ABSTRACT:
Breast cancer represents a collection of pathologies with different molecular subtypes, histopathology, risk factors, clinical behavior, and responses to treatment. “Basal-like” breast cancers predominantly lack the receptors for estrogen and progesterone (ER/PR), lack amplification of human epidermal growth factor receptor 2 (HER2) but account for 10–15% of all breast cancers, are largely insensitive to targeted treatment and represent a disproportionate number of metastatic cases and deaths. Analysis of interleukin (IL)-3 and the IL-3 receptor subunits (IL-3RA + CSF2RB) reveals elevated expression in predominantly the basal-like group. Further analysis suggests that IL-3 itself, but not the IL-3 receptor subunits, associates with poor patient outcome. Histology on patient-derived xenografts supports the notion that breast cancer cells are a significant source of IL-3 that may promote disease progression. Taken together, these observations suggest that IL-3 may be a useful marker in solid tumors, particularly triple negative breast cancer, and warrants further investigation into its contribution to disease pathogenesis.
Introduction
Breast cancer is the most common cancer in females (11.7% of total cases) representing 24.5% of all female cancers diagnosed and contributes to the highest rate of mortality amongst women (15.5%) (Sung et al. Citation2021). Although breast cancer is commonly referred to as a single disease, it represents a collection of pathologies with different molecular subtypes, histopathology, risk factors, clinical behavior, and responses to treatment (Morigi Citation2017; Pasha and Turner Citation2021). Molecular subtyping of tumors has stratified breast cancer into five main groups: luminal A, luminal B, human epidermal growth factor receptor (HER2) enriched, basal-like, and normal-like (Perou et al. Citation2000; Sørlie et al. Citation2001). Breast tumors of the luminal subtypes (A or B) account for 70% of all mammary carcinomas and most express the hormone receptors for estrogen (ER), progesterone (PR) or have enrichment of HER2. In contrast, 10–15% of women carry tumors of the basal-like subtype and ∼70–80% of these cancer cells do not express ER, PR or HER2 and are thus defined as triple-negative breast cancer (TNBC) (Lehmann et al. Citation2011; Sørlie et al. Citation2001). These stratifications have defined predictive characteristics and prognostic outcomes that also guide treatment options. For example, the standard of care for patients with ER-positive and HER2-amplified tumours is endocrine therapy and anti-HER2 therapy respectively (Pasha and Turner Citation2021). By contrast, the TROP2 antibody drug conjugate and anti-PD-1/L1 immune checkpoint therapies have shown benefit to some TNBC patients (Cortes et al. Citation2020; Pasha and Turner Citation2021; Schmid et al. Citation2018). TNBC is associated with a disproportionate number of metastatic cases and deaths associated with breast cancer (Liedtke et al. Citation2008; Sørlie et al. Citation2001). Clearly, a better understanding of the heterogeneity and pathogenesis of TNBC is warranted to uncover opportunities for targeted therapies and improved survival outcomes.
Interleukin-3 (IL-3) is a pluripotent cytokine (largely the product of activated T cells (Hercus et al. Citation2017; Varricchi et al. Citation2021)) which signals through its receptor (IL-3Rα:IL-3Rβ (IL3RA:CSF2RB)) to act on immune and non-immune cells. An example of the latter and highly relevant to breast cancer is the demonstration that IL-3 increases endothelial cell (EC) proliferation, migration, and the formation of new blood vessels (Dentelli et al. Citation1999; Korpelainen et al. Citation1996; Moldenhauer et al. Citation2015). In murine models of cancer, Brizzi et al have documented that IL-3 production by tumor-associated ECs (TECs) can increase blood vessel formation in vivo (Dentelli et al. Citation2005), and, importantly, that IL-3Rα blockade inhibits TEC release of pro-angiogenic extracellular vesicles (Lombardo et al. Citation2018) to reshape the anti-tumor immune response (Lopatina et al. Citation2022), and reduces metastatic spread of TNBC cells (Lopatina et al. Citation2020), thus clearly implicating IL-3 and its receptor in the pathogenesis of TNBC. Whether IL-3 is produced by the breast cancer cells themselves has remained a unanswered question.
In this study we compare the expression of IL-3 and its receptor components IL3RA and CSF2RB across breast cancer subtypes and their relationship with patient outcome. Immunohistochemistry is utilized to detect IL-3 and the IL-3R protein in tumor biopsies and patient-derived xenografts reveal whether the cancer cells themselves produce IL-3 and express the IL-3 receptors for a potential autocrine feedback loop.
Materials and methods
Ethics statement
The use of archived human breast cancer samples stored as formalin fixed paraffin embedded (FFPE) blocks was approved by the Human Research Ethics Committees of the Royal Adelaide Hospital (RAH) HREC/14/RAH/190. Animal experiments were approved by the Animal Ethics Committee of the Garvan Institute of Medical Research (ARA 21-10) and conform to the guidelines established by the “Australian Code of Practice for the Care and Use of Animals for Scientific Purposes”.
In silico analysis of human breast cancer survival
Survival analysis for breast cancer patients in relation to gene expression for IL3RA, CSF2RB, IL3, IL5 and CSF2 (GM-CSF) was assessed using BreastMark; an algorithm designed to interrogate gene expression data from ∼17,000 genes and clinical data from >4700 breast cancer samples; including luminal A (n = 1678), luminal B (n = 1519), HER2 (n = 423) and basal-like (n = 714) subtype (Madden et al. Citation2013). Disease-free survival (DFS, survival without occurrence), distant disease-free survival (DDFS, survival without metastasis) and overall survival (OS) were chosen as survival end points. Dichotomization into “high” and “low” expression is based on median gene expression levels. When genes are combined, high expression samples are characterized as those samples with greater median expression of each of the genes involved e.g. samples with greater than median expression levels of each of IL3, IL3RA and CSF2RB are classed as “high” expression. Survival curves are based on Kaplan-Meier estimates and the log-rank P-value is shown. Hazard ratios were calculated by Cox-regression analysis.
Immunohistochemistry of human tumors
Archived FFPE human breast cancer tissue sections were obtained from SA Pathology following human ethics approval and examined by immunohistochemistry (IHC). Similarly, human breast cancer tissue microarrays (TMAs #BR1201 & #BR10010e) were purchased (US Biomax, Rockville, MD, USA) and examined by IHC.
All in vivo experiments, procedures and endpoints were approved by the Garvan Institute of Medical Research Animal Ethics Committee (protocol ARA 21-10). Patient-derived xenografts (PDXs) were established using published methods (Johnson et al. Citation2016) wherein 1mm3 of human TNBC tumor pieces (harvested from primary (n = 13), residual disease (n = 4) or metastatic (n = 7) sites) were implanted into the cleared 4th mammary fat pads of 6–8 week old female NOD-SCID-IL2Rγc−/− (NSG) mice (Australian BioResources Pty Ltd, Perth, WA, AUS). Established tumors were harvested and processed as FFPE with 4 µm tissue sections by IHC and scored for staining intensity in a blinded manner.
FFPE tissue samples were dewaxed and antigen retrieval performed using citrate buffer pH6.5 prior to tissues being treated with 1% H2O2 for 10 min, blocked with either 10% normal rabbit serum (NRS) or 10% normal goat serum (NGS) in phosphate buffered saline (PBS) for 1 hour at room temperature (RT). Primary antibodies (IL-3, mouse IgG1, clone 3B11, Genetex, cat#GTX84295, 1:50 dilution; IL-3Rα (CD123), mouse IgG1, clone 32703, R&D Systems, cat#MAB301, 15 mg/ml; IL-3Rβ, mouse IgG1, clone 1C1, generated by Lopez laboratory, 15 mg/ml; isotype control, mouse IgG1, BD Pharmingen, cat#555746, used at appropriately matched concentrations; GM-CSF (CSF2), mouse IgG2b, clone OTI1A3, Origene, cat#TA808006, 1:150 (6.6 mg/ml); IL-5, rabbit polyclonal, (without antigen retrieval), Origene, cat#PP1027P2, 1:500 (1 mg/ml); PECAM-1 (CD31) M-20, goat polyclonal, Santa Cruz, sc-1506, 1:1000) diluted in PBS with 3% serum and incubated overnight at 4 °C. Secondary biotin antibodies (Goat anti-mouse, BD Pharmingen, cat#553999; rabbit anti-goat, Abcam, cat#AB5753; mouse anti-rabbit, BD Pharmingen, cat#550938, 1:500) were prepared in PBS with 3% serum and samples were incubated for 35 min at RT. Elite ABC kit and the DAB kit (cat#PK6-100 & SK-4100, Vectastain, Vector Laboratories, Burlingame, CA, USA) were used as per manufacturer’s instructions. Periodic Acid-Schiff (PAS) staining was performed following manufacturer’s instructions (cat# 395B-1KT, Sigma Aldrich, St Louis, Missouri, USA). Slides were then counterstained with hematoxylin (cat#S3309, DAKO, Denmark) and imaged for brightfield using NanoZoomer (Hamamatsu Photonics K.K., Hamamatsu, Japan) and for fluorescence using Axioscan (Zeiss, Oberkochen, Germany). For IL-3, IL-3Rα and IL-3Rβ, a blinded semiquantitative scoring system was employed whereby staining intensity was tallied manually on a 0-3 scale (0 = neg, 1 = low, 2 = medium, 3 = high intensity). Only TNBC samples on the TMAs were included in the analysis. For tumor vasculature (and also blinded), CD31+ blood vessels were quantified as a percentage of total tumor area using ImageJ (National Institutes of Health) software. Similarly, for quantification of VM, fluorescence emission from the PAS stain was detected in the orange channel with images converted to TIFF via the Zen software (Zeiss), split into RGB channels and the red channel image converted into a black and white 8-bit image. Using ImageJ, the threshold was adjusted to a value which accurately identified the fluorescent PAS networks with the “dark background” option selected (i.e. min 20, max 200). The tumor section was defined using a pentagon and the “measure” tool was used to determine the % area above the threshold. The final “VM score” represents the mean fluorescence value per tumor section.
Statistical analysis
For Kaplan-Meier estimates, the log-rank P-value is shown with hazard ratios calculated by Cox-regression analysis. Statistical analyses of IHC were performed using GraphPad Prism version 8.3.0 software (GraphPad, San Diego, CA, USA) using an ordinary one-way ANOVA with multiple comparisons, a paired t-test for patient matched primary and lymph node metastases or a Mann-Whitney test for unrelated sample comparisons. Results with p < 0.05 were considered to be statistically significant.
Results
Selective elevation of IL-3 in a subset of breast cancer patients with poor survival outcome
To investigate the potential contribution of IL-3 and the IL-3R in the pathogenesis of breast cancer, we utilized the publicly available resource BreastMark. This algorithm interrogates the expression of one or more genes against survival outcomes via access to 26 publicly available datasets which cover ∼17,000 genes in up to 4738 patient samples (Madden et al. Citation2013). Notably, the survival end points included disease-free survival (DFS, survival without occurrence), distant disease-free survival (DDFS, survival without metastasis) as well as overall survival (OS) (Madden et al. Citation2013). The swimmer plot in shows that for all patients diagnosed with breast cancer, tumoral IL3+IL3RA+CSF2RB levels that were above the median values were associated with a significantly reduced survival. Further separation into breast cancer subtypes revealed that only patients with luminal A or basal-like tumors experienced a significant reduction in survival when there was high expression of IL3+IL3RA + CSF2RB, and this was not evident for patients with luminal B or HER2 enriched tumors ().
Figure 1. IL-3, IL-3R, IL-5 and GM-CSF expression by human breast cancer subtypes.
(A) Swimmer plots representing gene expression of IL3+IL3RA+CSF2RB combined in breast cancer patients as a whole or separated into the molecular subtypes (luminal A, luminal B, HER2 enriched or basal-like) with number of patients, hazard ratio (HR) and the log-rank p value calculated by Cox-regression analysis; p < 0.05 considered significant. (B) Kaplan-Meier plots of the luminal A (Lum A, n = 1678), or basal-like subtype (n = 714) of breast cancer patients for gene expression of either IL3RA+CSF2RB, IL3, IL5 or CSF2 with high (blue) versus low (red) based on median expression levels, with HR and log-rank p value calculated by Cox-regression analysis; p < 0.05 considered significant. (C) Representative IHC analysis of IgG1 isotype control or antibodies targeting IL-3, IL-3Rα, IL-3Rβ, GM-CSF or IL-5 protein expression by human TNBC tumor tissue. DAB staining (brown) and hematoxylin counterstain (blue). White scale bar = 100µm. Yellow scale bar = 50µm.
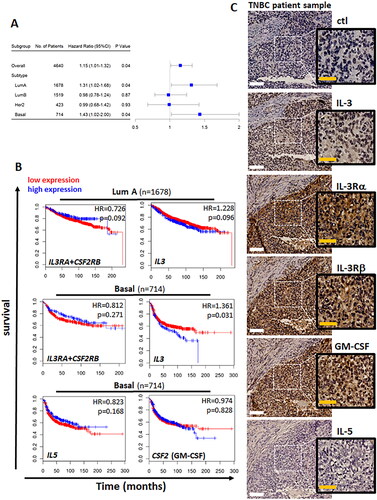
We next evaluated if IL3 or its receptor components (IL3RA + CSF2RB) were predictors of reduced survival for patients with luminal A or basal-like tumors. Elevated expression of IL3RA + CSF2RB did not have an impact on survival for patients with either luminal A or basal-like tumors. In contrast, elevated expression of IL3 was associated with poor survival outcomes for patients with basal-like tumors, an effect that was not observed in the luminal A tumors (). Since CSF2RB of the IL-3 receptor is also the receptor for IL-5 and GM-CSF, we investigated their possible association with basal-like breast cancer outcomes, however, no significant association was observed (). Taken together, these results suggest that IL-3 is a predictor of poor survival for patients with the basal-like breast cancer.
IL-3 and the IL-3R proteins are detectable in tumor samples by immunohistochemistry
To determine whether IL3, IL3RA and CSF2RB are translated into proteins, we performed immunohistochemistry (IHC) on basal-like TNBC samples. shows serial sections of a representative TNBC tumor with readily detectable protein for IL-3, IL-3Rα and IL-3Rβ. The tumor tissue also stained positive for GM-CSF, but not IL-5. In healthy breast tissue, IHC revealed restricted expression of IL-3, IL-3Rα and GM-CSF to ductal epithelial cells and very little to no staining for IL-3Rβ or IL-5 (). Notably, Supplementary Figure S1B confirmed antibody recognition via positive control tissues.
To investigate an association between tumor size and expression of IL-3, IL-3Rα or IL-3Rβ, a tissue microarray (TMA, #BR1201) with two cores from each of 41 TNBC patients was examined. These duplicate cores represented tumors of various sizes (i.e., n = 3 for T1 ≤ 2cm, n = 32 for T2 = 2–5cm, n = 3 for T3 > 5cm and n = 5 for T4 = spread beyond primary tumor) and a blinded scoring system wherein staining intensity was tallied on a 0–3 scale (and averaged per patient), revealed a range of expression levels for IL-3, IL-3Rα and IL-3Rβ across different tumor samples (). Data from a second TMA (#BR10010e, containing two cores from 12 TNBC patient matched primary and lymph node metastatic tumors) suggests that within a given patient, the expression of IL-3/IL-3R can vary slightly, but not significantly, between the primary and metastatic tissue (, where the average score of the two cores is presented per patient sample). Interestingly, this small cohort of patients did reveal a significant reduction in IL-3Rβ expression in the metastatic tumor; additional patient samples are required to build confidence in this observation.
Figure 2. Human TNBC TMAs – IL-3/IL-3R IHC scores –T1-T4, primary and metastases.
(A) Representative IHC of IL-3, IL-3Rα and IL-3Rβ protein expression by human TNBC TMA (#BR1201) across patients grouped in T1-4. DAB staining (brown) and hematoxylin counterstain (blue). Staining intensity scored for IL-3 (blue), IL-3Rα (black) or IL-3Rβ (green) with results shown as scores averaged from two cores for each of the 43 different tumor samples (T1 n = 3, T2 n = 32, T3 n = 3, T4 n = 5) mean ± sem with statistical analysis using one way ANOVA with multiple comparisons and p < 0.05 considered significant. White scale bar = 100µm. (B) TMA (#BR1001e) staining intensity scored for IL-3 (blue), IL-3Rα (black) or IL-3Rβ (green) for patient matched primary (open symbols) or metastasis sections (closed symbols). Results shown as scores averaged from two cores for each of the 12 different patient samples that are linked by a solid line from the patient primary to metastatic tumor. A paired t-test was performed for the patient matched primary and metastatic lesions with p < 0.05 considered significant.
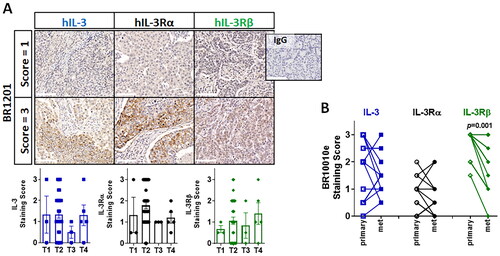
To confirm that IL-3 and its receptor subunits are expressed on breast cancer cells and not closely associated immune cells, we took advantage of the fact that IL-3 has very low homology between mouse and human (Manz Citation2007) and that the anti-IL-3 antibody (clone 3B11) recognizes human IL-3 only. Here, we investigated 24 different human TNBC patient-derived xenografts (PDXs, 13 derived from primary tissue and 11 from residual disease or metastasis) passaged in immune compromised NSG mice for IL-3 production by breast cancers. shows IHC with detectable IL-3 and the IL-3R subunits in human breast cancer cells harvested from both primary tumors as well as residual disease and metastases. Interestingly, while these are samples from unrelated donor samples, this small cohort suggests that there may be an increase in IL-3Rα expression with disease progression. Increasing sample sizes will be required to support this notion. Given that the PDX tumors are in an immune cell free environment, this provides direct evidence that IL-3 and its receptor subunits are expressed by TNBC cells.
Figure 3. Human TNBC PDXs – IL-3/IL-3R IHC scores – primary and metastasis.
Representative IHC of IL-3, IL-3Rα and IL-3Rβ protein expression by human TNBC PDXs. DAB staining (brown) and hematoxylin counterstain (blue). Staining intensity scored for primary (n = 13 tumors, open shapes) or residual disease/metastasis sections (n = 11 tumors, closed shapes) with results shown as individual scores for the 24 different tumors. A two-tailed Mann-Whitney test compared the primary and residual/metastatic lesions with p < 0.05 considered significant. Yellow scale bar = 50µm.
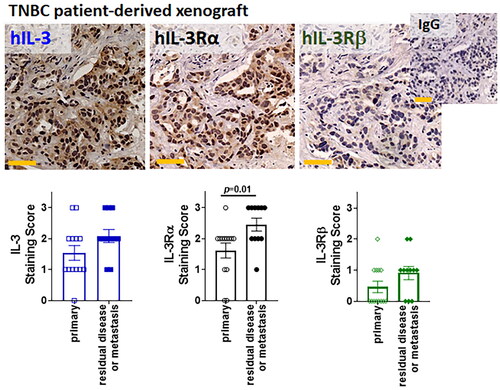
Comparable expression of IL-3 and its receptor subunits in healthy mammary ducts, DCIS and IDC
To address the unmet need to identify the ∼40% of patients with ductal carcinoma in situ (DCIS) who will progress to invasive ductal carcinoma (IDC) (Casasent, Edgerton, and Navin Citation2017), we investigated whether IL-3, IL-3Rα or IL-3Rβ might serve as a point for separation. Together with benign tissue (obtained following reduction mammoplasty or benign breast tissue with concurrent invasive carcinoma) IHC was used to compare expression levels of IL-3, IL-3Rα and IL-3Rβ across healthy, DCIS and IDC tissue. Using the aforementioned scoring system, shows that IL-3 and its receptor components are expressed in the mammary ducts of benign tissue as well as DCIS and IDC tumors. Also shown in , little/no protein was detected in the stroma, however IL-3, IL-3Rα and IL-3Rβ were all detected at similar levels in both DCIS and IDC tissues. Notably, while no difference was observed between DCIS and IDC samples, further analysis is required to determine whether IL-3-high DCIS tumors will progress to invasive disease.
Figure 4. Human breast tissue (benign, DCIS and IDC) – IL-3/IL-3R IHC scores – ducts, stroma, tumor tissue.
Staining intensity scored for IL-3 (blue), IL-3Rα (black) or IL-3Rβ (green) with results shown for ducts, stroma and tumor in benign breast tissue (n = 8, closed circles), DCIS (n = 18, closed squares) and IDC (n = 17, closed triangles). Results shown as individual sample scores with mean ± sem. Statistical analysis using one way ANOVA with multiple comparisons with p < 0.05 considered significant. N/A = not applicable.
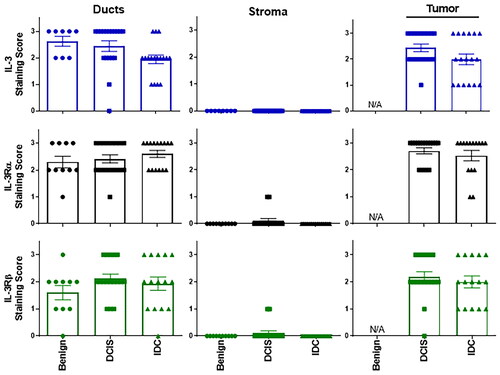
Elevated expression of IL-3 does not correlate with increased tumor vasculature
Finally, given the documented role for IL-3 in angiogenesis (Dentelli et al. Citation1999; Korpelainen et al. Citation1996; Moldenhauer et al. Citation2015), we examined whether levels of IL-3 within IDC tumors could be an indicator of tumor vasculature. To address this, we first separated the 17 IDC samples into groups that scored either neg/low (scores of 0-1), medium (score of 2) or relatively high (score of 3) levels of IL-3 by IHC. shows that 35% (6 of 17) of the IDC samples are negative, or low for IL-3 expression, 30% (5 of 17) have medium expression and 35% (6 of 17) have comparatively high expression of IL-3. Notable exclusion from this scoring was IL-3 expression observed within leukocytes distal to the tumor (, left panel bottom image). Tumor vasculature was then identified via IHC staining of CD31+ endothelial cells and percent area positive for CD31 determined using ImageJ software. shows (for this small cohort of samples) that CD31+ tumor vasculature did not significantly differ. With a documented role for IL-3 to promote vasculogenic mimicry (VM) by cancer cells (Koni et al. Citation2022), we examined whether IL-3 expression levels coincided with varied VM content in our patient cohort. To achieve this, Periodic Acid-Schiff (PAS) reagent was used to stain basement membrane structures (including those lining VM channels) magenta and also generates an autofluorescence signal that can be quantified via ImageJ to indicate VM networks in tumors (Tan et al. Citation2016). shows PAS-generated fluorescence images detected in the red channel for two representative patient samples. Segregation of the VM scores into tumor groups previously determined to express IL-3 at neg/low, medium or high levels, suggest that increasing IL-3 supports increased VM content.
Figure 5. Human IDC sections, IL-3 staining intensity and tumor vasculature.
(A) Representative IHC analysis of IL-3 protein expression by human IDC tumor tissue scored as blinded samples within an arbitrary 0–3 for negative-high DAB stain (brown) intensity with hematoxylin counterstain (blue). Black scale bar = 250µm. Right pie chart is the cumulative % scores for the neg/low, medium and high staining intensity of the 17 patient samples. Orange framed insert shows IL-3-positive T cells and bottom right is the isotype control staining. (B) Representative IHC analysis of CD31 staining of tumor vasculature in the aforementioned human IDC tumor samples previously scored 0-3 for negative-high IL-3 expression. Black scale bar = 250µm. The bar graph shows ImageJ quantified % CD31+ area detected across the 11 patient samples and grouped for IL-3 expression levels (white bar = neg/low (n = 3); light blue bar = medium (n = 5) and dark blue bar = high IL-3 (n = 3)). High magnification images illustrate CD31+ endothelial cells. (C) Representative PAS-stained fluorescence images of the aforementioned human IDC tumor samples previously scored 0–3 for negative-high IL-3 expression. The bar graph shows ImageJ quantified mean VM score for n = 11 patient samples grouped for IL-3 expression levels (white bar = neg/low (n = 3); light blue bar = medium (n = 5) and dark blue bar = high IL-3 (n = 3)). Statistical analysis using one way ANOVA with multiple comparisons, p < 0.05 considered significant.
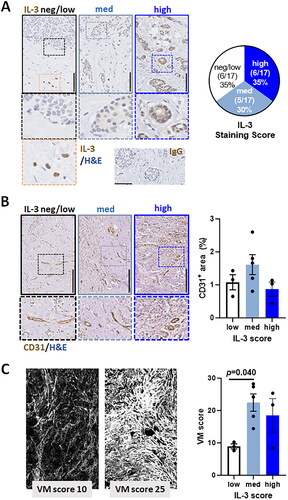
Discussion
While expression of cytokines and their receptors, such as IL-3, has proven useful in the identification and treatment of hematological malignancies (Laszlo et al. Citation2022), further stratification of breast cancer by cytokine expression has been limited. Here we show that the expression of IL-3 and the IL-3R subunits (IL-3 + IL-3RA + CSF2RB) was selectively elevated in the luminal A (HR 1.31, p < 0.04) and basal (HR 1.43, p < 0.04) breast cancer subtypes. Further investigation revealed that the IL-3 cytokine itself, rather than the IL-3R subunits, was associated with poor patient survival in basal-like tumors. Immunohistochemistry of human breast cancer biopsies and PDX samples supports the notion that breast cancer cells produce IL-3 and that it may be associated with disease progression. Building on this study, further investigation of IL-3 within a larger cohort of TNBC (that will be inherently heterogeneous) would benefit from an extension of our semiquantitative assessment of intensity staining (scored as 0–3) to a broader dynamic range such as H-score which includes intensity staining and the percentage of IL-3 positive cells (scored as 0–300).
IL-3 is a strongly restricted cytokine with activated T lymphocytes (Th1, Th2, CD8+ and Tregs) being the largest cellular source. However, IL-3 production can also be observed in transformed cells to potentially enhance cell viability and proliferation of cancer cells (Vellenga et al. Citation1987). Progenitor cells in acute myeloid leukemia overexpress the IL-3Rα (Jin et al. Citation2009; Jordan et al. Citation2000), which has been shown to provide a cell survival and proliferation advantage in vitro and poor outcomes for patients (Testa et al. Citation2002). Interestingly, IL-3 was recently deemed a key factor in the spontaneous genesis and subsequent maintenance of acute myeloid leukemic cells (Bulaeva et al. Citation2020). Our study raises the possibility that the pathogenic role of IL-3 in cancer may not be limited to leukemia but may also have an oncogenic role in solid tumors, particularly basal-like breast cancer. While initial IHC on patient samples could not discern between cancer cell and immune cell derived IL-3, the patient-derived xenografts established in immunocompromised mice, combined with the use of a human specific anti-IL-3 antibody, provide some evidence that this immune modulatory factor is cancer cell generated. The interplay between this and other stromal cells is yet to be fully elucidated. Of interest, tumor associated leukocytes also produce IL-3 and thereby further elevate local levels. This is exemplified by myeloid-derived suppressor cells and Tregs (both of which are poor prognosis factors) that are elevated in number in TNBC (Savas et al. Citation2018) and can secrete IL-3 (Sharma et al. Citation2018).
With previous documentation that IL-3 is pro-angiogenic (Dentelli et al. Citation1999; Citation2004; Citation2005; Citation2011; Korpelainen et al. Citation1993; Moldenhauer et al. Citation2015), Dentelli et al reported that, like ECs in normal healthy vasculature, tumor-derived ECs (TECs) respond to IL-3 with increased cell survival and increased expression of adhesion molecules E-selectin and intercellular adhesion molecule-1 (Dentelli et al. Citation2011). In murine models of cancer, Brizzi and team have documented that IL-3 promotes tumor vasculature in vivo (Dentelli et al. Citation2005), and that IL-3Rα blockade inhibits TEC release of pro-angiogenic extracellular vesicles (Lombardo et al. Citation2018) to reshape the anti-tumor immune response (Lopatina et al. Citation2022) and reduce metastatic spread of TNBC cells (Lopatina et al. Citation2020). In high grade breast cancer lesions, IL-3-producing tumor infiltrating lymphocytes (CD25+CD4+CD5+) have a paracrine effect on ECs and smooth muscle cells to support the stabilization of tumor vasculature (Dentelli et al. Citation2004). Although we did not detect a correlation between levels of IL-3 and CD31+ tumor vasculature, we attribute this to our small sample number rather than evidence against the current literature. Interestingly, we observed a correlation between PAS-stained basement membrane structures (indicative of VM networks (Warso et al. Citation2001; Tan et al. Citation2022; Tan et al. Citation2016)) with increased intra-tumoral IL-3. This supports a publication by the Brizzi laboratory who reported that human TNBC cell lines increase VM capability in response to IL-3 (in vitro and in vivo) (Koni et al. Citation2022).
Precisely how IL-3, IL-3Rα and IL-3Rβ are upregulated in breast cancer is yet to be determined, but it is well documented that hypoxia-inducible factor 1 α-subunit (HIF1-α) is hyperactivated in TNBC (Cancer Genome Atlas 2012; Mahara et al. Citation2016). A link between hypoxia and IL-3 has not been formally documented but our preliminary investigation of the promoter regions of IL-3 and its receptors reveals that all their promoters contain multiple HIF response elements (HRE; GCGTG or ACGTG). More specifically, two putative HREs exist within 1861 bp of the IL-3 transcriptional start site; seventeen putative HREs within 11,027bp of the IL-3Rα transcriptional start site, and three putative HREs within 4926 bp of the IL-3Rβ transcriptional start site. Investigating hypoxia and the tumor microenvironment may unveil the underlying molecular mechanisms responsible for elevated IL-3/IL-3R expression in breast cancer.
The TNBC subtype of breast cancer is intrinsically a diverse group of cancers and further subtyping may identify new molecular-based therapies. To this end, Lehmann and colleagues published a gene expression meta-analysis of 587 TNBC cases and propose four TNBC subtypes (Lehmann et al. Citation2011). Briefly, enriched gene expression signatures clustered into (i) basal like 1 & basal like 2 (∼35% of TNBC) that exhibit features of enhanced cell cycle and DNA repair responses, (ii) mesenchymal & mesenchymal stem-like (∼25% of TNBC) with enriched features of epithelial-to-mesenchymal transition and growth factor pathways, (iii) immunomodulatory (∼18% of TNBC) with elevated immune cell signaling and processes, (iv) luminal androgen receptor (∼10% of TNBC) with features linked to androgen receptor signaling and the remaining ∼10% of TNBC undefined (Lehmann et al. Citation2011). Notably, “IL-3 pathway” was listed in the gene ontologies for the suggested immunomodulatory and mesenchymal stem-like subtypes, providing evidence that IL-3 and its receptor expression may help stratify subsets of TNBC. A link between IL-3 and features of stemness by TNBC cells draws similarities to an in vitro prostate cancer study wherein IL-3 promoted expression of stemness factors SOX2 and CD44 in addition to the drug resistance gene ABCG (Yu et al. Citation2015).
These findings suggest that IL-3 mRNA and protein levels are elevated in breast cancer subtypes, particularly TNBC. With supporting literature by others that TNBC cells express the IL-3 receptor (Koni et al. Citation2022), our observation of IL-3 production by TNBC cells adds an important component to our understanding of this deadly disease. Further investigation could provide an opportunity to consider a personalized treatment approach for a subset of TNBC patients by, for example, tumoral administration of an anti-IL-3 antibody. Targeting the IL-3/IL-3R axis has already been achieved with anti-IL-3R drugs such as Tagraxofusp-erzs (ElzonrisR, a recombinant fusion protein composed of human IL-3 fused to truncated diphtheria toxin payload (Frankel et al. Citation2014)) demonstrating clinical responses in patients with haematological malignancies (Pemmaraju et al. Citation2019; Syed Citation2019). Similarly, Talacotuzumab, a humanized anti-IL-3Rα monoclonal antibody, has proven to be well tolerated (He et al. Citation2015) and demonstrated some response in patients with acute myeloid leukemia (Montesinos et al. Citation2021).
In summary, this report provides the first direct evidence that IL-3, rather than the receptor components (IL-3RA + CSF2RB), is a predictor of poor prognosis for basal-like TNBC patients. Histology on patient-derived xenografts has revealed that the cancer cells themselves produce IL-3 and express IL-3 receptors for possible autocrine pro-tumorigenic feedback loop. Histology has also shown that IL-3, IL-3Rα and IL-3Rβ are expressed in the ductal cells of healthy breast tissue as well as the primary tumor and metastatic tissue. Taken together, these preliminary findings suggest that IL-3 and its receptor may be useful markers in solid tumors, particularly TNBC, and warrant further investigation into their contribution to disease pathogenesis.
Authors’ contributions
EJT and SE performed the experiments. EJT, PAG, SM and CSB performed the analysis. EL provided unique resources. DT, GF, PAG, YK-G, WVI, GJL, EL, AFL and CSB provided clinical and/or scientific expertise. EJT and CSB wrote the first draft of the manuscript. All authors contributed to manuscript revision, read and approved the submitted version.
Supplemental Material
Download PDF (542.2 KB)Disclosure statement
No potential conflict of interest was reported by the author(s).
Data availability statement
Data sharing is not applicable to this article as no new data were created or analyzed in this study.
Additional information
Funding
References
- Bulaeva, E., D. Pellacani, N. Nakamichi, C. A. Hammond, P. A. Beer, A. Lorzadeh, M. Moksa, et al. 2020. “MYC-Induced Human Acute Myeloid Leukemia Requires a Continuing IL-3/GM-CSF Costimulus.” Blood 136 (24): 2764–2773. https://doi.org/10.1182/blood.2020006374
- Cancer Genome Atlas, Network. 2012. “Comprehensive Molecular Portraits of Human Breast Tumours.” Nature 490 (7418): 61–70. https://doi.org/10.1038/nature11412
- Casasent, A. K., M. Edgerton, and N. E. Navin. 2017. “Genome Evolution in Ductal Carcinoma in Situ: invasion of the Clones.” The Journal of Pathology 241 (2): 208–218. https://doi.org/10.1002/path.4840
- Cortes, J., D. W. Cescon, H. S. Rugo, Z. Nowecki, S. A. Im, M. M. Yusof, C. Gallardo, et al. 2020. “Pembrolizumab plus Chemotherapy versus Placebo plus Chemotherapy for Previously Untreated Locally Recurrent Inoperable or Metastatic Triple-Negative Breast Cancer (KEYNOTE-355): a Randomised, Placebo-Controlled, Double-Blind, Phase 3 Clinical Trial.” Lancet 396 (10265): 1817–1828. and https://doi.org/10.1016/S0140-6736(20)32531-9
- Dentelli, P., L. Del Sorbo, A. Rosso, A. Molinar, G. Garbarino, G. Camussi, L. Pegoraro, and M. F. Brizzi. 1999. “Human IL-3 Stimulates Endothelial Cell Motility and Promotes in Vivo New Vessel Formation.” The Journal of Immunology 163 (4): 2151–2159. 10.4049/jimmunol.163.4.2151
- Dentelli, P., A. Rosso, C. Calvi, B. Ghiringhello, G. Garbarino, G. Camussi, L. Pegoraro, and M. F. Brizzi. 2004. “IL-3 Affects Endothelial Cell-Mediated Smooth Muscle Cell Recruitment by Increasing TGF Beta Activity: potential Role in Tumor Vessel Stabilization.” Oncogene 23 (9): 1681–1692. https://doi.org/10.1038/sj.onc.1207290
- Dentelli, P., A. Rosso, G. Garbarino, C. Calvi, E. Lombard, P. Di Stefano, P. Defilippi, L. Pegoraro, and M. F. Brizzi. 2005. “The Interaction between KDR and Interleukin-3 Receptor (IL-3R) Beta Common Modulates Tumor Neovascularization.” Oncogene 24 (42): 6394–6405. https://doi.org/10.1038/sj.onc.1208786
- Dentelli, P., A. Rosso, C. Olgasi, G. Camussi, and M. F. Brizzi. 2011. “IL-3 is a Novel Target to Interfere with Tumor Vasculature.” Oncogene 30 (50): 4930–4940. https://doi.org/10.1038/onc.2011.204
- Frankel, A. E., J. H. Woo, C. Ahn, N. Pemmaraju, B. C. Medeiros, H. E. Carraway, O. Frankfurt, et al. 2014. “Activity of SL-401, a Targeted Therapy Directed to Interleukin-3 Receptor, in Blastic Plasmacytoid Dendritic Cell Neoplasm Patients.” Blood 124 (3): 385–392. https://doi.org/10.1182/blood-2014-04-566737
- He, S. Z., S. Busfield, D. S. Ritchie, M. S. Hertzberg, S. Durrant, I. D. Lewis, P. Marlton, et al. 2015. “A Phase 1 Study of the Safety, Pharmacokinetics and anti-Leukemic Activity of the anti-CD123 Monoclonal Antibody CSL360 in Relapsed, Refractory or High-Risk Acute Myeloid Leukemia.” Leukemia & Lymphoma 56 (5): 1406–1415. https://doi.org/10.3109/10428194.2014.956316
- Hercus, T. R., W. L. T. Kan, S. E. Broughton, D. Tvorogov, H. S. Ramshaw, J. J. Sandow, T. L. Nero, et al. 2017. “Role of the Beta Common (Betac) Family of Cytokines in Health and Disease.” Cold Spring Harbor Perspectives in Biology 10 (6): 1–26. https://doi.org/10.1101/cshperspect.a028514
- Jin, L., E. M. Lee, H. S. Ramshaw, S. J. Busfield, A. G. Peoppl, L. Wilkinson, M. A. Guthridge, et al. 2009. “Monoclonal Antibody-Mediated Targeting of CD123, IL-3 Receptor Alpha Chain, Eliminates Human Acute Myeloid Leukemic Stem Cells.” Cell Stem Cell 5 (1): 31–42. https://doi.org/10.1016/j.stem.2009.04.018
- Johnson, S. F., C. Cruz, A. K. Greifenberg, S. Dust, D. G. Stover, D. Chi, B. Primack, et al. 2016. “CDK12 Inhibition Reverses De Novo and Acquired PARP Inhibitor Resistance in BRCA Wild-Type and Mutated Models of Triple-Negative Breast Cancer.” Cell Reports 17 (9): 2367–2381. https://doi.org/10.1016/j.celrep.2016.10.077
- Jordan, C. T., D. Upchurch, S. J. Szilvassy, M. L. Guzman, D. S. Howard, A. L. Pettigrew, T. Meyerrose, et al. 2000. “The Interleukin-3 Receptor Alpha Chain is a Unique Marker for Human Acute Myelogenous Leukemia Stem Cells.” Leukemia 14 (10): 1777–1784. https://doi.org/10.1038/sj.leu.2401903
- Koni, M., I. Castellano, E. Venturelli, A. Sarcinella, T. Lopatina, C. Grange, M. Cedrino, et al. 2022. “Interleukin-3-Receptor-Alpha in Triple-Negative Breast Cancer (TNBC): An Additional Novel Biomarker of TNBC Aggressiveness and a Therapeutic Target.” Cancers 14 (16): 3918. https://doi.org/10.3390/cancers14163918
- Korpelainen, E. I., J. R. Gamble, W. B. Smith, G. J. Goodall, S. Qiyu, J. M. Woodcock, M. Dottore, M. A. Vadas, and A. F. Lopez. 1993. “The Receptor for Interleukin 3 is Selectively Induced in Human Endothelial Cells by Tumor Necrosis Factor Alpha and Potentiates Interleukin 8 Secretion and Neutrophil Transmigration.” Proceedings of the National Academy of Sciences of the United States of America 90 (23): 11137–11141. https://doi.org/10.1073/pnas.90.23.11137
- Korpelainen, E. I., J. R. Gamble, M. A. Vadas, and A. F. Lopez. 1996. “IL-3 Receptor Expression, Regulation and Function in Cells of the Vasculature.” Immunology and Cell Biology 74 (1): 1–7. https://doi.org/10.1038/icb.1996.1
- Laszlo, G. S., J. J. Orozco, A. R. Kehret, M. C. Lunn, J. Huo, D. K. Hamlin, D. Scott Wilbur, et al. 2022. “Development of [(211)at]Astatine-Based anti-CD123 Radioimmunotherapy for Acute Leukemias and Other CD123+ Malignancies.” Leukemia 36 (6): 1485–1491. https://doi.org/10.1038/s41375-022-01580-7
- Lehmann, B. D., J. A. Bauer, X. Chen, M. E. Sanders, A. B. Chakravarthy, Y. Shyr, and J. A. Pietenpol. 2011. “Identification of Human Triple-Negative Breast Cancer Subtypes and Preclinical Models for Selection of Targeted Therapies.” The Journal of Clinical Investigation 121 (7): 2750–2767. https://doi.org/10.1172/JCI45014
- Liedtke, Cornelia, Chafika Mazouni, Kenneth R. Hess, Fabrice André, Attila Tordai, Jaime A. Mejia, W. Fraser Symmans, et al. 2008. “Response to Neoadjuvant Therapy and Long-Term Survival in Patients with Triple-Negative Breast Cancer.” Journal of Clinical Oncology: official Journal of the American Society of Clinical Oncology 26 (8): 1275–1281. https://doi.org/10.1200/JCO.2007.14.4147
- Lombardo, G., M. Gili, C. Grange, C. Cavallari, P. Dentelli, G. Togliatto, D. Taverna, G. Camussi, and M. F. Brizzi. 2018. “IL-3R-Alpha Blockade Inhibits Tumor Endothelial Cell-Derived Extracellular Vesicle (EV)-Mediated Vessel Formation by Targeting the Beta-Catenin Pathway.” Oncogene 37 (9): 1175–1191. https://doi.org/10.1038/s41388-017-0034-x
- Lopatina, T., C. Grange, C. Cavallari, V. Navarro-Tableros, G. Lombardo, A. Rosso, M. Cedrino, et al. 2020. “Targeting IL-3Ralpha on Tumor-Derived Endothelial Cells Blunts Metastatic Spread of Triple-Negative Breast Cancer via Extracellular Vesicle Reprogramming.” Oncogenesis 9 (10): 90. https://doi.org/10.1038/s41389-020-00274-y
- Lopatina, Tatiana, Malvina Koni, Cristina Grange, Massimo Cedrino, Saveria Femminò, Giusy Lombardo, Enrica Favaro, and Maria Felice Brizzi. 2022. “IL-3 Signalling in the Tumour Microenvironment Shapes the Immune Response via Tumour Endothelial Cell-Derived Extracellular Vesicles.” Pharmacological Research 179: 106206. https://doi.org/10.1016/j.phrs.2022.106206
- Madden, S. F., C. Clarke, P. Gaule, S. T. Aherne, N. O”Donovan, M. Clynes, J. Crown, and W. M. Gallagher. 2013. “BreastMark: An Integrated Approach to Mining Publicly Available Transcriptomic Datasets Relating to Breast Cancer Outcome.” Breast Cancer Research: BCR 15 (4): R52. https://doi.org/10.1186/bcr3444
- Mahara, S., P. L. Lee, M. Feng, V. Tergaonkar, W. J. Chng, and Q. Yu. 2016. “HIFI-Alpha Activation Underlies a Functional Switch in the Paradoxical Role of Ezh2/PRC2 in Breast Cancer.” Proceedings of the National Academy of Sciences of the United States of America 113 (26): E3735–44. https://doi.org/10.1073/pnas.1602079113
- Manz, M. G. 2007. “Human-Hemato-Lymphoid-System Mice: Opportunities and Challenges.” Immunity 26 (5): 537–541. https://doi.org/10.1016/j.immuni.2007.05.001
- Moldenhauer, L. M., M. P. Cockshell, L. Frost, K. A. Parham, D. Tvorogov, L. Y. Tan, L. M. Ebert, et al. 2015. “Interleukin-3 Greatly Expands Non-Adherent Endothelial Forming Cells with Pro-Angiogenic Properties.” Stem Cell Research 14 (3): 380–395. https://doi.org/10.1016/j.scr.2015.04.002
- Montesinos, P., G. J. Roboz, C. E. Bulabois, M. Subklewe, U. Platzbecker, Y. Ofran, C. Papayannidis, et al. 2021. “Safety and Efficacy of Talacotuzumab plus Decitabine or Decitabine Alone in Patients with Acute Myeloid Leukemia Not Eligible for Chemotherapy: results from a Multicenter, Randomized, Phase 2/3 Study.” Leukemia 35 (1): 62–74. https://doi.org/10.1038/s41375-020-0773-5
- Morigi, C. 2017. “Highlights from the 15th St Gallen International Breast Cancer Conference 15–18 March, 2017, Vienna: tailored Treatments for Patients with Early Breast Cancer.” Ecancermedicalscience 11: 732. https://doi.org/10.3332/ecancer.2017.732
- Pasha, N., and N. C. Turner. 2021. “Understanding and Overcoming Tumor Heterogeneity in Metastatic Breast Cancer Treatment.” Nature Cancer 2 (7): 680–692. https://doi.org/10.1038/s43018-021-00229-1
- Pemmaraju, N., A. A. Lane, K. L. Sweet, A. S. Stein, S. Vasu, W. Blum, D. A. Rizzieri, et al. 2019. “Tagraxofusp in Blastic Plasmacytoid Dendritic-Cell Neoplasm.” The New England Journal of Medicine 380 (17): 1628–1637. https://doi.org/10.1056/NEJMoa1815105
- Perou, C. M., T. Sørlie, M. B. Eisen, M. van de Rijn, S. S. Jeffrey, C. A. Rees, J. R. Pollack, et al. 2000. “Molecular Portraits of Human Breast Tumours.” Nature 406 (6797): 747–752. https://doi.org/10.1038/35021093
- Savas, P., B. Virassamy, C. Ye, A. Salim, C. P. Mintoff, F. Caramia, R. Salgado,., et al. 2018. “Single-Cell Profiling of Breast Cancer T Cells Reveals a Tissue-Resident Memory Subset Associated with Improved Prognosis.” Nature Medicine 24 (7): 986–993. https://doi.org/10.1038/s41591-018-0078-7
- Schmid, Peter, Sylvia Adams, Hope S. Rugo, Andreas Schneeweiss, Carlos H. Barrios, Hiroji Iwata, Véronique Diéras, et al. 2018. “Atezolizumab and Nab-Paclitaxel in Advanced Triple-Negative Breast Cancer.” The New England Journal of Medicine 379 (22): 2108–2121. https://doi.org/10.1056/NEJMoa1809615
- Sharma, M., M. Das, E. Stephen-Victor, C. Galeotti, A. Karnam, M. S. Maddur, P. Bruneval, S. V. Kaveri, and J. Bayry. 2018. “Regulatory T Cells Induce Activation Rather than Suppression of Human Basophils.” Science Immunology 3 (23): 1–14. https://doi.org/10.1126/sciimmunol.aan0829
- Sørlie, T., C. M. Perou, R. Tibshirani, T. Aas, S. Geisler, H. Johnsen, T. Hastie, et al. 2001. “Gene Expression Patterns of Breast Carcinomas Distinguish Tumor Subclasses with Clinical Implications.” Proceedings of the National Academy of Sciences of the United States of America 98 (19): 10869–10874. https://doi.org/10.1073/pnas.191367098
- Sung, H., J. Ferlay, R. L. Siegel, M. Laversanne, I. Soerjomataram, A. Jemal, and F. Bray. 2021. “Global Cancer Statistics 2020: GLOBOCAN Estimates of Incidence and Mortality Worldwide for 36 Cancers in 185 Countries.” CA: a Cancer Journal for Clinicians 71 (3): 209–249. https://doi.org/10.3322/caac.21660
- Syed, Y. Y. 2019. “Tagraxofusp: First Global Approval.” Drugs 79 (5): 579–583. https://doi.org/10.1007/s40265-019-01087-z
- Tan, L. Y., M. P. Cockshell, E. Moore, K. K. Myo Min, M. Ortiz, M. Z. Johan, B. Ebert, et al. 2022. “Vasculogenic Mimicry Structures in Melanoma Support the Recruitment of Monocytes.” Oncoimmunology 11 (1): 2043673. https://doi.org/10.1080/2162402X.2022.2043673
- Tan, L. Y., C. Mintoff, M. Z. Johan, B. W. Ebert, C. Fedele, Y. F. Zhang, P. Szeto, et al. 2016. “Desmoglein 2 Promotes Vasculogenic Mimicry in Melanoma and is Associated with Poor Clinical Outcome.” Oncotarget 7 (29): 46492–46508. https://doi.org/10.18632/oncotarget.10216
- Testa, U., R. Riccioni, S. Militi, E. Coccia, E. Stellacci, P. Samoggia, R. Latagliata, et al. 2002. “Elevated Expression of IL-3Ralpha in Acute Myelogenous Leukemia is Associated with Enhanced Blast Proliferation, Increased Cellularity, and Poor Prognosis.” Blood 100 (8): 2980–2988. https://doi.org/10.1182/blood-2002-03-0852
- Varricchi, G., R. Poto, G. Marone, and J. T. Schroeder. 2021. “IL-3 in the Development and Function of Basophils.” Seminars in Immunology 54: 101510. https://doi.org/10.1016/j.smim.2021.101510
- Vellenga, E., D. Ostapovicz, B. O”Rourke, and J. D. Griffin. 1987. “Effects of Recombinant IL-3, GM-CSF, and G-CSF on Proliferation of Leukemic Clonogenic Cells in Short-Term and Long-Term Cultures.” Leukemia 1 (8): 584–589.
- Warso, M. A., A. J. Maniotis, X. Chen, D. Majumdar, M. K. Patel, A. Shilkaitis, T. K. Gupta, and R. Folberg. 2001. “Prognostic Significance of Periodic acid-Schiff-Positive Patterns in Primary Cutaneous Melanoma.” Clin Cancer Res 7 (3): 473–477.
- Yu, D., Y. Zhong, X. Li, Y. Li, X. Li, J. Cao, H. Fan, et al. 2015. “ILs-3, 6 and 11 Increase, but ILs-10 and 24 Decrease Stemness of Human Prostate Cancer Cells in Vitro.” Oncotarget 6 (40): 42687–42703. https://doi.org/10.18632/oncotarget.5883