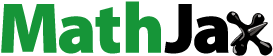
Abstract
Objective
To investigate the differences in the metabolic indicators and sex hormones between obese and non-obese patients with polycystic ovary syndrome (PCOS), and their impacts on endometrial receptivity (ER).
Methods
We selected 255 individuals with PCOS, and categorized them into the obese groups, including the OP group (obese patients with PCOS) and the ON group (obese patients without PCOS), and selected 64 individuals who were categorized in the non-obese groups, namely, the control groups, which comprise the NP group (non-obese patients with PCOS) and the NN group(non-obese patients without PCOS). The one-way analysis of variance (ANOVA) and Mann-Whitney U tests were used to compare the metabolic indicators, and sex hormone-associated and ER-associated indicators between the groups. The correlation between the aforementioned clinical markers and ER was analyzed using the Pearson’s correlation coefficient.
Results
(1) In comparison with the NP group, the OP group exhibited higher levels (p < .01) of free androgen index (FAI), anti-müllerian hormone (AMH), fasting insulin (FINS), insulin level within 60 min, 120 min, and 180 min—60minINS, 120minINS, and 180minINS, respectively, fasting blood glucose (FBG), blood glucose level within two hours (2hGlu), homeostatic model assessment for insulin resistance (HOMA-IR), triglyceride (TG), low-density lipoprotein cholesterol (LDL-C), waist-to-hip ratio (WHR), waist circumference, hip circumference, the ratio of the maximum blood flow velocity of the uterine artery during systole to the blood flow velocity of the uterine artery at the end of diastole (uterine artery S/D), and blood flow resistance index (RI) of the uterine artery. In comparison with the NP group, the OP group exhibited lower levels (p < .01) of sex hormone binding globulin (SHBG), dehydroepiandrosterone (DHEA), high molecular weight adiponectin (HMWA), and high-density lipoprotein cholesterol (HDL-C). (2) In the PCOS group, RI was significantly positively correlated with FAI, FINS, 120minINS, HOMA-IR, and WHR (p < .01), and significantly negatively correlated with SHBG, HDL-C, and HMWA (p < .01); uterine artery S/D was significantly positively correlated with FAI, FINS, 2hGlu, HOMA-IR, LDL-C, and WHR (p < .01), significantly positively correlated with 120minINS and FBG (p < .05), and significantly negatively correlated with SHBG and HMWA (p < .01).
Conclusion
(1) The OP group exhibited obvious metabolic disorders and poor ER, which was manifested as low levels of SHBG and HMWA, and high levels of FAI, HOMA-IR, WHR, uterine artery S/D, and RI. (2) In patients with PCOS, there was a substantial correlation between ER-associated indicators RI and uterine artery S/D and FAI, FINS, 120minINS, HOMA-IR, WHR, SHBG, and HMWA.
Introduction
Polycystic ovary syndrome (PCOS) is the most prevalent endocrine condition in women of reproductive age. The pathogenesis of PCOS may be associated with hyperandrogenemia (HA), hyperinsulinemia, an imbalance in the ratio of luteinizing hormone to follicle-stimulating hormone (LH/FSH), metabolic abnormalities, inflammation, advanced glycation end products (AGEs), and endoplasmic reticulum stress [Citation1]. Insulin resistance (IR) is the primary etiological factor in the pathogenesis of PCOS, and it is not related to obesity [Citation2]. HA is a potential factor that significantly affects IR in patients with PCOS [Citation3]. The impact of abdominal obesity on PCOS may be greater than anticipated. This impact is associated with HA and IR [Citation4].
Endometrial receptivity (ER) refers to a series of physiological changes in which the endometrium provides the best environment for embryo localization, adhesion, invasion, and implantation. Normal ER refers to the opening of the ‘implantation window’ of the endometrium at the appropriate time, which is a determining factor for successful embryo implantation. Decreased endometrial receptivity can lead to adverse pregnancy outcomes such as implantation failure, miscarriage, and biochemical pregnancy. Therefore, evaluation of ER plays an extremely important role, especially for PCOS patients who often suffer from infertility. At present, the evaluation of ER mainly includes evaluation methods based on ultrasound imaging, histopathology, molecular biology, and multi omics. Among them, ultrasound imaging technology is the most important noninvasive evaluation method, and its main indicators include endometrial thickness, morphology and blood flow signals, endometrial contractility and peristaltic waves, etc. It has the advantages of safety, affordability, repeatability, easy operation and high acceptance, and is widely used in clinical practice.
According to evidence-based findings, both hormone disorders and metabolic abnormalities in patients with PCOS affect ER [Citation5]. However, results are inconsistent among current studies. In this study, preliminary investigation on variations in ER between patients with PCOS, associated with different body mass index (BMI), as well as in ER between those with different metabolic homeostatic model assessment for insulin resistance (HOMA-IR), free androgen index (FAI), waist-to-hip ratio (WHR), high molecular weight adiponectin (HMWA), blood lipids, etc., was conducted, to find more about the correlation between metabolic disorders and ER, thereby providing a theoretical basis for improving ER in patients with PCOS at early stages and the pregnancy rate.
Study participants and methods
Study participants
Between December 2020 and October 2022, 319 patients who visited the Obstetrics and Gynecology Outpatient Department of the Second Hospital of Shanxi Medical University were enrolled in the study. Based on their PCOS and BMI values, the patients were divided into four groups: the OP group, which comprised of 110 obese patients with PCOS; the NP group, which consisted of 145 non-obese patients with PCOS; the ON group, which consisted of 28 obese patients without PCOS; and the NN group, which consisted of 36 non-obese patients without PCOS. Each participant signed the informed consent form. This study was approved by the ethics committee of the Shanxi Bethune Hospital.
Inclusion criteria: Patients who were aged 18 years old or above. According to the definition of obesity in the Asia-Pacific region, we defined obesity as BMI ≥ 25 kg/m2 [Citation6]. Patients with a BMI ranging between 18.5 and 25 kg/m2 were categorized into the non-obese groups. The Rotterdam PCOS diagnostic criteria were used while determining the diagnosis of PCOS [Citation7]. Participants selected in this study met a minimum of two out of the following three criteria: (1) Women experiencing irregular menstrual cycles and/or infrequent or absent ovulation. (2) Women exhibiting clinical and/or biochemical signs of elevated androgen hormone levels. (3) Women who received a preliminary diagnosis of polycystic ovaries through ultrasonography.
Exclusion criteria: (1) Pregnant women. (2) Women with a psychological or psychologically associated illness, with a history of: surgery, radiation, or chemotherapy for malignancies of adrenal glands in the pelvis, and smoking or chronic alcoholism. (3) Women with any one of the following diseases: Cushing’s syndrome, congenital adrenal hyperplasia, hyperprolactinemia, hypertension, diabetes mellitus, androgen-secreting tumors, fluid accumulation in the fallopian tube, intrauterine adhesion, multiple uterine endometrial polyps, chromosomal disorders, recurrent infertility, organic diseases of the uterus or ovary (such as endometriosis or adenomyosis), ovarian diseases, thyroid diseases, cardiovascular diseases, cerebrovascular diseases, severe malnutrition, liver diseases, kidney diseases, or other primary diseases. (4) Women who took hormonal medications or medications that have an impact on the levels of insulin or sex hormones in the last three months.
Study methods
Medical records of the study participants were compiled. Their body height and weight were measured, and their BMI was calculated. Their waist and hip circumferences were measured, and their WHR was calculated. All the study participants were required to fast for 12 h.
On the second day of the follicular phase, blood samples were taken to measure the levels of testosterone (T), luteinizing hormone (LH), follicle-stimulating hormone (FSH), estradiol (E2), progesterone (P), prolactin (PRL), and anti-müllerian hormone (AMH) using the chemiluminescence technique. Concurrently, blood specimens were taken for the oral glucose tolerance test (OGTT) and insulin (INS) release test. Venous blood samples were drawn to measure fasting blood glucose (FBG) using the hexokinase method and fasting insulin (FINS) levels using the chemiluminescence technique. After 5 min, the study participants orally consumed 165 ml of a 50% glucose solution (75 grams of glucose). Their venous blood samples were taken at regular intervals of 60, 120, and 180 min to measure the INS and glucose (Glu) levels. HOMA-IR was calculated based on the FINS and FBG values. IR was assessed using the following formula: Blood Glucose (mmol/L) × FINS (IU/mL)/22.5 = HOMA-IR. IR was considered when HOMA-IR value exceeded 2.4 [Citation8]. Sex hormone binding globulin (SHBG), dehydroepiandrosterone (DHEA), and high molecular weight adiponectin (HMWA) were measured using electro-chemiluminescence, liquid chromatography-mass spectrometry/mass spectrometry (LC-MS/MS), and chemiluminescence technique, respectively. FAI was assessed using the following formula: T (nmol/L) × 100/SHBG (nmol/L) = FAI. A patient with FAI above 4.5% was considered to have a high androgen hormone level [Citation9]. Thyroid-stimulating hormone (TSH), free thyroxine (FT4), and free triiodothyronine (FT3) levels were measured using the chemiluminescence technique. Blood lipids, including total cholesterol (TC), triglyceride (TG), high-density lipoprotein cholesterol (HDL-C), and low-density lipoprotein cholesterol (LDL-C), were analyzed using the enzymatic analysis technique.
ER-associated indicators, including endometrial thickness (EMT), blood flow resistance index (RI) of the uterine artery and uterine artery S/D, were assessed in luteal phase using the Doppler ultrasound.
Statistical method
The SPSS17.0 software was used to analyze the data. The normality test (Shapiro-Wilk test) and homogeneity of variance test (Levene’s test) were performed on the data. Normally distributed measured data are expressed as mean ± standard deviation , and one-way analysis of variance (one-way ANOVA) was used for comparison between the groups. Non-normally distributed measured data are expressed as the median (P25, P75), with inter-group comparisons conducted using the Mann-Whitney U test. The Pearson’s correlation coefficient was used to conduct a correlation analysis. A statistically significant difference was considered when the value of p was less than .05 (p < .05).
Results
Symptoms and physical signs of the OP, NP, ON, and NN groups
The clinical and biochemical characteristics of the 319 study participants were analyzed. Among them, a total of 255 women (average age: 24.59 years, range: 18–38 years) were diagnosed with PCOS according to the Rotterdam PCOS diagnostic criteria, of which 99% (253/255) experienced abnormal menstruation, 73% (187/255) manifested signs of hirsutism and/or acne, 73% (187/255) had hirsutism, 52% (133/255) had acne, 43% (110/255) had elevated total serum T levels, and 72% (183/255) exhibited changes toward polycystic ovaries. The control groups, referred to as the non-PCOS groups, comprised of 64 women (average age: 30.84 years, range: 18 to 44 years). Among them, 27% (17/64) experienced abnormal menstruation, 11% (7/64) manifested signs of hirsutism and/or acne, 9% (6/64) had hirsutism, 8% (5/64) had acne, 9% (6/64) had elevated total serum T levels, and 13% (8/64) exhibited changes toward polycystic ovaries ().
Table 1. Symptoms And physical signs in the OP, NP, ON, and NN groups.
Variations in sex hormone-associated indicators in the OP, NP, ON, and NN groups
The OP group exhibited higher levels of FAI and AMH than the NP group, with statistically significant differences (p < .01, ), and exhibited lower levels of SHBG and DHEA than the NP group, with statistically significant differences (p < .01, ). There were no statistically significant differences in T, LH, FSH, and LH/FSH between the groups (p > .05, ).
Table 2. Changes in sex hormone-associated indicators in the OP, NP, ON, and NN groups .
Variations in metabolism-associated indicators in the OP, NP, ON, and NN groups
Compared with the NP group, the OP group exhibited higher levels of FINS, 60minINS, 120minINS, 180minINS, FBG, 2hGlu, HOMA-IR, TG, HDL-C, LDL-C, WHR, waist circumference, and hip circumference, with statistically significant differences (p < .01, ), while exhibiting lower levels of HMWA, with a statistically significant difference (p < .01, ). There was no statistically significant difference in TC (p > .05, ).
Table 3. Changes in metabolism-associated indicators in the OP, NP, ON, and NN groups .
Variations in ER-associated indicators in the OP, NP, ON, and NN groups
The OP group exhibited higher levels of uterine artery S/D and RI in comparison to the NP group, with statistically significant differences (p < .01, ). There was no statistically significant difference in EMT (p > .05, ).
Table 4. Changes in ER-associated indicators in the OP, NP, ON, and NN groups .
Correlation between metabolism-associated indicators to FAI and SHBG
In the PCOS groups, FAI exhibited a significantly positive correlation with FINS, 120minINS, FBG, 2hGlu, HOMA-IR, TG, LDL-C, and WHR (p < .01, ), while presenting a significantly negative correlation with HDL-C and HMWA (p < .001, ). FAI was significantly positively correlated with HMWA in both OP (p = .012, ) and NP group (p < .001, ).
Table 5. Correlation between FAI (%) and metabolism-associated indicators.
In the PCOS groups, SHBG exhibited a significantly negative correlation with FINS, 120minINS, FBG, 2hGlu, HOMA-IR, TG, LDL-C, and WHR (p < .01, ), while presenting a significantly positive correlation with HDL-C (p < .01, ). In the NP group, SHBG was significantly negatively correlated with HMWA (p < .001, ).
Table 6. Correlation between SHBG and metabolism-associated indicators.
Correlation of sex hormone-associated and metabolism-associated indicators to ER-associated indicators—RI and uterine artery S/D
In the PCOS groups, RI exhibited a significantly positive correlation with FAI, FINS, 120minINS, HOMA-IR, and WHR (p < .05, ), while presenting a significantly negative correlation with SHBG, HDL-C, and HMWA (p < .05, ). In the NP group, RI was significantly positively correlated with 120minINS (p < .05, ).
Table 7. Correlation between RI with sex hormone-associated and metabolism-associated indicators.
In the PCOS groups, uterine artery S/D exhibited a significantly positive correlation with FAI, FINS, 2hGlu, HOMA-IR, LDL-C, and WHR (p < .01, ), as well as a significantly positive correlation with 120minINS and FBG (p < .05, ), while presenting a significantly negative correlation with SHBG and HMWA (p < .01, ). In the NP group, uterine S/D was significantly positively correlated with HDL-C (p = .008, ).
Table 8. Correlation between uterine artery S/D with sex hormone-associated and metabolism-associated indicators.
Discussion
Recent studies have provided ample proof that ER is affected by hormonal abnormalities and altered metabolism in patients with PCOS [Citation5]. A reduction in ER is one of the important causes of infertility. However, it has never received as much attention as ovulation dysfunction. To develop a theoretical framework for enhancing ER in patients with PCOS at an early stage, differences in metabolism-associated and sex hormones-associated indicators between obese and non-obese women with PCOS were compared in this study. We also preliminary analyzed the correlation between these indicators and ER-associated indicators, including EMT, RI and uterine artery S/D.
Variations in the levels of sex hormones in the OP and NP groups
Shi et al. [Citation10] observed that the obese PCOS group exhibited lower levels of LH, FSH, and SHBG and higher levels of FAI compared to the non-obese PCOS groups, with no evident differences in AMH and T. In another study conducted by Layegh et al. [Citation3] the obese PCOS group exhibited lower levels of DHEA compared to the non-obese PCOS groups, with no evident difference in T. In the current study, the changes of DHEA, SHBG, FAI and T in obese PCOS patients were consistent with the results of previous studies. However, our study found that there was no significant difference in LH, FSH and LH/FSH between obese and non-obese PCOS groups, which may be due to abnormal LH secretion and increased LH/FSH ratio in PCOS patients. Hyperandrogenemia inhibited the feedback of estrogen and progesterone to LH pulse release, and there was no significant difference in T between the two groups.
Variations in metabolism-associated indicators in the OP and NP groups
XBP1 levels were significantly higher in PCOS patients, particularly in overweight/obese PCOS patients, than in the controls. According to research conducted by Shi et al. [Citation10] it was discovered that the obese PCOS group exhibited higher levels of FINS, 60minINS, 120minINS, 180minINS, HOMA-IR, CHO, TG, LDL-C, and lower levels of HDL-C, in comparison to the non-obese PCOS group. Shirazi et al. [Citation11] reported that the obese PCOS group exhibited lower levels of HMWA than the non-obese PCOS group. IR existed in 72.2% of non-obese and 80.3% of obese individuals. Although there was no significant difference in IR, obese women presented a higher average value of HOMA-IR. Wang et al. [Citation12] reveled that there was no evident difference in HMWA between the obese and non-obese PCOS groups. There has been no consensus as to whether there is a difference in serum adiponectin between obese and non-obese PCOS populations [Citation13]. Our results were consistent with previous findings. In addition, the obese PCOS group exhibited significantly higher levels of FBG and 2hGlu. Moghetti et al. [Citation14] mentioned that IR and HA were two main links in the pathogenesis of PCOS. In our study, the difference in HOMA-IR between obese and non-obese PCOS groups suggests that different PCOS groups have different pathogenic pathways, and the specific mechanisms remain to be further studied. Bahçeci et al. [Citation15] demonstrated that X-box binding protein-1 (XBP1) levels were significantly higher in PCOS patients, particularly in overweight/obese PCOS patients, than in the controls. XBP1 is a critical transcription factor in the endoplasmic reticulum and has a vital role in glucose metabolism and lipid regulation in mammals. Also, the parameters associated with metabolic syndrome were related to XBP1 levels. It was speculated that endoplasmic reticulum stress could be associated with obesity.
Correlation between metabolism-associated indicators and sex hormones in the OP and NP groups
The increase in INS concentration in patients with PCOS lowers the serum SHBG levels, thereby increasing the bioavailability of free testosterone. In addition, these women had abnormal concentrations of gonadotropins. Under high-level INS stimulation, there is a significant increase in androgen synthesis in the adrenal glands and ovaries [Citation16]. In a previous study, the findings showed that in obese patients with PCOS, SHBG and HMWA significantly decreased, INS levels were significantly correlated with FAI, and there was no correlation between FAI and BMI or between FAI and HMWA [Citation11]. According to the research of Liou et al. [Citation17] obese women with PCOS had more severe ovulation dysfunction and a higher level of total serum T; in women with PCOS, BMI was significantly positively correlated with total serum T and significantly negatively correlated with total serum LH. Shi et al. [Citation10] mentioned that FAI was positively correlated with HOMA-IR in both the obese and non-obese PCOS groups, and FAI was negatively correlated with SHBG. Additionally, HOMA-IR was negatively correlated with SHBG. Cengzi et al. [Citation18] showed a significant positive correlation between 2hGlu and AMH in adolescent obese patients with PCOS than in controls. In this study, HOMA-IR in PCOS groups is significantly positively correlated with FAI and negatively correlated with SHBG, which is consistent with the results of previous studies. Obesity and hyperinsulinemia inhibit hepatic SHBG secretion, leading to increased concentrations of free androgens. Under the action of high androgen, muscle utilization of INS-mediated glucose is significantly reduced, thus reducing insulin sensitivity and aggravating IR. Hyperinsulinemia directly acts on the INS receptors on the ovarian membrane cells and affects follicle development. IR selectively affects tissue energy metabolism, increases the sensitivity of ovarian membrane cells to LH response, and induces cytochrome P450 synergies to increase androgen synthesis and secretion [Citation19].
Variations in ER-associated indicators in the OP and NP groups
There are currently few reports on ER in PCOS-afflicted obese and non-obese individuals. Endometrial thickness (EMT), endometrial volume, endometrial pattern, endometrial blood flow, RI and uterine S/D are some of the endometrial applications of ultrasound in the field of ER [Citation20]. EMT has a positive correlation with the prevalence of pregnancy, according to certain studies [Citation21]. The presence of blood supply disorders can cause slow proliferation of the endometrial layer, decreased uterine receptivity, and inability to provide strong conditions for embryo implantation. In other words, a lower RI of the endometrial artery provides a better environment for the remodeling and regeneration of blood vessels during the embryo implantation period. We found that the obese PCOS group had higher RI and uterine S/D than the non-obese PCOS group. This may be related to the increase in inflammatory factors caused by obesity. Elci et al. [Citation22] reveled that serum CRP level was significantly elevated in obese and non-obese women with PCOS in comparison to control subjects. This elevation was more obvious in the obese PCOS group than in the other group. Guney et al. [Citation23] demonstrated that endotrophin level was significantly higher in PCOS patients. Endotrophin can increase the proinflammatory cytokines and causes insulin resistance and also associated with pathologies such as diabetes mellitus, atherosclerosis, metabolic syndrome. In addition, Comstock et al. [Citation19] claimed that obesity changes gene expression involved in implant-related chemokine signaling pathways during implantation. Obese women present significantly different endometrial gene expression from the control group, which is more obvious when PCOS or infertility is associated.
Correlation of sex hormone and metabolism-associated indicators with ER-associated indicators in the OP and NP groups and its possible mechanisms
It is reported that IR, HA, and obesity are capable of altering ER in patients with PCOS [Citation12]. In the current study, RI and uterine artery S/D, indicators associated with ER, exhibited significantly correlation with sex hormone and metabolism-associated indicators in patients with PCOS. The possible mechanisms for these correlations are as follows: Patients with PCOS experience a strong unfavorable impact from excess androgen on endometrial blood flow. Vasoconstrictors like androgen, lower uterine blood flow [Citation24]. There is a correlation between vascular resistance and serum T levels. As it is generally known, androgen receptors (AR) are widely distributed in the uterine glands, luminal epithelium, and stroma [Citation25]. As androgens bind with AR, ER is decreased, resulting in the failure of embryo implantation. The Wilms tumor suppressor gene (WT1) is expressed by endometrial stromal cells during the embryo implantation window [Citation26]. WT1 decreases as AR increases. There may be a possible correlation between ER impairment in women with PCOS-associated infertility and the HA-WT1 pathway [Citation27]. IR increases the availability of FAI, which affects the functions of granular cells and follicular development [Citation28]. In human endometrial stromal cells, over expressed tumor necrosis factor-alpha (TNF-α) negatively affects insulin sensitivity by inhibiting adiponectin signaling and blocking the translocation of glucose transporter-4 (GLUT-4) to the membrane, resulting in aberrant energy metabolism [Citation29]. This may be one of the causes of impaired ER in patients with PCOS. GLUT is currently also referred to as the SLC2A family. A possible explanation for reduction in SLC2A4 in obese patients with PCOS is that reduction in the expression of endometrial IR and SLC2A4 lead to reduction in glucose translocation, resulting in abnormal cellular glucose utilization, and ultimately abnormal endometrial differentiation and embryo implantation [Citation30]. Therefore, it appears that the expression of SLC2A4 in endometrial cells is influenced by obesity and INS independently, which is more evident than the HA status of PCOS [Citation31]. According to Chang et al. [Citation32] IR affects endometrial functions and the embryo implantation process. The mammalian target of rapamycin (mTOR) signal, which is regulated by INS, is crucial for the metabolism of glucose and lipids in skeletal muscles and liver [Citation33]. It has been proven by previous research that mTOR is of vital importance for ER [Citation27]. INS may play an important role in the endometrium. Women with PCOS, obesity, or type 2 diabetes mellitus are frequently subjected to hyper-insulinemia and IR for a long term. Despite the fact that embryos can be implanted in the endometrium, existing research has shown that genes associated with ER, including estrogen receptor (Esr) 1, progesterone receptor (Pgr), Hoxa10, and Esr 2, were disorderly expressed in maternal mouse with hyper-insulinemia. Therefore, hyper-insulinemia in maternal individuals may alter the process of ER establishment [Citation34]. The mTOR signal may impair ER in maternal bodies during the periods of hyper-insulinemia and IR. It has been reported that serum INS levels can directly change the expression of ER-associated markers. Adiponectin is one of the biological markers of ER [Citation35]. Obesity can exacerbate low-grade chronic inflammation [Citation36], and lead to reduction in serum SHBG levels [Citation37], thereby increasing the severity of HA. Additionally, obesity contributes to an excessive accumulation of IR and AGEs [Citation36,Citation38]. Although the precise mechanisms of endometrial inflammation caused by HA and IR are yet unknown, in vitro and in vivo research have revealed that several endometrial cell lines and patients with PCOS have deregulated levels of inflammatory-associated markers.
In summary, patients diagnosed with PCOS suffer from metabolic and sex hormone secretion disorders. Obese individuals with PCOS exhibit more pronounced symptoms, characterized by decreased levels of SHBG and HMWA, and elevated levels of FAI, HOMA-IR, and WHR. There is currently insufficient evidence to demonstrate that metabolic and sex hormone disorders in patients with PCOS affect ER function. Hence, a lack of consensus has been seen in this regard. In this study, it was discovered that FAI, FINS, 120minINS, HOMA-IR, WHR, SHBG, and HMWA are significantly correlated with ER-associated indicators—RI and uterine artery S/D. This may lead to impairment in embryo implantation and adverse pregnancy outcomes, hence, controlling metabolic disorders at early stages is of great importance.
To advance the development of targeted therapeutic approaches and enhance pregnancy outcomes for individuals diagnosed with PCOS, further investigation into the potential mechanisms responsible for ER impairment stemming from metabolic disturbances is imperative.
Research limitations
There are some limitations in this study. First, uterine artery is the blood vessel that supplies the entire uterus, so its blood flow parameters cannot most accurately reflect the blood supply status of the endometrial region. Second, ER refers to a series of corresponding changes in the endometrium that enable embryo implantation, which is a complex process, and only ultrasound and Doppler parameters cannot comprehensively and accurately evaluate the receptive state of the endometrium. Moreover, ultrasound examination is subjective and influenced by different examiners and equipment. While this study is only a preliminary discussion. Further explorations may confirm our findings by evaluating combination of histopathology, molecular biology and etc. Third, unfortunately, our analysis indicators did not include ovulation status and pregnancy outcomes, which will provide the most direct ER evaluation.
Conclusion
In this study, we analyzed the differences in the metabolic indicators and sex hormones between obese and non-obese patients with PCOS, and their effect on ER. The results showed that obese patients with PCOS had obvious metabolic disorders and poor ER, which manifested as lower levels of SHBG and HMWA, and higher levels of FAI, HOMA-IR, WHR, uterine artery S/D, and RI. In patients with PCOS, FAI, FINS, 120minINS, HOMA-IR and WHR were significantly positively correlated with ER-associated indicators—RI and uterine artery S/D. Conversely, there is a significantly negative correlation between these indicators and SHBG and HMWA. Therefore, controlling metabolic disorders at an early stage is a significant contributor in improving pregnancy outcomes. The ultimate goal is to create targeted therapeutic plans that can enhance pregnancy outcomes for patients diagnosed with PCOS.
Author contributions
Xiao-li Li and Shi-wei Liu conceived the idea and conceptualized the study. Yan-fei Ji and Yu Feng collected the data. Xiao-li Li and Yan-fei Ji analyzed the data. Yan-fei Ji and Yu Feng did statistical analysis. Xiao-li Li and Yan-fei Ji drafted the manuscript, then Xiao-li Li and Shi-wei Liu reviewed the manuscript. All authors read and approved the final draft.
Ethics approval and consent to participate
I confirm that I have read the Editorial Policy pages. This study was conducted with approval from the Ethics Committee of Shanxi Bethune Hospital. This study was conducted in accordance with the declaration of Helsinki. Written informed consent was obtained from all participants.
Acknowledgements
We would like to acknowledge the hard and dedicated work of all the staff that implemented the intervention and evaluation components of the study.
Disclosure statement
No potential conflict of interest was reported by the author(s).
Data availability statement
The data used to support the findings of this study are available from the corresponding author upon request.
Additional information
Funding
References
- Ortiz-Flores AE, Luque-Ramírez M, Escobar-Morreale HF. Polycystic ovary syndrome in adult women. Med Clin. 2019;152(11):1–8. doi:10.1016/j.medcli.2018.11.019.
- Zeng X, Xie YJ, Liu YT, et al. Polycystic ovarian syndrome: correlation between hyperandrogenism, insulin resistance and obesity. Clin Chim Acta. 2020;502:214–221. doi:10.1016/j.cca.2019.11.003.
- Layegh P, Mousavi Z, Farrokh Tehrani D, et al. Insulin resistance and endocrine-metabolic abnormalities in polycystic ovarian syndrome: comparison between obese and non-obese PCOS patients. IJRM. 2016;14(4):263–270. doi:10.29252/ijrm.14.4.263.
- Couto Alves A, Valcarcel B, Mäkinen VP, et al. Metabolic profiling of polycystic ovary syndrome reveals interactions with abdominal obesity. Int J Obes. 2017;41(9):1331–1340. doi:10.1038/ijo.2017.126.
- Ejzenberg D, Gomes TJO, Monteleone PAA, et al. Prognostic factors for pregnancy after intrauterine insemination. Int J Gynaecol Obstet. 2019;147(1):65–72. doi:10.1002/ijgo.12898.
- The Asia-Pacific perspective: redefining obesity and its treatment. Melbourne: international Diabetes Institute, 2000.
- Rotterdam ESHRE/ASRM-Sponsored PCOS Consensus Workshop Group. Revised 2003 consensus on diagnostic criteria and long-term health risks related to polycystic ovary syndrome. Fertil Steril. 2004;81(1):19–25. doi:10.1016/j.fertnstert.2003.10.004.
- Soonthornpun S, Setasuban W, Thamprasit A, et al. Novel insulin sensitivity index derived from oral glucose tolerance test. J Clin Endocrinol Metab. 2003;88(3):1019–1023. doi:10.1210/jc.2002-021127.
- Chang WY, Knochenhauer ES, Bartolucci AA, et al. Phenotypic spectrum of polycystic ovary syndrome: clinical and biochemical characterization of the three major clinical subgroups. Fertil Steril. 2005;83(6):1717–1723. doi:10.1016/j.fertnstert.2005.01.096.
- Shi W, Zhao Q, Zhao X, et al. Analysis of endocrine and metabolic indexes in non-obese patients with polycystic ovary syndrome and its compare with obese patients. Diabetes Metab Syndr Obes. 2021;14:4275–4281. doi:10.2147/DMSO.S329108.
- Shirazi FKH, Khodamoradi Z, Jeddi M. Insulin resistance and high molecular weight adiponectin in obese and non-obese patients with Polycystic Ovarian Syndrome (PCOS). BMC Endocr Disord. 2021;21(1):45. doi:10.1186/s12902-021-00710-z.
- Wang C, Wen YX, Mai QY. Impact of metabolic disorders on endometrial receptivity in patients with polycystic ovary syndrome. Exp Ther Med. 2022;23(3):221. doi:10.3892/etm.2022.11145.
- Wickham EP, 3rd, Cheang KI, Clore JN, et al. Total and high-molecular weight adiponectin in women with the polycystic ovary syndrome. Metabolism. 2011;60(3):366–372. doi:10.1016/j.metabol.2010.02.019.
- Moghetti P, Tosi F, Bonin C, et al. Divergences in insulin resistance between the different phenotypes of the polycystic ovary syndrome. J Clin Endocrinol Metab. 2013;98(4):E628–E637. doi:10.1210/jc.2012-3908.
- Bahçeci E, Kaya C, Karakaş S, et al. Serum X-box-binding protein 1 levels in PCOS patients. Gynecol Endocrinol. 2021;37(10):920–924. doi:10.1080/09513590.2021.1942449.
- De Leo V, Musacchio MC, Cappelli V, et al. Genetic, hormonal and metabolic aspects of PCOS: an update. Reprod Biol Endocrinol. 2016;14(1):38. doi:10.1186/s12958-016-0173-x.
- Liou TH, Yang JH, Hsieh CH, et al. Clinical and biochemical presentations of polycystic ovary syndrome among obese and nonobese women. Fertil Steril. 2009;92(6):1960–1965. doi:10.1016/j.fertnstert.2008.09.003.
- Cengiz H, Ekin M, Dagdeviren H, et al. Comparison of serum anti-Müllerian hormone levels in normal weight and overweight-obese adolescent patients with polycystic ovary syndrome. Eur J Obstet Gynecol Reprod Biol. 2014;180:46–50. doi:10.1016/j.ejogrb.2014.06.018.
- Comstock IA, Diaz-Gimeno P, Cabanillas S, et al. Does an increased body mass index affect endometrial gene expression patterns in infertile patients? A functional genomics analysis. Fertil Steril. 2017;107(3):740–748.e2. doi:10.1016/j.fertnstert.2016.11.009.
- Bonilla-Musoles F, Raga F, Osborne NG, et al. Endometrial receptivity: evaluation with ultrasound. Ultrasound Q. 2013;29(1):3–20. doi:10.1097/RUQ.0b013e318281b60a.
- Nishihara S, Fukuda J, Ezoe K, et al. Does the endometrial thickness on the day of the trigger affect the pregnancy outcomes after fresh cleaved embryo transfer in the clomiphene citrate-based minimal stimulation cycle? Reprod Med Biol. 2020;19(2):151–157. doi:10.1002/rmb2.12315.
- Elci E, Kaya C, Cim N, et al. Evaluation of cardiac risk marker levels in obese and non-obese patients with polycystic ovaries. Gynecol Endocrinol. 2017;33(1):43–47. doi:10.1080/09513590.2016.1203893.
- Guney G, Taskin MI, Baykan O, et al. Endotrophin as a novel marker in PCOS and its relation with other adipokines and metabolic parameters: a pilot study. Ther Adv Endocrinol Metab. 2021;12:20420188211049607. doi:10.1177/20420188211049607.
- Lam P, Johnson I, Raine-Fenning N. Endometrial blood flow is impaired in women with polycystic ovarian syndrome who are clinically hyperandrogenic. Ultrasound Obstet Gynecol. 2009;34(3):326–334. doi:10.1002/uog.7314.
- Chen X, He J, Ding Y, et al. The role of MTOR in mouse uterus during embryo implantation. Reproduction. 2009;138(2):351–356. doi:10.1530/REP-09-0090.
- Gonzalez D, Thackeray H, Lewis PD, et al. Loss of WT1 expression in the endometrium of infertile PCOS patients: a hyperandrogenic effect? J Clin Endocrinol Metab. 2012;97(3):957–966. doi:10.1210/jc.2011-2366.
- Younas K, Quintela M, Thomas S, et al. Delayed endometrial decidualisation in polycystic ovary syndrome; the role of AR-MAGEA11. J Mol Med (Berl). 2019;97(9):1315–1327. doi:10.1007/s00109-019-01809-6.
- Glueck CJ, Goldenberg N. Characteristics of obesity in polycystic ovary syndrome: etiology, treatment, and genetics. Metabolism. 2019;92:108–120. doi:10.1016/j.metabol.2018.11.002.
- Oróstica L, García P, Vera C, et al. Effect of TNF-α on molecules related to the insulin action in endometrial cells exposed to hyperandrogenic and hyperinsulinic conditions characteristics of polycystic ovary syndrome. Reprod Sci. 2018;25(7):1000–1009. doi:10.1177/1933719117732157.
- Zhai J, Liu CX, Tian ZR, et al. Effects of metformin on the expression of GLUT4 in endometrium of obese women with polycystic ovary syndrome. Biol Reprod. 2012;87(2):29. doi:10.1095/biolreprod.112.099788.
- Mozzanega B, Mioni R, Granzotto M, et al. Obesity reduces the expression of GLUT4 in the endometrium of normoinsulinemic women affected by the polycystic ovary syndrome. Ann N Y Acad Sci. 2004;1034(1):364–374. doi:10.1196/annals.1335.038.
- Chang EM, Han JE, Seok HH, et al. Insulin resistance does not affect early embryo development but lowers implantation rate in in vitro maturation-in vitro fertilization-embryo transfer cycle. Clin Endocrinol. 2013;79(1):93–99. doi:10.1111/cen.12099.
- Sharawy MH, El-Awady MS, Megahed N, et al. Attenuation of insulin resistance in rats by agmatine: role of SREBP-1c, mTOR and GLUT-2. Naunyn Schmiedebergs Arch Pharmacol. 2016;389(1):45–56. doi:10.1007/s00210-015-1174-6.
- Li R, Wu J, He J, et al. Mice endometrium receptivity in early pregnancy is impaired by maternal hyperinsulinemia. Mol Med Rep. 2017;15(5):2503–2510. doi:10.3892/mmr.2017.6322.
- Wang Y, Xie X, Zhu W. Serum adiponectin and resistin levels in patients with polycystic ovarian syndrome and their clinical implications. J Huazhong Univ Sci Technolog Med Sci. 2010;30(5):638–642. doi:10.1007/s11596-010-0556-8.
- Riley JK, Jungheim ES. Is there a role for diet in ameliorating the reproductive sequelae associated with chronic low-grade inflammation in polycystic ovary syndrome and obesity? Fertil Steril. 2016;106(3):520–527. doi:10.1016/j.fertnstert.2016.07.1069.
- Motta AB. The role of obesity in the development of polycystic ovary syndrome. Curr Pharm Des. 2012;18(17):2482–2491. doi:10.2174/13816128112092482.
- Liao Y, Huang R, Sun Y, et al. An inverse association between serum soluble receptor of advanced glycation end products and hyperandrogenism and potential implication in polycystic ovary syndrome patients. Reprod Biol Endocrinol. 2017;15(1):9. doi:10.1186/s12958-017-0227-8.