ABSTRACT
The objective of present investigation was to appraise the effects of piperine on STZ-induced diabetic cardiomyopathy in rats. Diabetes was induced in Sprague-Dawley rats with intraperitoneal STZ injection, and the rats were assigned to seven groups. Electrocardiograph, hemodynamic, various biochemical, molecular, and histological parameters were examined. Treatment with piperine significantly (p < 0.05) restored altered myocardial functions, inhibited cardiac marker, and restored electrocardiogram and hemodynamic alterations. The elevated level of cardiac oxido-nitrosative stress and decreased cardiac Na-K-ATPase concentration, after STZ administration, were significantly (p < 0.05) attenuated by piperine treatment. Piperine also considerably (p < 0.05) increased myocardial mitochondrial enzyme activity. STZ-induced alteration in heart ANP, BNP, cTn-I, Bcl2, Bax/Bcl2, and caspase3 mRNA expression was significantly (p < 0.05) restored by piperine treatment. Piperine administration reduced histopathological aberrations induced by STZ. In conclusion, the present investigation suggests that piperine ameliorates STZ-induced diabetic cardiomyopathy via modulation of caspase-3, Bcl2, Bax/Bcl2 pathways.
Abbreviations: ACE: Angiotensin-Converting Enzyme; ANOVA: Analysis of Variance; ANP: Atrial Natriuretic Peptide; APAF: Apoptotic Protease-Activating Factor; ARB: Angiotensin Receptor Blockers; ATP: Adenosine Triphosphate; Bax: Bcl-2-associated X protein; Bcl2: B-cell lymphoma 2; BPM: Beats Per Minute; BNP: brain natriuretic peptide; CAD: Caspase-3-Activated DNase; cDNA: Complementary DNA; CK-MB: Creatine Kinase-MB; CPCSEA: Committee for the Purpose of Control And Supervision of Experiments on Animals; cTn-I: cardiac troponin I; DBP: Diastolic Blood Pressure; DCM: Diabetic Cardiomyopathy; DNA: Deoxyribonucleic Acid; DPX: DisterenePhthalate Xylene; ECG: Electrocardiogram; ETC: Electron Transport Chain; GOD-POD: Glucose Oxidase Peroxidase; GSH: Glutathione; IAEC: Institutional Animal Ethics Committee; IL-6: Interleukin-6; IL-1b: Interleukin-1b; LDH: Lactate Dehydrogenase; LV: Left Ventricle; LVEDP: left ventricular end-diastolic Pressure; MABP: Mean Arterial Blood Pressure; MDA: Malondialdehyde; mRNA: Messenger Ribonucleic Acid; MTT: 3- (4,5-Dimethylthiazol-2-yl)-2,5-DiphenyltetrazoliumBromide; NADH: Nicotinamide Adenine Dinucleotide Phosphate; NADPH: Nicotinamide Adenine Dinucleotide Phosphate Hydrogen; NO: nitric oxide; NP: Natriuretic Peptides; OXPHOS: Oxidative Phosphorylation; p.o.: per os; PCR: Polymerase Chain Reaction; RT-PCR: Reverse Transcriptionpolymerase Chain Reaction; PPAR: Peroxisome Proliferator-Activated Receptor Gamma; RAS: Renin-Angiotensin System; RNA: Ribonucleic Acid; ROS: Reactive Oxygen Species; SBP: Systolic Blood Pressure; SDH: Succinate Dehydrogenase; SEM: Standard Error Means; SOD: superoxide dismutase: STZ: Streptozotocin; TNF: Tumor Necrosis Factor Alpha; TnI: Troponin I
Graphical Abstract
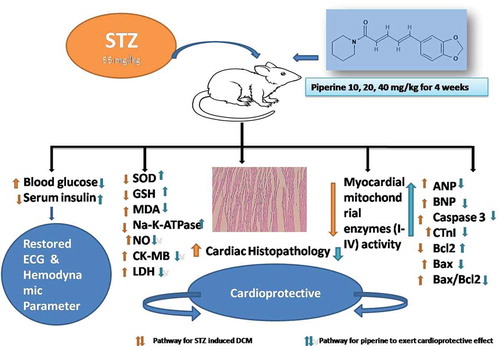
Piperine Ameliorates Streptozotocin-Induced Diabetic Cardiomyopathy in Rats.
Diabetes is gradually becoming a severe disease condition globally. A recent estimation is that about 535 million people will be affected by diabetes in the next 15 years [Citation1]. Due to the hyperglycemic condition heat problem will excessively increase in coronary artery disease and hypertension condition [Citation2]. Diabetes is associated with structural and functional changes of the left ventricular myocardium, which is termed as Diabetic cardiomyopathy (DCM) [Citation3,Citation4].DCM occurs due to diastolic and systolic dysfunction of the left ventricle in diabetic patients (Type I and Type II) [Citation5]. Predominant contributors for progression of DCM are ventricular hyperplasia, metabolic disorder, extracellular matrix abnormalities, coronary microvascular disease, oxidative stress, and cell necrosis [Citation6]. Studies have reported that diabetes causes the generation of free radicals, which assist in cardiac disorder and cell autophagy [Citation7,Citation8]. Researchers have also noted that excessive quantities of reactive oxygen species (ROS) were produced in the heart muscle due to hyperglycemia, resulting in apoptosis in a diabetic heart [Citation9,Citation10]. It has been observed that oxidative stress activated various apoptotic signaling pathways such as caspase-3 activation pathways. Besides, insulin resistance and hyperlipidemia are both causes of apoptosis and oxidative stress [Citation9,Citation11]. Pro-inflammatory cytokines (tumor necrosis factor-alpha (TNF-α), interleukin-6 (IL-6), and interleukin-1β (IL-1β)) have a significant role in insulin resistance and inflammatory cascade involved in the development of DCM [Citation12]. Antioxidants have been found useful in avoidance and treatment of DCM associated complications, earning them the title of natural treatment for DCM [Citation13].
Treatments for DCM mainly include intense glycemic control with oral hypoglycemics, frequent insulin, beta-blockers, and angiotensin-converting enzyme inhibitors (ACEi)/angiotensin receptor blockers (ARB) [Citation14]. Oral hypoglycemia reduces the production of hepatic glucose and decreases intestinal glucose absorption. Insulin also stimulates peripheral glucose uptake by skeletal muscle cells and fat, along with inhibiting glucose production from the liver. Beta-blockers inhibit the production of adrenalin, and ACE and ARB are found to be active on renin and angiotensin receptors, which help to reduce blood pressure. But these regimens have met with limited success in clinical trials even after being administered in a combined form. Moreover, these therapies have several side effects that limit their use [Citation15]. Hitherto, no singular effective treatment is available for DCM. Thus there is a scarcity to find effective treatment strategies for the prevention of DCM.
Piperine is reported to be the most abundant alkaloid present in black pepper (Piper nigrum Linn), which is extensively used as a spice [Citation16]. It has been reported that piperine possesses several inherent therapeutic qualities such as antioxidants [Citation17,Citation18], anti-apoptotic, and anti-inflammatory, anticonvulsant [Citation19], antimutagenic [Citation20], hepatoprotective activity [Citation21], immune inflection, antidepressive disorders, and elevating obesity and diabetes [Citation22]. Studies have found that Piperine antagonized the inflammatory process, which was induced by lipopolysaccharide and reduced the development of type I interferon [Citation23]. Studies have also reported that piperine inhibited pro-oxidant enzymes resulting in diminished blood glucose levels and up-regulated internal antioxidant enzymes [Citation24]. Also, it is found that secretion of inflammatory factors was markedly inhibited by piperine, and it also activated AMP protein kinase through pyroptosis in lipopolysaccharide-primed macrophages [Citation24]. Piperine is also known to be able to prevent cardiac disorder by activating peroxisome proliferator-activated receptor gamma (PPAR-γ) pathway [Citation25]. However, the effect of piperine in DCM complications has not been evaluated completely. The current research aims to investigate the cardioprotective effect of piperine against diabetes induced by streptozotocin (STZ) in laboratory rats by estimating several hemodynamic and biochemical parameters, along with molecular and apoptotic changes.
Materials and methods
Animals
Adult (8 to 10 weeks old) male Sprague-Dawley rats (200–250 g) were obtained from the Laboratory Animal Center of the Affiliated Hospital of North Sichuan Medical College. They were maintained at 24°C ± 1°C, with a relative humidity of 45–55% and a normal dark/light cycle. The animals had free access to standard pellet chow and water throughout the experimental protocol. All experiments were carried out between 0900 and 1700 h. All the experimental protocols involved in this experiment were carried out in accordance with guidelines and approved by the animal care and use committee of the Affiliated Hospital of North Sichuan Medical College.
Drugs and chemicals
Piperine (purity ≥97%, CAS No.: 94–62-2, synonyms: (E,E)-5-(3,4-methylenedioxyphenyl)-2,4-pentadienoylpiperidide, and 1-piperoylpiperidine) and STZ were purchased from Sigma Chemical Co. (St Louis, MO, USA). Total ribonucleic acid (RNA) Extraction kit and One-step Reverse transcription-polymerase chain reaction (RT-PCR) kit was purchased from MP Biomedicals India Private Limited, India.
Induction and assessment of diabetes
A single dose of 55 mg/kg STZ, prepared in citrate buffer (pH 4.4, 0.1 M), was injected intraperitoneally to induce diabetes [Citation26]. Age‐matched control rats received an equal volume of citrate buffer and were used along with diabetic animals. Diabetes was confirmed 48 h after STZ injection, blood samples were collected via retro-orbital plexus technique using heparinized capillary glass tubes, and plasma glucose levels were estimated by the enzymatic glucose oxidase peroxidase (GOD‐POD) diagnostic kit method. Rats having plasma glucose levels of more than 250 mg/dL were selected and used for the present study. Body weight and plasma glucose levels were measured before and at the end of the experiment.
Experimental design
Four weeks after the STZ injection, non-diabetic and diabetic rats were randomly selected and allocated into seven groups of 18 animals each as follows:
[A] Non-diabetic animals
Normal non-diabetic (ND): animals received oral gavage of 1 mL of 1% aqueous dimethyl sulfoxide (DMSO)
[B] Diabetic animals
Diabetic (STZ) control: animals received oral gavage of 1 mL of 1% aqueous DMSO.
Diabetic (STZ)+I (10): animals received a subcutaneous injection of insulin (10 IU/kg, s.c.) and 1 mL of 1% aqueous DMSO
Diabetic (STZ)+ P (10): animals received oral gavage of piperine (10 mg/kg) in 1 mL of 1% aqueous DMSO.
Diabetic (STZ)+ P (20): animals received oral gavage of piperine (20 mg/kg) in 1 mL of 1% aqueous DMSO.
Diabetic (STZ)+ P (40): animals received oral gavage of piperine (40 mg/kg) in 1 mL of 1% aqueous DMSO
Diabetic (STZ)+ P (40)+I (10):animals received oral gavage of piperine (80 mg/kg) in 1 mL of 1% aqueous DMSOalong with subcutaneous injections of insulin (10 IU/kg, s.c.).
Piperine was freshly prepared in three different dosages (10, 20, and 40 mg/kg) and administered orally to all groups at a pre-fixed time once daily for 28 days after week 5 of STZ injection. The fasting blood glucose levels were estimated using a glucometer at two intervals, i.e. day 0 (before the start of the study) and day 28 (end of the study) [Citation27]. At the end of the treatment period, rats were anesthetized by ethereal anesthesia, and blood was withdrawn by a retro-orbital puncture. Each blood sample was collected into separate vials for determination of serum parameters. Post blood collection and surgical procedure for assessment of electrocardiographic, hemodynamic, and left ventricle (LV) contractile function, animals were sacrificed by cervical dislocation. The heart of sacrificed animals was rapidly removed and stored at 80°C for biochemical (n = 6) and RT-PCR analyzes (n = 4). Heart of three rats (n = 3) from each group was isolated and fixed for histopathological evaluation.
Serum biochemistry
Serum was separated by centrifugation using Eppendorf Cryocentrifuge (model No. 5810, Germany), maintained at 4°C, and run at a speed of 7,000 rpm for 15 min. Serum lactate dehydrogenase (LDH), Creatine Kinase-MB (CK-MB), serum insulin, and blood glucose levels were measured by spectrophotometer (UV/VISspectrophotometer, Jasco V-530, Japan) using reagent kits according to manufacturer’s protocol (Accurex Biomedical Pvt. Ltd., Mumbai, India).
Measurement of electrocardiographic, hemodynamic, and left ventricular function
At the end of the study period, animals were anesthetized with urethane (1.25 g/kg, i.p.). The motivation behind this choice was the minimal effect of urethane on cardiovascular and respiratory systems, combined with long-lasting anesthesia and rapid onset, following i.p. administration. Additionally, the duration of anesthesia is sufficient to carry out a surgical procedure and record hemodynamic and electrocardiographic parameters. Blood pressure was measured using a polyethylene cannula (PE 50), filled with heparinized saline (100 IU/mL), and connected to a pressure transducer. The cannula was connected to a transducer, and the signal amplified with the help of a bio-amplifier. Further, left ventricular systolic pressure was measured using a Millar mikro-tip transducer catheter (Model SRP-320, Millar instrument, INC 320–7051, Houston, TX) inserted into the left ventricle via the right carotid artery, and connected to a bio-amplifier. Electrocardiographic, hemodynamic changes, and left ventricular (LV) contractile function were recorded using an eight-channel recorder Power lab having LABCHART-6 pro software, by means of a data acquisition system (AD Instruments Pvt. Ltd. with software LABCHART 7.3 pro software, Australia).
Biochemical estimation
Tissue homogenate preparation
All animals were sacrificed at the end of the study, i.e., on the 56th day; the heart was immediately isolated. Tissue homogenates were prepared with 0.1 M tris-HCl buffer (pH 7.4), and supernatant of homogenates were employed to estimate superoxide dismutase (SOD), reduced glutathione (GSH), lipid peroxidation (MDA content), nitric oxide (NO content) and Na-K-ATPase as described previously [Citation28,Citation29].
Mitochondrial enzymes estimation
Heart mitochondrial isolation was measured according to a previously described method [Citation30]. Mitochondrial complex-I activity was measured spectrophotometrically according to a previously described method [Citation31]. Mitochondrial Complex-II activity Succinate dehydrogenase (SDH) was measured spectrophotometrically according to an already described method [Citation31]. Mitochondrial redox activity (Complex-III), i.e. the MTT reduction rate, was used to assess the activity of mitochondrial respiratory chain in isolated mitochondria. It was determined, according to an already established method [Citation31]. Mitochondrial complex-IV (Cytochrome oxidase assay) activity was assayed in heart muscle mitochondria according to the previously described method [Citation31].
ANP, BNP, cTn-I, Bcl2, Bax/Bcl2, and caspase3 messenger ribonucleic acid (mRNA) expression by RT-PCR in heart
The levels of messenger ribonucleic acid (mRNA) were analyzed in heart tissue using RT-PCR, as described previously [Citation32]. Single-stranded complementary DNA (cDNA) was synthesized from 5 mg of total cellular RNA using a reverse transcriptase kit, as described previously [Citation33]. Amplification of b-actin served as control for sample loading and integrity. Polymerase chain reaction (PCR) products were detected by electrophoresis on a 1.5% agarose gel containing ethidium bromide. The size of amplicons was confirmed using a 100-bp ladder as standard size marker. The amplicons were visualized, and images were captured using a gel documentation system (Alpha Innotech Inc., San Leandro, CA, USA). The expression of all the genes was assessed by generating densitometry data for band intensities in different sets of experiments. It was produced by analyzing the gel images in Image J software (Version 1.33, Wayne Rasband, NIH, Bethesda, MD, USA), semi-quantitatively. The band intensities were compared with constitutively expressed b-actin. The intensity of mRNAs was standardized against that of the b-actin mRNA from each sample, and the results were expressed as the PCR-product/b-actin mRNA ratio.
Histopathological evaluation
The isolated tissue was trimmed into small pieces and preserved in 10% formalin for 24 h. Specimens were cut in sections of 3–5 μm in thickness by microtome and stained by hematoxylin-eosin. The samples were mounted by disterene phthalate xylene (DPX). The photomicrographs of each tissue section were observed using Cell Imaging software for Life Science microscopy (Olympus Soft Imaging Solution GmbH, Munster, Germany).
Statistical analysis
Data were expressed as mean ± standard error means (SEM). Data analysis was performed using Graph Pad Prism 5.0 software (Graph Pad, San Diego, CA, USA). Data were analyzed by one-way analysis of variance (ANOVA), while Tukey’s multiple range tests and Dunnett’s Multiple Comparison Test were applied for post hoc analysis. A value of p < 0.05 was considered to be statistically significant.
Results
Effect of piperine on body weight, plasma glucose level, and serum insulin in rats
No significant (p < 0.05) difference was found in body weight, plasma glucose level, and serum insulin between normal and diabetic control rats before the administration of intraperitoneal STZ to the diabetic control rats. After eight weeks of peritoneal STZ injection, a significant (p < 0.05) decrease in body weight, serum insulin, and significant (p < 0.05) increase in blood glucose level were observed in diabetic control rats in comparison with normal ND rats. However, rats treated with piperine (20 and 40 mg/kg) and insulin (10 mg/kg) showed marked (p < 0.05) raise in body weight, serum insulin, and decreased blood glucose level compared to control rats. Moreover, it was also found that the combination treatment of insulin–piperine showed significantly (p < 0.05) increased body weight, serum insulin than piperine alone ().
Table 1. Effect of piperine on STZ-induced alterations in body weight, heart weight, heart weight body weight ratio, blood glucose level, food intake, water intake, urine output, and serum insulin in diabetic rats
Effect of piperine on food intake, water intake, and urine output in rats
Before the induction of DCM by intraperitoneal STZ, there was no notable (p < 0.05) difference in consumption of food and water along with urine output between control rats and normal ND rats. After eight weeks of STZ injection, there was a significant (p < 0.05) increase in food intake, water intake, and urine output of diabetic (STZ) control rats as compared to normal ND rats. Four weeks of piperine (20 and 40 mg/kg) and insulin (10 mg/kg) treated rats displayed significant (p < 0.05) inhibition of increased food intake, water intake, and urine output as compared to diabetic control rats. Additionally, the prevention of increased food intake and urine output were found to be significant (p < 0.05) in insulin–piperine combination-treated rats compared to piperine alone treated rats ().
Effect of piperine on absolute and relative heart weight in rats
Absolute and relative heart weight ratios markedly (p < 0.05) increased after eight weeks of STZ consumption in diabetic control rats as compared to normal, ND rats. However, piperine-treated diabetic rats (20 and 40 mg/kg), and insulin 10 mg/kg treated rats displayed significantly (p < 0.05) increased absolute and relative heart weight as compared to diabetic control rats. Whereas there were no remarkable (p < 0.05) changes in the combination treatment of insulin–piperine treated rats as compared to piperine alone ( and ).
Figure 1. Effect of piperine on STZ induced increased heart volume in diabetic rats
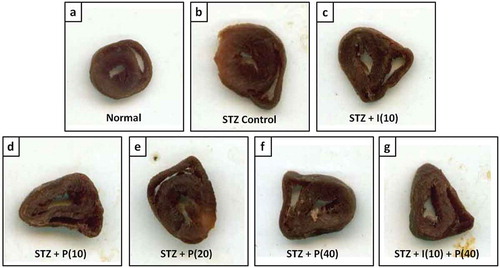
Effect of piperine on electrocardiography (ECG) in rats
Before the induction of DCM by intraperitoneal STZ, there were no notable (p < 0.05) differences in heart rate, QRS interval, QT Interval, QTc Interval, and RR interval of diabetic control rats and normal ND rats. Eight weeks of STZ injection resulted in significantly (p < 0.05) increased QRS interval, QT Interval, QTc Interval, and RR interval, and decreased heart rate of diabetic control rats induced by STZ as compared to normal ND rats. Four weeks of piperine (20 and 40 mg/kg) and insulin (10 mg/kg) treatment significantly (p < 0.05) prevented STZ induced increased QRS interval, QT Interval, QTc Interval, and RR interval and decreased heart rate as compared to diabetic control rats. Additionally, decrease in QRS interval, QT Interval, QTc Interval, and RR interval were found to be significant (p < 0.05) in insulin–piperine combination-treated rats compared to piperine alone treated rats ().
Table 2. Effect of piperine on STZ-induced alterations in echocardiogram parameters in diabetic rats
Effect of piperine on hemodynamic parameters in rats
A remarkable (p < 0.05) increase in systolic blood pressure (SBP), diastolic blood pressure (DBP), left ventricular end-diastolic pressure (LVEDP), Min dp/dt, pressure time index, and tau was observed in diabetic control rats as compared with normal, ND rats after eight weeks of peritoneal STZ injection. Whereas after eight weeks of peritoneal STZ injection, a notable (p < 0.05) decrease in mean arterial blood pressure (MABP), Max dp/dt, and contractility index were observed in diabetic control rats compared with normal, ND rats. However, rats treated with piperine (20 and 40 mg/kg) and insulin (10 mg/kg) showed markedly (p < 0.05) decreased SBP, DBP, LVEDP, Min dp/dt, pressure time index, and tau compared to diabetic control rats and increased MABP, Max dp/dt, and contractility index collated with diabetic control rats. Moreover, combination treatment of insulin–piperine on rats showed significantly (p < 0.05) decreased SBP, DBP, LVEDP, Min dp/dt, and pressure time index compared to piperine and insulin alone. However, no notable (p < 0.05) difference was found in tau, MABP, Max dp/dt, and contractility index between combination treatment of insulin–piperine treated rats and piperine alone treated rats ().
Effect of piperine on SOD, GSH, MDA, NO in rats
SOD and GSH markedly (p < 0.05) decreased in diabetic control rats compared with normal ND rats. However, notably (p < 0.05) increased MDA, and NO levels were observed in STZ injected diabetic control rats collated with normal, ND rats. Rats administered with piperine (20 and 40 mg/kg) and insulin (10 mg/kg) significantly (p < 0.05) ameliorated decreased SOD, and GSH level in the heart collated to diabetic control rats. Whereas a significant decrease in MDA and NO levels in piperine (20 and 40 mg/kg) and insulin (10 mg/kg) treated rats was reported as collated with diabetic control rats. Moreover, combination treatment of insulin–piperine had a significant (p < 0.05) effect on reduction of MDA and NO levels, and increase in SOD and GSH level as collated with piperine alone ().
Table 3. Effect of piperine on STZ-induced alterations hemodynamic parameters in diabetic rats
Effect of piperine on Na-K-ATPase, mitochondrial complex (I to IV), CK-MB, LDH in rats
There was a significant (p < 0.05) decrease inNa-K-ATPase, Complex (I to IV), and significant (p < 0.05) increase in serum CK-MB, serum LDH in diabetic control rat collated to normal, ND rats. Four-week treatment with piperine (20 and 40 mg/kg) and insulin (10 mg/kg) markedly (p < 0.05) ameliorated decreased Na-K-ATPase, Complex (I to IV) and markedly (p < 0.05) fell the elevated level of serum CK-MB, serum LDH as collated with diabetic control rats. Moreover, combination treatment of insulin–piperine was also found to be significant (p < 0.05) in reducing serum CK-MB, serum LDH and increasing Na-K-ATPase, Complex (I to IV) as compared to piperine alone ( and ).
Table 4. Effect of piperine on STZ-induced alterations SOD, GSH, MDA, NO, Na-K-ATPase, serum CK-MB and serum LDH in diabetic rats
Table 5. Effect of piperine on STZ-induced alterations in myocardial mitochondrial enzymes activity (complex I, complex II, complex III, and complex IV) in diabetic rats
Effect of piperine on the ANP, BNP, cTn-I, Bcl2, Bax/Bcl2, and caspase3 mRNA expression in rats
In mRNA expression, the experiment reported that the levels of atrial natriuretic peptide (ANP), brain natriuretic peptide (BNP), cardiac troponin (cTn-I), B-cell lymphoma 2 (Bcl2), the ratio of Bcl-2-associated X protein and B-cell lymphoma 2 (Bax/Bcl2), and caspase3were notably (p < 0.05) up-regulated in diabetic control rats collated with normal, ND rats. In contrast, apoptosis regulator Bcl2 was significantly (p < 0.05) down-regulated in diabetic control rats juxtaposed with normal, ND rats. After four weeks, treatment with piperine (20 and 40 mg/kg) and insulin (10 mg/kg) markedly improved the up-regulation of ANP, BNP, cTn-I, Bax/Bcl2 ratio, caspase3, and down-regulation of Bcl2 mRNA expression collated with diabetic control rats. Moreover, combination treatment of insulin–piperine had significantly (p < 0.05) down-regulated ANP, BNP, cTn-I, Bax/Bcl2 ratio, and caspase3 mRNA expression and up-regulated Bcl2 mRNA expression as compared to piperine alone ().
Figure 2. Effect of piperine on STZ-induced altered mRNA protein expression in diabetic rats
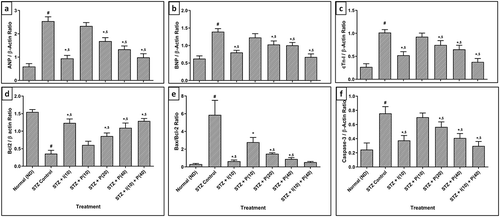
Effect of piperine on histopathological alteration in rats
Heart tissues from several groups were stained with H&E stain for the analysis of myocardial degeneration, interstitial inflammation, and interstitial hemorrhage. Heart tissue from normal ND rats revealed normal morphologies without inflammation, hemorrhage, and necrosis. Cardiac tissue of control rats with diabetes showed significant (p < 0.05) myocardial degeneration, interstitial hemorrhage, and interstitial inflammation. Treatment with piperine (20 and 40 mg/kg) and insulin (10 mg/kg) markedly (p < 0.05) reduced severe myocardial degeneration, interstitial inflammation, and interstitial hemorrhage as collated with the control rats with diabetes. Moreover, myocardial degeneration, interstitial hemorrhage, and interstitial inflammation of heart tissue were markedly (p < 0.05) improved in rats with a combination treatment of insulin–piperine as compared to piperine alone ().
Figure 3. Effect of piperine on STZ-induced alterations in heart histopathology in diabetic rats
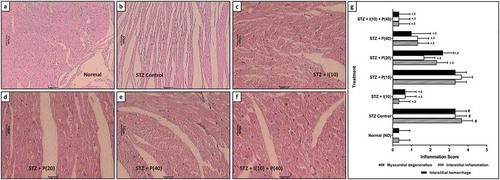
Discussion
Diabetes is a chronic metabolic disorder that results in persistent increased blood glucose levels more than usual. Studies have reported that patients with sustained hyperglycemia are more prone to other risk factors of diabetes, such as diabetic nephropathy, DCM, and cardiac hypertrophy [Citation34]. Researchers have also found that due to dysfunction of the diastolic and systolic pressure (early and delay) in diabetes, retention of sodium, calcium, and potassium loss take place in the intracellular membrane. Studies have found that cardiac dysfunction takes place at 2 to 3 months and even advance at 15 days from day one of diabetes. In this study, piperine and combination treatment of piperine-insulin have shown a significant effect on blood glucose, electrocardiogram, hemodynamic, antioxidant, and cell apoptosis. It is reported that piperine has agonistic activity on the β adrenergic receptors, which increases blood glucose levels during glycogenolysis and gluconeogenesis [Citation27].
In the present investigation, both moderate and higher doses of piperine have significantly increased body weight and decreased food intake, urine output, water intake. Piperine has also reduced hypoglycemia and increased the level of insulin in serum compared with the diabetic control rats, which were manifested clearly in a previous report [Citation27]. Increased blood glucose level exhibits gluconeogenesis and glycogenolysis in the liver, which stimulates β2 adrenergic receptors. Piperine has an agonistic effect on β adrenergic receptors; thus, piperine reduced increased blood glucose levels and raised insulin secretion.
In this study, STZ-induced DCM which was evident by abnormal ECG changes such as decreased heart rate, prolongation of QRS interval, QTc interval, RR interval, QT interval. This ECG abnormality takes place in DCM rats because of circulating free fatty acids, upregulated systemic inflammation, dysfunction of endothelial vasomotor, and effects of vascular system due to advanced glycation. Piperine and combination of piperine and insulin showed significant restoration of ECG parameters. Among all the treatment groups, higher and moderate dose, and combination of insulin and piperine showed the best results in terms of restoring ECG readings to normal levels.
Studies have reported that the initial stage of DCM is diastolic dysfunction in the left ventricle (LV) after the initiation of systolic dysfunction [Citation35]. The pathology behind the dysfunction of LV in diabetic rats is not entirely illustrated. In this experiment, due to hyperglycemia, rats have abnormal cardiac tissue, decreased heart weight, and increased heart/body weight ratio. Besides, this abnormal hemodynamic condition resulted in hypertrophic cardiomyopathy, and fibrosis leading to impairment of ventricular relaxation and decrease in compliance of cardiac muscle. SBP, DBP, LVEDP, Min dp/dt, tau, and pressure time index significantly increased, and heart rate, MABP, Max dp/dt, contractility index decreased due to changes in LV wall thickness in STZ-induced diabetic control rats. Whereas piperine, moderate, and higher dose, significantly prevented the increase in SBP, DBP, LVEDP, Min dp/dt, tau, and pressure time index. It improved decreased heart rate, MABP, Max dp/dt, and contractility index.
Researchers have reported that reactive oxygen species (ROS) are found to be up-regulated in diabetic people [Citation36]. Studies have also indicated that the development and progression of DCM are due to ROS generation in the heart myocardium. In prediabetes also, dysfunction of LV diastolic and ventricular hypertrophy can occur due to elevated oxidative stress. It is also reported that oxidative stress originates from subsarcolemmal mitochondria in pre-diabetic patients [Citation37]. It has been mentioned in a previous study that the production of ROS and imbalance of ROS pathway are associated with superoxide-mediated damage and cellular dysfunction [Citation38,Citation39]. The oxidative stress can be defensively prevented with antioxidants in diabetic myocardium [Citation40]. In our investigation, higher levels of SOD and GSH, and lower MDA levels were reported in the control diabetic rats, which corroborates with the existing literature. Piperine and the combination of insulin and piperine have reduced the MDA level by increasing the SOD and GSH levels. Studies have suggested that diabetes-associated cardiac inflammation may be the hallmark of the development of DCM [Citation41]. The rats treated with piperine and the combination of insulin and piperine showed a remarkable down-regulation of MDA content and up-regulation of SOD and GSH content in heart tissue.
Researchers have found in recent studies that in cardiomyopathy, the activity of cardiac markers such as LDH and CK-MB were increased due to myocardial dysfunction. Similarly, in our investigation also, we have seen the serum CK-MB, and LDH activities increased in STZ-induced diabetic control rats. In patients with diabetes, due to cardiac muscle damage and ventricular dysfunction, the movement of LDH and CK-MB is growing [Citation42]. In this experiment, we observe that these serum cardiac markers (LDH, CK-MB) have been up-regulated markedly in control diabetic rats induced by STZ. In contrast, piperine treatment brought these cardiac markers levels noticeably close to normal. Troponin T (TnT) is the amino acid sequence found in myocardial tissue, which is altered due to cardiac damage [Citation43]. In cardiac tissue, the calcium-mediated interaction takes place between myosin and actin, which is controlled by TnT and troponin I (TnI). Several studies have reported that due to hyperglycemia, cardiac risk can occur; thus, the release of cTnT is high [Citation44]. In our experiment, the level of cTnT was increased due to hyperglycemia, whereas piperine and combination of piperine and insulin-treated rats showed cTnT level near to normal.
The natriuretic peptides (NP) are established to be a remarkable biomarker to detect and monitor heart failure (HF). ANP and BNP are found to be the two primary cardiac synthesized NPs, which have several potential activities such as natriuretic, diuretic, vasodilator, and renin- and aldosterone-suppression [Citation45]. In pressure-induced cardiac hypertrophy, genetic mutation of ANP results in the exaggerated condition of the heart [Citation46,Citation47]. In patients with DCM, rising ANP levels enhances the severity of cardiac dysfunction. Due to the expansion of volume and extreme pressure-overload in ventricles, BNP was released from ventricular heart muscles. During hypertension, heart failure, or myocardial infarction, the elevation of BNP levels has been observed [Citation48]. Here in diabetic control rats, significant amount of BNP and ANP was found. However, rats treated with piperine showed a markedly low level of ANP and BNP.
Histopathological examination of the cardiac tissue from STZ-induced diabetic control rats showed that diabetes is the primary cause of abnormal ultrastructural changes such as myocardial degeneration, interstitial inflammation, and interstitial hemorrhage. These abnormalities are responsible for elevated oxido-nitrosative stress, which is associated with an extensive production of ROS. Piperine treatment showed inhibition of STZ-induced myocardial degeneration, interstitial inflammation, and interstitial hemorrhage.
For further elucidation of the cardioprotective effect of piperine against STZ-induced DCM, the present study investigated the impact of piperine on mRNA protein expression. Studies have found that various mechanisms are responsible for cell apoptosis, such as dyslipidemia, mitochondrial dysfunction, ROS generation, inflammation, hyperglycemia in DCM [Citation49]. In this study, diabetic rats noticeably exhibited up-regulated cardiac levels of Bax, caspase-3, and down-regulated Bcl-2. Bax, which is a pro-apoptotic protein, binds to the mitochondrial membrane for the generation of channels, and the secretion of cytochrome C. Cytochrome C is further inserted to apoptotic protease-activating factor (APAF)-1. Researchers have found that caspase-3 is activated through apoptosome, which involves inducing necrosis to promote the activation of DNA fragmentation factor subunit beta, such as caspase-3-activated DNase (CAD), resulting in fragmentation of DNA [Citation50]. It has already been observed that apoptosis due to ATP-induction was inhibited with the help of the potentiality of piperine through defeats of the signaling pathway of caspase-3 in HeLa cells [Citation51].
The current research data are in agreement with the previous experiment as our present research also demonstrated that piperine had down-regulated caspase 3 apoptotic proteins in STZ-induced diabetic rats. Piperine decreased Bax and up-regulated Bcl-2 protein in diabetic control rats. Bcl-2 is associated with cytochrome c from mitochondria, which leads to preventing the secretion of cytochrome c from mitochondria, resulting in protection against apoptosis [Citation51]. Researchers have also noticed that piperine-enhanced cell apoptosis by inhibiting the Bax apoptotic protein and the results of the current research are in line with the previous report [Citation52].
Various researches have demonstrated the prospect of insulin as a therapy for diabetes. Insulin is adequate for both type I and type II diabetes. It is the hormone that directly acts on lowering the blood glucose level. However, due to prolonged use of insulin, several side effects can be seen, such as swelling of arms and legs, weight gain, low blood sugar (hypoglycemia), injection site reactions, skin changes at the injection site (lipodystrophy). On the flip side, naturally originated plant moieties are found to be a favorable alternative therapy for DCM. Researchers have identified the potential of other alkaloids as well such as berberine [Citation53], Matrine α type alkaloids [Citation54], and piperine [Citation27]. Piperine has also been documented for its blood glucose reducing potential. Thus evidence from the current research demonstrates that piperine perhaps substitutes remedial treatment of DCM clinically.
Conclusion
In conclusion, the current study demonstrated that piperine attenuates STZ-induced diabetic cardiomyopathy by reducing oxidative stress. Piperine (1-piperonal piperidine) obtained from Piper spp. ameliorated echocardiogram and restored left ventricular function by reducing the left ventricular wall thickness. Moreover, piperine was also found to maintain the activity of heart mitochondria by renovating the complexes in the respiratory chain (Complex I–IV) and cTn-I level, and it also prevented apoptosis (Caspase 3, Bax, Bcl2). Piperine also modulated renin- and aldosterone-suppression via ANP and BNP down-regulation (Graphical Abstract). Thus piperine may be a better approach for the treatment of DCM.
Summary
In the current investigation, the efficacy of piperine was examined against STZ-induced DCM in rats. Piperine treatment significantly attenuated STZ-induced alterations in hemodynamic (SBP, DBP, LVEDP, MABP, Max dp/dt, Min dp/dt, contractility index, pressure time index, tau) and electrocardiographic (heart rate, QRS interval, QT Interval, QTc Interval, and RR interval), blood glucose and serum (insulin, CK-MB, LDH) biochemistry, oxido-nitrosative stress in cardiac tissue, the activity of heart mitochondrial enzymes (I–IV), altered mRNA expressions of ANP, BNP, cTn-I, Bcl2, the ratio of Bax and Bcl2 and caspase3 as well as cardio histological aberrations. Thus, piperine can be considered as an essential therapeutic moiety for the management of DCM patients.
Author’s contribution
YW: Concepts, Design, Manuscript preparation, Manuscript editing, Manuscript review
HS: Experimental studies, Data acquisition, Manuscript editing, Manuscript review
JZ: Concepts, Design, Statistical analysis, Manuscript review
ZX: Concepts, Manuscript preparation, Manuscript editing, Manuscript review
CX: Data acquisition, Manuscript editing, Manuscript review
Disclosure statement
The authors declare no conflict of interest.
Additional information
Funding
References
- Guariguata L, Whiting DR, Hambleton I, et al. Global estimates of diabetes prevalence for 2013 and projections for 2035. Diabetes Res Clin Pract. 2014 Feb;103(2):137–149.
- Boudina S, Abel ED. Diabetic cardiomyopathy, causes and effects. Rev Endocr Metab Disord. 2010 Mar;11(1):31–39.
- Bugger H, Bode C. The vulnerable myocardium. Hämostaseologie. 2015;35(1):17–24.
- Trachanas K, Sideris S, Aggeli C, et al. Diabetic cardiomyopathy: from pathophysiology to treatment. Hellenic J Cardiol. 2014 Sep-Oct;55(5):411–421.
- Falcão-Pires I, Leite-Moreira AF. Diabetic cardiomyopathy: understanding the molecular and cellular basis to progress in diagnosis and treatment. Heart Fail Rev. 2012;17(3):325–344.
- Picatoste B, Ramirez E, Caro-Vadillo A, et al. Sitagliptin reduces cardiac apoptosis, hypertrophy and fibrosis primarily by insulin-dependent mechanisms in experimental type-II diabetes. Potential roles of GLP-1 isoforms. PLoS One. 2013;8(10):e78330.
- Giacco F, Brownlee M. Oxidative stress and diabetic complications. Circ Res. 2010 Oct 29;107(9):1058–1070.
- Varga ZV, Giricz Z, Liaudet L, et al. Interplay of oxidative, nitrosative/nitrative stress, inflammation, cell death and autophagy in diabetic cardiomyopathy. Biochim Biophys Acta. 2015 Feb;1852(2):232–242.
- Huang WQ, Liu SR, Huang ZM, et al. Magic electron affection in preparation process of silicon nanocrystal. Sci Rep. 2015 Apr 24;5(1):9932.
- Mohamed A, Amer MA, Hemieda FA, et al. Cardioameliorative effect of punicalagin against streptozotocin-induced apoptosis, redox imbalance, metabolic changes and inflammation. Egypt J Basic Appl Sci. 2015 Dec;2(4):247–260
- Korkmaz-Icöz S, Al Said S, Radovits T, et al. Oral treatment with a zinc complex of acetylsalicylic acid prevents diabetic cardiomyopathy in a rat model of type-2 diabetes: activation of the Akt pathway. Cardiovasc Diabetol. 2016;15(1):75.
- Bhattacharya S, Manna P, Gachhui R, et al. D-Saccharic acid 1, 4-lactone protects diabetic rat kidney by ameliorating hyperglycemia-mediated oxidative stress and renal inflammatory cytokines via NF-κB and PKC signaling. Toxicol Appl Pharmacol. 2013;267(1):16–29.
- Wen HL, Liang ZS, Zhang R, et al. Anti-inflammatory effects of triptolide improve left ventricular function in a rat model of diabetic cardiomyopathy. Cardiovasc Diabetol. 2013 Mar 25;12(1):50.
- Borghetti G, von Lewinski D, Eaton DM, et al. Diabetic cardiomyopathy: current and future therapies. Beyond glycemic control. Front Physiol. 2018;9:1514.
- Ge ZD, Lian Q, Mao X, et al. Current status and challenges of NRF2 as a potential therapeutic target for diabetic cardiomyopathy. Int Heart J. 2019 May 30;60(3):512–520.
- Srinivasan K. Black pepper and its pungent principle-piperine: a review of diverse physiological effects. Crit Rev Food Sci Nutr. 2007;47(8):735–748.
- Rauscher FM, Sanders RA, Watkins JB 3rd. Effects of piperine on antioxidant pathways in tissues from normal and streptozotocin-induced diabetic rats. J Biochem Mol Toxicol. 2000;14(6):329–334.
- Prakash UN, Srinivasan K. Gastrointestinal protective effect of dietary spices during ethanol-induced oxidant stress in experimental rats. Appl Physiol Nutr Metab. 2010 Apr;35(2):134–141.
- Mishra A, Punia JK, Bladen C, et al. Anticonvulsant mechanisms of piperine, a piperidine alkaloid. Channels. 2015;9(5):317–323.
- El Hamss R, Idaomar M, Alonso-Moraga A, et al. Antimutagenic properties of bell and black peppers. Food Chem Toxicol. 2003 Jan;41(1):41–47.
- Matsuda H, Ninomiya K, Morikawa T, et al. Protective effects of amide constituents from the fruit of Piper chaba on d-galactosamine/TNF-α-induced cell death in mouse hepatocytes. Bioorg Med Chem Lett. 2008;18(6):2038–2042.
- Meghwal M, Goswami TK. Piper nigrum and piperine: an update. Phytother Res. 2013 Aug;27(8):1121–1130.
- Bae GS, Kim MS, Jung WS, et al. Inhibition of lipopolysaccharide-induced inflammatory responses by piperine. Eur J Pharmacol. 2010 Sep 10;642(1–3):154–162.
- Liang Y-D, Bai W-J, Li C-G, et al. Piperine suppresses pyroptosis and interleukin-1β release upon ATP triggering and bacterial infection. Front Pharmacol. 2016;7:390.
- Yan J, Xu SC, Kong CY, et al. Piperine alleviates doxorubicin-induced cardiotoxicity via activating PPAR-gamma in mice. PPAR Res. 2019;2019:2601408.
- Bhatt LK, Veeranjaneyulu A. Minocycline with aspirin: a therapeutic approach in the treatment of diabetic neuropathy. Neurol Sci. 2010 Dec;31(6):705–716.
- Atal S, Agrawal RP, Vyas S, et al. Evaluation of the effect of piperine per se on blood glucose level in alloxan-induced diabetic mice. Acta Pol Pharm. 2012 Sep-Oct;69(5):965–969.
- Kandhare AD, Ghosh P, Ghule AE, et al. Elucidation of molecular mechanism involved in neuroprotective effect of C oenzyme Q 10 in alcohol‐induced neuropathic pain. Fundam Clin Pharmacol. 2013;27(6):603–622.
- Visnagri A, Kandhare AD, Kumar VS, et al. elucidation of ameliorative effect of Co-enzyme Q10 in streptozotocin-induced diabetic neuropathic perturbation by modulation of electrophysiological, biochemical and behavioral markers. Biomed Aging Pathol. 2012;2(4):157–172.
- Ketkar S, Rathore A, Kandhare A, et al. Alleviating exercise-induced muscular stress using neat and processed bee pollen: oxidative markers, mitochondrial enzymes, and myostatin expression in rats. Integr Med Res. 2015;4(3):147–160.
- Andreazza AC, Shao L, Wang JF, et al. Mitochondrial complex I activity and oxidative damage to mitochondrial proteins in the prefrontal cortex of patients with bipolar disorder. Arch Gen Psychiatry. 2010 Apr;67(4):360–368.
- Visnagri A, Kandhare AD, Bodhankar SL. Renoprotective effect of berberine via intonation on apoptosis and mitochondrial-dependent pathway in renal ischemia reperfusion-induced mutilation. Ren Fail. 2015 Apr;37(3):482–493.
- Kandhare AD, Ghosh P, Bodhankar SL. Naringin, a flavanone glycoside, promotes angiogenesis and inhibits endothelial apoptosis through modulation of inflammatory and growth factor expression in diabetic foot ulcer in rats. Chem Biol Interact. 2014;219:101–112.
- Katsuda Y, Sasase T, Tadaki H, et al. Contribution of hyperglycemia on diabetic complications in obese type 2 diabetic SDT fatty rats: effects of SGLT inhibitor phlorizin. Exp Anim. 2015;64(2):161–169.
- Schannwell CM, Schneppenheim M, Perings S, et al. Left ventricular diastolic dysfunction as an early manifestation of diabetic cardiomyopathy. Cardiology. 2002;98(1–2):33–39.
- Wold LE, Ren J. Streptozotocin directly impairs cardiac contractile function in isolated ventricular myocytes via a p38 map kinase-dependent oxidative stress mechanism. Biochem Biophys Res Commun. 2004 Jun 11;318(4):1066–1071.
- Koncsos G, Varga ZV, Baranyai T, et al. Diastolic dysfunction in prediabetic male rats: role of mitochondrial oxidative stress. Am J Physiol Heart Circ Physiol. 2016 Oct 1;311(4):H927–H943.
- Boudina S, Abel ED. Diabetic cardiomyopathy revisited. CIRC J. 2007 Jun 26;115(25):3213–3223.
- Khullar M, Al-Shudiefat AA, Ludke A, et al. Oxidative stress: a key contributor to diabetic cardiomyopathy. Can J Physiol Pharmacol. 2010 Mar;88(3):233–240.
- Kandhare AD, Raygude KS, Ghosh P, et al. Therapeutic role of curcumin in prevention of biochemical and behavioral aberration induced by alcoholic neuropathy in laboratory animals. Neurosci Lett. 2012 Mar 5;511(1):18–22.
- Mano Y, Anzai T, Kaneko H, et al. Overexpression of human C-reactive protein exacerbates left ventricular remodeling in diabetic cardiomyopathy. Circ J. 2011;75(7):1717–1727.
- Huang TH, Peng G, Kota BP, et al. Pomegranate flower improves cardiac lipid metabolism in a diabetic rat model: role of lowering circulating lipids. Br J Pharmacol. 2005 Jul;145(6):767–774.
- O’brien P, Smith D, Knechtel T, et al. Cardiac troponin I is a sensitive, specific biomarker of cardiac injury in laboratory animals. Lab Anim. 2006;40(2):153–171.
- Henri C, Heinonen T, Tardif J-C. The role of biomarkers in decreasing risk of cardiac toxicity after cancer therapy: supplementary issue: biomarkers and their essential role in the development of personalised therapies (A). Cancer Biomark. 2016;8(BIC):S31798.
- Nishikimi T, Maeda N, Matsuoka H. The role of natriuretic peptides in cardioprotection. Cardiovasc Res. 2006 Feb 1;69(2):318–328.
- Franco V, Chen YF, Oparil S, et al. Atrial natriuretic peptide dose-dependently inhibits pressure overload-induced cardiac remodeling. Hypertension. 2004 Nov;44(5):746–750.
- Fillmore N, Mori J, Lopaschuk GD. Mitochondrial fatty acid oxidation alterations in heart failure, ischaemic heart disease and diabetic cardiomyopathy. Br J Pharmacol. 2014 Apr;171(8):2080–2090.
- Staudt A, Staudt Y, Hummel A, et al. Effects of immunoadsorption on the nt-BNP and nt-ANP plasma levels of patients suffering from dilated cardiomyopathy. Ther Apheresis Dialysis. 2006 Feb;10(1):42–48.
- Xia Z, Huynh T, Ren P, et al. Large domain motions in Ago protein controlled by the guide DNA-strand seed region determine the Ago-DNA-mRNA complex recognition process. PLoS One. 2013;8(1):e54620.
- Liu YL, Gao X, Jiang Y, et al. Expression and clinicopathological significance of EED, SUZ12 and EZH2 mRNA in colorectal cancer. J Cancer Res Clin Oncol. 2015 Apr;141(4):661–669.
- Jafri A, Siddiqui S, Rais J, et al. Induction of apoptosis by piperine in human cervical adenocarcinoma via ROS mediated mitochondrial pathway and caspase-3 activation. Excli J. 2019;18:154–164.
- Patel N, Joseph C, Corcoran GB, et al. Silymarin modulates doxorubicin-induced oxidative stress, Bcl-xL and p53 expression while preventing apoptotic and necrotic cell death in the liver. Toxicol Appl Pharmacol. 2010;245(2):143–152.
- Chang W, Chen L, Hatch GM. Berberine as a therapy for type 2 diabetes and its complications: from mechanism of action to clinical studies. Biochem Cell Biol. 2015;93(5):479–486.
- Kochanowska-Karamyan AJ, Hamann MT. Marine indole alkaloids: potential new drug leads for the control of depression and anxiety. Chem Rev. 2010 Aug 11;110(8):4489–4497.