Abstract
Certain MADS-box transcription factors play central roles in regulating fruit ripening. RIPENING INHIBITOR (RIN), a tomato MADS-domain protein, acts as a global regulator of ripening, affecting the climacteric rise of ethylene, pigmentation changes, and fruit softening. Previously, we showed that two MADS-domain proteins, the FRUITFULL homologs FUL1 and FUL2, form complexes with RIN. Here, we characterized the FUL1/FUL2 loss-of-function phenotype in co-suppressed plants. The transgenic plants produced ripening-defective fruits accumulating little or no lycopene. Unlike a previous study on FUL1/FUL2 suppressed tomatoes, our transgenic fruits showed very low levels of ethylene production, and this was associated with suppression of the genes for 1-aminocyclopropane-1-carboxylic acid synthase, a rate-limiting enzyme in ethylene synthesis. FUL1/FUL2 suppression also caused the fruit to soften in a manner independent of ripening, possibly due to reduced cuticle thickness in the peel of the suppressed tomatoes.
Graphical Abstract
The tomato FRUITFULL homologs (FULs) interact with RIPENING INHIBITOR (RIN) and FULs knockdown inhibits fruit ripening similarly to the rin mutation.
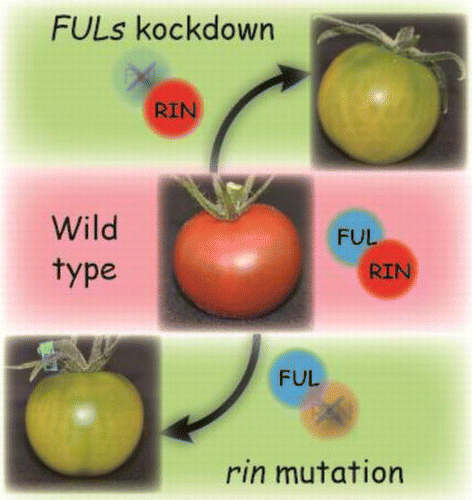
Fruit ripening is a developmental stage unique to plants that bear fleshy fruits. In ripening, the fruits become colorful, aromatic, and rich in flavor and nutrition; these characteristics attract animals that serve as seed carriers. Fleshy fruits also include many of the most important foods in the human diet, supplying water, vitamins, sugars, and antioxidants. Because fruit quality drastically changes during ripening, a better understanding of the ripening regulatory mechanism is crucial to the improvement of fruit quality.
To understand the biological mechanisms of fruit ripening, tomato (Solanum lycopersicum) has been studied most extensively, in view of the many biological advantages of this model system.Citation1) In particular, characterization of diverse ripening mutants, such as ripening inhibitor (rin), Colorless non-ripening (Cnr), and non-ripening (nor), have made large contributions to advancing the understanding of ripening.Citation2–4) Of these mutants, rin has been examined in numerous studies spanning about half a century, because it completely inhibits all ripening-associated physiological changes, including red pigmentation, softening, volatile production, and even the climacteric rise of respiration and ethylene production.Citation5) Exogenous ethylene does not induce ripening of rin mutant fruits; therefore, RIN acts upstream of ethylene signaling. RIN encodes a SEPALLATA (SEP)-class MADS-box transcription factor (TF),Citation2) and the rin mutation impairs RIN transcriptional activation.Citation6) To elucidate the RIN-dependent mechanisms regulating fruit ripening, we (and others) have identified direct RIN target genes by chromatin immunoprecipitation (ChIP) assay, and this revealed that RIN directly regulates genes involved in a broad range of metabolic pathways, including ethylene production and carotenoid synthesis.Citation7–11)
In plants, MADS-box TFs play important roles in almost every developmental process, especially in reproductive development, including floral transition, organ identity specification, fruit development, and gametogenesis.Citation12) In flower development, MADS-box TFs specify floral organs via the quartet model, a refinement of the classic ABC model.Citation13) In the quartet model, floral organs are specified by tetramers composed of organ-specific combinations of four classes of MADS-domain proteins: A-, B-, C-, and E-classes. SEPs, which belong to the E-class, are required for flower development, and SEP3 plays a pivotal role in tetramers by forming complexes with other MADS-domain proteins.Citation14,15)
Because RIN is a SEP homolog, it is expected that other tomato MADS domain proteins might function in the regulation of ripening by forming MADS-box TF complexes with RIN. Thus, to find novel ripening regulators, tomato MADS-box genes have been investigated extensively. These efforts identified TAGL1, a MADS-box gene encoding a SHATTERPROOF (SHP) homolog, as a regulator of fruit ripening through ethylene production.Citation16–18) Additionally, Bemer et al.Citation19) reported that two MADS-box genes encoding FRUITFULL homologs (FUL1 and FUL2, previously called TDR4 and SlMBP7, respectively) redundantly regulate fruit ripening. They reported that, unlike ripening regulation by RIN and TAGL1, the FUL homologs regulate only ethylene-independent ripening, because their transgenic lines, containing an RNAi construct designed to suppress FUL1 and FUL2, produced ethylene at levels equal to the wild type.
We previously screened for RIN-binding MADS-box proteins expressed in ripening tomato fruits, and we identified FUL1 and FUL2.Citation20) We found that RIN forms heterodimers with FUL1 and FUL2 and that the heterodimers can bind to a typical target DNA motif for MADS-box proteins in vitro. Moreover, in vivo analysis revealed that the FUL homologs bind to the promoters of the two genes that encode a rate-limiting enzyme for ripening-associated ethylene production, 1-aminocyclopropane-1-carboxylic acid (ACC) synthase (ACS2 and ACS4).Citation21,22) These results strongly suggest that the FUL homologs are involved in ripening-associated ethylene production, though this is inconsistent with a result described by Bemer et al.Citation19) To verify the possibility that the FUL homologs regulate ethylene-dependent ripening through transcriptional regulation of the 1-aminocyclopropane-1-carboxylic acid synthase (ACS) genes, we independently developed FUL1/FUL2 co-suppression lines. In this report, we describe the phenotype of the transgenic lines, which showed defects in ripening and produced ethylene at very low levels, indicating the involvement of FUL1/FUL2 in ripening-associated ethylene production.
Materials and methods
Plant transformation and growth
An RNA interference (RNAi) vector targeting FUL2, and probably also targeting FUL1, was constructed as follows: A FUL2 fragment with high sequence similarity to FUL1 (Supplemental Fig. 1; see Biosci. Biotechnol. Biochem. Web site) was amplified with oligonucleotide primers FUL2-RI-F2 and FUL2-RI-R2 (Supplemental Table 1). The resulting fragments were inserted into pBI-sense, antisense-GW (Inplanta Innovations, Kanagawa, Japan) via the Gateway system with the pENTR/D-TOPO entry vector (Invitrogen, Carlsbad, CA). The resulting plasmid was transformed into tomato cv. Ailsa Craig by the Agrobacterium tumefaciens-mediated method, as described previously.Citation23) The transgenic plants were grown in a growth room under a 16 h light at 25 °C and 8 h dark at 20 °C cycle. To induce fruit setting for sterile lines of the transgenic plants, Tomato tone (including 0.15% 4-chlorophenoxy acetic acid, Ishihara Biosciences, Tokyo, Japan) was diluted 100-fold and sprayed on anthesis-stage flowers.
Characterization of fruit phenotypes
Ethylene production from the fruits was measured with a gas chromatograph (Model GC8A, Shimadzu, Kyoto, Japan), as described previously.Citation24) Briefly, the fruit was enclosed in a 1 L chamber for 2 h, and then 1 mL of gas was withdrawn and injected into the gas chromatograph. The lycopene content of the fruits was measured by a method described previously.Citation25) Briefly, 2 g of fruit flesh was mixed with 20 mL of acetone:hexane (4:6), and the absorbance values of the supernatant were determined by a SmartSpec 3000 Spectrophotometer (Bio-Rad, Hercules, CA). The lycopene content was determined by the following calculation: lycopene content = −0.0458A663 + 0.204A645 + 0.372 A505 − 0.0806 A453. Fruit firmness was measured with a Firm Tester model SA-1 (Toyo Seiki, Tokyo, Japan).Citation26) For cuticular lipid staining, hand-sliced fruit tissues were stained with 0.1% (w/v) Sudan Red 7B for 1 h and then rinsed with water several times.Citation27)
Quantitative reverse transcription-PCR (qRT-PCR)
Total RNA was extracted from the fruits with an RNeasy Plus Kit (Qiagen, Valencia, CA). Total RNA was reverse transcribed into cDNA with a PrimeScript II 1st strand cDNA Synthesis Kit (Takara, Otsu, Japan). Real-time PCR amplification was carried out with a THUNDERBIRD SYBR qPCR Mix (Toyobo, Osaka, Japan) for all genes except FUL2 with a 7300 Real-Time PCR System (Applied Biosystems, Carlsbad, CA). For FUL2, qRT-PCR analysis was carried out with a primer pair for the full length of the coding region (744 bp) to avoid amplification of short cDNAs derived from partial FUL2 mRNAs cleaved by an RNAi-mediated process. KOD SYBR qPCR Mix (Toyobo), which can analyze amplification rates for long PCR fragments (≤ 2 kb), was used for FUL2 expression analysis. Relative quantification of the expression of each gene was performed by the 2−ΔΔCT method.Citation28) A gene for clathrin adaptor complexes medium subunit (CAC; SGN-U314153) was used as reference.Citation29) The primer sequences are listed in Supplemental Table 1.
Results
Suppression of FUL1 and FUL2 inhibited tomato fruit ripening
Based on the high sequence similarity between FUL1 and FUL2 (Supplemental Fig. 1) and the results of our previous study,Citation20) we hypothesized that FUL1 and FUL2 act redundantly in the regulation of fruit ripening. Therefore, we decided to suppress FUL1 and FUL2 simultaneously, and constructed an RNAi vector using a FUL2 fragment that shows 78% identity to the corresponding sequence of FUL1. The RNAi vector was transformed into the Ailsa Craig (AC) tomato cultivar. We obtained five independent transgenic lines, which bore fruits that failed to ripen normally; at the age when the AC fruits turned red, the transgenic fruits remained yellow (Fig. (A)). Most of the fruits from these five transgenic lines never turned red, even after storage for several months, similarly to the rin mutant fruits, but sectored red pigmentation was occasionally observed in some fruits after long storage (Supplemental Fig. 2(A)). Of the five RNAi lines, two set fruits and generated seeds by self-pollination, but the fruit set rate was lower than that of the wild type. Moreover, the fruits of the other three lines failed to initiate expansion after pollination, but the ovaries/small fruits did not abscise from the plant (Supplemental Fig. 2(B)). This failure was remedied by the application of a fruit-setting reagent that contains a synthetic auxin, 4-chlorophenoxy-acetic acid (4-CPA), but the developed fruits contained no seeds. An increase in ploidy, which happens occasionally during transformant development, can cause reduced fertility, but the ploidy of the five transformants was equivalent to AC (diploid) (Supplemental Fig. 3). We further analyzed two self-fertile lines (TF18 and TF68) and one sterile line (TF2).
Fig. 1. Suppression of FUL1 and FUL2 Inhibited Tomato Fruit Ripening.
Note: (A) FUL1/FUL2 suppressed tomatoes at the age corresponding to the red coloring stage of AC fruits. Scale bars indicate 10 mm. (B) Expression of FUL1, FUL2, and RIN in the suppressed tomatoes. Three suppression lines (TF2, TF18, and TF68) were analyzed, along with a rin mutant and the wild-type (Ailsa Craig, AC), by quantitative RT-PCR (qRT-PCR). Levels of transcripts are shown as fold-change values as compared to the expression in AC at 45 DAP. Error bars indicate standard deviations (SD) from the experiments for three independent fruits.
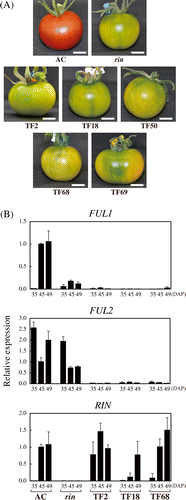
We confirmed the reduction of FUL1/FUL2 expression in the transgenic lines by quantitative RT-PCR (Fig. (B)). FUL2 expression in ripening-defective fruits from the three lines was less than 3% of that in AC at the pre-ripening stage, 35 d after pollination (DAP), and less than 7% at 45 DAP, when the AC fruits had already started ripening and expressing RIN (Fig. (B)). We also found severe inhibition of FUL1, as FUL1 expression in the transgenic lines was about 3% or less of that in AC during ripening (45 DAP; Fig. (B)). RIN was expressed in the FUL1/FUL2 suppressed lines at levels comparable to AC, but the onset of RIN induction varied among the suppressed lines. As compared to AC, RIN expression was detected earlier in TF2, later in TF18, and at the same age in TF68 (Fig. (B)).
Ethylene production was reduced in the FUL1/FUL2 suppressed lines
Next, we examined ripening-associated ethylene production in fruits of the three FUL1/FUL2 suppressed lines (TF2, TF18, and TF68). The AC fruits harvested at 40 DAP started ethylene production within 5 d, and ethylene increased rapidly until it reached a peak 1 week after the first sign of ethylene production (Fig. (A)). When we analyzed the FUL1/FUL2 suppressed fruits, we found that, compared to the AC fruits, the three RNAi lines produced ethylene at very low levels throughout the examination period, similarly to the rin mutant fruits (Fig. (A)). We analyzed the expression of the two ACC synthase (ACS) genes, ACS2 and ACS4, which are responsible for ethylene production during ripening. Consistent with ethylene production, the suppressed fruits showed very low levels of ACS2 and ACS4 expression (Fig. (B)) suggesting that the defect in ethylene production in the FUL1/FUL2 suppressed lines was caused by the failure to upregulate the ACS genes.
Fig. 2. Inhibition of Ethylene Synthesis by Suppression of FUL1/FUL2.
Note: (A) Ethylene production of FUL1/FUL2 suppressed tomatoes. Fruits of AC, the rin mutant, and three transgenic lines (TF2, TF18, and TF68) were harvested at 40 DAP, and ethylene levels were measured everyday. (B) Expression of ACS2 and ACS4 in the suppressed tomatoes. Expression analyses by qRT-PCR were conducted as described in Fig. .
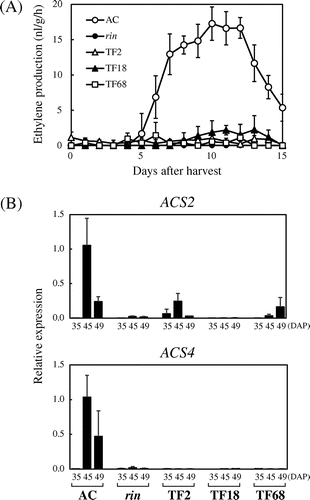
Lycopene production was reduced in the FUL1/FUL2 suppressed lines
Lycopene accumulation was measured for fully ripened fruits of the wild-type and corresponding aged fruits from the FUL1/FUL2 suppressed plants. The suppressed fruits showed little or no lycopene accumulation as compared to the AC fruits, as also observed for the rin mutant fruits (Fig. (A)). Consistent with this, the suppressed fruits showed strong repression of a gene encoding phytoene synthase (PSY1), which catalyzes a rate-limiting step of carotenoid biosynthesis (Fig. (B)).
Fig. 3. Inhibition of Lycopene Production by Suppression of FUL1/FUL2.
Note: (A) Lycopene contents in the transgenic tomatoes. Lycopene was measured for the same fruits used to measure ethylene production (15 d after harvest). ND, not detected. (B) Expression of PSY1 in the suppressed tomatoes. Expression analyses by qRT-PCR were conducted as described in Fig. .
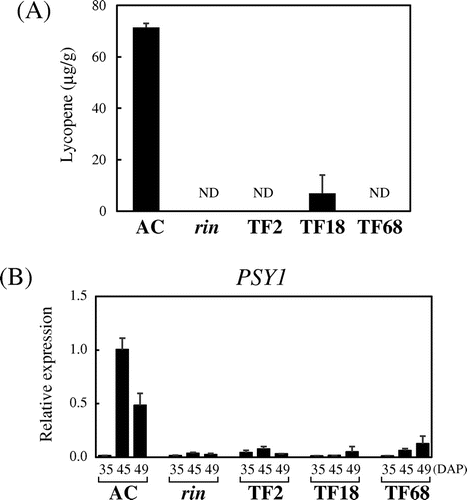
Fruit softening occurred in the FUL1/FUL2 suppressed lines
As described above, depressed ethylene production and lycopene synthesis in the FUL1/FUL2 suppressed fruits resembled the rin mutant phenotype. In contrast, the fruit firmness of the FUL1/FUL2 suppressed lines decreased at a rate comparable to the AC fruits, and unlike the rin mutant fruits (Fig. (A)). This decreased fruit firmness appeared to be inconsistent with the observation that the genes for cell-wall modifying enzymes polygalacturonase (PG2A) and endo-β-1,4-glucanase (Cel2) were not upregulated in the FUL1/FUL2 suppressed fruits (Fig. (B)). These phenotypes in the FUL1/FUL2 suppressed fruits, a failure of ripening but reduced firmness, were similar to TAGL1 suppressed lines.Citation16–18) Because TAGL1 suppression reduced the accumulation of cuticle on the peel,Citation16,18) we analyzed cuticle accumulation in the FUL1/FUL2 suppressed fruits. We found that the FUL1/FUL2 suppressed fruits had a thinner layer of cuticle than the rin mutant fruits (Fig. (C)). Indeed, expression of the genes for the enzymes involved in the cuticle biosynthesis pathway, such as GDSL family esterase/lipase (GDSL1) and glycerol-3-phosphate acyltransferase 6 (SlGPAT6), was significantly suppressed in the transgenic fruits at the pre-ripening stage (35 DAP; Fig. (D)). These two genes were expressed at very low levels in the 45 DAP fruits of AC and rin mutants, but their expression increased several-fold in the TF18 fruits. However, the increased expression level in TF18 was significantly lower than the level at the pre-ripening stage of AC and the rin mutants. Expression of TAGL1 at the pre-ripening stage was equivalent as between AC, the rin mutants, and TF18, but the expression in TF18 at 45 DPA was about half of that in AC (Fig. (D)).
Fig. 4. Reduced Fruit Firmness by FUL1/FUL2 Suppression.
Note: (A) Measurement of fruit firmness of the FUL1/FUL2 suppression lines. Breaker stage fruits, which started coloring (red for AC and yellow for the transgenic lines and the rin mutants), were harvested and firmness was measured everyday (see “Materials and methods”). (B) Expression of PG2A and Cel2 in the fruits of the suppression lines. Expression analyses by qRT-PCR were conducted as described in Fig. . Error bars indicate SD for experiments on three independent fruits in all assays, except for the assay of Cel2 expression in AC at 45-DAP, which used duplicate samples. (C) Cuticle in the peel of the rin mutant and the TF18 transgenic fruits. Cuticular lipids in the fruit tissues were stained with Sudan Red 7B. Scale bars indicate 50 μm. (D) Expression of SlGPAT6, GDSL1, and TAGL1 in the fruits of the FUL1/FUL2 suppression lines. Expression in TF18 at 35 DAP and 45 DAP was analyzed in the rin mutant and the wild-type by qRT-PCR. Levels of transcripts are shown as fold-change values as compared to expression in AC at 45 DAP. Error bars indicate SD for experiments on three independent fruits.
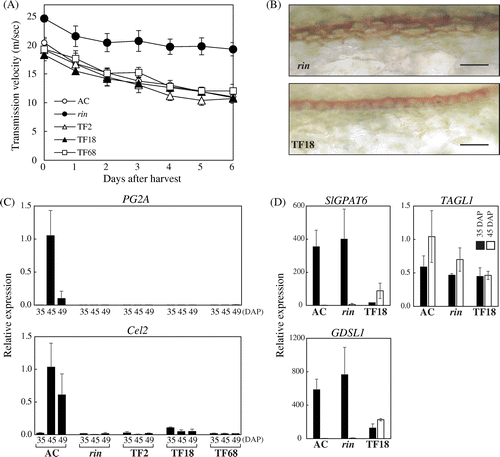
Discussion
FUL1 and FUL2 regulate tomato fruit ripening via upregulation of ethylene synthesis
This study clearly demonstrated that suppression of the tomato FUL homologs inhibits fruit ripening phenomena, including ripening-associated ethylene production. Based on the following evidence, we have hypothesized that the FUL homologs regulate ethylene-inducible ripening processes: (i) RIN is a dominant regulator of ripening-associated ethylene production,Citation5) and FUL1 and FUL2 form complexes with RIN,Citation10,20) (ii) ChIP assays have indicated that FUL1 and FUL2 bind to the ACS gene promoters that are target sites of RIN.Citation6,7) The results for FUL1/FUL2 suppression found in the present study are fully consistent with our previous results, strongly suggesting that FUL1 and FUL2 regulate fruit ripening via positive regulation of ripening-associated ethylene production. Bemer et al.Citation19) have conducted a co-suppression analysis of FUL1 and FUL2 in tomato, and they found that the homologs are redundantly involved in the regulation of fruit ripening, as in our study. However, substantial differences exist between the results of the two studies. The most crucial has to do with the regulation of ethylene production by FUL1 and FUL2. A previous study concluded that FUL1 and FUL2 do not affect ethylene production during ripening.Citation19) This discrepancy might have resulted from stronger suppression of FUL1 and FUL2 in our study (expression in the transgenic lines to less than 3 and 7% of wild-type levels, respectively) in comparison with the previous study (to more than 5 and 12% of wild-type levels, respectively). The different levels of suppression might have been due to the different DNA sequences used for RNAi. We used a FUL2 fragment for RNAi, while the previous study used a FUL1 fragment. The line of evidence provided by our previous and current studies confirms the positive regulatory role of FUL1/FUL2 in ethylene production. Therefore, we conclude that FUL1 and FUL2 are involved in the regulation of ethylene production associated with ripening.
Reduction of fruit firmness is caused by different mechanisms in the wild-type and the FUL1/FUL2 suppressed fruits
The FUL1/FUL2 suppressed fruits showed defects in ripening phenomena, including ethylene production and lycopene synthesis, while the fruits softened similarly to wild-type, unlike the rin mutant fruits, which never soften. The reduction of firmness in the FUL1/FUL2 suppressed fruits was, however, not due to degradation of the cell wall, because, similarly to the rin mutants, the genes for cell-wall degradation, such as PG and Cel2, were not upregulated in the suppressed fruits. The thin cuticle on the peel of the FUL1/FUL2 suppressed fruit suggests a possible explanation of the softening. Excess dehydration from the peel of the suppressed fruits might result in abnormal softening. A similar phenotype was found in TAGL1 silenced tomatoes, which also showed reduced firmness although ethylene production and lycopene accumulation were defective, and reduced pericarp thickness and the thinner cuticle layer were postulated as a possible explanation of the softening.Citation16,18) We observed lower expression of the genes involved in cuticle biosynthesis in the pre-ripening stage fruit of the suppressed line. FUL2 is expressed strongly at the pre-ripening stage, but FUL1 is not,Citation20) suggesting that FUL2 is responsible for cuticle biosynthesis before ripening.
Conserved function of FUL homologs in plants
FUL1/FUL2 suppression affected fruit setting, and three of the suppressed lines failed to set any normal fruits. In these plants, the ovaries, which were not abscised from the plants even after senescence of the flower, did not expand unless treated with a reagent with auxin activity. In addition, the fruits that developed after auxin treatment included no seeds. Similarly, the Arabidopsis fruitfull (ful) mutation causes severe defects in fruit expansion, but dissimilarly, the fruits include seeds.Citation30) This suggests that the FUL homologs have conserved roles in fruit expansion while, unlike the Arabidopsis homologs, the tomato homologs are also involved in the regulation of initial seed development. A study of a FUL homolog in the bilberry (Vaccinium myrtillus) revealed that it regulates anthocyanin biosynthesis pathways during fruit ripening,Citation31) suggesting that, in fleshy fruit bearing plants, FUL homologs have acquired an activity regulating fruit ripening.
MADS-box TF complexes regulating tomato fruit ripening
We hypothesize that RIN forms a complex of MADS-box TFs that regulates fruit ripening. Our previous study found that FUL1 and FUL2 are plausible candidates for components of the ripening regulator complex.Citation20) In this study, we demonstrated that the suppression of FUL1 and FUL2 inhibited ripening, at least partially through ethylene-dependent pathways. These results are consistent with the hypothesis that the FUL homologs and RIN compose a complex that regulates ripening. Moreover, the phenotypes of the FUL1/FUL2 suppressed lines, including defective ripening but decreased fruit firmness, were similar to TAGL1 suppressed fruits. Two possibilities might explain the similar phenotypes of the TAGL1 and FUL1/FUL2 suppressed plants: the FUL homologs and TAGL1 may act in an identical transcriptional cascade, or the FUL homologs may form a complex with TAGL1. FUL1/FUL2 suppression negatively affected TAGL1 expression during ripening (Fig. (D)), and TAGL1 suppression also partially reduced FUL1 expression,Citation18) but there are no data on the effect of TAGL1 suppression on FUL2 expression. Thus, at least partially, TAGL1 and the FUL homologs are involved in the regulation of each other’s expression. The interaction between the FUL homologs and TAGL1 has not been demonstrated, but a yeast two-hybrid screen for RIN binding proteins identified interaction between RIN and TAGL1.Citation10) Further analysis of the interactions between these MADS proteins might reveal the TF complex required for ripening regulation, and also refine the floral quartet model of MADS-domain proteins for fruit development.
Quantification of mRNAs targeted by RNAi
To investigate the effects of RNAi suppression, qPCR is used to measure mRNA levels. qPCR usually targets short DNA fragments (< 200 bp) for reliability, because, in the general PCR reaction, the amplification efficiency of a long target fragment is not high enough to quantify the level in a reliable manner. RNAi-mediated cleavage of target mRNAs eliminates intact mRNAs but leaves detectable mRNA fragments. Therefore, we measured FUL2 mRNA accumulation in the RNAi lines by qRT-PCR with a primer pair that amplifies the full-length FUL2 coding region (744 bp). We also used qPCR reagents suitable for amplification of long fragments (see “Materials and Methods”). The assay detected severely decreased levels of FUL2 mRNA. We also conducted qRT-PCR with a primer pair for a short region of FUL2 (78 bp). This assay detected a higher level of mRNA in the RNAi lines than that detected by the full-length assay (Supplemental Fig. 4(A)). To clarify which result more accurately represented the functional mRNA level, we conducted an RNA gel blotting assay, and found that the intact FUL2 RNA in an RNAi line was below the detection limit (Supplemental Fig. 4(B)), indicating that the qRT-PCR assay for the full-length coding region most likely represents the actual level of intact FUL2 transcripts. As demonstrated here, to investigate an RNAi effect, one must measure the level of intact mRNA, since measurement of a portion of the mRNA can produce false high readings.
Supplemental material
The supplemental material for this paper is available at http://dx.doi.org/10.1080/09168451.2014.878221.
Supplemental Table 1 and Figures 1 to 4
Download PDF (390.9 KB)Acknowledgments
This work was supported by Scientific Technique Research Promotion Program for Agriculture, Forestry, Fisheries, and Food Industry to Y. I.
Notes
Abbreviations: rin, ripening inhibitor; FUL, FRUITFULL; ACC, 1-aminocyclopropane-1-carboxylic acid; SEP, SEPALLATA; qRT-PCR, quantitative reverse transcription-polymerase chain reaction; RNAi, RNA interference; ChIP, chromatin immunoprecipitation.
References
- Klee HJ, Giovannoni JJ. Genetics and control of tomato fruit ripening and quality attributes. Annu. Rev. Genet. 2011;45:41–59.10.1146/annurev-genet-110410-132507
- Vrebalov J, Ruezinsky D, Padmanabhan V, White R, Medrano D, Drake R, Schuch W, Giovannoni J. A MADS-box gene necessary for fruit ripening at the tomato ripening-inhibitor (rin) locus. Science. 2002;296:343–346.10.1126/science.1068181
- Manning K, Tör M, Poole M, Hong Y, Thompson AJ, King GJ, Giovannoni JJ, Seymour GB. A naturally occurring epigenetic mutation in a gene encoding an SBP-box transcription factor inhibits tomato fruit ripening. Nat. Genet. 2006;38:948–952.10.1038/ng1841
- Giovannoni JJ. Fruit ripening mutants yield insights into ripening control. Curr. Opin. Plant Biol. 2007;10:283–289.10.1016/j.pbi.2007.04.008
- Tigchelaar EC, McGlasson WB, Buescher RW. Genetic regulation of tomato fruit ripening. HortScience. 1978;13:508–513.
- Ito Y, Kitagawa M, Ihashi N, Yabe K, Kimbara J, Yasuda J, Ito H, Inakuma T, Hiroi S, Kasumi T. DNA-binding specificity, transcriptional activation potential, and the rin mutation effect for the tomato fruit-ripening regulator RIN. Plant J. 2008;55:212–223.10.1111/tpj.2008.55.issue-2
- Fujisawa M, Nakano T, Ito Y. Identification of potential target genes for the tomato fruit-ripening regulator RIN by chromatin immunoprecipitation. BMC Plant Biol. 2011;11:26.10.1186/1471-2229-11-26
- Fujisawa M, Shima Y, Higuchi N, Nakano T, Koyama Y, Kasumi T, Ito Y. Direct targets of the tomato-ripening regulator RIN identified by transcriptome and chromatin immunoprecipitation analyses. Planta. 2012;235:1107–1122.10.1007/s00425-011-1561-2
- Fujisawa M, Nakano T, Shima Y, Ito Y. A large-scale identification of direct targets of the tomato MADS box transcription factor RIPENING INHIBITOR reveals the regulation of fruit ripening. Plant Cell. 2013;25:371–386.10.1105/tpc.112.108118
- Martel C, Vrebalov J, Tafelmeyer P, Giovannoni JJ. The tomato MADS-box transcription factor RIPENING INHIBITOR interacts with promoters involved in numerous ripening processes in a COLORLESS NONRIPENING-dependent manner. Plant Physiol. 2011;157:1568–1579.10.1104/pp.111.181107
- Qin G, Wang Y, Cao B, Wang W, Tian S. Unraveling the regulatory network of the MADS box transcription factor RIN in fruit ripening. Plant J. 2012;70:243–255.10.1111/tpj.2012.70.issue-2
- Smaczniak C, Immink RG, Angenent GC, Kaufmann K. Developmental and evolutionary diversity of plant MADS-domain factors: insights from recent studies. Development. 2012;139:3081–3098.10.1242/dev.074674
- Theissen G, Saedler H. Plant biology. Floral quartets. Nature. 2001;409:469–471.10.1038/35054172
- Honma T, Goto K. Complexes of MADS-box proteins are sufficient to convert leaves into floral organs. Nature. 2001;409:525–529.10.1038/35054083
- Smaczniak C, Immink RG, Muiño JM, Blanvillain R, Busscher M, Busscher-Lange J, Dinh QD, Liu S, Westphal AH, Boeren S, Parcy F, Xu L, Carles CC, Angenent GC, Kaufmann K. Characterization of MADS-domain transcription factor complexes in Arabidopsis flower development. Proc. Nat. Acad. Sci. USA. 2012;109:1560–1565.10.1073/pnas.1112871109
- Giménez E, Pineda B, Capel J, Antón MT, Atarés A, Pérez-Martín F., García-Sogo B, Angosto T, Moreno V, Lozano R. Functional analysis of the Arlequin mutant corroborates the essential role of the Arlequin/TAGL1 gene during reproductive development of tomato. PLoS One. 2010;5:e14427.10.1371/journal.pone.0014427
- Itkin M, Seybold H, Breitel D, Rogachev I, Meir S, Aharoni A. TOMATO AGAMOUS-LIKE 1 is a component of the fruit ripening regulatory network. Plant J. 2009;60:1081–1095.10.1111/tpj.2009.60.issue-6
- Vrebalov J, Pan IL, Arroyo AJ, McQuinn R, Chung M, Poole M, Rose J, Seymour G, Grandillo S, Giovannoni J, Irish VF. Fleshy fruit expansion and ripening are regulated by the Tomato SHATTERPROOF gene TAGL1. Plant Cell. 2009;21:3041–3062.10.1105/tpc.109.066936
- Bemer M, Karlova R, Ballester AR, Tikunov YM, Bovy AG, Wolters-Arts M, Rossetto PdeB, Angenent GC, de Maagd RA. The tomato FRUITFULL homologs TDR4/FUL1 and MBP7/FUL2 regulate ethylene-independent aspects of fruit ripening. Plant Cell. 2012;24:4437–4451.
- Shima Y, Kitagawa M, Fujisawa M, Nakano T, Kato H, Kimbara J, Kasumi T, Ito Y. Tomato FRUITFULL homologues act in fruit ripening via forming MADS-box transcription factor complexes with RIN. Plant Mol. Biol. 2013;82:427–438.10.1007/s11103-013-0071-y
- Barry CS, Llop-Tous MI, Grierson D. The regulation of 1-aminocyclopropane-1-carboxylic acid synthase gene expression during the transition from system-1 to system-2 ethylene synthesis in tomato. Plant Physiol. 2000;123:979–986.10.1104/pp.123.3.979
- Nakatsuka A, Murachi S, Okunishi H, Shiomi S, Nakano R, Kubo Y, Inaba A. Differential expression and internal feedback regulation of 1-aminocyclopropane-1-carboxylate synthase, 1-aminocyclopropane-1-carboxylate oxidase, and ethylene receptor genes in tomato fruit during development and ripening. Plant Physiol. 1998;118:1295–1305.10.1104/pp.118.4.1295
- Sun HJ, Uchii S, Watanabe S, Ezura H. A highly efficient transformation protocol for Micro-Tom, a model cultivar for tomato functional genomics. Plant Cell Physiol. 2006;47:426–431.10.1093/pcp/pci251
- Kitagawa M, Ito H, Shiina T, Nakamura N, Inakuma T, Kasumi T, Ishiguro Y, Yabe K, Ito Y. Characterization of tomato fruit ripening and analysis of gene expression in F1 hybrids of the ripening inhibitor (rin) mutant. Physiol. Plant. 2005;123:331–338.10.1111/ppl.2005.123.issue-3
- Nagata M, Yamashita I. Simple method for simultaneous determination of chlorophyll and carotenoids in tomato fruits. Nippon Shokuhin Kogyo Gakkaishi. 1992;39:925–928.10.3136/nskkk1962.39.925
- Sugiyama J, Otobe K, Hayashi S, Usui S. Firmness measurement of muskmelons by acoustic impulse transmission. Trans. ASAE. 1994;37:1235–1241.10.13031/2013.28201
- Brundrett MC, Kendrick B, Peterson CA. Efficient lipid staining in plant material with sudan red 7B or fluoral yellow 088 in polyethylene glycol-glycerol. Biotech. Histochem. 1991;66:111–116.10.3109/10520299109110562
- Livak KJ, Schmittgen TD. Analysis of relative gene expression data using real-time quantitative PCR and the 2-ΔΔCT Method. Methods. 2001;25:402–408.10.1006/meth.2001.1262
- Expósito-Rodríguez M, Borges AA, Borges-Pérez A, Pérez JA. Selection of internal control genes for quantitative real-time RT-PCR studies during tomato development process. BMC Plant Biol. 2008;8:131.10.1186/1471-2229-8-131
- Gu Q, Ferrándiz C, Yanofsky MF, Martienssen R. The FRUITFULL MADS-box gene mediates cell differentiation during Arabidopsis fruit development. Development. 1998;125:1509–1517.
- Jaakola L, Poole M, Jones MO, Kämäräinen-Karppinen T, Koskimäki JJ, Hohtola A, Häggman H, Fraser PD, Manning K, King GJ, Thomson H, Seymour GB. A SQUAMOSA MADS box gene involved in the regulation of anthocyanin accumulation in bilberry fruits. Plant Physiol. 2010;153:1619–1629.10.1104/pp.110.158279