocyte protective effect of quercetin should also be verified in vivo in animal models to provide a more reliable evidence-based basis for the treatment of AIH with quercetin and improve the scientific results of the study.
5. Conclusion
Our study reveals that quercetin exerts protective effects in Con A-induced AIH hepatocyte model in vitro by mitigating inflammation, apoptosis and ferroptosis. Quercetin significantly inhibits the activation of IL-1β in macrophages to attenuate the inflammatory response, thus eventually alleviating hepatocyte damage. In addition, quercetin inhibits the hepatocytes apoptosis and ferroptosis by activating the Caspase 3/8/9 and GPX4 signaling pathways to alleviate hepatic damage. Therefore, our study assesses the roles of quercetin in Con A-induced hepatocytes injury and provides evidence that quercetin might be a potential therapeutic drug for AIH. Of course, more studies might be needed to do and clarify the effect of quercetin.
Author contributions
Yang Liu and Tiezheng Hou conceived and designed the project. Yiwen Hou completed the main experiments, wrote the paper and analyzed the data. Chen Chen, Zhurong Li Jiawen Wu, Sixue Lyu, Di Guo and Ying Liu assisted in conducting the experiments and analyzing the data. Yang Liu and Tiezheng Hou critically revised the manuscript.
Disclosure statement
No potential competing interest was reported by the authors.
Data availability statement
The data that supports the findings of this study is available from the corresponding author upon reasonable request.
Additional information
Funding
References
- Liu SP, Bian ZH, Zhao ZB, et al. Animal models of autoimmune liver diseases: a comprehensive review. Clin Rev Allergy Immunol. 2020;58(2):252–271.
- Sucher E, Sucher R, Gradistanac T, et al. Autoimmune hepatitis-Immunologically triggered liver pathogenesis-diagnostic and therapeutic strategies. J Immunol Res. 2019;2019:9437043–9437019.
- Horst AK, Kumashie KG, Neumann K, et al. Antigen presentation, autoantibody production, and therapeutic targets in autoimmune liver disease. Cell Mol Immunol. 2021;18(1):92–111.
- Liu Y, Chen H, Hao J, et al. Characterization and functional prediction of the microRNAs differentially expressed in a mouse model of concanavalin A-induced autoimmune hepatitis. Int J Med Sci. 2020;17(15):2312–2327.
- Lee B, Holt EW, Wong RJ, et al. Race/ethnicity is an independent risk factor for autoimmune hepatitis among the San Francisco underserved. Autoimmunity. 2018;51(5):258–264.
- Czaja AJ. Immune inhibitory proteins and their pathogenic and therapeutic implications in autoimmunity and autoimmune hepatitis. Autoimmunity. 2019;52(4):144–160.
- Tsutsui H, Nishiguchi S. Importance of Kupffer cells in the development of acute liver injuries in mice. Int J Mol Sci. 2014;15(5):7711–7730.
- Wang HX, Liu M, Weng SY, et al. Immune mechanisms of concanavalin a model of autoimmune hepatitis. World J Gastroenterol. 2012;18(2):119–125.
- Zhang P, Yin Y, Wang T, et al. Maresin 1 mitigates concanavalin A-induced acute liver injury in mice by inhibiting ROS-mediated activation of NF-kappa B signaling. Free Radic Biol Med. 2020;147:23–36.
- Wang Y, Zhang W, Xu Y, et al. Extracellular HMGB1 impairs macrophage-mediated efferocytosis by suppressing the Rab43-controlled cell surface transport of CD91. Front Immunol. 2022;13:767630.
- Snijders R, Gevers TJG, Heneghan MA, et al. Reply to: correspondence on ‘systematic review of response criteria and endpoints in autoimmune hepatitis by the international autoimmune hepatitis group: defining endpoints that guide treatment in autoimmune hepatitis. J Hepatol. 2022;77(5):1464–1466.
- Li H. Advances in anti-hepatic fibrotic therapy with traditional Chinese medicine herbal formula. J Ethnopharmacol. 2020;251:112442.
- Hsueh TP, Tsai TH. Preclinical study of simultaneous pharmacokinetic and pharmacodynamic herb-drug interactions between Yin-Chen-Hao-Tang and spironolactone. BMC Complement Med Ther. 2020;20(1):253.
- Tian F, Ruan QJ, Zhang Y, et al. Quantitative analysis of six phenolic acids in Artemisia capillaris (Yinchen) by HPLC-DAD and their transformation pathways in decoction preparation process. J Anal Methods Chem. 2020;23:8950324.
- Wu YL, Li ZL, Zhang XB, et al. Yinchenhao decoction attenuates obstructive jaundice-induced liver injury and hepatocyte apoptosis by suppressing protein kinase RNA-like endoplasmic reticulum kinase-induced pathway. World J Gastroenterol. 2019;25(41):6205–6221. 7
- Read MA. Flavonoids: naturally occurring anti-inflammatory agents. Am J Pathol. 1995;147(2):235–237.
- Orsolić N, Knezević AH, Sver L, et al. Basic I. Immunomodulatory and antimetastatic action of propolis and related polyphenolic compounds. J Ethnopharmacol. 2004;94(2-3):307–315.
- Chirumbolo S. The role of quercetin, flavonols and flavones in modulating inflammatory cell function. Inflamm Allergy Drug Targets. 2010;9(4):263–285.
- Dixon SJ, Lemberg KM, Lamprecht MR, et al. Ferroptosis: an iron-dependent form of nonapoptotic cell death. Cell. 2012;149(5):1060–1072.
- Jiang X, Stockwell BR, Conrad M. (2021). Ferroptosis: mechanisms, biology and role in disease. Nat Rev Mol Cell Biol. 2021;22(4):266–282.
- Liu Y, Hao H, Hou T. Concanavalin A-induced autoimmune hepatitis model in mice: mechanisms and future outlook. Open Life Sci. 2022; 2817(1):91–101.
- Hou Y, Liu Y, Chen C, et al. Phenotypic profiling of CD279 and CD185 level on helper T cells in patients with autoimmune hepatitis. Scand J Immunol. 2022;97(1):e13230.
- Gantner BN, LaFond KM, Bonini MG. Nitric oxide in cellular adaptation and disease. Redox Biol. 2020;34:101550.
- MacMicking J, Xie QW, Nathan C. Nitric oxide and macrophage function. Annu Rev Immunol. 1997;15(1):323–350.
- Halliday N, Dyson JK, Thorburn D, et al. Review article: experimental therapies in autoimmune hepatitis. Aliment Pharmacol Ther. 2020;52(7):1134–1149.
- Terziroli Beretta-Piccoli B, Mieli-Vergani G, Vergani D. Autoimmune hepatitis: standard treatment and systematic review of alternative treatments. World J Gastroenterol. 2017;23(33):6030–6048.
- Gelow K, Chalasani S, Green K, et al. Utilization and impact of complementary and alternative medicines in symptomatic autoimmune hepatitis patients. Dig Dis Sci. 2022;67(7):2891–2898.
- Liu J, Ma Z, Li H, et al. Chinese medicine in the treatment of autoimmune hepatitis: progress and future opportunities. Animal Model Exp Med. 2022;5(2):95–107.
- Xu D, Hu MJ, Wang YQ, et al. Antioxidant activities of quercetin and its complexes for medicinal application. Molecules. 2019;24(6):1123.
- Li Y, Yao J, Han C, et al. Quercetin, inflammation and immunity. Nutrients. 2016;8(3):167.
- Jafarinia M, Sadat Hosseini M, Kasiri N, et al. Quercetin with the potential effect on allergic diseases. Allergy Asthma Clin Immunol. 2020;16(1):36.
- Song X, Wang Y, Gao L. Mechanism of antioxidant properties of quercetin and quercetin-DNA complex. J Mol Model. 2020;26(6):133.
- Hyder MA, Hasan M, Mohieldein AH. Comparative levels of ALT, AST, ALP and GGT in liver associated diseases. Eur. J. Exp. Biol. 2013;3(2):280–284.
- Heymann F, Hamesch K, Weiskirchen R, et al. The concanavalin a model of acute hepatitis in mice. Lab Anim. 2015;49(1 Suppl):12–20.
- Yan L, Luo H, Li X, et al. d-Pinitol protects against endoplasmic reticulum stress and apoptosis in hepatic ischemia-reperfusion injury via modulation of AFT4-CHOP/GRP78 and caspase-3 signaling pathways. Int J Immunopathol Pharmacol. 2021;35:20587384211032098. 20587384211032098.
- Guicciardi ME, Gores GJ. Apoptosis as a mechanism for liver disease progression. Semin Liver Dis. 2010;30(4):402–410.
- Hirschhorn T, Stockwell BR. The development of the concept of ferroptosis. Free Radic Biol Med. 2019;133:130–143.
- Su LJ, Zhang JH, Gomez H, et al. Reactive oxygen species-induced lipid peroxidation in apoptosis, autophagy, and ferroptosis. Oxid Med Cell Longev. 2019;2019:5080843.
- Ding Y, Chen X, Liu C, et al. Identification of a small molecule as inducer of ferroptosis and apoptosis through ubiquitination of GPX4 in triple negative breast cancer cells. J Hematol Oncol. 2021;14(1):19.